| Front page | | Contents | | Previous | | Next |
Degradation of Estrogens in Sewage Treatment Processes
5 Results and discussion
5.1 Sorption
5.1.1 Sorption kinetics
The time until sorption equilibrium was established was estimated by measuring water concentrations in an experiment with 1 g/L sludge and a water concentration of the respective steroid estrogen of 400
ng/L at different times after addition of the steroid estrogen. The results of these experiments are shown in Figure 5-1.
Click here to see Figure 5-1.
Between 87 % and 97 % of the loss of estrogens from the water phase had occurred in 0.5 hours. For E1 and EE2 no difference between two hours and six hours equilibrium time could be seen (less than 3
% change). It is therefore concluded that the sorption reaction had reached equilibrium after two hours. For E2 a tendency for a further decrease in the water concentration is seen after six hours (further 11
% change). The three steroid estrogens are similar in size and lipophilicity, therefore the sorption kinetic should be similar for the three. It is assumed that the apparent lower water concentrations of E2 at six
hours reaction time is an artefact of the random error on the concentration determination since no explanation based on the physical-chemical properties can be given. Based on this experiment the
equilibrium time used for the experiments to determine the sorption isotherms was set to three hours.
5.1.2 Isotherms
Isotherms from the sorption experiments with the three steroids are shown in Figures 5-2 and 5-3.
Click here to see Figure 5-2.
Click here to see Figure 5-3.
The Freundlich sorption isotherm defines the equilibrium between concentrations of a chemical in water and solid (Schwarzenbach et al., 2003):
CS = KF·CWn
Equation 1:
The Freundlich equation for describing sorption isotherms (Schwarzenbach et al., 2003). Cs is the concentration in the solids in ng/kg, Cw is the concentration in water in ng/L and KF and n
are the Freundlich parameters that characterise the sorption isotherm.
The parameters for the Freundlich equation were calculated by non-linear curve fitting as shown in Table 5-1.
Table 5-1
Parameters for the Freundlich equation description of the sorption isotherms for steroid estrogens to activated sludge from Egå and Lundtofte STPs.
|
Egå STP |
Lundtofte STP |
E1 |
E2 |
EE2 |
E1 |
E2 |
EE2 |
KF (l/kg ) |
822±918 |
594±281 |
267±257 |
89 ±105 |
1106 ±627 |
383±245 |
n |
0.945±0.146 |
0.926±0.064 |
1.080±0.120 |
1.154 ±0.155 |
0.770 ±0.093 |
1.020±0.092 |
r² |
0.82 |
0.94 |
0.95 |
0.75 |
0.93 |
0.81 |
Replicates |
12 |
14 |
23 |
9 |
8 |
11 |
The width of the 95 % confidence range for the Freundlich parameters, KF, shown in table 5-1 is in the order of 65-110 % of the estimated value of the parameter. This suggests that when these constants
are used for calculating conditional Kd-values and estimating the sorbed fractions of steroid estrogens (tables 5-2 and 5-4), the errors on the calculated results should be in that range. Estimation of the errors
on parameters with non-linear fitting using a generalised method is a very weak statistical method, which tends to give estimates of variation much higher than what is intuitively expected from the variation in
the data. This is due to the non-specificity of the method as it designed to apply to all types of non-linear expressions.
The calculations of the inter-experimental errors on the Freundlich parameters give confidence ranges above 50 % of the parameter values. However, when the experiments were repeated (data not shown)
during the method optimization (2-3 independent experiments), the variations between experiments were only in the order of 15 % of the parameter values. Therefore, it is believed that the realistic
confidence range is rather in the order of ±25 %.
5.1.3 Kd-values
Kd-values can be calculated for representative water concentrations based on the Freundlich isotherms as:
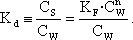
Equation 2:
Formula for generating conditional Kd-values for steroid estrogens at different water concentrations from the Freundlich equation.
By inserting relevant values for water concentrations of steroid estrogens into Equation 2 a table of Kd-values can be generated as shown in Table 5-2. It should be noted that the lower concentrations
chosen for the table are extrapolations below the experimental concentrations range, which may hide systematic deviation, not covered in the experiment.
