| Bottom | | Front page |
Pesticides Research no. 91, 2004
Biological Control of Weevils (Strophosoma melanogrammum and S.
capitatum) in Greenery Plantations in Denmark
Contents
Preface
Dansk sammendrag
Summary
1 Introduction
2 Virulence of hyphomycete fungi against Strophosoma melanogrammum and S.capitatum
3 Implementation of molecular methods for identification of Strophosoma spp. larvae
4 Biology of Strophosoma melanogrammum and S. capitatum
5 Effects of hyphomycete fungi against Strophosoma spp. in greenery plantations
6 Effects of fungi on non-target invertebrates and spatio-temporal distribution
7 Discussion and Conclusions
8 Perspectives
9 References
Preface
The present report is based on results from the two projects: ”Biological control of weevils in greenery production in Denmark” (Journal no. 7041-0317) and ”Optimisation of biological control of
Strophosoma spp.” (Journal no. 7041-0081). The Royal Veterinary and Agricultural University, Department of Ecology, conducted both projects with financial support from the Danish Environmental
Protection Agency. The former project was carried out from April 2001 to December 2002 and the latter project from November 2002 to December 2003. Selected results obtained in the EU-project
“Biocontrol of Important Soil Dwelling Pests by Improving the Efficacy of Insect Pathogenic Fungi” (BIPESCO, EU FAIR6 CT-98-4105) are included in this report because of a high level of synergy
between the projects.
The authors wish to thank Odsherred State Forest District, The Danish Forest and Nature Agency, for allowing us to perform field experiments in ”Storskoven”, Bidstrup, Zealand and especially the forest
managers Stefan Bech Skov and Hans Christian Jessen for their great hospitality and interest in our work.
Many colleagues from the Department of Ecology supported and encouraged us throughout the work. First of all we would like to thank Jan Martin, Chris Lomer (†) and Holger Philipsen for contributing to
a fruitful and inspiring working atmosphere, and for their ongoing enthusiasm. Christina Wolsted, Rasmus Eliasen, Charlotte Fischer, Karen Marie Kjeldsen and Tina Ziska are all thanked for their skilled
technical assistance.
We thank Peter Lüth, Stephan Brückner and Sebastian Kiewnick, all from Prophyta in Germany, for providing fungal material for the field experiments. We are also grateful to Erik Christiansen, Norwegian
Forest Research Institute and Åke Lindelöw, Swedish University of Agricultural Sciences for providing Strophosoma specimens for molecular analyses.
Furthermore, the authors wish to acknowledge the members of the steering committee for their support and collaboration, especially Jørn Kirkegaard (Chairman), The Danish Environmental Protection
Agency.
Finally, partners from the EU-project BIPESCO are all thanked for collaboration on the projects.
Frederiksberg, June 21, 2004
Dansk sammendrag
Gråsnuder (Strophosoma melanogrammum og S. capitatum) forårsager skade på klippegrønt i danske bevoksninger af nobilis (Abies procera), som er en højværdiafgrøde. I Danmark er der et politisk
ønske om at erstatte kemisk bekæmpelse med miljøvenlig bekæmpelse, herunder biologisk bekæmpelse.
Projektet præsenteret i denne rapport havde til formål at evaluere potentialet for biologisk bekæmpelse af S. melanogrammum og S. capitatum ved hjælp af insektpatogene svampe. Følgende elementer
indgik: 1) bio-assays til udvælgelse af virulente insektpatogene svampe, 2) molekylær karakterisering af Strophosoma spp., 3) feltstudier af S. melanogrammum og S. capitatum fænologi, 4) feltforsøg
med henblik på måling af effektiviteten af et udvalgt isolat af svampen Metarhizium anisopliae på Strophosoma spp., 5) måling af effekter på non-target og fordeling af det udbragte isolat over tid og i rum.
I bio-assays blev svampene M. anisopliae (5 isolater), Beauveria bassiana (7 isolater), Paecilomyces farinosus (3 isolater) og Verticillium lecanii (2 isolater) testet overfor gråsnuder. Alle isolater af B.
bassiana og V. lecanii samt to af isolaterne af P. farinosus var isoleret fra naturligt inficerede voksne Strophosoma spp. biller. To metoder blev anvendt, dypning af voksne i en konidieopløsning samt
overførsel af larver til jord, hvorpå der var iblandet svamp. Alle testede svampe var i stand til at inficere Strophosoma spp. Den gennemsnitlige overlevelsestid for voksne var 16 til 20 dage ved 15°C,
afhængigt af isolat. For larver var den gennemsnitlige overlevelsestid mellem 20 og 30 dage (ved 15°C) ved behandling med B. bassiana og M. anisopliae.
