| Bottom | | Front page |
Environmental Project no. 899, 2004
Degradation of Estrogens in Sewage Treatment Processes
Contents
List of Abbreviations
Summary and conclusions
Sammenfatning og konklusioner
1 Introduction
2 Sewage treatment in Denmark
3 Estrogens in sewage and sewage treatment processes
4 Experimental Programme
5 Results and discussion
6 General discussion and conclusions
7 References
Appendix 1: Instrumentation details
Appendix 2: Batch reactor characterisation
List of Abbreviations
AE2 |
E2-acetate |
BOD5 |
Biochemical Oxygen Demand during 5-days test |
COD |
Chemical Oxygen Demand |
CS |
Concentration of a compound in the solid phase (i.e. sludge) |
CW |
Concentration of a compound in the water phase |
DEPA |
Danish Environmental Protection Agency |
DFU |
Danish University of Pharmaceutical Sciences |
DS |
Dry Solids |
E1 |
Estrone (natural steroid hormone, metabolite of E2) |
E1-3Glu |
Estrone conjugated with glucuronic acid at the 3-hydroxy position |
E1-3Sul |
Estrone conjugates with sulphate at the 3-hydroxy position |
E2 |
17β-estradiol (natural steroid hormone) |
E3 |
Estriol (natural steroid hormone, metabolite of E2) |
EE2 |
17α-ethinylestradiol (artificial steroid hormone) |
EEQ |
E2 Estrogenicity Equivalents |
HRT |
Hydraulic Retention Time |
k |
Reaction rate constant (for the degradation of estrogens) |
Kd |
Equilibrium constant i.e. the observed distribution ratio of a compound between solid and water phase at equilibrium |
KF |
The Freundlich equilibrium constant (the equilibrium constant in the Freundich equation describing the adsorption process) |
KOW |
The equilibrium constant describing the distribution ratio of a compound between n-octanol and water at equilibrium. |
LC |
Liquid Chromatograph/Liquid Chromatography |
LOEC |
Lowest Observed Effect Concentration |
MeEE2 |
Methyl-EE2 |
MLSS |
Mixed Liquid Suspended Solids |
MS |
Mass Spectrometer/Mass Spectrometry |
N |
Nitrogen |
NERI |
National Environmental research Institute (of Denmark) |
NPO |
Nitrogen, Phosphorus and Organic matter |
O |
Oxygen |
P |
Phosphorus |
PE |
Person Equivalents (= 60 gram of BOD5 per day) |
RESS |
Removal of Excess Suspended Solids |
SPE |
Solid Phase Extraction |
SRT |
Sludge Retention Time |
SS |
Suspended Solids |
STP |
Sewage Treatment Plant |
T½ |
Half-life i.e. the time it takes to remove/degrade 50% of the start concentration of a compound |
TS |
Total Solids |
VMP |
VandMiljøPlan (Water Environment Action Plan) |
VSS |
Volatile Suspended Solids |
Summary and conclusions
Scientific and public concern about the possible impacts of steroid hormones (estrogens) being released into the aquatic environment has been increasing in the last few years. Public sewage treatment plants
(STPs) are believed to be a major source of discharge of these substances, but knowledge about the specific processes regulating the fate in STPs is still sparse.
In recognition of this, the Danish Environmental Protection Agency (DEPA) has funded the present project, which has the objective to generate more knowledge about the ability of the key treatment
processes at typical, modern Danish STPs (i.e. sorption and degradation in the activated sludge stage) to remove estrogens from the water phase.
The report mainly presents the documentation and results of the experimental laboratory work performed, but two literature based introductory chapters describe the present situation regarding treatment of
public sewage in Denmark and the present knowledge about estrogens in sewage and their fate in STPs, respectively.
Most of the 1,267 Danish STPs bigger than 30 PE have been upgraded significantly since 1987 when the first Water Environment Action Plan was launched by the Danish Parliament. The plan included
more strict emission standards for nutrients and organic matter for STPs > 5,000 PE and, thus, rendered technological upgrading of the majority of Danish STPs necessary.
Today, about one fifth of the STPs treat almost 90% of the total volume of sewage in Denmark. Typically, these plants have mechanical treatment and biological treatment including removal of nitrogen and
organic matter in activated sludge systems, a chemical precipitation step and finally settling of suspended particles in a clarifier tank (MBNDK plants). Many are, in addition to this, equipped with a filter or
lagoon after the settling step.
The STP delivering the activated sludge for the main part of the experimental work, Egå STP near the city of Århus, is fairly representative of the MBNDK type of STPs in Denmark. The designed treatment
capacity of the plant is 90,000 PE and the effluent quality with respect to general parameters is somewhat better than the average indicating that Egå is a well-run STP.
The substances chosen for the experimental study were the natural estrogens estrone (E1) and 17β-estradiol (E2), and the synthetic estrogen used in most contraceptive pills, 17α-ethinylestradiol (EE2).
These are the substances exhibiting the highest estrogenic activity. In addition to these, two conjugates of E1 (a glucuronide- and a sulphate conjugate; E1-3GLU and E1-3SUL) were included as estrogens
are excreted from the human body and enter the sewage systems as conjugates. The conjugates possess in themselves virtually no estrogenic activity but they can be transformed back into the free, active
form in the sewage system.
The studies published of the fate of estrogens in STPs are new and relative few, but they indicate that the primary treatment step has only very limited effect while the main removal of estrogens take place in
the activated sludge system. E2 disappears quickly (is transformed into E1) while the removal of E1 takes place a little more slowly and more variably, and the rate by which EE2 is removed is even lower.
Conjugates, in particular the glucuronides, are cleaved easily in sewage systems to produce the free, active forms of the estrogens. Based on the literature, a long sludge retention time appears to improve the
treatment efficiency.
The experimental study comprised sorption experiments with free estrogens using sludge from two different STPs (Egå and Lundtofte), testing of the abiotic stability in sterile water, and the degradation under
aerobic and denitrifying conditions, respectively (conjugates only aerobically).
The adsorption isotherm was determined using a modified version of the US EPA procedure while biodegradation experiments were performed in glass reactors with 0.50 g of activated sludge/L being fed
daily with artificial peptone sewage according to OECD. To maintain homogeneity and aerobic conditions in the reactors, oxygen was added via an inlet in the bottom of the reactor. In the anaerobic
experiments the oxygen was replaced by nitrogen but otherwise the set-up was similar to the aerobic experiment.
The sorption experiments showed that the substances were sorbed almost quantitatively to the sludge within only about 0.5 hour. The (logarithmic) sorption equilibrium constant, Log Kd, was about 2.6 for
E2 and EE2 in both sludges tested while for E1 it was 2.8 in Egå sludge and 2.3 in Lundtofte sludge. The latter result is most consistent with observations found in the literature i.e. slightly lower than for E2
and EE2. The results indicate that at common sludge densities in Danish STPs about 35-45% of E1 and 55-65% of E2 and EE2 can be expected to be sorbed to sludge in the aeration tanks.
The test of abiotic stability in water revealed that practically no degradation took place under sterile conditions and, hence, any observed degradation can be attributed to the action of microorganisms
including enzymatic reactions.
The aerobic degradation was very fast for E1 and E2 i.e. with half-lives of a few minutes or even lower at sludge concentrations commonly found at STPs, while the half-life of EE2 was more than 100
times higher i.e. from 1.4 hours to 11 hours, depending on the sludge concentration (fastest degradation at highest concentration). The glucuronide conjugate of E1, E1-3GLU, was transformed at a slightly
slower rate than E1/E2 i.e. with a half-life of 2-16 minutes, whereas the transformation of the sulphate conjugate, E1-3SUL, took place at a rate more resembling that of EE2. In conclusion, the observed
order of aerobic degradability was: E2 > E1 > E1-3Glu >> EE2 > E1-3Sul.
Under anaerobic conditions in the activated sludge, the degradation rates for E1 and EE2 were considerably (10-20 times) lower than under aerobic conditions while the degradation of E2 was not
significantly changed i.e. still in the order of few minutes or lower depending on sludge concentration.
The measured degradation rate constants were used to estimate the stability
of steroid estrogens in Egå STP using the actual hydraulic retention times
in the activated sludge compartments. The estimation predicted that more than 99.9% of E1, E2 and E1-3Glu in the sewage would be removed while about 3.3 % of EE2 would still remain in the effluent. It
was not possible to predict the degradation rate of E1-3Sul. The predicted removal efficiencies are higher than typically observed in monitoring studies at STPs.
Sammenfatning og konklusioner
Der har i de seneste år været en stigende opmærksomhed og bekymring både blandt miljøfolk og i offentligheden omkring de mulige effekter af spredningen af hormonforstyrrende stoffer (østrogener) i
vandmiljøet. Renseanlæg for almindeligt spildevand anses for at være en sandsynlig hovedkilde til udledning af sådanne stoffer, men den tilgængelige viden om de processer i renseanlæg, der betyder noget
for fjernelsen af østrogener fra spildevand, er endnu meget begrænset.
Derfor har Miljøstyrelsen iværksat dette projekt, som har til formål at tilvejebringe ny viden om almindelige renseanlægsprocessers evne til fjerne østrogener fra spildevandet dvs. især adsorption til slam og
mikrobiologisk nedbrydning af stofferne. Rapporten beskriver især det gennemførte eksperimentelle arbejde, men giver også en generel introduktion til spildevandsrensning i Danmark samt viden fra
litteraturen om østrogener i spildevand og deres skæbne i renseanlæg.
Der var i 2002 i alt 1267 renseanlæg >30 PE i Danmark, hvoraf omkring en femtedel renser ca. 90% af det samlede spildevandsvolumen. De danske renseanlæg >5.000 PE er blevet væsentligt opgraderet
siden iværksættelsen af den første Vandmiljøplan (VMP 1) i 1987, således at de i dag kan leve op til de strengere udledningskrav for næringssalte og organisk stof, der blev indført som en del af planen. Det
typiske, større danske renseanlæg foretager i dag en indledende mekanisk rensning af spildevandet, hvorefter det underkastes biologisk behandling, herunder fjernelse af kvælstof og omsætning af organisk
stof i et aktivslam-system, og kemisk fældning af fosfor. Til sidst passerer det rensede spildevand gennem en efterklaringstank (+ evt. filter) før det ledes ud.
Slammet, der er benyttet til hovedparten af forsøgene i dette projekt, stammer fra renseanlægget i Egå ved Århus. Dette anlæg på 90.000 PE må betegnes som rimeligt repræsentativt for de større
renseanlæg i Danmark, men har dog en afløbskvalitet, der er lidt bedre end gennemsnittet.
De østrogener, der har indgået i det eksperimentelle arbejde er de naturlige østrogener østron (E1) og 17â-østradiol (E2) samt 17á-ethinyløstradiol (EE2), der er det aktive stof i de fleste p-piller. Disse tre
stoffer tegner sig i almindelighed for langt størstedelen af den østrogene aktivitet, der kan måles i spildevand. Desuden er to konjugater af E1 (et glucuronid og en sulfat) blevet undersøgt da det er på
konjugeret form at østrogener udskilles fra kroppen og ledes ud i spildevandssystemet. Konjugaterne er i sig selv ikke østrogent aktive, men kan i spildevandet eller renseanlægget blive tilbagetransformeret
til deres oprindelige, frie former (E1, E2 el. EE2).
Forskningen omkring østrogener i spildevand og renseanlæg er ret ny og der foreligger kun ret få publicerede undersøgelser om emnet. Disse indikerer imidlertid, at det indledende mekaniske rensetrin kun
har ringe effekt på østrogener, og at den væsentligste fjernelse finder sted i aktivslam-trinnet. E2 forsvinder hurtigt (omdannes til E1), mens E1 fjernes lidt langsommere og i mere varierende grad. EE2 er
noget langsommere nedbrydeligt end de naturlige østrogener. Konjugater, især glucuronider, synes at blive spaltet ret let i spildevandssystemer, således at de frie moderstoffer genopstår. En høj slamalder
synes ifølge litteraturen at have en positiv effekt på fjernelse af østrogener i renseanlæg.
Projektets eksperimentelle del omfattede sorptionsforsøg med aktivt slam fra hhv. Egå og Lundtofte renseanlæg (kun frie østrogener), dokumentation af østrogenernes abiotiske stabilitet i vand samt
nedbrydningen i aktivslam under hhv. nitrificerende (aerobe) og denitrificerende (anaerobe) betingelser (konjugater kun testet i det aerobe testsystem).
Isotermen for sorption til slam blev bestemt efter en modificeret udgave af US EPA's standardmetode, mens den mikrobielle nedbrydning i det aktive slam foregik i cylindriske 1 liters reaktorer af glas med
0,5 g aktivslam/liter og daglig tilførsel af næringsstoffer i form af kunstigt spildevand fremstillet efter OECD's standard. For at sikre en homogen blanding af slam og vand samt at opretholde aerobe
betingelser i reaktoren blev systemet kontinuert gennem-boblet med ilt via en slange monteret i bunden. De anaerobe forsøg foregik på tilsvarende vis, blot blev der her tilført kvælstof til reaktoren i stedet for
ilt.