Table 5-2
Calculated conditional Kd's for steroid estrogen sorption to activated sludge from Egå and Lundtofte STPs based on the fitted parameters for the Freundlich equation description of the sorption isotherms.
Cw |
Egå STP |
Lundtofte STP |
LogKD,E1 |
LogKD,E2 |
LogKD,EE2 |
LogKD,E1 |
LogKD,E2 |
LogKD,EE2 |
ng/L |
l/kg |
l/kg |
l/kg |
l/kg |
l/kg |
l/kg |
10 |
2.86 |
2.70 |
2.51 |
2.10 |
2.81 |
2.60 |
20 |
2.84 |
2.68 |
2.53 |
2.15 |
2.74 |
2.61 |
50 |
2.82 |
2.65 |
2.56 |
2.21 |
2.65 |
2.62 |
100 |
2.80 |
2.63 |
2.59 |
2.26 |
2.58 |
2.62 |
200 |
2.79 |
2.60 |
2.61 |
2.30 |
2.51 |
2.63 |
500 |
2.77 |
2.57 |
2.64 |
2.36 |
2.42 |
2.64 |
1000 |
2.75 |
2.55 |
2.67 |
2.41 |
2.35 |
2.64 |
The sorption isotherms for E2 and EE2 are very similar for sludge from the two STPs. E1 apparently sorbs stronger to sludge from Egå STP than E2 and EE2 compared to sludge from Lundtofte STP where
E1 sorbs less than E2 and EE2.
The difference in sorption tendency between E1, E2 and EE2 found in the experiment with sludge from Lundtofte appears to agree better than that from Egå with the Kd's that are estimated from Kow's by a
specific QSAR equation for sorption of lipophilic chemicals to activated sludge as shown in Table 5-3. This approach estimates that the Kd–value for E1 should be less than or equal to Kd for E2 and EE2.
Therefore the Kd determined for sorption of E1 to sludge from Egå STP should be used with caution.
Table 5-3
Kow-values for the steroid estrogens according to three sources and predicted
Kd-values based on a QSAR (Matter-Müller et al., 1980) developed for lipophilic chemicals that uses Kow to estimate the Kd for activated sludge.
|
Log(Kow) |
Log(Kd) |
Reference |
E1 |
E2 |
EE2 |
E1 |
E2 |
EE2 |
Lai et al., 2000 |
3.43 |
3.94 |
4.15 |
2.69 |
3.03 |
3.17 |
Holthaus et al., 2002 |
|
3.1 |
3.9 |
|
2.5 |
3.0 |
Geyer et al., 2000 |
4.1 |
4.0 |
4.2 |
3.1 |
3.1 |
3.2 |
5.1.4 Prediction of sorbed fractions
In the stability experiments with activated sludge the content of dry solids (DS) is 0.5 g/L. In conventional STP the activated sludge is operated between 3 and 5 g DS/l. In Egå STP the MLSS in the effluent
is typically 0.005 g DS/L, while regulations in Denmark and the EU allow maximally 0.030 g DS/L and 0.050 g DS/L, respectively, in STP effluents.
Using the conditional Kd-values at the 100 ng/L concentration level for Lundtofte sludge, the fraction of steroid estrogens that would be sorbed at equilibrium in these sludge densities is calculated with
equation 3 as shown in table 5-4.

Equation 3
Equation for calculating the sorbed fraction of the total steroid estrogen concentrations at different densities of activated sludge (MLSS).
If the MLSS in the activated sludge tank and the excess sludge production (mass of activated sludge removed from the STP per volume of treated sewage) (RESS) is known the fraction of the total steroid
estrogen load into the activated sludge tank that will be removed at equilibrium assuming no degradation occurs can be calculated as follows (Equation 4).

Equation 4
Equation for calculating the removed fraction of the steroid estrogen load at a given densities of activated sludge (MLSS) and Removal rate for Excess Suspended Solids (RESS).