Molekylære metoder blev taget i anvendelse og videreudviklet som hjælp til identifikation af de to Strophosoma arter i larvestadiet. Dele af cytochrom oxidase subunits I and II i mDNA blev amplificeret
samt sekventeret. Indenfor hver art af Strophosoma var der konsensus og specifikke primere blev designet til både S. melanogrammum og S. capitatum. En kombination af tre primere gav det bedste
resultat mht. identifikation af Strophosoma larver. Metoden kan, efter modifikation, tages i anvendelse til lignende studier indenfor snudebillefamilien (Curculionidae).
Projektet dokumenterede, at de voksne biller af både S. melanogrammum og S. capitatum ernærer sig på nålene i bevoksningen i foråret og efteråret. Æggene lægges i trækronerne om foråret, og efter
klækning søger larverne mod jorden for at gennemføre deres udvikling. Voksne biller spredes ved at kravle i bevoksningen, idet de kan tilbagelægge flere meter pr uge. Larvespredning med vinden synes
ikke at være af betydning. Den totale udviklingstid for S. melanogrammum og S. capitatum er som hovedregel 15 til 17 måneder. Udviklingstiden fra æg til voksen synes dog for S. capitatum at være
mere variabel. Den generation, der startes om foråret, vil derfor primært fremkomme som voksne om efteråret det følgende år.
En konidieopløsning af M. anisopliae (isolat BIPESCO 5) blev udsprøjtet på jorden med det formål at bekæmpe larver af Strophosoma spp. i sommeren 2000 og 2001, og det viste sig muligt at reducere
populationen af gråsnuder i de behandlede plots signifikant. Effekten var imidlertid først til stede efteråret det følgende år pga. larvernes lange udviklingstid i jorden. Der klækkede 77 voksne S.
melanogrammum pr. m² i behandlede plots i forhold til 102 pr. m² i ubehandlede plots i foråret 2002. For S. capitatum var tallene henholdsvis 46 pr. m² i behandlede plots i forhold til 69 pr. m² i de
ubehandlede plots. Et andet forsøg initieret i foråret 2001 viste at det er muligt at starte infektioner i voksne biller af Strophosoma ved udbringning af en konidieopløsning af M. anisopliae. Efter udbringning
af svampen nåede prevalenser af svampeinfektioner op på over 80 %. En signifikant effekt på gråsnudernes populationsniveau blev dokumenteret i det efterfølgende år.
Non-target effekter af M. anisopliae blev undersøgt ved at indsamle insekter og mider (især flåter) i de behandlede plots. Resultaterne er et udtryk for det økologiske værtspektrum af M. anisopliae (som
ikke kunne dokumenteres naturligt forekommende i bevoksningen). Syv dage efter behandling blev der fundet prevalenser af M. anisopliae på over 50 % blandt indsamlede tæger (Hemiptera), biller
(Coleoptera) samt flåter. I de ubehandlede plots var der ingen infektion blandt non-targets. Op til 277 dage efter behandling kunne der konstateres infektioner blandt non-targets. På de behandlede arealer
kunne M. anisopliae dokumenteres 418 dage efter udbringning ved isolering fra jorden.
Ny viden blev opnået på flere områder med hensyn til billernes fænologi, og det blev påvist, at molekylære metoder kan være værdifulde redskaber til artsidentifikation. Det lykkedes at udvælge og teste
virulente isolater af svampe i laboratoriet, og det kunne påvises, at M. anisopliae kunne inficere Strophosoma spp. i felten med en reduktion af populationen af disse det efterfølgende år. Der blev påvist
non-target effekter, men deres reelle effekt på populationsniveau kendes ikke. Metodesættene, der blev anvendt i projektet, kan efter modifikation anvendes til andre systemer. Det konkluderes, at det er
muligt at udvikle biologisk bekæmpelse overfor S. melanogrammum og S. capitatum.
Der vil være mange faktorer, der afgør, om det er muligt at udvikle kommercielt tilgængelig biologisk bekæmpelse, trods de mange resultater fra projektet. Vi vil især pege på nedenstående punkter: For det
første kræves undersøgelser i flere bevoksningstyper samt lokaliteter til fuld forståelse af gråsnudernes fænologi. For det andet bør en egentlig monitering tilvejebringes, således at skadestatus vurderes
korrekt. For det tredje foreslås yderligere effektivitetsstudier af svampe under feltforhold. For det fjerde vil vi pege på, at langtidseffekter på non-targets bør undersøges nøjere. Endelig skal de økonomiske
aspekter klarlægges udfra flere vinkler: producenter af klippegrønt, producenter og distributører af biologiske bekæmpelsesmidler og endelig den samfundsmæssige vinkel. I den sidste ende bør det være den
potentielle samfundsmæssige nytte, der afgør, hvorvidt biologisk bekæmpelse i dette system bør udvikles hen imod praktisk brug.
Summary
Weevils from the genus Strophosoma cause damage in Danish greenery plantations. The crop, noble fir (Abies procera), is used for decoration purposes and has a high economic value. Further, it is the
political intention in Denmark to replace chemical control by environmentally sustainable control, including biological control.