Resultaterne af sorptionseksperimentet viste, at østrogenerne sorberede næsten kvantitativt til slammatricen i løbet af ca. en halv time. Der blev fundet en Log Kd på omkring 2,6 for både E2 og EE2 i
begge slamprøver, mens værdien for E1 var 2,8 i slam fra Egå og 2,3 i slam fra Lundtofte renseanlæg. Sidstnævnte resultat stemmer bedst overens med data fundet i litteraturen, der angiver, at sorptionen af
E1 er lidt lavere end for E2 og EE2. Resultaterne indikerer, at ved almindelige slamkoncentrationer i danske renseanlæg vil omkring 35-45% af E1 og 55-65% af E2 og EE2 sorbere til slammet.
Testen for abiotisk stabilitet viste, at der praktisk taget ikke foregik nogen nedbrydning i vand under sterile betingelser, og at eventuel nedbrydning i de biotiske systemer således kan tilskrives mikrobielt
betingede reaktioner (incl. enzymatiske reaktioner).
Den aerobe nedbrydning af E1 og E2 i aktivslammet foregik meget hurtigt, dvs. med halveringstider på få minutter ved de testede koncentrationer og endda endnu lavere ved almindeligt forekommende
slamkoncentrationer i danske renseanlæg (ca. 4 g slam/liter; beregnede halveringstider). Halveringstiden for EE2 var mere end 100 gange så høj, dvs. fra 1,4 timer ved den høje slamkoncentration (4 g/L) til
11 timer ved den testede koncentration. Glucuronidkonjugatet af E1, E1-3Glu, blev omdannet en smule langsommere end E1 og E2 (halveringstid 2-16 minutter), mens sulfatkonjugatet, E1-3Sul, blev
nedbrudt betydeligt langsommere, dvs. med en hastighed mere i retning af EE2's. Sammenfattende kan de undersøgte østrogeners aerobe nedbrydelighed i slam beskrives som: E2 > E1 > E1-3Glu >> EE2
> E1-3Sul.
Under anaerobe (denitrificerende) betingelser i det aktive slam foregik nedbrydningen af E1 og EE2 betydeligt langsommere (10-20 gange) end i det aerobe forsøg, mens nedbrydningshastigheden for E2
ikke var væsentligt forskellig, dvs. halveringstiden var også her nogle få minutter.
Ud fra de eksperimentelt bestemte hastighedskonstanter samt de hydrauliske opholdstider i aktivslam-anlægget på Egå renseanlæg er der givet et estimat på østrogennedbrydningen under virkelige forhold.
Ifølge estimatet vil mere end 99,9 % af E1, E2 og E1-3Glu blive fjernet fra spildevandet, mens 3,3 % EE2 vil kunne forekomme i udløbet. Fjernelsen af E1-3Sul kunne ikke bestemmes. De estimerede
fjernelsesgrader er højere, end hvad der normalt observeres i moniteringsstudier på renseanlæg.
1 Introduction
1.1 Background
During the last few years, there has been a growing scientific and public interest and concern about the possible impacts of female hormones (estrogens) and other so-called endocrine disruptors that are
emitted to the aquatic environment where they can cause disturbances in the sexual differentiation of fish and amphibians (typically feminisation). Public sewage treatment plants (STPs) are believed to be a
major source of estrogen release into the aquatic environment.
The Danish Minister of Environment recognises the need for more information and generation of new knowledge within this field and, therefore, the Danish Environmental Protection Agency (DEPA) has
funded and initiated a number of studies on the occurrence, fate and impacts of estrogenic substances in the (aquatic) environment, e.g. a review on feminisation in fish (DEPA 2002) and a study on the
occurrence and levels of estrogens in influents and effluents of selected Danish STPs (DEPA 2003a).
A number of studies have been published internationally during the last approximately five years, which document the presence of natural and synthetic estrogens in raw sewage as well as in the effluents from
STPs and, in very low concentrations, in receiving water bodies. However, detailed studies of the fate of these substances on their way through the different stages of sewage treatment are still sparse and
need to be understood better if estrogen removal at Danish STPs is to be improved.
1.2 Objectives
The objective of the present project is to generate more knowledge about the fate of natural and synthetic estrogens during traditional treatment processes employed at common municipal Danish STPs for
urban sewage.
The results of the investigations will provide knowledge about the ability of the key treatment processes to remove estrogens from the sewage. This knowledge can form the basis e.g. for later estimation of
the discharges of estrogens from the STPs to the aquatic environment and/or be used to assess how the design and operation of STPs can be optimised in terms of removal of these substances and thereby
minimisation of the load on the environment.
1.3 Organisation
The project has been carried out in collaboration between the Danish Pharmaceutical University (DFU) and COWI A/S. COWI has been responsible of the project management and co-ordination, and of
general descriptive and editing activities while DFU has been in charge of the experimental investigations.
The following persons have participated in the project:
DFU |
Bent Halling-Sørensen (scientifically responsible at DFU)
Henrik R. Andersen
Martin Hansen
Flemming Ingerslev |
COWI: |
Frank Stuer-Lauridsen (PM until 31 October 2003)
Jesper Kjølholt (PM from 1 November 2003)
Thomas Dueholm Blicher |
Line W. Hollesen was the project responsible person at DEPA (Office of wastewater and water supplies), and, further, a steering committee was formed for which - in addition to DEPA, COWI and DFU -
the following external persons were appointed:
Bo Neergaard Jacobsen, DANVA (Danish Water and Waste Water Association)
Gunnar Tholstrup, Office of Environment, Århus Municipality
Thomas Plesner, County of Århus.
The project was initiated in August 2003 with a kick-off meeting between DEPA, COWI and DFU. In mid September 2003 a status and progress report outlining the remaining experimental activities was
forwarded and approved by DEPA. The steering committee discussed the work and the results hereof at a meeting on 25 November 2003, and has afterwards commented on the draft final report.
The Municipality of Århus has kindly placed the Egå STP at the project team's disposal for sludge sampling and is greatly acknowledged for providing resources and transport in relation to the sampling in the
autumn of 2003.
2 Sewage treatment in Denmark
The treatment of municipal sewage in Denmark has during the last 15 years undergone significant improvements following the launching of a comprehensive national Danish Action Plan for the Water
Environment (VMP I) in 1987. A follow-up action plan, VMP II, was initiated in 1998 and a further follow-up on that, VMP III, is presently being prepared and will be commenced in 2004.
The first VMP was initiated with the specific aim of significantly reducing the loads of nitrogen, phosphorus and organic matter (NPO) on the Danish water environment that in many places (coastal waters)
up through the 1980'ies suffered from eutrofication and episodes of severe oxygen deficit with increasingly shorter intervals. One of the most important means to achieve this goal was to invest heavily in
order to build new or modernise existing municipal sewage treatment plants (STPs) that could comply with new, stricter emission limits for NPO (8 mg/L, 1.5 mg/L and 15 mg/L, respectively).
Later, when the VMP II was prepared, increasing concern about heavy metals and other chemical pollutants in the environment lead to the launching of a national water environment monitoring programme,
NOVA 2003, which, in addition to NPO, also included a large number of specific pollutants. These were in particular to be monitored at point sources. From 2004, a revised and extended version of the
programme, NOVANA, will be in force.
Still, however, the designs and technologies of Danish STPs are aimed to minimise the discharge of NPO-substances rather than handling specific chemical compounds. This chapter is intended to provide a
brief overview of the situation regarding STPs in Denmark.
2.1 Overview of STPs in Denmark
The national statistics on STPs only concern treatment plants being larger than 30 PE (1 PE is defined as 60 grams of BOD5/day), which covers the vast majority of public sewage in Denmark as about 89%
of the population is connected to such plants.
The number of STPs was 1,267 in 2002 of which more than 80% were operated by municipalities - including all the large plants - while the rest were run by private companies or organisations. The number
of STPs at the beginning of the VMP I (1989) was 1,980 i.e. more than 700 plants have disappeared in about 13 years (DEPA 2003d).
The volume of sewage passing through the Danish STPs was about 810 million m³ in 2002 compared to 720 million m³ in 2001 (DEPA 2003d and 2002a). The figures include infiltration and surface runoff,
the latter constituting about 100 million m³ in an average year (2001 is closer to the average than 2002, which was a year with much precipitation). Of this total volume, some 50-60 % was discharged
directly into marine or brackish receiving waters while the rest was discharged to streams and lakes.
The size distribution of the Danish treatment plants was in 2002 as shown in Table 2-1 (DEPA 2003d).
Table 2-1
Size distribution of Danish STPs in 2002 (DEPA 2003d)
Capacity of STP |
Number of STPs |
PE-load,% of total |
>30 PE
>500 PE
>2,000 PE
>5,000 PE
>15,000 PE
>50,000 PE
>100,000 PE |
1,267
658
441
274
130
63
30 |
100
99
98
93
83
68
48 |
The discharge of NPO-substances in 2001 was dramatically lower than in the mid 1980'ies, i.e. prior to the launching of the VMP I. Thus, the organic matter discharges (as BOD5) has been reduced by
95%, phosphorus by 92% and nitrogen by 79%. Table 2-2 below gives an overview of the NPO discharge in 1989 and 2001, respectively, and of the present (2001) average treatment efficiency of the
Danish STPs (NERI 2002).
Table 2-2
nitrogen, phosphorus and organic matter in sewage from Danish STPs in 1989 and 2001 (NERI 2002).
|
Year |
Organic matter (BOD5) |
Nitrogen (tot-N) |
Phosphorus (tot-P) |
Total amounts (t/year) |
1989 2001 |
36,400 2,550 |
18,000 4,220 |
4,470 470 |
Reduction (%) |
|
93 |
77 |
89 |
Conc., untreated (mg/L)
Conc., treated (mg/L) |
2001 2001 |
203 3.5 |
43 5.9 |
9.4 0.65 |
Treatment efficiency (%) |
|
98 |
86 |
93 |
2.2 Types of treatment technologies
The Danish STPs apply a considerable range of treatment technologies, which in terms of complexity are more or less directly proportional to their size. A simplified categorisation of 1,250 out of the total
1,267 Danish STPs larger than 30 PE is shown in Table 2-3 (based on data in DEPA 2003d. Specifications for 17 plants are missing).
Table 2-3
Overview of main treatment technologies at Danish STPs and their relative share of the volume of treated sewage.
STP Category |
No. of plants |
Share of sewage
volume (%) |
A. MBND/MBNDK |
300 |
88.4 |
B. MBN/MBNK |
362 |
8.7 |
C. MB/MBK |
171 |
1.7 |
D. M/MK |
302 |
0.8 |
E: Wetland treatment |
59 |
0.3 |
F. Biological sandfilters |
56 |
0.1 |
Total |
1,250 |
100.0 |
M: Mechanical treatment
B: Biological treatment
N: Nitrification
D: Denitrification
K: Chemical precipitation
As could be anticipated, the highest efficiency (in terms of NPO removal) is found at the most advanced plants (Category A), which, at the national level, are also the most important in terms of volume and
load (in PE) treated.
The plants in categories A-C all remove organic matter efficiently, i.e. more than 90%, while category D plants only remove some 55-70%. The figures for nitrogen removal are slightly lower, but the same
trend between the different STP categories is observed. With regard to phosphorus only the most advanced plants (category A-B) remove this element with efficiency higher than 80% while the removal in
category C plants is only some 50-60% and in category plants even lower; about 35-45%.
A typical, modern Danish STP is based on the activated sludge process. Most commonly, the plants operate according to the principle of recirculation or alternation. The following main stages will be found
at most Danish STPs:
Pre-treatment: |
Screen Grit and grease trap Primary settling (optional) |
Biological treatment: |
Anaerobic step (biological phosphorus removal)
Denitrification step (nitrate removal)
Nitrification step (ammonia and organic removal) |
Chemical treatment: |
Addition of chemicals for precipitation of phosphorus (often along with the biological treatment) |
Final treatment: |
Secondary settling
Sand filtration (option) |
Sludge treatment: |
Sludge thickening
Anaerobic digester (optional)
Sludge dewatering |
The first step in wastewater treatment is a mechanical step. The screen removes larger objects from the wastewater, whereas the grit and grease trap removes grit, grease and supernatant material. The
discarded material is usually incinerated and the residues placed on landfills. Most large STPs are, in addition to the screen and trap, also equipped with a primary settling tank.
The biological treatment consists of a denitrification step (no oxygen present) and nitrification step (oxygen present). Nitrate is converted to free nitrogen in the denitrification step while in the nitrification step,
ammonia is concerted to nitrate (via nitrite), and organic material is converted to carbon dioxide. To remove the nitrate formed in the nitrification step, the sludge is re-circulated back into the denitrification
step.
As mentioned above some STPs are operated according to the principle of alternation. With alternation, the wastewater is treated in the same tank with shift between denitrification and nitrification, whereas
operation according recirculation is moved from one tank (denitrification) to another tank (nitrification).
An anaerobic step for biological phosphorus removal is installed on many STP lately, but chemical phosphorus precipitation is still the most common method for phosphorus removal on Danish STPs.
The final step in wastewater treatment is separation (secondary settling tank) of activated sludge and treated wastewater. The treated wastewater is discharged to the receiving water.
The removed sludge is split into a fraction for sludge treatment and another fraction is transferred back to the biological step for maintaining of biological activity. Sludge treatment typically consists of sludge
thickening and sludge dewatering. Approximately 45% (sludge production) of the Danish STPs are installed with anaerobic digester for further sludge treatment (DEPA 2003c). The excess sludge is mainly
discharged for agriculture use (approximately 53% in 2001). Due to limits for heavy metals and other chemical pollutants the sludge amount for agriculture use are decreased during the last 8 years, whereas
sludge discharged to incineration and other use are increased within the same period (DEPA 2003c).
Figure 2-1 shows a typical STP design based on recirculation.