A typical value for the removal of excess activated sludge from an STP is 100 mg/L of treated sewage (Henze et al., 2002). This figure is derived from the daily production of excess sludge divided by the
daily wastewater flow. As mentioned it is typical that MLSS in the activated sludge tank is 4 g DS/L. If these values are used, the removal of steroid estrogens with activated sludge can be estimated as
shown in table 5-4.
Table 5-4
Predicted sorbed fraction for representative sludge densities using Kd values taken from the sorption isotherms at CW = 100 ng/L and equation 3 and 4.
|
|
Egå |
Lundtofte |
MLSS |
E1 |
E2 |
EE2 |
E1 |
E2 |
EE2 |
Log(Kd) |
|
2.80 |
2.63 |
2.58 |
2.26 |
2.58 |
2.62 |
Fractions of steroid estrogens sorbed in the activated sludge tank at high MLSS. |
5.0 g/L ds |
76% |
68% |
66% |
48% |
66% |
68% |
Fractions of steroid estrogens sorbed in the activated sludge tank at medium MLSS. |
4.0 g/L ds |
72% |
63% |
60% |
42% |
60% |
63% |
Fractions of steroid estrogens sorbed in the activated sludge tank at low MLSS. |
3.0 g/L ds |
65% |
56% |
53% |
35% |
53% |
56% |
Fractions of steroid estrogens sorbed in the stability experiments |
0.5 g/L ds |
24% |
18% |
16% |
8.3% |
16% |
17% |
Fractions of steroid estrogens load removed with excess sludge assuming sludge production is 100 mg/L ds and the MLSS in the
activated sludge tank is 4 g/L ds. |
100 mg/L ds |
1.8% |
1.6% |
1.5% |
1.1% |
1.5% |
1.6% |
Fractions of steroid estrogens sorbed in the STP effluent at highest daily SS allowed by EU regulations |
50 mg/L ds |
3.1% |
2.1% |
1.9% |
0.90% |
1.9% |
2.0% |
Fractions of steroid estrogens sorbed in the STP effluent at highest average SS allowed by Danish regulations |
30 mg/L ds |
1.9% |
1.3% |
1.1% |
0.54% |
1.1% |
1.2% |
Fractions of steroid estrogens sorbed in the STP effluent at the actual SS for Egå STP |
5 mg/L ds |
0.31% |
0.21% |
0.19% |
0.091% |
0.19% |
0.21% |
The sorbed fractions in the activated sludge tank have implications for the predictions of the degradation of the steroid estrogens in the activated sludge tank, which is discussed in Section 5.5. In the stability experiments with sludge the MLSS was 0.5 g DS/L, which is predicted to give a dissolved fraction of steroid estrogens of 92-76 %. In an activated sludge tank with high MLSS of 5 g DS/L, only 52 – 24 % of the total estrogen concentration is dissolved in water. Presumably bacteria only degrade the dissolved steroid estrogens. When 48-76 % of the steroid estrogens are not in the water phase the degradation rate will be reduced proportionally. Further, if the desorption from the sludge takes place at a lower rate than that of the degradation in water, the desorption reaction becomes the rate limiting step for the overall degradation of the steroid estrogens.
The predicted sorbed fractions also have consequences for the design of the analytical procedures for such experiments. Thus, if one wishes to measure the steroid estrogens in a sample from an activated
sludge tank, both water and sludge should be extracted and analysed. If only the water phase is analysed, up to 76 % of the steroid estrogens may be missed. Contrary to this, if the sample was taken from
the effluent, the error introduced by only extracting the water phase could maximally be 3.1 %.
A practical implication of the above is that when the concentrations of effluent suspended solids are below the EU or national regulations, the sorbed fraction is of minor significance.
5.2 Abiotic degradation in water without activated sludge
As shown in Figure 5-4 no clear tendency for reduction of the three steroid estrogens were seen. Also no trace of E1 was seen in the experiment with E2. This could have indicated that auto-oxidation of E2
occurred. Similarly, no trace of E2 could be found in the experiment with E1. This would have occurred if abiotic reduction of E2 occurred during the test.
No clear sign of formation of E1 by hydrolysis of either E1-3Glu or E1-3Sul was seen in the stability experiment.