The project aimed to evaluate the potential for biological control of Strophosoma melanogrammum and S. capitatum by using insect pathogenic fungi. The following elements were included: 1) bio-assays
to select virulent insect pathogenic fungi, 2) molecular characterization of Strophosoma spp., 3) field studies on Strophosoma spp. phenology, 4) field experiments to assess the efficacy of a selected isolate
of the fungus Metarhizium anisopliae, 5) non target effects and spatio-temporal distribution of the applied M. anisopliae.
For bio-assays, isolates of M. anisopliae (5), Beauveria bassiana (7), Paecilomyces farinosus (3) and Verticillium lecanii (2) were tested. All isolates of B. bassiana and V. lecanii as well as two of the
P. farinosus originated from naturally infected adults of Strophosoma spp. The bio-assay methodology included both dipping adults into a conidial suspension of a fungus, and mixing conidial suspensions
into soil, onto which larvae were added. All tested fungi were able to cause mycosis in Strophosoma spp. For adults, the average survival time ranged between 16 and 20 days at 15°C, depending on
isolate. For larvae, the median lethal time ranged between 20 and 30 days at 15°C for B. bassiana and M. anisopliae.
Molecular methods were implemented to assist in the identification of Strophosoma spp. larvae. It was possible to amplify and get sequences of part of cytochrome oxidase subunits I and II in mDNA.
Consensus was found within each species of Strophosoma and specific primers were designed for both S. melanogrammum and S. capitatum. A combination of three primers gave the best results for the
identification of Strophosoma larvae. The method can, with further modification, be used for similar studies within the family Curculionidae.
The study documented that adults of both S. melanogrammum and S. capitatum feed on the greenery stand in spring and autumn. Eggs are laid in the canopy during spring and after hatching the larvae
seek to the soil to complete their development. Adult weevils disperse by walking within the stands at distances of several meters per week, while wind dispersal of larvae is apparently of no importance. For
both S. melanogrammum and S. capitatum, the development time is in general 15-17 months, although S. capitatum may have a greater flexibility in its development time from egg to adult. The generation
initiated in the spring thus emerges in the autumn the following year.
M. anisopliae (isolate BIPESCO 5) was applied against larvae of Strophosoma spp. in summer 2000 and 2001, and it was possible to reduce the target population significantly in the treated plots. The
effect was, however, not seen until autumn the following year, due to the long development time of the larvae in the soil: In treated plots, 77 S. melanogrammum per m² emerged versus 102 per m² in
untreated plots in spring 2002. For S. capitatum the data were 46 per m² versus 69 per m². In 2001 infections of M. anisopliae were initiated in adult of both Strophosoma species with prevalences
reaching more than 80%. Again, the effect on population level was, however, not registered until autumn the year following the application of M. anisopliae.
The non-target effects of M. anisopliae were studied by sampling insects and mites in the treated plots. The results therefore reflect the ecological host range of M. anisopliae (which did not occur in the soil
in the plots before treatment). Seven days after treatment, the two sampled insect orders (Hemiptera and Coleoptera) and ticks were found with prevalences of M. anisopliae above 50%, compared to no
infection in the untreated controls. Infections in non-targets were found as long as 277 days after treatment. However, the effect on population level of non-target is still unexplored. The persistence of the
fungus was also reflected in the fact that conidia of M. anisopliae were documented 418 days after application, by plating a soil suspension onto agar from the treated soil.
Overall, the project brought new insight on the phenology of the weevils. Also it documented that molecular methods can assist in the species identification of larvae. The project was successful to select and
test virulent fungal isolates in the laboratory and to show that M. anisopliae was able to infect Strophomosa spp. in the field with a reduction in population size the following year. Non-target effects were
observed in the field but were evaluated the effects on the population level are unknown. The project implemented and further developed a range of methods applicable for the tested system and also for
other systems. It is concluded that it is possible to develop biological control of S. melanogrammum and S. capitatum.
A development towards commercial usage depends, however, on many factors. Despite the new insight obtained in this study, we stress the following points: Firstly, the phenology of the target insects must
be fully understood by studying this in different types of stand and geographical regions. Secondly, the pest status of S. melanogrammum and S. capitatum must be assessed by more monitoring. Thirdly,
more extensive field trials must be performed to obtain more data on efficacy. Fourthly, non-target effects should be more studied especially with respect to long-term effects. Finally, the economic aspects of
biological control must be evaluated from several points of view: the grower, the producer and distributor of a biocontrol agent, and the society. In the bottom line, the societal benefits should be the main
factor to determine development towards practical use.
1 Introduction
1.1 Background
1.2 Project objectives
1.2.1 Specific project objectives
1.1 Background
Insects in forestry have predominantly been controlled by means of chemical insecticides. The extensive use of chemical insecticides has, however, caused problems for the environment, the major concerns
relating to side effects on non-target organisms and percolation into the ground water. This has led to an increasing interest and need for more environmentally friendly alternatives such as biological control.