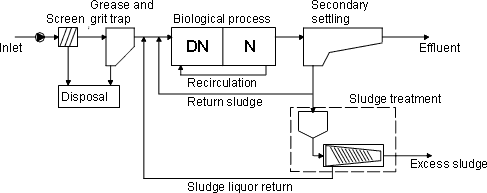
Figure 2-1
STP based on recirculation principle.
2.3 The STP selected for this study
Activated sludge for the major part of the experiments performed in this study was supplied from the STP at Egå, a suburb situated a few km north of Denmark's second largest city, Århus. Egå STP treats
sewage from the northern, suburban parts of Århus.
Egå STP is a so-called MBNDK type of sewage treatment plant, i.e. it treats sewage mechanically, biologically (under anaerobic, aerobic and denitrifying conditions) and chemically, and thus removes
organic matter, nitrogen and phosphorus to a high degree. With a capacity corresponding to about 90,000 PE it is one of the larger Danish STPs, and it receives a higher than average load from industries
and other enterprises (about 55% compared to an average of about 45% for other large STPs in Denmark). In the catchment is also a major regional hospital (Skejby Sygehus).
The total sludge retention time, SRT, at Egå STP is approximately 35 days (higher than for average Danish SRTs, namely 25-30 days) and the hydraulic retention time, HRT, for the STP is approximately
0.5-2 days (depending on volume of rainfall). The HRT in the anaerobic tank, denitrifying tank and nitrifying tank is on average 1.5, 6.5 and 6.5 hours, respectively.
The combined treatment of sewage at the plant reduces COD with 97%, total-N with 94% and total-P with 96%. The quality of the effluent (mean volume of 19,000 m³/day) appears to be somewhat better
than the average for this type of STP (COD: 24 mg/L, BOD5: 1.6 mg/L, total-N: 3.7 mg/L, total-P: 0.4 mg/L) and the performance parameters suggest that Egå is a well-run STP.
Egå STP was chosen for the study for a number of reasons:
1) It was fairly representative of larger Danish municipal STPs
2) It was already well described with regard to operational parameters and quality of inflow and outflow etc.
3) The plant was well known to the project team, thus making practical arrangements in relation to sampling easy and reliable.
4) Other related investigations had taken place and continue to take place in the catchments to and receiving water of Egå STP.
Regarding the last item, the choice of Egå STP as a source of sludge for the study, was based on the wish to provide synergy to the total work on estrogens and endocrine disruptors in Denmark. Thus, both
the municipality and county of Århus had been proactive and previously engaged in work on the issue of hormone disrupting compounds. Additionally, the municipality was at that time initiating further
environmental studies on their STPs and receiving waters, and had volunteered to fund the additional costs associated with sampling and transport etc for the sorption and degradation study.
3 Estrogens in sewage and sewage treatment processes
This chapter serves as an introduction to the following chapters describing the experimental work and results, which constitute the core of this project. The text in this chapter provides a brief summary of
selected, newer journal articles and reports on the occurrence and fate of estrogens in municipal STPs and on laboratory simulation studies of their behaviour in treatment processes. The intention is provide
an overview of newer, representative data rather than a comprehensive review of existing knowledge.
3.1 Estrogenic substances and their excretion
The main active estrogenic substance in humans is 17β-estradiol (E2) but the primary metabolite of E2, estrone (E1), also exhibits some estrogenic activity. An even less active metabolite, estriol (E3), is also
produced to a significant extent. 17α-ethinylestradiol (EE2), which is the most widely used active substance in contraceptive pills, also has a high estrogenic activity. Hence, the substances that appear to be
the most obvious to focus on in the context of this project are E1, E2 and EE2.
In order for the human body to inactivate and excrete estrogenic substances, which are hydrophobic and thus hard to eliminate, they must be transformed into more soluble forms that can be excreted via the
kidneys. Therefore, the estrogens are transformed by the metabolic system into soluble conjugates - a reaction by which they also loose their estrogenic activity.
The conjugation process is enzymatically mediated and generates sulphate- and glucuronic acid esters of the hydroxyl groups in the 3- and 7-position of the basic steroid structure. For E2 and EE2, which
both have two hydroxyl groups, eight different conjugates are theoretically possible, while for E1 there are only two. In human urine, natural estrogens only occur in their conjugated form, which, however,
can be transformed back into the parent substances by microorganisms and enzymes in sewage.
3.2 Levels of estrogens in untreated and treated sewage
A number of studies on the occurrence and levels of estrogens in municipal sewage have been published in recent years. Some of the studies report contents of estrogens in sewage only based on their
activity as measured by in vitro assays (e.g. the YES assay), while others have determined single substances by chemical analytical methods.
In Denmark, only very limited data exist where in the same study both influent and effluent concentrations of estrogens have been studied. DEPA (2003a) recently published a report on the occurrence of the
estrogens E1, E2 and EE2 in influents and effluents sampled at selected public STPs by qualified grab sampling (sample composed of five sub-samples taken with few minutes intervals). The levels observed
at the two plants where most samples were taken (Avedøre STP and Usserød STP) were comparable to those reported from other countries (Table 2-2).
Table 3-1
Selected estrogens (E1, E2 and EE2) in influents and effluents at two Danish public STPs (DEPA 2003a).
STP |
Estrogen |
Influent (ng/L) |
Effluent (ng/L) |
Avedøre STP (MBNDK) |
E1 E2 EE2 |
19 - 75
6.1 - 27 <1 - 1.7 |
5 - 11
<1 - 4.5 <1 - 5.2 |
Usserød STP (MBNDK + sand filter) |
E1 E2 EE2 |
30 - 61
8.8 - 22 1.7 - 4.8 |
<2
<1 <1 - 1.1 |
In the same study also the contents of a number of xenoestrogens (alkylphenols, bisphenol A, phthalates etc.) were determined analytically, and their share of the total estrogenicity was calculated based on
E2-equivalence factors (EEQs) in the YES-assay (an in vitro assay based on a genetically modified yeast strain). The xenoestrogens were found to account only for a limited part of the total estrogenic
activity (only the influent samples were possible to calculate; Table 3-2). This is consistent with observations from other studies.
It should be mentioned that the estrogenicity of a substance determined by an in vitro assay such as e.g. the YES-assay is not necessarily the same as the estrogenicity in vivo. Thus, the estrogenic activities
of E2 and EE2 in in vitro assays are typically about the same, while EE2 in laboratory experiments with fish has been shown to be 14-130 times more active (stated as LOEC-values) than E2 (Metcalfe et
al. 2001, Thorpe et al. 2003).
Table 3-2
The estrogenic activity of natural and synthetic estrogens (E1, E2 and EE2), and
of selected xenoestrogens, in influents of two Danish STPs (3 sampling rounds)
stated as E2-equivalents, and the xenoestrogens' share of the total estrogenic
activity (among the measured substances) (DEPA 2003a).
|
Avedøre STP |
Usserød STP |
1 |
2 |
3 |
1 |
2 |
3 |
EEQ-E* |
9.8 |
10.7 |
36.5 |
30.8 |
25.1 |
15.7 |
EEQ-XE** |
2.4 |
2.0 |
2.4 |
1.6 |
1.7 |
1.7 |
EEQ-XE in % of EEQ(total) |
20% |
16% |
6% |
5% |
6% |
10% |
* EEQ-E = sum of E1, E2 and EE2
** EEQ-XE = sum of NPE, NP, OP, Bisphenol A, DEP, DBP, BBP, DEHP and DOP.
Table 3-3 shows examples of levels of estrogenic compounds (stated as E2-equivalents) observed in influents and effluents at public STPs in some European countries outside Denmark and in the USA
(adapted from DEPA 2003a).
Table 3-3
Levels of estrogens in influents and effluents at public STPs in various countries, stated as E2-equivalents (DEPA 2003a).
Country |
Influent conc. (ng/L) |
Effluent conc. (ng/L) |
No. of samples (infl./effl.) |
Netherlands |
1 2 |
<l.o.d. - 25 32 ± 31 |
<l.o.d. - 0.6 3.1 ± 2.4 |
8 / 9 15 / 9 |
Germany |
1 2 |
56 - 58 17 |
5.6 - 11 10.5 |
2 / 2 1 / 1 |
Italy |
16 - 28 |
2.9 - 7.5 |
3 / 3 |
England |
n.a. |
0.4 - 6.6* |
0 / 7 |
U.S.A. |
18 - 24 |
5.0 - 10.6 |
3 / 3 |
l.o.d. = Limit of detection.
* One, very deviating result (46.7 ng/L) was omitted.
A review and assessment report on possible analytical methods for the determination of estrogens in the environment was recently published by DEPA (2003b). The report also includes a brief review of
data (primarily from Germany and Belgium) on the levels of a number of estrogens in raw and treated sewage and in sewage sludge. The data are shown in Table 3-4.
Table 3-4
Representative selection of expected and measured environmental concentrations in STP influents, effluents and sludge (<LOD and >LOQ indicate that estrogens were detected at concentrations below limits of detection or quantification) (from DEPA 2003b).
|
E1 |
E2 |
E3 |
EE2 |
MeEE2 |
Estimated |
Influent (ng/L) |
12-102 |
5-44 |
49-115 |
1.1-5.1 |
N.D. b) |
Effluent (ng/L)a) |
0.6-51 |
0.3-22 |
2.5-58 |
0.06-2.6 |
N.D. b) |
Sludge (ng/g) |
2.7-25 |
>1-5.1 |
N.D. b) |
N.D. b) |
N.D. b) |
Measured |
Influent (ng/L) |
44-490 |
11-180 |
<LOD-263 |
<LOD-120 |
5.3-120 |
Effluent (ng/L) |
<LOD-82 |
<LOD-21 |
<LOD-28 |
<LOD-62 |
N.D. b) |
Sludge (ng/g) |
<LOQ-37 |
<LOQ-49 |
N.D. b) |
<LOQ-17 |
<LOQ |
a) Concentrations in sewage effluent are estimated assuming 50-95% removal.
b) N.D. indicates that no data are available in literature.
Svenson et al. (2003) performed in 1999 sampling of raw and treated sewage at 20 Swedish public STPs ranging from simple to advanced, complex technologies and found at the latter (which resemble
typical, modern Danish plants) influent levels from approximately 3-25 ng EEQ/L and effluent levels from <0.1-5 ng EEQ/L (measured as total estrogenic activity in a yeast-based screening assay).
3.3 The fate of estrogens in sewage treatment plants
Only rather recently, i.e. since the late 1990ies, have reports on studies of the fate of estrogens in sewage treatment plants begun to appear in the international literature. Some of the key publications and their
main findings are briefly reviewed below.
Ternes et al. studied a number of natural and synthetic estrogens in sewage at a municipal STP near Frankfurt/Main (Ternes et al. 1999a), and found that about 2/3 of the incoming 17β-estradiol (E2) and
16α-hydroxyestrone was eliminated in the STP whereas the elimination efficiencies for estrone (E1) and 17α-ethinylestradiol (EE2) were low (<10%). In subsequent laboratory experiments with activated
sludge from the same plants, Ternes et al. (1999b) confirmed the persistence of EE2 under aerobic conditions while both E1 and E2 were degraded fairly rapidly under these conditions (E2 via E1).
Glucuronides of E2 were rapidly cleaved thus producing free E2.
In a recent study at 20 STPs in Sweden (Svenson et al. 2003), removal efficiencies of estrogenic activity (yeast-based assay) in the whole range from practically 0% to more than 99% were observed.
Simple treatment with only chemical precipitation using Fe- or Al-salts resulted in no removal at all while precipitation with lime appeared to be more effective (73%, only one plant). Trickling filters were less
effective than activated sludge systems to eliminate estrogenic activity, and the highest removal rates were obtained at plants with comprehensive treatment technologies i.e. combined biological and chemical
removal of organic matter, nitrogen and phosphorus. Samples filtered at 20 µm were also studied, and it was found that practically no estrogenicity was associated with particles larger than this size.
Andersen et al. (2003) investigated the fate of E1, E2 and EE2 at the STP of Wiesbaden, Germany. They observed an overall elimination efficiency of E1 and E2 of >98%, while EE2 elimination was slightly
lower; about 90%. E1 and E2 were found to be degraded in the activated sludge system while EE2 primarily was degraded only in the nitrifying tank. In the effluent, however, all concentrations were below
1 ng/L.
The fate of selected estrogens in sewage from six municipal STPs in Rome (dominated by domestic sewage) was recently studied by D'Ascenzo et al. (2003). They found that conjugated estrogens were
easily cleaved by the sewage, presumably by the â-glucuronidase enzyme produced by fecal coliform bacteria. However, the degradation of the studied sulphate, E1-3-sulphate (E1-3Sul), was somewhat
less. The elimination of free and conjugated estrogens at the STPs was 84-97% except for E1-3Sul, which was only reduced by 64%.
Matsui et al. (2000) found in a study at three STPs in Japan that in the influent E2 accounted for 34% of the total estrogenicity while in the effluent it was almost 100%. Matsui et al. also observed that
primary treatment contributed only little to the observed overall removal of estrogenicity, which primarily took place in the activated sludge part of the system. It was found that during the dewatering of
sludge, large amounts of estrogens were released to the water phase (which is afterwards recycled back into the plant). Concentrations close to 500 ng EEQ/L were observed in the water phase.