Fitting of the concentration profiles in figures 5-4 and 5-5 to the first order kinetics equations in Box 1 (Figure 4-3) yielded degradation constants from which T½ could be calculated. These values are shown
in table 5-6. It is seen that the lower 95 % confidence limit for T½ is higher than 9 days for each chemical. Further, except for E2 and one of the four EE2 replicates, the fitted first order rate constants were
not significantly different from zero at the 95 % confidence level.
Table 5-5
First order reaction constants and half-lives for steroid estrogens in the abiotic experiments. The 95 % confidence limit on the values that has been estimated from the variation found within each experiment is
shown in brackets. The value for T½ and its higher confidence limit could not be calculated for E1-3Sul.
Compound |
k, abiotic |
T½ |
kE1, abiotic |
-0.019 |
±0.019 |
L·g-1·d-1 |
NA |
d |
kE2, abiotic |
0.042 |
±0.039 |
L·g-1·d-1 |
16 (9 - 196) |
d |
kE1-3Glu, abiotic |
-0.0080 |
±0.050 |
L·g-1·d-1 |
NA |
|
kE1-3Sul, abiotic |
-0.057 |
±0.082 |
L·g-1·d-1 |
(> 26.4 ) |
d |
kEE2, abiotic #1 |
0.039 |
±0.036 |
L·g-1·d-1 |
18 (9 - 217) |
d |
kEE2, abiotic #2 |
0.0038 |
±0.022 |
L·g-1·d-1 |
184 (> 26.4) |
d |
kEE2, abiotic #3 |
-0.0071 |
±0.030 |
L·g-1·d-1 |
NA |
|
kEE2, abiotic #4 |
0.011 |
±0.015 |
L·g-1·d-1 |
64 (> 27.3) |
d |
Click here to see Figure 5-4.
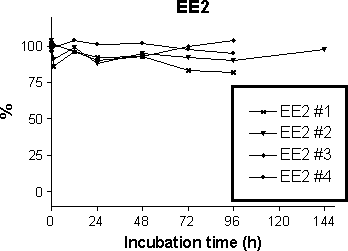
Figure 5-5
Degradation of 500 ng/L EE2 in four independent abiotic experiments.
5.3 Degradation in aerobic activated sludge suspension
Significant degradation of the five steroid estrogens was found except for E1-3Sul. E2 was degraded the fastest followed by E1. It appears that the only degradation process for E2 is oxidation to E1 since
the sum of E1 and E2 was approximately constant in the first minutes of the degradation experiments with E2. EE2 was the most stable of the estrogens.
Click here to see Figure 5-6.
The degradation experiment with EE2 was made as triplicate independent experiments and therefore the variability between reactors could be estimated. The half lives in the three experiments varied
between 10 h and 12 h. The contribution to the error on estimating the degradation rates from variation between reactors is thus in the order of 10 %, which is not more than could be expected when the
error on the chemical analysis of steroid estrogens is considered.
The stability of E1-3Glu was not much higher than the stability of E1. Therefore only a small peak in concentrations of E1 was found in the experiment with E1-3Glu.
In the experiment with E1-3Sul no significant amount of E1 was detected during the three days of incubation. It is therefore concluded that E1-3Sul is degraded slower than E1 in this experiment.
The first order reaction rate constants obtained by fitting the concentration curves can be used to calculate half lives of each test compound using the formula in Box 1 (Figure 4-3). In the batch experiments,
the sludge concentration is 0.5 g/L whereas a typical sludge density found in the activated sludge treatment step of an STP is about 4 g/L. The calculated values are shown in table 5-6.
Table 5-6
First order reaction constants and half-lives for steroid estrogens in experiments with activated sludge in the aerobic reactors. The 95 % confidence limit on the values that has been estimated from the
variation found within each experiment is shown in brackets. The value for T½ and its higher confidence limit could not be calculated for E1-3Sul.