In Danish forestry the economically most important insect problems and consequently the most intensive use of chemical insecticides occur in the production of Christmas trees and decoration green
(Kirkeby-Thomsen and Ravn, 1997; Ravn, 2000). Abies nordmanniana (Spach.) and Abies procera Rehder are the dominant tree species in this production today. No total estimate of the cultivated areas
exists, but for A. procera the area is at least 9.300 ha, while for A. nordmanniana it is at least 21.500 ha (Christensen, pers. com.). The greenery has a high export value, exceeding 165 mio USD per year
(Mainz, 2003). The market demands a very high product quality, and no damages from feeding of insect pests are accepted.
In the Danish greenery production, weevils (Coleoptera: Curculionidae) are frequently occurring insect pests. In particular, two species from the genus Strophosoma, the nut leaf weevil S. melanogrammum
Förster and S. capitatum Degeer, are economically important pests (Harding, 1993; Kirkeby-Thomsen and Ravn, 1997; Thorbek and Ravn, 1999; Ravn, 2000). The damage is caused by the adult weevils
feeding on the needles (Fig. 1.1). The weevils feed on current-year needles as well as elder needles. Although weevil damages are observed in the whole canopy, the damages are most pronounced in the
top of the crown, where also the needles of the leader are frequently heavily grazed upon. The damage may result in economically significant losses for the growers.
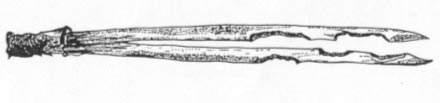
Figure 1.1: Damage on needles caused by Strophosoma spp. (from Sedlag and Kulicke, 1979).
Both Strophosoma species are polyphagous and inhabiting woodland. The adults have been reported to feed on important forest trees such as pine, spruce, larch, douglas fir, true firs, beech, birch, mountain
ash and oak (Grimm, 1973; Szmidt and Stakowiak, 1980; Ehnström, 1985; Palm, 1996; Urban, 1999), but also on hazel and herbaceous understory vegetation (Wagner and Schaefer, 1987; Parry et al.,
1990). Needles, leaves and buds are the preferred food, but in case of shortage of foliage, the weevils also feed on bark of young shoots.
In European forestry, Strophosoma spp. are generally regarded as insect pests in newly established cultures of conifers and deciduous trees as eg. beech and oak. In Denmark, however, in recent years
weevil damages in stands of noble fir and nordmann fir appear to have become an increasing problem in the production of decoration green (Harding, 1993; Harding et al., 1998; Harding and Ravn, 1999;
Thorbek and Ravn, 1999). S. melanogrammum seems to be the most common species in the Danish greenery plantations (Thorbek, 1998), whereas S. capitatum is considered of major importance in
most other European countries.
S. melanogrammum and S. capitatum occur together in the stands. The adults are morphologically very similar, brown and covered by greyish hairs and scales, but are easily separated in the adult stage by
the presence of a naked black stripe (“melanogram”) on the elytra in S. melanogrammum (Fig. 1.2). Populations of S. melanogrammum consist solely of parthenogentic females, whereas both males and
females are known for S. capitatum (Sedlag and Kulicke, 1979; Palm, 1996)
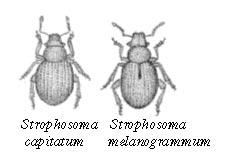
Figure 1.2: Drawings of the economically most important pest weevils in the Danish greenery production: Strophosoma melanogrammum and S. capitatum (from Sedlag and Kulicke, 1979)
In both species, the adults have two periods of feeding activity: One during spring prior to oviposition and one during late summer-autumn (Grimm, 1973; Schauermann, 1973; Sedlag and Kulicke, 1979;
Palm, 1996; Thorbek and Ravn, 1999; Urban, 1999; Harding et al., 2002). The larvae develop in the soil, where they are believed to graze on roots (Palm, 1996). However, only little is known about the
biology of the larvae. Curculionid larvae are difficult to identify and within the genus Strophosoma, species identification is not possible (Hansen, 1964; Scherf, 1964). Therefore, great uncertainty exists
about the development time of the two Strophosoma species, although this information is crucial for expectations to efficacy of control of the weevil populations. Reports range from 3-5 months (Schindler,
1974; Palm, 1996; Urban, 1999; Thorbek and Ravn, 1999) to 12-17 months (Schauermann, 1973; Sedlag and Kulicke, 1979) from oviposition to emergence of the offspring.
Control of insect pests in the production of greenery was earlier based on the use of chemical pesticides. However, from 2003 no chemical pest control is allowed in state forestry (The Danish Environmental
Protection Agency, 1998). For privately owned forests there is a political wish from the state authorities to phase out chemical pesticides (Ravn, 2000). Biological control using natural enemies of the pest
populations may thus provide an alternative to conventional chemical treatment.