In another investigation of estrogens and other endocrine disruptors at Japanese STPs, Tanaka et al. (2000) reported that at most of the plants studied (total of 20) the total estrogenicity (as determined by
the YES assay) of the treated wastewater could be attributed to E2 to a very high degree. However, in the raw sewage there was at many STPs a significant content of estrogenicity that could not be
explained by the concentrations measured chemically of 44 known or suspected endocrine disruptors.
In a review article, Johnson & Sumpter (2001) conclude based on field data that the activated sludge treatment process appear to consistently be able to remove more than 85% of E2, E3 and EE2 whereas
the removal of E1 is less and more variable. Researchers from the same group (Kirk et al. 2002) describe results of studies at five public STPs in the UK, where removal rates for estrogens (measured only
as the sum of estrogenic activity by a yeast-based in vitro assay) were generally found to be 70% or higher. Very limited removal occurred in the primary treatment step while the activated sludge treatment
resulted in significant reductions. Only the fate of free (i.e. not conjugated) estrogens could be studied due to the detection method applied.
A recent Danish literature review on substances causing feminisation of fish (DEPA 2002) also includes a review of observations on the fate of estrogens in STPs. It is concluded that the advanced STPs
generally cope better with estrogens than more simple plants (e.g. only mechanical treatment) and that a high hydraulic retention time and a high sludge retention time in the activated sludge treatment process
has a positive influence on the ability of an STP to remove estrogens.
To summarise the above review of selected recent literature, it has generally been observed that primary treatment alone results in no or only limited removal of estrogens from sewage while secondary
treatment involving activated sludge very significantly reduces the levels of all estrogens. A long sludge retention time appears to have a positive influence on the ability of the activated sludge system to
eliminate estrogens. It appears that the substances E2 and E3 are very efficiently removed in the latter systems while the removal rate of E1 is somewhat lower and more variable and EE2 even more so as it
only undergoes significant degradation in the nitrification step. Conjugated forms of estrogens are easily cleaved in sewage and STP processes to produce the free, active forms of the estrogens. However,
there is a lack of studies describing in more detail the relative influence of the presumed two main removal processes involved in the activated sludge system i.e. sorption to sludge particles and
microbiological degradation.
4 Experimental Programme
4.1 Overview
The experimental programme performed in this project has aimed to describe the two main removal processes for estrogens related to the stage of sewage treatment being considered the most important,
namely sorption and degradation (stability) in the activated sludge treatment step.
Five estrogens were selected for the study:
- The two natural steroid estrogens estrone (E1) and 17β-estradiol (E2).
- The active ingredient in most contraceptive pills, 17α-ethinylestradiol (EE2).
- Two representatives of the two most abundant groups of conjugated estrogen metabolites: estrone-3-glucuronide (E1-3Glu) of the glucuronides and estrone-3-sulphate (E1-3Sul) of the sulphate esters.
Sorption: Only sorption of the free estrogens was measured, as the conjugated estrogens are more hydrophilic and thus are not believed to sorb to sludge to a great extent.
Stability in sterile water: Stability of all five estrogens was measured in sterile water as a control experiment (possible sorption to glass walls or other experimental artefacts) and to establish a baseline for
stability of estrogens. If estrogens degrade abiotically within a certain time, they are not likely to exist longer in any environment than this experiment shows.
Stability in activated sludge suspensions: Stability of five estrogens in activated sludge suspensions were tested under aerobic conditions. However, to reduce the number of samples that had to be
measured, experiments with addition of E1 were omitted. It is well known from other studies that in activated sludge E2 is oxidized very fast to E1. Therefore, the degradation of E1 could be followed in the
experiment where E2 was added.
Stability of the free estrogens with activated sludge suspensions were tested under anaerobic conditions. Again, the experiment with addition of E2 was used to measure degradation of E1. It was not
possible within the constraints set by time and resources to measure degradation of conjugated estrogens under anaerobic conditions. It is likely that aerobic or anaerobic conditions will not affect the
degradation of conjugated estrogens.
4.2 Activated sludge characteristics
4.2.1 Activated sludge used in the sorption experiments
The sludge used for the sorption experiment was settled activated sludge taken from Egå STP at 7.30 AM on the 26th of August 2003. Preliminary sorption experiments were carried out using sludge taken
from Lundtofte STP at 9 AM on the 15th of July 2003. Upon arrival at the laboratory about three or one hours later, respectively, the sludge was immediately washed three times using tap water (US EPA
standard, see Section 4.3.2) and centrifuged to remove dissolved molecules. The sludge was then freeze dried. The dried sludge was sterilized by heating at 103 °C for 120 min.
The total solids and volatile solids in the activated sludge suspension were measured according to Dansk Standard DS 204 (Dansk Standardiseringsråd, 1991). Further, the temperature was measured in the
tank of the STP from which the sludge was taken. The data from the characterisation of the collected sludge are shown in table 4-1.
Table 4-1
Characterisation parameters for the activated sludge used in all experiments.
STP |
Experiment |
Date of sample collection |
Temperature in activated sludge tank
°C |
MLSS
g/L |
VSS
g/L |
DS
g/L |
Loss on ignition
% |
Loss on ignition after washing % |
Lundtofte |
Sorption |
26-8 2003 |
ND |
10.3 |
ND |
ND |
ND |
64.9±0.1 |
Egå1 |
Sorption |
15-7 2003 |
18.7 |
4.6 |
3.4 |
5.4 |
67 |
74.2±0.1 |
Egå2 |
Degradation |
6-10 2003 |
16.2 |
4.4 |
3.3 |
5.1 |
69 |
ND |
4.2.2 Activated sludge used in the degradation experiments
The sludge used for the biodegradation experiment was activated sludge taken from Egå STP at 7.30 AM on the 6th October 2003. On arrival at the laboratory about three hours later, the sludge was
immediately aerated until the total solids concentration was determined and the batch experiment reactors could be started. The batch reactors were started about 12 hours after the sludge was taken from
the activated sludge tank of the STP. The data from the characterisation of the collected sludge are shown in Table 4–1.
4.3 Sorption experiments
In the experiment, the sorption equilibrium between sludge and water was allowed to adjust. A range of estrogen concentrations were tested.
The water used was similar to the artificial sewage described by Nyholm et al. (1996) except that the organic carbon sources were omitted to avoid risk of excessive bacterial growth.
4.3.1 Sorption kinetics
An experiment was carried out to determine the minimal time for establishing equilibrium between the water phase and solid phase (activated sludge). Activated sludge from Egå STP was used for this
experiment. The experiment design was based on determining water concentrations at different times after a 1 g/L sludge suspension was spiked with 400 ng/L of each steroid estrogen. Based on this
experiment the equilibration time for the experiment was set to 3 hours. This experiment was not performed on sludge from Lundtofte STP. Instead, the sorption experiment was allowed to equilibrate for 6 h
in experiments with sludge from Lundtofte SPT.
4.3.2 Sorption isotherm
It was intended to perform the sorption isotherm experiment as suggested in the US EPA standard (US EPA, 1996a; US EPA, 1996b). This standard suggests that a range of equilibrium concentrations are
produced by adding different amounts of sludge to bottles with a fixed concentration of the compound. The reduction in aqueous concentration of the measured compound provided by the sorption process
is used to establish the different equilibrium concentrations needed to experimentally determine a sorption isotherm. This experimental design was attempted with sludge from Lundtofte STP varying in
concentrations from 0.1 g/L to 5 g/L. However, it was found that aqueous equilibrium concentrations varied relatively little (less than two decades). Given the normal analytical random error and additional
variation caused by inhomogeneity of the sludge, the isotherms were poorly described.
It was therefore decided to use a modified experimental design still based on varying the ratios of compound concentration to sludge concentration as prescribed in the standard. We used different
concentrations of steroid estrogens covering four decades spaced equally on a logarithmic scale and equilibrated with a fixed sludge concentration of 1.0 g/L. Since the analytical method was not able to
quantitate the estrogens precisely over 4 decades of concentrations, different sample volumes were extracted ranging from 1 to 1000 ml. The volumes and starting concentrations used for the experiments are
shown in table 4-2.
The sorption test was performed at 16 °C using 100 ml, 500 ml or 1000 ml bluecap®-bottles in a laboratory shaker. The modified experimental design reduced the random variation compared to the scale
of the isotherms and provided a more precise determination of isotherms.
Table 4-2
Sample volumes and initial concentrations used for the different concentration replicates in the experiments for determining the sorption isotherms of E1, E2 and EE2. CO is the nominal starting concentration of each steroid estrogen. One replicate was made for each steroid estrogen at each concentration level.
Lundtofte STP |
|
Egå STP |
Sample volume |
CO |
Sample volume |
CO |
mL |
ng/L |
mL |
ng/L |
1 |
44272 |
1 |
47434 |
5 |
4427 |
5 |
15000 |
10 |
1400 |
10 |
4743 |
25 |
443 |
25 |
1500 |
70 |
140 |
70 |
474 |
200 |
44 |
200 |
150 |
950 |
14 |
950 |
47 |
950 |
8 |
950 |
15 |
4.3.3 Analysis
Sample volumes varied from 1 ml to 950 ml to allow exact quantification of a wide range of concentrations. Samples were filtered before extraction by solid phase extraction (SPE) and analysed as
described in Section 4.6.
4.4 Study of abiotic degradation in water
The stability of estrone (E1), 17β-estradiol (E2), 17α-ethinylestradiol (EE2), estrone-3glucuronide (E1-3Glu) and estrone-3sulphate (E1-3Sul) was followed for 4 or 6 days in a sterile mineral medium.
4.4.1 Experiments
One experiment was made for each chemical except EE2 for which the experiment was made in quadruplicate (in order to determine the variability between reactors rather than studying this substance in
particular detail). For E1, E2 and EE2 the recovery of a 500 ng/L starting concentration was measured. In the experiment with E1, E2 was also measured and likewise E1 was measured in the experiment
with E2 since E1 and E2 can be transformed to each other by simple oxidation or reduction reactions. Since an analytical method was not available for analysing estrogen conjugates E1 was measured in
experiments with E1-3Glu and E1-3Sul. The main degradation reaction for these two conjugates is expected to be hydrolysis which forms E1. In these experiments the starting concentration was 500 ng/L
E1 equivalents.
4.4.2 Experimental procedure
Experiments were performed in 1 l glass reactors as described by Nyholm et al. (1996) using phosphate buffered water adjusted to pH = 7.1±0.1. The experiment took place in a thermostatic room at a
temperature of 16 °C. Stirring of the reactors was done by bubbling air at a rate of 15 l/h. Stock solutions of the test compounds were made in methanol and addition was done with 150 µl methanol solution
per reactor. Samples were taken after 5 min, 1 h, 12 h, 24 h, 48 h, 72 h and 96 h. The two compounds expected to be most persistent with activated sludge, E1 and E1-Sul, were also sampled after 144 h.
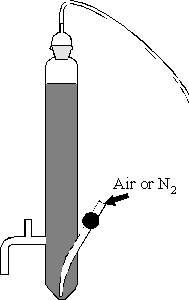
Figure 4-1
Schematic drawing of the glass reactor used for all degradation experiments.
4.4.3 Analysis
Samples of 100 ml were extracted with SPE and analysed as described in Section 4.6. No filtering or clean-up was used.
4.5 Study of degradation in activated sludge
The degradation of E2 and EE2 were investigated in diluted activated sludge with oxygen and nitrate as oxidation source. E1-3Glu and E1-3Sul were investigated in diluted activated sludge only with oxygen.
4.5.1 Experiments
One experiment was made for each chemical except EE2 in aerobic reactors for which the experiment was made in triplicate (to determine variability between the reactors).
For E2 and EE2 the recovery using a 500 ng/L starting concentration was determined. In the experiment with E2, E1 was also measured since E2 can be transformed to E1 by a simple reduction reaction.
Since an analytical method was not available for analysing estrogen conjugates E1 was measured in experiments with E1-3Glu and E1-3Sul. The main degradation reaction for these two conjugates is
expected to be hydrolysis which forms E1. In these experiments the starting concentration was 500 ng/L E1 equivalents.
4.5.2 Experimental procedure
Experiments were performed in 1 l glass reactors with 0.50 g/L of activated sludge and 1.00 ml phosphate buffered mineral media (Ca2+, Mg2+, Na+, Cl-, SO42-) at 16 °C with pH = 7.8±1.0. Reactors
were fed with OECD artificial peptone sewage with modifications according to Nyholm et al. (1996) at a starting concentration of 100 mg/L BOD. Each day the BOD was replenished with further 25 mg/L.
The activated sludge was added 12 h prior to initiating the experiment started to give the sludge bacteria time to adapt to the oxidation sources (O2 or NO3-) in the reactors. Samples were taken after 3 min,
6 min, 20 min, 40 min, 90 min, 3 h, 6 h, 12 h, 24 h, 48 h and 72 h. Further, six blank samples and six control samples were taken. Control samples were samples of sludge suspensions taken from the
reactors before addition of test compounds, which was stabilised according to the analytical procedure to stop biodegradation activity (pH adjusted to pH = 3), before addition of test compound at the
concentration used in the degradation experiment.
4.5.2.1 Stability experiment in aerobic activated sludge
In reactors with oxygen as oxidation source stirring was done by bubbling air at a rate of 15 l/h resulting in a dissolved oxygen concentration of 7.25 mg/L.
4.5.2.2 Stability experiment in denitrifying activated sludge
In reactors with nitrate as oxidation source stirring was done by bubbling nitrogen at a rate of 15 l/h. Nitrate was added at the beginning of the experiment to a concentration of 20 mg-N/l. During the
experiment nitrate was measured and replenished at least twice daily.