Compound |
k, aerobic |
T½ at MLSS = 0.5 g/L |
T½ at MLSS = 4 g/L |
kE1,bio,aerob |
405 |
±158 |
L·g-1·d-1 |
4.9 |
(3.5 - 8.1) |
min |
0.62 |
(0.44 - 1) |
min |
kE2,bio,aerob |
768 |
±419 |
L·g-1·d-1 |
2.6 |
(2 - 6) |
min |
0.32 |
(0.21 - 1) |
min |
kE1+E2,bio,aerob |
271 |
±183 |
L·g-1·d-1 |
7.4 |
(4 - 23) |
min |
0.92 |
(0.55 - 3) |
min |
kE1-3Glu,bio,aerob |
124 |
±65 |
L·g-1·d-1 |
16 |
(11 - 34) |
min |
2.0 |
(1.32 - 4) |
min |
kE1-3Sul,bio,aerob |
-60 |
±1670 |
L·g-1·d-1 |
(> 1.24) |
min |
(> 0.15) |
min |
kEE2,bio,aerob #1 |
3.3 |
±1.4 |
L·g-1·d-1 |
10 |
(7 - 18) |
h |
1.3 |
(0.89 - 2.3) |
h |
kEE2,bio,aerob #2 |
3.0 |
±1.2 |
L·g-1·d-1 |
11 |
(8 - 18) |
h |
1.4 |
(0.99 - 2.2) |
h |
kEE2,bio,aerob #3 |
2.8 |
±1.0 |
L·g-1·d-1 |
12 |
(9 - 18) |
h |
1.5 |
(1.08 - 2.3) |
h |
The half-lives presented in Table 5-6 for the aerobic degradation in sludge at 4 g MSLL/l may well underestimate the real time required to reduce the total concentration of the estrogens if the desorption
from sludge particles is slower than the degradation reactions (this is, however, not known and has not been possible to investigate in this study).
5.4 Degradation in denitrifying activated sludge suspension
When oxygen was excluded from the activated sludge slurry and bacteria had to use nitrate as electron donor, the degradation rates for E1 and EE2 were reduced significantly (10- to 20-fold), whereas the
degradation of E2 was not markedly changed.
Click here to see Figure 5-7.
Table 5-7
First order reaction constants and half-lives for steroid estrogens in experiments with activated sludge in the denitrifying reactor. The 95 % confidence limits on the values (estimated from the variation found
within each experiment) are shown in brackets. A highest confidence limit could not be calculated for the T½ of EE2.
Compound |
k, denitrifying |
T½ at MLSS = 0.5 g/L |
T½ at MLSS = 4 g/L |
kE1, bio, anaerob |
14 |
±5 |
L·g-1·d-1 |
143 |
(103 - 231) |
min |
18 |
(13 - 29) |
min |
kE2, bio, anaerob |
497 |
±283 |
L·g-1·d-1 |
4.0 |
(3 - 9) |
min |
0.50 |
(0.32 - 1) |
min |
kE1+E2, bio, anaerob |
27 |
±21 |
L·g-1·d-1 |
75 |
(42 - 355) |
min |
9.3 |
(5.2 - 44) |
min |
kEE2, bio, anaerob |
0.17 |
±0.17 |
L·g-1·d-1 |
8.3 |
(> 5884) |
d |
1.0 |
(> 735) |
d |
5.5 Predictions of steroid estrogens stability
5.5.1 Loss during treatment at STPs
The results of the degradation experiments with activated sludge suspensions performed in the laboratory can be used to estimate the loss of the steroid estrogens during the activated sludge treatment in a
real STP. The remaining total concentration after treatment in each compartment is calculated from the experimentally determined first order reaction constant for the relevant steroid estrogen and
RedOx-environment from tables 5-6 and 5-7, using Equation 5.
The combined removal after treatment in both the aerobic and the denitrifying activated sludge compartments are calculated as the product of the remaining fractions from each treatment step as shown in
Equation 6.
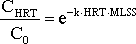
Equation 5
Equation for calculating the remaining fraction of the steroid estrogen total concentration after treatment in a given RedOx-environment/compartment of the activated sludge treatment with a given activated sludge concentration (MLSS) for a time period equal to the average hydraulic retention time HRT of the compartment.

Equation 6
Equation for calculating the remaining fraction of the steroid estrogen total concentration after degradation of the steroid estrogen in both the aerobic and the denitrifying activated sludge
treatment.