Only few records exist concerning natural enemies of Strophosoma spp. Parasitation of the adult beetles by braconid wasps and parasitic flies have been reported as well as predation by ground beetles and
spiders (Schindler, 1964; Grimm, 1973), and eggs parasitized by a so far unidentified braconid have occasionally been found (Harding et al., 2002). Yet, parasitation levels appear to be very low and of no
major importance to the population levels.
Until recently, no documentation of naturally occurring entomopathogenic fungi has been recorded. Lately, however, the entomopathogenic fungi Beauveria bassiana (Bals.) Vuill., Paecilomyces farinosus
(Holm ex. C.F. Gray) Smith & Gray and Verticillium lecanii (Zimmermann) Viégas have been recorded to regularly kill adult weevils (Vestergaard et al., 2000; Eilenberg et al., 2003). No records exist
concerning natural enemies of larvae, but it is believed that entomopathogens may play an important role in the regulation of the larvae, since they are known to be an important mortality factor for many other
soil-dwelling larvae including Curculionidae (Rath, 1992; Marchal, 1977; Verkleij et al, 1992; Zimmermann, 1993; Keller et al., 1999, 2000; Vestergaard et al., 2000). Microbial control by
entomopathogenic fungi may therefore be a possibility to minimise the damage caused by S. melanogrammum and S. capitatum.
1.2 Project objectives
The aim of the project was to clarify the potential of insect pathogenic fungi for microbial control of Strophosoma spp.
Emphasis was on the potential of using fungi belonging to the class Hyphomycetes. A number of biological parameters of the weevils and the fungi have been studied to assess the feasibility of a variety of
possible control strategies.
1.2.1 Specific project objectives
The specific objectives were as follows:
- To investigate the virulence of hyphomycete fungi against S. melanogrammum and S. capitatum (chapter 2).
- To develop a molecular method for identification of S. melanogrammum and S. capitatum in the larval stage (chapter 3).
- To elucidate the biology and life cycle of S. melanogrammum and S. capitatum (chapter 4).
- To investigate the effect of hyphomycete fungi against S. melanogrammum and S. capitatum under field conditions (chapter 5).
- To investigate non-target effects of application of hyphomycete fungi in forest stands (chapter 6).
- To discuss the perspectives for the future use of hyphomycete fungi for control of weevils in greenery plantations (chapter 7-8).
2 Virulence of hyphomycete fungi against Strophosoma melanogrammum and S.capitatum
2.1 Definitions and terms used in laboratory bioassays with entomopathogenic fungi
2.2 Bioassay methodology
2.3 Analysis of bioassay data
2.3.1 Concentration response bioassays (LC50)
2.3.2 Correlated observations over time at one concentration
2.4 Bioassays with adult Strophosoma melanogrammum
2.4.1 Determination of average survival time at different temperatures
2.4.2 Determination of lethal concentration of M. anisopliae BIPESCO 5
2.5 Bioassays with Strophosoma spp. larvae
2.5.1 Determination of lethal time
2.6 Discussion
2.7 Conclusions
The first step toward utilisation of insect pathogenic fungi as a biological control agent is to select biologically and ecologically fit pathogens. In order to compare the efficacy of pathogens, assays are normally
carried out under laboratory conditions to maintain the maximum control over variability that might affect the results of the test. Comparative studies must be conducted under standardised conditions, which
permit normal or close to normal behaviour and avoid abnormally high mortality among the untreated insects (Lacey, 1997b). In this chapter emphasis is given to bioassay methodology and data obtained in
the projects.
2.1 Definitions and terms used in laboratory bioassays with entomopathogenic fungi
Pathogenicity of an insect pathogen is defined as the ability to produce disease in insects (Lacey, 1997a). The proof of pathogenicity is the first step towards studies on virulence. The virulence of an insect
pathogen is defined as the quality or property of being virulent or the disease-producing power of a micro-organism (Lacey, 1997a). Assessment of the virulence of an insect pathogen requires quantitative
studies.
In studies of time-response relationships the terms average survival time (AST) and median lethal time (LT50) are the most common expressions of the time required to kill a given insect. LT50 is defined as
the time period required to kill 50 % of the test insect population when subjected to a given concentration or dose of an insect pathogen. AST is the average lifetime of the population of interest. The terms
are often used as a quantitative expression of the virulence of fungi. The shorter the lethal time or survival time is for the test insect population subjected to a fungal isolate, the higher the virulence.
Furthermore, the term LT50 or AST provides much of the information needed to understand the dispersal of the disease in the insect population and the dynamics of the host-pathogen system (Goettel and
Inglis, 1997).
In studies of dose-response relationships, the terms LC50 and LD50 are the most common expressions of virulence. LC50 is the concentration of a given insect pathogen required to kill 50 % of the test
insect population within a given period of time, whereas LD50 expresses the dose required to kill 50% of the population. With respect to hyphomycete fungi, LC50 is the appropriate term since the
methodology only admits of an estimate of the concentration used and not of the dose actually received by the test insects (Goettel and Inglis, 1997).