4.5.3 Analysis
Samples of 100 ml were extracted with SPE and analysed as described in Section 4.6. The particles in the sample was loaded on top of the cartridge rather than removed by filtration before the extraction
(as was done in the sorption experiment). This made it possible to elute the particle bound steroid estrogens together with the steroid estrogens on the SPE column. This was done in order to extract all
estrogens present in the sample rather than only the estrogens dissolved in the water phase.
4.5.4 Batch reactor characterisation
The chemical environment in the batch reactor was characterised by measuring O2 and pH with selective electrodes and nitrate with a colorimetric test kit (see appendix 1 for details on instrumentation)
several times a day during the experiments.
The stability of the biodegradation activity in the sludge slurry over the experimental period was further estimated by measuring the cumulative biological oxygen demand (BOD) over 24 h in samples taken
from reactors with both types of RedOx regimes every day of the experiment.
4.6 Chemical analysis
4.6.1 Analytical procedures
4.6.1.1 Sorption experiment
Samples from the sorption experiment were separated from the sludge by filtration over a GF/C filter (47 mm diameter, Whatman International Ltd, Maidstone, England) or by centrifugation depending on
what was most convenient for the size of sample. Each water sample was acidified to pH = 3 using sulphuric acid and spiked with 250 ng E2-acetate, which functions as the extraction pseudo standard. All
samples were extracted onto the 500 mg version of the BondElut C18 solid phase extraction cartridge (Varian, USA). The cartridge was then freeze dried. The estrogens were eluted from the cartridge with
5 ml acetone. This extract was evaporated and reconstituted in 200 µl H2O:MeOH (35:65), which was transferred to the analysis.
4.6.1.2 Abiotic stability experiment
Samples from the abiotic stability experiment were treated exactly as samples for the sorption experiment except that no solids had to be removed.
4.6.1.3 Activated sludge stability experiments
Each sample from the sludge biodegradation experiment were acidified to pH = 3 exactly at the time the sample was taken, to stop the biodegradation processes. Samples were spiked with 100 ng of
deuterated standards (d4-E1, d5-E2 and d5-EE2) as extraction standards. The sample containing both water and sludge were then loaded onto a solid phase extraction cartridge. The particles in the sample
was loaded on top of the cartridge rather than removed by filtration before extraction according to a method described by Ternes et al. (1999). This was done in order to extract all estrogens present in the
sample rather than only the estrogens dissolved in the water phase. In this experiment the 500 mg version of the ISOLUTE C18 solid phase extraction cartridge (ICT, Germany), was used rather than the
cartridge from Varian, as the ICT cartridge was previously evaluated for this purpose (Joss et al. 2003). Then all the water had been drawn through the cartridge the sludge was left on top of the column. The
cartridge was then dried by freeze drying. The estrogens were eluted from the cartridge with 2 x 2.5 ml acetone. This extract was evaporated and reconstituted in 200 µl acetone:hexane 35:65. The reduced
extract was purified by passing in through a custom made 1 g silica gel column with 5 ml acetone:hexane 35:65 mixture (Andersen et al., 2003). The clean-up removed most of the coloured matrix from the
extract. This eluate was evaporated and reconstituted in 200 µl H2O:MeOH 35:65, which was transferred to the analysis.
In the method evaluation of the analytical procedure for this sample type it was found that the use of silica gel clean-up was essential for being able to detect the estrogens in this matrix rich sample type. Even
after the clean-up some parts of the matrix were still left and made the ionisation efficiency for the MS detector unstable. This problem could be satisfactory solved by using deuterated standards of each of
the analytes. As the deuterated standards were chemically exactly like the analyte they represent the variation in ionisation efficiency was exactly matched by the same variation in the ionisation of the
standard.
4.6.2 Quantification standards and control samples
For each sample series a range of concentrations of steroid estrogens and extraction standards spiked to samples of drinking water was used to create a standard curve. These spiked samples were
extracted and cleaned with the same procedure as the real samples. Thus all systematic losses in the extraction and clean-up procedure for the samples were repeated on the standards and, hence, the effects
will cancel each other. Further, each sample series included blank samples and spike recovery samples.
The extraction standards (E2-acetate (AE2) and deuterated standards) were used in an in-method statistical control system as described by Ternes et al. (2002).
4.6.3 Instrumentation for quantification
Quantification of steroid estrogens concentrations in the extracts was done by LC-triplequadropole-MS-MS. The Sciex API 3000 LC-triplequad-MS-MS was used with an APPI (Atmospheric Pressure
Photo Ionisation) ion source.
Chromatographic separation of the analytics before the quantification by MS-MS was done with a HPLC system (Agilent 1100 Series) using a C18 XTerra®RP18 3,5m, 3.0 x 150mm column and gradient
elution by H2O:MeOH from 35:65 to 0:100. The HPLC had a total run time of 60 min and retention times as shown in Table 4-3.
Table 4-3
Retention times and m/Z for mother and daughter ions used for quantification of the steroid estrogens.
|
Retention time
(min) |
Mother ion
(m/Z) |
Daughter ion 1
(m/Z) |
Daughter ion 2
(m/Z) |
E1 |
16.12 |
271.4 |
157.1 |
159.3 |
E2 |
19.03 |
255.2 |
133.2 |
159.3 |
EE2 |
19.73 |
279.4 |
133.3 |
159.3 |
AE2 |
32.52 |
255.2 |
133.2 |
159.3 |
d4-E1 |
16.12 |
275.4 |
257.2 |
161.2 |
d5-E2 |
19.03 |
260.3 |
161.1 |
162.1 |
d5-EE2 |
19.73 |
283.3 |
161.2 |
135.2 |
4.6.4 MS-MS parameters
For each steroid estrogen a characteristic mother ion formed from the molecule in the ionisation head of the MS-MS is selected by the mass detector. This ion is fragmented in the MS-MS and two
characteristic daughter ions formed in the fragmentation process are selected for identification and quantification (see Figure 4-2 and Table 4-3).
Click here to see Figure 4-2.
Only when both ions were present in the correct ratio between the two, the signal could be used for quantification. A high certainty for quantification of the correct analyte is inherent to the chosen
quantification method. Each analyte had to elute at the correct retention time in the LC system. Then the ionisation should produce an ion with a correct mass, and fragmentation of this ion should produce
two new ions with correct masses.
4.7 Data treatment for stability experiments
Each concentration profile obtained in the abiotic and activated sludge stability experiment was fitted to first order reaction expressions as shown in Box 1 (Figure 4-3). This was done by integrating the
expression with appropriate limits and fit the resulting expression to the observed concentration profiles.
Since E1 and E2 are degraded very fast in this experimental setup, it is difficult to measure the degradation rate precisely. Therefore, the net loss of the steroid estrogen structure was also determined by
fitting the first order degradation expression to the sum of the concentrations of E1 and E2. This should be interpreted as the effective rate constant for loss of the estrogenic structure.
Box 1: Fitting formulas
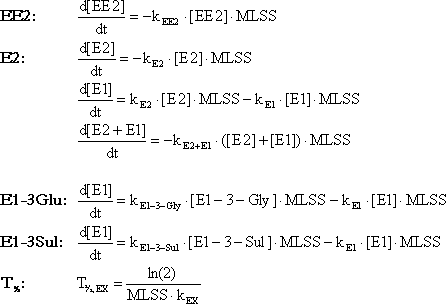
Figure 4-3
Box 1: Fitting formulas for first order reaction rate constants and half-lives.
T½, EX and kEX is the half-life and first order reaction constant, respectively, for any of the degradation reactions.
5 Results and discussion
5.1 Sorption
5.1.1 Sorption kinetics
The time until sorption equilibrium was established was estimated by measuring water concentrations in an experiment with 1 g/L sludge and a water concentration of the respective steroid estrogen of 400
ng/L at different times after addition of the steroid estrogen. The results of these experiments are shown in Figure 5-1.
Click here to see Figure 5-1.
Between 87 % and 97 % of the loss of estrogens from the water phase had occurred in 0.5 hours. For E1 and EE2 no difference between two hours and six hours equilibrium time could be seen (less than 3
% change). It is therefore concluded that the sorption reaction had reached equilibrium after two hours. For E2 a tendency for a further decrease in the water concentration is seen after six hours (further 11
% change). The three steroid estrogens are similar in size and lipophilicity, therefore the sorption kinetic should be similar for the three. It is assumed that the apparent lower water concentrations of E2 at six
hours reaction time is an artefact of the random error on the concentration determination since no explanation based on the physical-chemical properties can be given. Based on this experiment the
equilibrium time used for the experiments to determine the sorption isotherms was set to three hours.
5.1.2 Isotherms
Isotherms from the sorption experiments with the three steroids are shown in Figures 5-2 and 5-3.
Click here to see Figure 5-2.
Click here to see Figure 5-3.
The Freundlich sorption isotherm defines the equilibrium between concentrations of a chemical in water and solid (Schwarzenbach et al., 2003):
CS = KF·CWn
Equation 1:
The Freundlich equation for describing sorption isotherms (Schwarzenbach et al., 2003). Cs is the concentration in the solids in ng/kg, Cw is the concentration in water in ng/L and KF and n
are the Freundlich parameters that characterise the sorption isotherm.
The parameters for the Freundlich equation were calculated by non-linear curve fitting as shown in Table 5-1.
Table 5-1
Parameters for the Freundlich equation description of the sorption isotherms for steroid estrogens to activated sludge from Egå and Lundtofte STPs.
|
Egå STP |
Lundtofte STP |
E1 |
E2 |
EE2 |
E1 |
E2 |
EE2 |
KF (l/kg ) |
822±918 |
594±281 |
267±257 |
89 ±105 |
1106 ±627 |
383±245 |
n |
0.945±0.146 |
0.926±0.064 |
1.080±0.120 |
1.154 ±0.155 |
0.770 ±0.093 |
1.020±0.092 |
r² |
0.82 |
0.94 |
0.95 |
0.75 |
0.93 |
0.81 |
Replicates |
12 |
14 |
23 |
9 |
8 |
11 |
The width of the 95 % confidence range for the Freundlich parameters, KF, shown in table 5-1 is in the order of 65-110 % of the estimated value of the parameter. This suggests that when these constants
are used for calculating conditional Kd-values and estimating the sorbed fractions of steroid estrogens (tables 5-2 and 5-4), the errors on the calculated results should be in that range. Estimation of the errors
on parameters with non-linear fitting using a generalised method is a very weak statistical method, which tends to give estimates of variation much higher than what is intuitively expected from the variation in
the data. This is due to the non-specificity of the method as it designed to apply to all types of non-linear expressions.
The calculations of the inter-experimental errors on the Freundlich parameters give confidence ranges above 50 % of the parameter values. However, when the experiments were repeated (data not shown)
during the method optimization (2-3 independent experiments), the variations between experiments were only in the order of 15 % of the parameter values. Therefore, it is believed that the realistic
confidence range is rather in the order of ±25 %.
5.1.3 Kd-values
Kd-values can be calculated for representative water concentrations based on the Freundlich isotherms as:
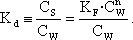
Equation 2:
Formula for generating conditional Kd-values for steroid estrogens at different water concentrations from the Freundlich equation.
By inserting relevant values for water concentrations of steroid estrogens into Equation 2 a table of Kd-values can be generated as shown in Table 5-2. It should be noted that the lower concentrations
chosen for the table are extrapolations below the experimental concentrations range, which may hide systematic deviation, not covered in the experiment.
Table 5-2
Calculated conditional Kd's for steroid estrogen sorption to activated sludge from Egå and Lundtofte STPs based on the fitted parameters for the Freundlich equation description of the sorption isotherms.
Cw |
Egå STP |
Lundtofte STP |
LogKD,E1 |
LogKD,E2 |
LogKD,EE2 |
LogKD,E1 |
LogKD,E2 |
LogKD,EE2 |
ng/L |
l/kg |
l/kg |
l/kg |
l/kg |
l/kg |
l/kg |
10 |
2.86 |
2.70 |
2.51 |
2.10 |
2.81 |
2.60 |
20 |
2.84 |
2.68 |
2.53 |
2.15 |
2.74 |
2.61 |
50 |
2.82 |
2.65 |
2.56 |
2.21 |
2.65 |
2.62 |
100 |
2.80 |
2.63 |
2.59 |
2.26 |
2.58 |
2.62 |
200 |
2.79 |
2.60 |
2.61 |
2.30 |
2.51 |
2.63 |
500 |
2.77 |
2.57 |
2.64 |
2.36 |
2.42 |
2.64 |
1000 |
2.75 |
2.55 |
2.67 |
2.41 |
2.35 |
2.64 |
The sorption isotherms for E2 and EE2 are very similar for sludge from the two STPs. E1 apparently sorbs stronger to sludge from Egå STP than E2 and EE2 compared to sludge from Lundtofte STP where
E1 sorbs less than E2 and EE2.
The difference in sorption tendency between E1, E2 and EE2 found in the experiment with sludge from Lundtofte appears to agree better than that from Egå with the Kd's that are estimated from Kow's by a
specific QSAR equation for sorption of lipophilic chemicals to activated sludge as shown in Table 5-3. This approach estimates that the Kd–value for E1 should be less than or equal to Kd for E2 and EE2.
Therefore the Kd determined for sorption of E1 to sludge from Egå STP should be used with caution.