The data for the STP (hydraulic retention times (HRT) in compartments and MLSS) used in this scenario are those of Egå STP from where the sludge used in the degradation experiments originated. For
Egå STP the average HRT in the denitrifying tank is 1.5 hours and the average HRT in the aerobic tank is 6.7 hours. The HRT in the aerobic tank is longer than the denitrifying tank because nitrification,
which occurs only in aerobic conditions, is the slowest of the nitrogen conversion processes in STPs. The typical MLSS is
4 g DS/l in Egå STP. With these parameters and the calculated reaction rate constants, the removal of steroid estrogens is estimated as shown in table 5-8.
Table 5-8
Prediction of the fraction of steroid estrogens remaining after activated sludge treatment in Egå STP.
RedOX |
Aerobic |
Denitrifying |
Combined |
MLSS |
4.0 g DS/L |
4.0 g DS/L |
4.0 g DS/L |
HRT in activated sludge compartment |
0.28 day |
0.06 day |
0.34 day |
Compound |
|
|
|
E1 |
0.000 (0.00-0.00)% |
3.0 (0.8-12)% |
0.000 (0.00-0.00)% |
E2 |
0.000 (0.00-100)% |
0.000 (0.00-0.00)% |
0.000 (0.00-0.00)% |
E1+E2 |
0.000 (0.00-0.00)% |
0.12 (0.00-25)% |
0.000 (0.00-0.00)% |
E1-3Glu |
0.000 (0.00-0.19)% |
ND |
NA |
E1-3Sul |
NA |
ND |
NA |
EE2 #1 |
2.6 (0.55-12)% |
96 (92-100)% |
2.5 (0.5-13)% |
EE2 #2 |
3.4 (0.89-13)% |
96 (92-100)% |
3.3 (0.8-13)% |
EE2 #3 |
4.2 (1.4-13)% |
96 (92-100)% |
4.0 (1.2-13)% |
The values in Table 5–8 for the combined removal efficiency of estrogens in the activated sludge system at Egå STP allow the effluent concentrations to be estimated if the influent concentrations are known.
Since these data do not exist for Egå STP, an estimate can be based on the influent concentrations measured at two other Danish STPs (Avedøre and Usserød, see Table 3–1). For E1 and E2, the predicted
effluent concentration is 0 ng/L, since the predicted removal rate is 100 %. The predicted effluent concentration for EE2 is 0.16 (0.04 – 0.6) ng/L based on the highest influent concentration measured at
Usserød STP (4.8 ng/L). All these concentrations are below the limit of quantification of standard analytical methods today.
As appears from Table 3-1, the measured effluent concentrations at Avedøre STP are higher than predicted, while the values from Usserød STP are closer to the predicted values. The difference between
the effluent concentrations at these two plants is probably due to the shorter total sludge retention time (SRT) at Avedøre compared to Usserød (20-25 and 33 days, respectively), and maybe also to the fact
that Usserød has a sand filter installed after the clarifier tank. The SRT at Usserød STP is almost as high as that of Egå STP.
As discussed previously, the predictions of the removal efficiency do not take into consideration the effects of sorption in the activated sludge tank, which may reduce the effective degradation rate. E.g.
steroid estrogens that have been sorbed in the activated sludge tank may be released from the activated sludge during the subsequent settling process in the clarifier. Hence, the predicted removal rates are
probably too high
5.5.2 Loss during transport of samples
In the part of the scientific community that researches the fate of steroid estrogens in STPs and measure steroid estrogen concentrations in STP effluents there is an ongoing discussion about procedures for
conserving and transporting samples for analysis. The scenario in table 5-8 clearly suggests that samples must be conserved immediately irrespectively of the RedOx environment in the sample (presumably
they will quickly become anaerobic from the respiration in the activated sludge). In tables 5-6 and 5-7 the half-lives for E1 and E2 are estimated to be about or less than 1 min in the activated sludge
suspension. Therefore, addition of one or more preserving chemicals is important immediately after sampling. The recommended preservation method is addition of sulphuric acid. Further, a silver salt may be
added to the samples, which should be stored at less than 4 °C.