2.2 Bioassay methodology
Standardised bioassay systems are not available for entomopathogenic Hyphomycetes. This is mostly due to the wide range of hosts, which vary in their requirements. Therefore, specific bioassays must be
developed for most host-pathogen combinations. However, the most common quantitative bioassay method for insects feeding above the soil surface is the `dipping method' (Goettel and Inglis, 1997,
Eilenberg et al., 2003). One or a series of aqueous suspensions are prepared with a known concentration of conidia. Insects are immersed singly or in cohorts into the suspension. After a specified time the
suspension is quickly drained off by suction (Hall, 1976). Another possibility is to present inoculum via a secondary substrate. The most common method is to dip the substrate, usually the food source into
the suspension and then transfer the insects onto it. The insects will then pick up the inoculum by contact with the substrate as they feed or move on it (Goettel and Inglis, 1997). A study comparing the effect
of the two dipping methods described above showed that the median lethal time was prolonged when dipping the substrate rather than the insect (Eilenberg et al., 2003).
For soil dwelling insect larvae such as, for example, larvae of Strophosoma spp. bioassays are often performed by incorporation of the inoculum into the soil substrate (Goettel and Inglis, 1997).
2.3 Analysis of bioassay data
The most used response in bioassays is the categories “dead” or “alive” and the response is thus binary having only two possibilities.
2.3.1 Concentration response bioassays (LC50)
The only explanatory variable considered in concentration response bioassays is the concentration. The response of the test subject is assumed to be functionally related to the dose or concentration level so
that as the concentration or dose of the pathogen increases more test subjects respond by dying. The equation of a binary response with a single explanatory variable (concentration) is given by:
Pi = F (a+ bxi), where
Pi is the probability of response, xi is the ith concentration or a function of that dose (eg. logarithm of concentration), a is the intercept of the regression line, b is the slope of the regression line, and F is the
distribution function. The most used functions are either probit or logit functions (Robertson and Preisler, 1992). Data for concentration-response relationships (LC50) presented in this report was calculated
using probit as the distribution function.
2.3.2 Correlated observations over time at one concentration
Standard probit or logit analysis techniques are, however, not applicable to serial time mortality data because observations made on the same group of insects at different times are correlated (Robertson and
Preisler, 1992, Throne et al., 1995). Basically two statistical methods have been used for analysing bioassay data where test insects have been subjected to a pathogen followed by regular recording of
mortality of the same group of insects. At present mainly two methods are used for analysis of serial time mortality data. This is the Kaplan-Meier Survival Analysis to calculate the average survival time in a
given population and logistic regression to calculate the medium lethal time (Kessler and Nielsen, 2000). In this report the virulence of a given isolate is calculated and given as average survival time (AST).
2.4 Bioassays with adult Strophosoma melanogrammum
2.4.1 Determination of average survival time at different temperatures
2.4.1.1 Materials and methods
Test insect: Adults of S. melanogrammum collected from the field in spring 2001 were used as test insects. Before use, weevils were in quarantine for three weeks to ensure healthy test material.
Isolates: Fourteen isolates were tested in laboratory bioassays (Table 2.1) and included two V. lecanii isolates (KVL 98-6 and 98-8); seven B. bassiana isolates (KVL 98-20, 98-35, 99-117, 00-125,
00-126, 00-127, 00-128) and three P. farinosus isolates (KVL 99-28, 00-88, 00-124) which all originated from naturally infected adult Curculionidae collected in Denmark. Furthermore, two M.
anisopliae isolates (BIPESCO 5, KVL 00-31) were selected based on earlier records of successful control of other insect pests (Vestergaard et al., 1995; 2002). The two M. anisopliae isolates originated
from a naturally infected Cydia pomonella L. collected in Austria by Dr. Gisbert Zimmermann and from Danish soil, respectively.
Table 2.1. Insect host and geographical origin of isolates of entomopathogenic fungal isolates tested in laboratory bioassay.
Species and accessions no. |
Original host |
Locality |
Year |
Verticillium lecanii |
|
|
|
KVL 98-6 |
Strophosoma sp. |
Torbenfeld, DK |
1998 |
KVL 98-8 |
Strophosoma sp. |
Grib Skov, DK |
1998 |
Beauveria bassiana |
|
|
|
KVL 98-20 |
Strophosoma sp. |
Torbenfeld, DK |
1998 |
KVL 98-35 |
S. melanogrammum |
Vesterlyng, DK |
1998 |
KVL 99-117 |
S. melanogrammum |
Vallø, DK |
1999 |
KVL 00-125 |
S. melanogrammum |
Gisselfeld, DK |
2000 |
KVL 00-126 |
S. capitatum |
Gisselfeld, DK |
2000 |
KVL 00-127 |
Strophosoma sp. |
Gisselfeld, DK |
2000 |
KVL 00-128 |
Strophosoma sp. |
Gisselfeld, DK |
2000 |
Paecilomyces farinosus |
|
|
|
KVL 99-28 |
S. melanogrammum |
Vesterlyng, DK |
1998 |
KVL 00-88 |
O. singularis |
Bidstrup, DK |
2000 |
KVL 00-124 |
S. melanogrammum |
Gisselfeld, DK |
2000 |
Metarhizium anisopliae |
|
|
|
BIPESCO 5 * |
C. pomonella |
Austria |
1967 |
KVL 00-31 |
Tenebrio (bait) |
Gisselfeld, DK |
1999 |
* BIPESCO 5 is a descendant of isolate 275-86 from Horticulture Research International culture collection.