Table 5-3
Kow-values for the steroid estrogens according to three sources and predicted
Kd-values based on a QSAR (Matter-Müller et al., 1980) developed for lipophilic chemicals that uses Kow to estimate the Kd for activated sludge.
|
Log(Kow) |
Log(Kd) |
Reference |
E1 |
E2 |
EE2 |
E1 |
E2 |
EE2 |
Lai et al., 2000 |
3.43 |
3.94 |
4.15 |
2.69 |
3.03 |
3.17 |
Holthaus et al., 2002 |
|
3.1 |
3.9 |
|
2.5 |
3.0 |
Geyer et al., 2000 |
4.1 |
4.0 |
4.2 |
3.1 |
3.1 |
3.2 |
5.1.4 Prediction of sorbed fractions
In the stability experiments with activated sludge the content of dry solids (DS) is 0.5 g/L. In conventional STP the activated sludge is operated between 3 and 5 g DS/l. In Egå STP the MLSS in the effluent
is typically 0.005 g DS/L, while regulations in Denmark and the EU allow maximally 0.030 g DS/L and 0.050 g DS/L, respectively, in STP effluents.
Using the conditional Kd-values at the 100 ng/L concentration level for Lundtofte sludge, the fraction of steroid estrogens that would be sorbed at equilibrium in these sludge densities is calculated with
equation 3 as shown in table 5-4.

Equation 3
Equation for calculating the sorbed fraction of the total steroid estrogen concentrations at different densities of activated sludge (MLSS).
If the MLSS in the activated sludge tank and the excess sludge production (mass of activated sludge removed from the STP per volume of treated sewage) (RESS) is known the fraction of the total steroid
estrogen load into the activated sludge tank that will be removed at equilibrium assuming no degradation occurs can be calculated as follows (Equation 4).

Equation 4
Equation for calculating the removed fraction of the steroid estrogen load at a given densities of activated sludge (MLSS) and Removal rate for Excess Suspended Solids (RESS).
A typical value for the removal of excess activated sludge from an STP is 100 mg/L of treated sewage (Henze et al., 2002). This figure is derived from the daily production of excess sludge divided by the
daily wastewater flow. As mentioned it is typical that MLSS in the activated sludge tank is 4 g DS/L. If these values are used, the removal of steroid estrogens with activated sludge can be estimated as
shown in table 5-4.
Table 5-4
Predicted sorbed fraction for representative sludge densities using Kd values taken from the sorption isotherms at CW = 100 ng/L and equation 3 and 4.
|
|
Egå |
Lundtofte |
MLSS |
E1 |
E2 |
EE2 |
E1 |
E2 |
EE2 |
Log(Kd) |
|
2.80 |
2.63 |
2.58 |
2.26 |
2.58 |
2.62 |
Fractions of steroid estrogens sorbed in the activated sludge tank at high MLSS. |
5.0 g/L ds |
76% |
68% |
66% |
48% |
66% |
68% |
Fractions of steroid estrogens sorbed in the activated sludge tank at medium MLSS. |
4.0 g/L ds |
72% |
63% |
60% |
42% |
60% |
63% |
Fractions of steroid estrogens sorbed in the activated sludge tank at low MLSS. |
3.0 g/L ds |
65% |
56% |
53% |
35% |
53% |
56% |
Fractions of steroid estrogens sorbed in the stability experiments |
0.5 g/L ds |
24% |
18% |
16% |
8.3% |
16% |
17% |
Fractions of steroid estrogens load removed with excess sludge assuming sludge production is 100 mg/L ds and the MLSS in the
activated sludge tank is 4 g/L ds. |
100 mg/L ds |
1.8% |
1.6% |
1.5% |
1.1% |
1.5% |
1.6% |
Fractions of steroid estrogens sorbed in the STP effluent at highest daily SS allowed by EU regulations |
50 mg/L ds |
3.1% |
2.1% |
1.9% |
0.90% |
1.9% |
2.0% |
Fractions of steroid estrogens sorbed in the STP effluent at highest average SS allowed by Danish regulations |
30 mg/L ds |
1.9% |
1.3% |
1.1% |
0.54% |
1.1% |
1.2% |
Fractions of steroid estrogens sorbed in the STP effluent at the actual SS for Egå STP |
5 mg/L ds |
0.31% |
0.21% |
0.19% |
0.091% |
0.19% |
0.21% |
The sorbed fractions in the activated sludge tank have implications for the predictions of the degradation of the steroid estrogens in the activated sludge tank, which is discussed in Section 5.5. In the stability experiments with sludge the MLSS was 0.5 g DS/L, which is predicted to give a dissolved fraction of steroid estrogens of 92-76 %. In an activated sludge tank with high MLSS of 5 g DS/L, only 52 – 24 % of the total estrogen concentration is dissolved in water. Presumably bacteria only degrade the dissolved steroid estrogens. When 48-76 % of the steroid estrogens are not in the water phase the degradation rate will be reduced proportionally. Further, if the desorption from the sludge takes place at a lower rate than that of the degradation in water, the desorption reaction becomes the rate limiting step for the overall degradation of the steroid estrogens.
The predicted sorbed fractions also have consequences for the design of the analytical procedures for such experiments. Thus, if one wishes to measure the steroid estrogens in a sample from an activated
sludge tank, both water and sludge should be extracted and analysed. If only the water phase is analysed, up to 76 % of the steroid estrogens may be missed. Contrary to this, if the sample was taken from
the effluent, the error introduced by only extracting the water phase could maximally be 3.1 %.
A practical implication of the above is that when the concentrations of effluent suspended solids are below the EU or national regulations, the sorbed fraction is of minor significance.
5.2 Abiotic degradation in water without activated sludge
As shown in Figure 5-4 no clear tendency for reduction of the three steroid estrogens were seen. Also no trace of E1 was seen in the experiment with E2. This could have indicated that auto-oxidation of E2
occurred. Similarly, no trace of E2 could be found in the experiment with E1. This would have occurred if abiotic reduction of E2 occurred during the test.
No clear sign of formation of E1 by hydrolysis of either E1-3Glu or E1-3Sul was seen in the stability experiment.
Fitting of the concentration profiles in figures 5-4 and 5-5 to the first order kinetics equations in Box 1 (Figure 4-3) yielded degradation constants from which T½ could be calculated. These values are shown
in table 5-6. It is seen that the lower 95 % confidence limit for T½ is higher than 9 days for each chemical. Further, except for E2 and one of the four EE2 replicates, the fitted first order rate constants were
not significantly different from zero at the 95 % confidence level.
Table 5-5
First order reaction constants and half-lives for steroid estrogens in the abiotic experiments. The 95 % confidence limit on the values that has been estimated from the variation found within each experiment is
shown in brackets. The value for T½ and its higher confidence limit could not be calculated for E1-3Sul.
Compound |
k, abiotic |
T½ |
kE1, abiotic |
-0.019 |
±0.019 |
L·g-1·d-1 |
NA |
d |
kE2, abiotic |
0.042 |
±0.039 |
L·g-1·d-1 |
16 (9 - 196) |
d |
kE1-3Glu, abiotic |
-0.0080 |
±0.050 |
L·g-1·d-1 |
NA |
|
kE1-3Sul, abiotic |
-0.057 |
±0.082 |
L·g-1·d-1 |
(> 26.4 ) |
d |
kEE2, abiotic #1 |
0.039 |
±0.036 |
L·g-1·d-1 |
18 (9 - 217) |
d |
kEE2, abiotic #2 |
0.0038 |
±0.022 |
L·g-1·d-1 |
184 (> 26.4) |
d |
kEE2, abiotic #3 |
-0.0071 |
±0.030 |
L·g-1·d-1 |
NA |
|
kEE2, abiotic #4 |
0.011 |
±0.015 |
L·g-1·d-1 |
64 (> 27.3) |
d |
Click here to see Figure 5-4.
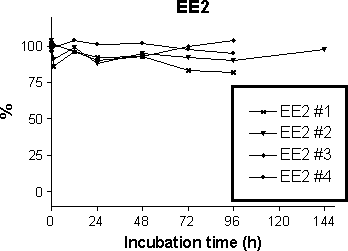
Figure 5-5
Degradation of 500 ng/L EE2 in four independent abiotic experiments.
5.3 Degradation in aerobic activated sludge suspension
Significant degradation of the five steroid estrogens was found except for E1-3Sul. E2 was degraded the fastest followed by E1. It appears that the only degradation process for E2 is oxidation to E1 since
the sum of E1 and E2 was approximately constant in the first minutes of the degradation experiments with E2. EE2 was the most stable of the estrogens.
Click here to see Figure 5-6.
The degradation experiment with EE2 was made as triplicate independent experiments and therefore the variability between reactors could be estimated. The half lives in the three experiments varied
between 10 h and 12 h. The contribution to the error on estimating the degradation rates from variation between reactors is thus in the order of 10 %, which is not more than could be expected when the
error on the chemical analysis of steroid estrogens is considered.
The stability of E1-3Glu was not much higher than the stability of E1. Therefore only a small peak in concentrations of E1 was found in the experiment with E1-3Glu.
In the experiment with E1-3Sul no significant amount of E1 was detected during the three days of incubation. It is therefore concluded that E1-3Sul is degraded slower than E1 in this experiment.
The first order reaction rate constants obtained by fitting the concentration curves can be used to calculate half lives of each test compound using the formula in Box 1 (Figure 4-3). In the batch experiments,
the sludge concentration is 0.5 g/L whereas a typical sludge density found in the activated sludge treatment step of an STP is about 4 g/L. The calculated values are shown in table 5-6.
Table 5-6
First order reaction constants and half-lives for steroid estrogens in experiments with activated sludge in the aerobic reactors. The 95 % confidence limit on the values that has been estimated from the
variation found within each experiment is shown in brackets. The value for T½ and its higher confidence limit could not be calculated for E1-3Sul.
Compound |
k, aerobic |
T½ at MLSS = 0.5 g/L |
T½ at MLSS = 4 g/L |
kE1,bio,aerob |
405 |
±158 |
L·g-1·d-1 |
4.9 |
(3.5 - 8.1) |
min |
0.62 |
(0.44 - 1) |
min |
kE2,bio,aerob |
768 |
±419 |
L·g-1·d-1 |
2.6 |
(2 - 6) |
min |
0.32 |
(0.21 - 1) |
min |
kE1+E2,bio,aerob |
271 |
±183 |
L·g-1·d-1 |
7.4 |
(4 - 23) |
min |
0.92 |
(0.55 - 3) |
min |
kE1-3Glu,bio,aerob |
124 |
±65 |
L·g-1·d-1 |
16 |
(11 - 34) |
min |
2.0 |
(1.32 - 4) |
min |
kE1-3Sul,bio,aerob |
-60 |
±1670 |
L·g-1·d-1 |
(> 1.24) |
min |
(> 0.15) |
min |
kEE2,bio,aerob #1 |
3.3 |
±1.4 |
L·g-1·d-1 |
10 |
(7 - 18) |
h |
1.3 |
(0.89 - 2.3) |
h |
kEE2,bio,aerob #2 |
3.0 |
±1.2 |
L·g-1·d-1 |
11 |
(8 - 18) |
h |
1.4 |
(0.99 - 2.2) |
h |
kEE2,bio,aerob #3 |
2.8 |
±1.0 |
L·g-1·d-1 |
12 |
(9 - 18) |
h |
1.5 |
(1.08 - 2.3) |
h |
The half-lives presented in Table 5-6 for the aerobic degradation in sludge at 4 g MSLL/l may well underestimate the real time required to reduce the total concentration of the estrogens if the desorption
from sludge particles is slower than the degradation reactions (this is, however, not known and has not been possible to investigate in this study).
5.4 Degradation in denitrifying activated sludge suspension
When oxygen was excluded from the activated sludge slurry and bacteria had to use nitrate as electron donor, the degradation rates for E1 and EE2 were reduced significantly (10- to 20-fold), whereas the
degradation of E2 was not markedly changed.
Click here to see Figure 5-7.
Table 5-7
First order reaction constants and half-lives for steroid estrogens in experiments with activated sludge in the denitrifying reactor. The 95 % confidence limits on the values (estimated from the variation found
within each experiment) are shown in brackets. A highest confidence limit could not be calculated for the T½ of EE2.
Compound |
k, denitrifying |
T½ at MLSS = 0.5 g/L |
T½ at MLSS = 4 g/L |
kE1, bio, anaerob |
14 |
±5 |
L·g-1·d-1 |
143 |
(103 - 231) |
min |
18 |
(13 - 29) |
min |
kE2, bio, anaerob |
497 |
±283 |
L·g-1·d-1 |
4.0 |
(3 - 9) |
min |
0.50 |
(0.32 - 1) |
min |
kE1+E2, bio, anaerob |
27 |
±21 |
L·g-1·d-1 |
75 |
(42 - 355) |
min |
9.3 |
(5.2 - 44) |
min |
kEE2, bio, anaerob |
0.17 |
±0.17 |
L·g-1·d-1 |
8.3 |
(> 5884) |
d |
1.0 |
(> 735) |
d |
5.5 Predictions of steroid estrogens stability
5.5.1 Loss during treatment at STPs
The results of the degradation experiments with activated sludge suspensions performed in the laboratory can be used to estimate the loss of the steroid estrogens during the activated sludge treatment in a
real STP. The remaining total concentration after treatment in each compartment is calculated from the experimentally determined first order reaction constant for the relevant steroid estrogen and
RedOx-environment from tables 5-6 and 5-7, using Equation 5.