The results of the stability experiments with activated sludge suspensions may also be used to estimate the stability of steroid estrogens in samples of STP effluents where low concentrations of activated
sludge is found as mentioned in Section 5.1.4 and Table 5-4. Samples are often taken far from the laboratory and, hence, due to logistic difficulties the time from sampling to extraction may be as much as 24
hours. However, in this project the time between sampling at Egå STP and sample treatment was initiated at DFU was only about 3 hours (0.13 days). If it is assumed that samples remain aerobic until they
reach the laboratory, the estimated stability of estrogens in the samples at the sludge concentrations discussed in Section 5.1.4 and Table 5-4 will be as shown in table 5-9.
Table 5-9
Fraction of steroid estrogens predicted to remain in a sample of a STP effluent after transport assuming no preservation is used for the sample.
Effluent MLSS for |
Egå (actual) |
Danish regulation |
EU-regulation |
MLSS |
0.005 g DS/L |
0.005 g DS/L |
0.030 g DS/L |
0.050 g DS/L |
Sample transport time |
0.13 day |
1 day |
1 day |
1 day |
Compound |
|
|
|
|
E1 |
78 (75-80)% |
13 (10-18)% |
0.000 (0.00-0.00)% |
0.000 (0.00-0.00)% |
E2 |
62 (37-100)% |
2 (0-100)% |
0.000 (0.00-100)% |
0.000 (0.00-100)% |
E1+E2 |
84 (82-87)% |
26 (21-32) % |
0.03 (0.01-0.10)% |
0.000 (0.00-0.00)% |
E1-3Glu |
93 (86-100)% |
54 (30-97)% |
2.4 (0.1-85)% |
0.20 (0.00-76)% |
E1-3Sul |
NA |
NA |
NA |
NA |
EE2 #1 |
100 (98-100)% |
98 (98-99)% |
91 (87-95)% |
85 (79-91)% |
EE2 #2 |
100 (98-100)% |
98 (98-99)% |
91 (88-95)% |
86 (81-91)% |
EE2 #3 |
100 (98-100)% |
99 (98-99)% |
92 (89-95)% |
87 (82-91)% |
The results in table 5-9 suggest that even when the sludge concentration is low and the transport time is short, appreciable losses of E1 and E2 could occur. If the sludge concentrations were as high as the
regulatory limits for STP effluents in Denmark or the EU, the samples would be spoiled if the transport time to the laboratory was 1 day. Therefore, samples from STPs should always be preserved before
they are transported to the laboratory.
5.6 Batch reactor characterisation
In order to know how well the batch experiments simulate the conditions in sewage treatment plants several parameters that characterise the reactors conditions were measured during the experiment. These
parameters were the oxygen concentration, pH, nitrate concentration and BOD1. The full details are given in Appendix 2 and only the main findings will be summarized here.
The reactors were kept at pH of 6.5 - 8.5, which is within tolerable limits for normal function of activated sludge bacteria. The denitrifying reactors had to have added acid regularly because the denitrification
consumes protons.
The aerobic reactors had an oxygen level about 7.25 mg O2/L which corresponds to ca. 80 % oxygen saturation. Therefore, the aerobic bacteria were well supplied with oxygen as in the aerobic tanks of
real STPs the typical concentration is 1-2 mg O2/L. In the denitrifying reactors the oxygen level was less than 0.1 mg O2/L. At this level no bacteria will function aerobically. In the denitrifying reactors nitrate
was consumed faster than 20 mg N·L-1·d-1. This together with the measured low oxygen concentration and the observed removal of protons (increased pH) provides evidence that the denitrifying reactors
were actually denitrifying.
The consumption of oxygen over 24 hours was similar between samples taken from the reactors on the three days of the experiment. However, some difference was observed between the denitrifying and
aerobic reactors. This indicates that the bacteria population or densities were not changing during the experiment. Thus, all parameters determined for the reactors indicate the reactors were operated in a
way that satisfactorily simulates the activated sludge tanks of a typical STP.
| Front page | | Contents | | Previous | | Next | | Top |
Version 1.0 November 2004, © Danish Environmental Protection Agency
|