Bioassay procedure: In initial bioassays infectivity and virulence of all fourteen isolates were tested against S. melanogrammum at 20°C. The conidia were harvested from cultures grown on 1.5%
Sabouraud dextrose agar (SDA) plates by flooding the plates with sterile 0.05% aqueous (w/v) Triton X-100 and gently agitated with a glass rod. Conidia were separated from agar and hyphae by
centrifugation for 5 min at 3000 rpm. The pellet was washed twice with sterile 0.05% Triton X-100 with intervening centrifugation steps. The conidial concentration was determined using a haemocytometer
and adjusted to 107 conidia/ml with 0.05% Triton X-100. The viability of the conidia was assessed on 1.5% SDA plates after 24 hours of incubation at 23°C by counting the number of germinating conidia
out of 300. The germination rate ranged from 94 to 100%.
Ten weevils were inoculated by immersion for 10 sec in 10 ml conidial suspension. The suspension and the weevils were poured onto filter paper in a Buchner funnel and excess suspension was removed by
vacuum. Insects were carefully transferred individually to medicine cups (30 ml) containing 5 ml of 3% water agar and a small twig of A. procera. The medicine cups were sealed with polyethylene (PE) cling
film, a semi-permeable membrane that ensures humid conditions without condensation. Finally, cups were closed with a plastic ring, and incubated at 20°C with a 16:8 h photoperiod. Controls consisted of
weevils treated with 0.05% Triton X-100 only. Three times 10 weevils were used per treatment and mortality was recorded every day for five weeks. During the incubation period medicine cups and twigs
were renewed whenever they appeared dry. After the initial bioassays two B. bassiana (KVL 98-20, 00-125), two P. farinosus (KVL 00-88, 00-124) and one M. anisopliae (BIPESCO 5) isolate were
selected for further testing at lower temperature (12°C and 15°C) against S. melanogrammum and against females of S. capitatum at 20C. Finally, B. bassiana (KVL 00-125) and M. anisopliae
(BIPESCO 5) were tested against males of S. capitatum.
Data-analysis: The LIFETEST procedure in SAS vers. 8.2 (SAS Institute, 1999) was used to compute nonparametric estimates of the survivor function by the product-limit method. Pair-wise comparisons
for differences in average survival time (AST) between populations subjected to different treatments or between Strophosoma species were tested for significance using a log-rank chi-square test.
2.4.1.2 Results
In none of the bioassays performed control mortality exceeded 10% and the test methods used are therefore regarded as reliable. All tested isolates were able to infect the target it was tested against, and
average survival time (AST) for all the tested isolates is given in table 2.2. Among the tested isolates a huge variation was found with AST for S. melanogrammum ranging from 13.1 ± 0.7 to 22.6 ± 0.6 days
when weevils were incubated at 20°C. Neither original host insect of the isolate nor fungal species did influence the AST of the weevils.
Three B. bassiana isolates (KVL 98-20; KVL 00-125 and KVL 00-126) and one M. anisopliae isolate (BIPESCO 5) caused AST below 15 days which were significantly lower than all other isolates
tested against S. melanogrammum at 20°C (P-values from log-rank chi-square test all ≤0.0181) but not mutually different (P-values from log-rank ? chi-square test all ≥ 0.5027). At lower
temperatures the AST were prolonged but all tested isolates were able to infect and cause mycosis. For all isolates tested against both S. melanogrammum and S. capitatum AST were significantly longer for
S. capitatum (P-values from log-rank chi-square test all < 0.0001).
Table 2.2: Average survival time (AST) ± S.E. for field collected adults of Strophosoma melanogrammum and S. capitatum after inoculation with hyphomycete fungi. After subjection to the fungi S. melanogrammum was incubated at 20, 15 and 12°C and S. capitatum incubated at 20°C . –: Isolate not tested.