The combined removal after treatment in both the aerobic and the denitrifying activated sludge compartments are calculated as the product of the remaining fractions from each treatment step as shown in
Equation 6.
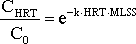
Equation 5
Equation for calculating the remaining fraction of the steroid estrogen total concentration after treatment in a given RedOx-environment/compartment of the activated sludge treatment with a given activated sludge concentration (MLSS) for a time period equal to the average hydraulic retention time HRT of the compartment.

Equation 6
Equation for calculating the remaining fraction of the steroid estrogen total concentration after degradation of the steroid estrogen in both the aerobic and the denitrifying activated sludge
treatment.
The data for the STP (hydraulic retention times (HRT) in compartments and MLSS) used in this scenario are those of Egå STP from where the sludge used in the degradation experiments originated. For
Egå STP the average HRT in the denitrifying tank is 1.5 hours and the average HRT in the aerobic tank is 6.7 hours. The HRT in the aerobic tank is longer than the denitrifying tank because nitrification,
which occurs only in aerobic conditions, is the slowest of the nitrogen conversion processes in STPs. The typical MLSS is
4 g DS/l in Egå STP. With these parameters and the calculated reaction rate constants, the removal of steroid estrogens is estimated as shown in table 5-8.
Table 5-8
Prediction of the fraction of steroid estrogens remaining after activated sludge treatment in Egå STP.
RedOX |
Aerobic |
Denitrifying |
Combined |
MLSS |
4.0 g DS/L |
4.0 g DS/L |
4.0 g DS/L |
HRT in activated sludge compartment |
0.28 day |
0.06 day |
0.34 day |
Compound |
|
|
|
E1 |
0.000 (0.00-0.00)% |
3.0 (0.8-12)% |
0.000 (0.00-0.00)% |
E2 |
0.000 (0.00-100)% |
0.000 (0.00-0.00)% |
0.000 (0.00-0.00)% |
E1+E2 |
0.000 (0.00-0.00)% |
0.12 (0.00-25)% |
0.000 (0.00-0.00)% |
E1-3Glu |
0.000 (0.00-0.19)% |
ND |
NA |
E1-3Sul |
NA |
ND |
NA |
EE2 #1 |
2.6 (0.55-12)% |
96 (92-100)% |
2.5 (0.5-13)% |
EE2 #2 |
3.4 (0.89-13)% |
96 (92-100)% |
3.3 (0.8-13)% |
EE2 #3 |
4.2 (1.4-13)% |
96 (92-100)% |
4.0 (1.2-13)% |
The values in Table 5–8 for the combined removal efficiency of estrogens in the activated sludge system at Egå STP allow the effluent concentrations to be estimated if the influent concentrations are known.
Since these data do not exist for Egå STP, an estimate can be based on the influent concentrations measured at two other Danish STPs (Avedøre and Usserød, see Table 3–1). For E1 and E2, the predicted
effluent concentration is 0 ng/L, since the predicted removal rate is 100 %. The predicted effluent concentration for EE2 is 0.16 (0.04 – 0.6) ng/L based on the highest influent concentration measured at
Usserød STP (4.8 ng/L). All these concentrations are below the limit of quantification of standard analytical methods today.
As appears from Table 3-1, the measured effluent concentrations at Avedøre STP are higher than predicted, while the values from Usserød STP are closer to the predicted values. The difference between
the effluent concentrations at these two plants is probably due to the shorter total sludge retention time (SRT) at Avedøre compared to Usserød (20-25 and 33 days, respectively), and maybe also to the fact
that Usserød has a sand filter installed after the clarifier tank. The SRT at Usserød STP is almost as high as that of Egå STP.
As discussed previously, the predictions of the removal efficiency do not take into consideration the effects of sorption in the activated sludge tank, which may reduce the effective degradation rate. E.g.
steroid estrogens that have been sorbed in the activated sludge tank may be released from the activated sludge during the subsequent settling process in the clarifier. Hence, the predicted removal rates are
probably too high
5.5.2 Loss during transport of samples
In the part of the scientific community that researches the fate of steroid estrogens in STPs and measure steroid estrogen concentrations in STP effluents there is an ongoing discussion about procedures for
conserving and transporting samples for analysis. The scenario in table 5-8 clearly suggests that samples must be conserved immediately irrespectively of the RedOx environment in the sample (presumably
they will quickly become anaerobic from the respiration in the activated sludge). In tables 5-6 and 5-7 the half-lives for E1 and E2 are estimated to be about or less than 1 min in the activated sludge
suspension. Therefore, addition of one or more preserving chemicals is important immediately after sampling. The recommended preservation method is addition of sulphuric acid. Further, a silver salt may be
added to the samples, which should be stored at less than 4 °C.
The results of the stability experiments with activated sludge suspensions may also be used to estimate the stability of steroid estrogens in samples of STP effluents where low concentrations of activated
sludge is found as mentioned in Section 5.1.4 and Table 5-4. Samples are often taken far from the laboratory and, hence, due to logistic difficulties the time from sampling to extraction may be as much as 24
hours. However, in this project the time between sampling at Egå STP and sample treatment was initiated at DFU was only about 3 hours (0.13 days). If it is assumed that samples remain aerobic until they
reach the laboratory, the estimated stability of estrogens in the samples at the sludge concentrations discussed in Section 5.1.4 and Table 5-4 will be as shown in table 5-9.
Table 5-9
Fraction of steroid estrogens predicted to remain in a sample of a STP effluent after transport assuming no preservation is used for the sample.
Effluent MLSS for |
Egå (actual) |
Danish regulation |
EU-regulation |
MLSS |
0.005 g DS/L |
0.005 g DS/L |
0.030 g DS/L |
0.050 g DS/L |
Sample transport time |
0.13 day |
1 day |
1 day |
1 day |
Compound |
|
|
|
|
E1 |
78 (75-80)% |
13 (10-18)% |
0.000 (0.00-0.00)% |
0.000 (0.00-0.00)% |
E2 |
62 (37-100)% |
2 (0-100)% |
0.000 (0.00-100)% |
0.000 (0.00-100)% |
E1+E2 |
84 (82-87)% |
26 (21-32) % |
0.03 (0.01-0.10)% |
0.000 (0.00-0.00)% |
E1-3Glu |
93 (86-100)% |
54 (30-97)% |
2.4 (0.1-85)% |
0.20 (0.00-76)% |
E1-3Sul |
NA |
NA |
NA |
NA |
EE2 #1 |
100 (98-100)% |
98 (98-99)% |
91 (87-95)% |
85 (79-91)% |
EE2 #2 |
100 (98-100)% |
98 (98-99)% |
91 (88-95)% |
86 (81-91)% |
EE2 #3 |
100 (98-100)% |
99 (98-99)% |
92 (89-95)% |
87 (82-91)% |
The results in table 5-9 suggest that even when the sludge concentration is low and the transport time is short, appreciable losses of E1 and E2 could occur. If the sludge concentrations were as high as the
regulatory limits for STP effluents in Denmark or the EU, the samples would be spoiled if the transport time to the laboratory was 1 day. Therefore, samples from STPs should always be preserved before
they are transported to the laboratory.
5.6 Batch reactor characterisation
In order to know how well the batch experiments simulate the conditions in sewage treatment plants several parameters that characterise the reactors conditions were measured during the experiment. These
parameters were the oxygen concentration, pH, nitrate concentration and BOD1. The full details are given in Appendix 2 and only the main findings will be summarized here.
The reactors were kept at pH of 6.5 - 8.5, which is within tolerable limits for normal function of activated sludge bacteria. The denitrifying reactors had to have added acid regularly because the denitrification
consumes protons.
The aerobic reactors had an oxygen level about 7.25 mg O2/L which corresponds to ca. 80 % oxygen saturation. Therefore, the aerobic bacteria were well supplied with oxygen as in the aerobic tanks of
real STPs the typical concentration is 1-2 mg O2/L. In the denitrifying reactors the oxygen level was less than 0.1 mg O2/L. At this level no bacteria will function aerobically. In the denitrifying reactors nitrate
was consumed faster than 20 mg N·L-1·d-1. This together with the measured low oxygen concentration and the observed removal of protons (increased pH) provides evidence that the denitrifying reactors
were actually denitrifying.
The consumption of oxygen over 24 hours was similar between samples taken from the reactors on the three days of the experiment. However, some difference was observed between the denitrifying and
aerobic reactors. This indicates that the bacteria population or densities were not changing during the experiment. Thus, all parameters determined for the reactors indicate the reactors were operated in a
way that satisfactorily simulates the activated sludge tanks of a typical STP.
6 General discussion and conclusions
The existing literature on investigations on the fate of estrogens in sewage treatment plants (STPs) suggests that the central part in the process is the activated sludge system, where removal of nitrogen takes
place along with degradation of general organic matter. Most commonly such systems are operated both under aerobic and denitrifying conditions.
The two presumably most important removal processes in the activate sludge part of an STP, sorption to sludge particulate matter and degradation, were studied in the laboratory using activated sludge
from a modern Danish STP; Egå STP near the city of Århus (sorption also studied with sludge from Lundtofte STP). Egå STP is considered fairly representative of large STPs in Denmark, which handle the
vast majority of the municipal sewage. However, the plant appears to perform slightly better than the average (based on data on effluent quality of general parameters and NPO).
The estrogenic substances studied comprised the natural female steroid hormones estrone (E1) and 17β-estradiol (E2) as well as the active ingredient in most contraceptive pills, 17α-ethinylestradiol (EE2).
Furthermore, two conjugated forms of E1, estrone-3-glucuronide (E1-3Glu) and estrone-3-sulphate (E1-3Sul), were included in the study because estrogens are excreted from the human body as
conjugates and, thus, enter the sewage system in this form.
The sorption experiments included only E1, E2 and EE2 as free estrogens because the conjugated forms are very hydrophilic and, hence, not believed to be sorbed to any significant degree. The substances
were found to sorb almost quantitatively to the sludge within only about 0.5 hour. The (logarithmic) sorption equilibrium constant, Log Kd, was about 2.6 for E2 and EE2 in both types of sludge tested while
for E1 it was 2.8 in Egå sludge and 2.3 in Lundtofte sludge.
The latter result is most consistent with observations found in the literature i.e. slightly lower than for E2 and EE2. Values for Log Kd of E1, E2 and EE2 in the literature tend be a little higher than found in
this study. The results indicate that at common sludge densities in Danish STPs about 35-45% of E1 and 55-65% of E2 and EE2 (all unconjugated) can be expected to be sorbed to sludge.
The sorbed fractions in the activated sludge tank have implications for the prediction of stability of the steroid estrogens in the activated sludge tank. In the stability experiments with sludge the MLSS was 0.5
g DS/L, which is predicted to give a dissolved fraction of steroid estrogens of 76-92 %. In an activated sludge tank with a typical MLSS of 5 g DS/L only 24-52 % of the total estrogen concentration is
dissolved in water.
The degradation of E1, E2 and EE2 and two conjugated forms of E1 was studied under aerobic and anaerobic conditions in a simulated activated sludge system using sludge from Egå STP. An initial study
of the abiotic stability in water revealed that practically no degradation took place under such conditions and, hence, the observed degradation can be attributed to the action of microorganisms including
enzymatic reactions.
The aerobic degradation was very fast for E1 and E2 i.e. with half-lives of a few minutes or even lower at sludge concentrations commonly found at STPs, while the half-life of EE2 was more than 100 times
higher i.e. from 1.4 hours to 11 hours, depending on the sludge concentration (fastest degradation at highest concentration). The glucuronide conjugate of E1, E1-3Glu, was transformed at a slightly slower
rate than E1/E2 i.e. with a half-life of 2-16 minutes, whereas the transformation of the sulphate conjugate, E1-3Sul, took place at a rate more resembling that of EE2. In conclusion, the observed order of
aerobic degradability of the estrogens was:
E2 > E1 > E1-3Glu >> EE2 > E1-3Sul.
Under anaerobic conditions (not to be confused with anaerobic digestion of sludge), the degradation rates for E1 and EE2 in the activated sludge were considerably (10-20 times) lower than under aerobic
conditions while the degradation of E2 was not significantly changed i.e. still in the order of few minutes or lower depending on sludge concentration.
The observations on estrogen degradation are consistent with the international literature in which fast degradation in activated sludge systems at STPs is reported, however, with EE2 and E1-3SUL being
more slowly degraded than the others and the degradation of E1 being somewhat variable.
The measured degradation rate constants were used to estimate the stability
of steroid estrogens in Egå STP using the actual hydraulic retention times
in the activated sludge compartments. The estimation predicted that more than 99.9% of E1, E2 and E1-3Glu in the sewage would be removed while about 3.3 % of EE2 would still remain in the effluent. It
was not possible to predict the degradation rate of E1-3Sul. Based on these values and influent concentrations measured at other Danish STPs, the effluent concentrations of E1, E2 and E1-3Glu are
estimated to be close to zero, while a maximum concentration of 0.6 ng/L is estimated for EE2. These values are somewhat too low compared to monitoring results, probably because of effects of sorption,
which have not been possible to include in the predictive model.
The measured degradation rate constants were also used to estimate the stability of steroid estrogens in the effluent of Egå STP in order to predict if samples taken for analysis of steroid estrogens could be
transported without preservation to the analytical laboratory. Even with the unusually low concentration of activated sludge in Egå STP of 5 mg DS/L, the concentrations of E1, E2 and E1-3Glu would be
reduced significantly after only 3 hours. Therefore, all samples for estrogen analysis should always be preserved, e.g. by acidification with sulphuric acid, prior to transportation.
It is important to recognise that the predictions made for the two removal processes (sorption and degradation) are made independently of each other, while in practice they most likely interact. In practice,
degradation of steroid estrogens will only take place in the water phase. If the dissolved fraction of the steroid estrogens is changed, the degradation rate is probably changed proportionally. In the laboratory
experiments, the dissolved fraction of steroid estrogens was estimated to constitute only about one third of the total amount.
This implies that the estimated removal in the activated sludge tanks for Egå STP may be overestimated with about 200%. If the sorption to sludge takes place fast and the desorption rate is slower than the
degradation rate (neither have currently been measured), then the desorption reaction will be rate limiting for the overall degradation of the estrogens. This means that the degradation rate will be reduced to
that of the desorption as soon as the first 25-50 % of the initial concentrations of the steroid estrogens have been degraded.
7 References
Andersen, H.R., Siegrist, H., Halling-Sørensen, B. & Ternes, T.A. (2003). Fate of Estrogens in a Municipal Sewage Treatment Plant. Environmental Science and Technology. Vol. 37 No. 18, pp.
4021-4026.
Dansk Standardiseringsråd (1991). DS 204 - Tørstof og Gløderest.
In: "Standarder for Vand og Miljø - Fysiske og kemiske metoder"; 22-25.
D'Ascenzo, G., Corcia, A Di, Gentili, A., Mancini, R., Mastropasqua, R., Nazzari, M. & Samperi, R. (2003). Fate of natural estrogen conjugates in municipal sewage transport and treatment facilities. The
Science of the Total Environment, Vol. 302 pp. 199-209.
DEPA (1996). Activated Sludge Biodegradability Simulation Test. Environmental Project No. 337, 1996. Danish Environmental Protection Agency.
DEPA (2002a). Punktkilder 2001. Orientering fra Miljøstyrelsen Nr. 7, 2002. (Point Sources 2001. Danish Environmental Protection Agency. In Danish).
DEPA (2002b). Feminisation of fish - The effect of estrogenic compounds and their fate in sewage treatment plants and nature. Environmental Project No. 729, 2002.
DEPA (2003a). Hormonforstyrrende stoffer og lægemidler i spildevand. Miljøprojekt Nr. 799, 2003 fra Miljøstyrelsen. (Endocrine Disruptors and Pharmaceuticals in Municipal Wastewater. In Danish with
an English summary).
DEPA (2003b). Evaluation of Analytical Chemical Methods for Detection of Estrogens in the Environment. Working Report No. 44, 2003. Danish Environmental Protection Agency.
DEPA (2003d). Punktkilder 2002. Orientering fra Miljøstyrelsen Nr. 10, 2003. (Point Sources 2002. Danish Environmental Protection Agency, 2003. In Danish).
Geyer H.J., Rimkus G., Scheunert I., Kaune A., et al. (2000). Bioaccumulation and Occurence of Endocrine-Disrupting Chemicals (EDCs), Persistent Organic Pollutants (POPs), and Other Organic
Compouds in Fish and Other Organisms Including Humans. In:Bioaccumulation (Beek B, ed), Heidelberg, Germany:Springer-Verlag Berlin, 2000.
Holthaus K.I.E., Johnson A.C., Jürgens MD, Williams R.J., et al. (2002). The potential for estradiol and ethinylestradiol to sorb to suspended and bed sediments in some English rivers.
Environ.Toxicol.Chem.; 21: 2526-2535.
Johnson, A.C. & Sumpter, J.P. (2001). Critical Review: Removal of Endocrine-Disrupting Chemicals in Activated Sludge Treatment Works. Environmental Science and Technology. Vol. 35 No. 24, pp.
4697-4703.
Joss, A. (12-9-2003). Personal Communication from Dr. Adriano Joss from EAWAG about oxygen saturation in aeration tanks of active sludge STPs.
Joss, A., Andersen, H.R., Ternes T., Richle, P.R. et al. (2003). Removal of estrogens in municipal wastewater treatment under aerobic and anaerobic conditions: consequences for plant optimization
(Unpublished).
Kirk, L.A., Tyler, C.R., Lye, C.M., Sumpter, J.P. (2002). Changes in estrogenic and androgenic activities at different stages of treatment in wastewater treatment works. Environmental Toxicology and
Chemistry. Vol. 21 No. 5, pp. 972-979.
Lai K.M., Johnson K.L., Scrimshaw M.D., Lester J.N. (2000). Binding of waterborne steroid estrogens to solid phases in river and estuarine systems. Environmental Science & Technology; 34:
3890-3894.
Layton A.C., Gregory B.W., Seward J.R., Schultz T.W., et al. (2000). Mineralization of steroidal hormones by biosolids in wastewater treatment systems in Tennessee U.S.A. Environmental Science and
Technology; 34: 3925-3931.
Löffler D, Herrmann N, Alder A, Bago B, et al. (2003). Fate and Analysis of PPCPs in sludge and sediments. POSEIDON Symposion - Presentation of Project Results, Braunschweig, October 2003; 19
(Abstract)
Matsui, S., Takigami, H., Taniguchi, N., Adachi, J., Kawami, H. & Shimizu, Y. (2000). Estrogen and estrogen mimics contamination in water and the role of sewage treatment. Water Science and
Technology. Vol. 42 No. 12, pp. 173-179.
Matter-Müller C., Gujer W., Giger W., Stumm W. (1980). Non-biological elimination mechanisms in biological sewage treatment plant. Progress in Water Technology; 12: 299-314.
NERI (2002). Vandmiljø 2002. Tilstand og udvikling - faglig sammenfatning. Faglig rapport fra DMU nr. 423, 2002. (Water Environment 2002. National Environmental Research Institute. In Danish).
Metcalfe, C.D., Metcalfe, T.L., Kiparissis, Y., Koenig, B.G. (2001). Estrogenic potency of chemicals detected in sewage treatment plant effluents as determined by in vivo assays with Japanese medaka
(Oryzias latipes). Environmental Toxicology and Chemistry vol. 20, pp. 297-308.
Svenson, A., Allard, A.-S. & Ek, M. (2003). Removal of estrogenicity in Swedish municipal treatment plants. Water Research Vol. 37, pp. 4433-4443.
Tanaka, H., Yakou, Y., Takahashi, A., Higashitani, T., Tomori, K. (2000). Comparison between estrogenicities estimated from DNA recombinant yeast assay and from chemical analyses of endocrine
disruptors during sewage treatment. Conference Preprint Book 1: Industrial Wastewater Treatment.
1st World Water Congress of IWA, Paris 3-7 July 2000.
Ternes, T.A., Stumpf, M., Mueller, J., Haberer, K., Wilken, R.-D., Servos, M. (1999a). Behavior and occurrence of estrogens in municipal sewage treatment plants - I. Investigations in Germany, Canada
and Brazil. The Science of the Total Environment 225, pp. 81-90.
Ternes, T.A., Kreckel, P., Mueller, J. (1999b). Behavior and occurrence of estrogens in municipal sewage treatment plants - II. Aerobic batch experiments with activated sludge. The Science of the Total
Environment 225, pp. 81-90.
Ternes, T.A., Andersen H.R., Gilberg D., Bonerz M. (2002). Determination of estrogens in sludge and sediments by liquid extraction and GC/MS/MS. Analytical Chemistry 74: 3498-3504.
Thorpe, K.L., Hutchinson, T.H., Hetheridge, M.J., Scholze, M. (2003). Relative potencies and combination effects of steroidal estrogens in fish. Environmental Science and Technology Vol. 37, pp.
1142-1149.
US EPA (1996a). Draft guideline "OPPTS 835.1110 Activated Sludge Sorption Isoterm".
US EPA (1996b). Draft guideline "OPPTS 835.1220 Sediment and Soil Adsorption/Desorption Isoterm".
Vader, J.S., van Ginkel, C.G., Sperling, F.M.G.M., de Jong, J. et al. (2000). Degradation of ethinylestradiol by nitrifying activated sludge. Chemosphere 41: 1239-1243.
Wegener, G., Persin, J., Karrenbrock, F., Rörden, O. et al. (1999). Vorkommen und Verhalten von natürlichen und synthetischen Estrogenen und deren Konjugate in der aquatischen Umwelt (Presence and
Behaviour of Natural and Synthetic Estrogens and their Conjugates in the Aquatic Environment). Vom Wasser 92: 347-360.
Appendix 1:
Instrumentation details
BOD5: WTW Oxitop OC100/ Oxitop C100. Method according to WTW Application report No. BOD 266230.
Data fitting and statistics: GraphPad Prism version 3.00/4.00 for Windows, GraphPad Software, San Diego California USA, www.graphpad.com".
Nitrate: Merck Nitrate Test 0.2-25,0 mg/L NO3--N Spectroquant® #114773. Quantified by adsorption at 517,1 nm as measured with a Specord S100 from Carl Zeiss Technology.
Oxygen: WTW Oxi323.
pH: Radiometer PHM95.
Steroid Estrogens analysis: HPLC separation was done with a C18 XTerra®RP18 3,5m3.0x150mm column and gradient elution by H2O:MeOH from 35:65 to 0:100. Ionisation by Atmospheric
Pressure Photo Ionisation (APPI) with the Sciex API 3000 triplequad-MS-MS for detection.
Appendix 2:
Batch reactor characterisation
In order to know how well the batch experiments simulate the conditions in sewage treatment plants several parameters that characterise the reactors conditions were measured during the experiment. These
parameters were the oxygen concentration, pH, nitrate concentration and BOD1.
Oxygen
In reactors with air as the oxidation source, the O2–concentration was 7.25 ± 1.25 mg/L during the experiments. At the experimental conditions the saturation concentration of O2 in contact with the
atmosphere is about 9 mg/L. In the aeration step of STP the typical concentration of O2 is around 2-3 mg/L (Joss, 2003). Thus, that consumption of oxygen took place apparent, but the oxygen
concentration in the reactors was higher than is common in the aeration tank of a real STP.
Further, since the active sludge density in the reactor is only about a tenth of the normal density in STPs the concentration of oxygen consuming bacteria is less in the batch experiment than in the real STP. If
the air flow was reduced sufficiently to reduce the oxygen saturation to 2-3 mg/L in the reactor the stirring that the air bubbles create was not sufficient to keep the active sludge particles (flocks) in
suspension. It is assumed that this difference does not affect the metabolic potential of the aerobic bacteria as the enzymes in aerobic sludge bacteria are already about 90 % saturated with O2 at an oxygen
concentration of 2 mg/L (Joss, 2003).
In reactors with nitrate as oxidation source O2–concentration was less than 0,1 mg/L throughout the experiment. At this low concentration of oxygen the bacteria would have to use nitrate as their only
oxidation source.
Nitrate
In reactors with nitrate as oxidation source the nitrate is consumed and has to be replenished twice daily. A high consumption of nitrate above 20 mg-N·l-1·d-1 is observed over the entire experimental
period as shown in figure 8. This shows that the bacteria are actively degrading the organic material of the artificial sewage by denitrification. Apparently the nitrate consumption increased in the last day of the
experiment. This could be due to an increase in the bacteria biomass or change of the species composition towards bacteria, which are more efficient metabolizers under denitrifying conditions.
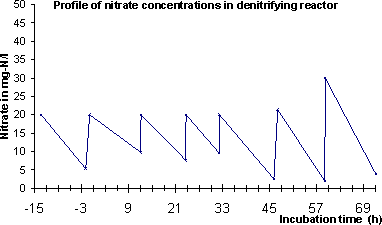
Figure 0-1:
Profile of nitrate concentrations in the batch experiment with denitrifying bacteria.
pH
pH was measured at selected times during the experimental period. pH was almost stable in the aerobic reactors, while an increase in pH was observed in the denitrifying reactor over time. See figure 9. To
keep pH between 6,5 and 8,5, mineral acid was added two to four times daily.
In the denitrifying reactor the continuous tendency for increase of pH is explained by consumption of protons during denitrification:
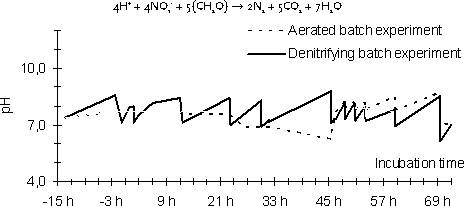
Figure 0-2:
Profile of pH in oxygenated and denitrifying batch experiments. The pH of the denitrifying reactors was repeatedly adjusted to pH = 7 with acid.
Bacterial activity estimated by BOD1
If the bacterial biodegradation activity in the batch experiment reactors changed significantly over the time of the experiment the assumed first order degradation kinetics for the estrogens which is assumed in
the data treatment would not be valid.
To estimate how much the bacterial activity changed during the experiment a sample was taken from the aerated and the denitrifying reactors at the beginning of the experiment and every 24 h of the
experiment. The samples were transferred to WTW BOD bottles, which is normally used to measure oxygen consumption of water samples. The oxygen consumption was measured for 24 h for each
sample. The oxygen consumption profiles could then be compared between samples taken from the reactor on the three days of the experiment as shown in figure 10. It is seen that the biodegradation
activity of the active sludge suspensions did not change much between the three days of the experiment. However, the activity varies between sludge suspensions taken from the aerated and the denitrifying
batch experiments.
Click here to see Figure 0-3.
| Top | | Front page |
Version 1.0 November 2004, © Danish Environmental Protection Agency
|