Species and accessions no. |
AST at 20°C for S. melanogrammum |
AST at 15°C for S. melanogrammum |
AST at 12°C for S. melanogrammum |
AST at 20°C for S. capitatum ? |
AST at 20°C for S. capitatum ? |
Verticillium lecanii |
|
|
|
|
|
KVL 98-6 |
22.6 ± 0.6 |
- |
- |
- |
- |
KVL 98-8 |
17.9 ± 0.8 |
- |
- |
- |
- |
Beauveria bassiana |
|
|
|
|
|
KVL 98-20 |
13.1 ± 0.7 |
15.6 ± 0.5 |
19.0 ± 0.9 |
24.7 ± 1.5 |
- |
KVL 98-35 |
17.3 ± 0.7 |
- |
- |
- |
- |
KVL 99-117 |
15.6 ± 0.6 |
16.9 ± 0.7 |
- |
- |
- |
KVL 00-125 |
13.3 ± 0.3 |
16.3 ± 0.7 |
20.5 ± 0.9 |
25.4 ± 1.0 |
22.7 ± 1.3 |
KVL 00-126 |
13.7 ± 0.7 |
- |
- |
- |
- |
KVL 00-127 |
19.3 ± 0.7 |
- |
- |
- |
- |
KVL 00-128 |
18.8 ± 0.6 |
- |
- |
- |
- |
Paecilomyces farinosus |
|
|
|
|
|
KVL 99-28 |
18.5 ± 0.7 |
- |
- |
- |
- |
KVL 00-88 |
17.1 ± 0.6 |
19.5 ± 0.9 |
21.6 ± 1.0 |
28.0 ± 1.5 |
- |
KVL 00-124 |
- |
19.0 ± 1.0 |
22.0 ± 0.8 |
26.0 ± 1.3 |
- |
Metarhizium anisopliae |
|
|
|
|
- |
BIPESCO 5 |
13.5 ± 0.6 |
16.2 ± 1.0 |
19.8 ± 1.2 |
21.8 ± 1.3 |
23.4 ± 1.3 |
KVL 00-31 |
18.6 ± 0.7 |
- |
- |
- |
- |
2.4.2 Determination of lethal concentration of M. anisopliae BIPESCO 5
On the basis of the results of the bioassays described above as well as the ability to sporulate under large scale conditions on rice (C. Lomer, pers. com) one isolate, M. anisopliae BIPESCO 5, was chosen
for further test in a concentration-response bioassay as well as field experiments (Chapter 5).
2.4.2.1 Materials and methods
The bioassay procedure was the same as described above. Concentrations were adjusted to following concentrations: 1x105, 1x106, 1x107, 1x108 and 1x109 conidia/ml. Test insects were collected from the field in spring 2000.
Data analysis: The computer program Polo-PC (LeOra Software, 1987) was used to compute the concentration-response relationships (LC50) and probit was used as the distribution function.
2.4.2.2 Results
Results of concentration-response bioassay with BIPESCO 5 against spring-collected adults of S. melanogrammum are shown in Fig 2.1. The LC50 value was estimated to 2.1x107 conidia per ml (95 %
confidence limit: 0.8 – 4.5x107) after 21 days at 20°C; 16:8 L:D.
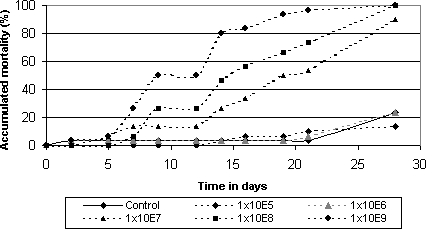
Figure 2.1: Concentration-response bioassay with Metarhizium anisopliae (Isolate no. BIPESCO 5) against adults of Strophosoma melanogrammum collected in spring.
2.5 Bioassays with Strophosoma spp. larvae
2.5.1 Determination of lethal time
2.5.1.1 Material and methods
Test insect: Larvae (3. and 4. instar) of Strophosoma spp. collected from the soil in a Noble fir plantation at `Storskoven' Bidstrup, Denmark, during the summer of 2001 were used as test insects.
Fungal material: Two isolates of B. bassiana (KVL 98-20, Boverol®); one P. farinosus (KVL 99-28) and one M. anisopliae (BIPESCO 5) isolates were tested against Strophosoma spp. larvae.
Conidial suspensions were prepared as described in paragraph 2.4.1.
Bioassay procedure: For each isolate tested 3 ml of a conidia suspension adjusted to 108 conidia/ml or 3 ml Triton-X (control) were mixed into 30 g of field collected soil corresponding to the
concentration obtained in the field experiments just after spraying (see chapter 5). The soil was transferred to 30 ml cups and ten larvae were introduced into each cup. All isolates were tested in constant
darkness at 15°C. Three times 10 larvae were used per treatment. Mortality was recorded weekly for six weeks.
2.5.1.2 Results
No larvae died in the control treatment and all tested isolates were able to infect and cause mycosis in larvae of Strophosoma spp. The results are shown in fig. 2.2. Among the tested isolates the most
virulent isolate was M. anisopliae BIPESCO 5, which resulted in 80% mortality and mycosis. The average survival time ranged between 20 and 30 days at 15°C for B. bassiana and M. anisopliae. The
average survival time for P. farinosus (KVL 99-28) was not calculated since the mortality never exceeded 18%.
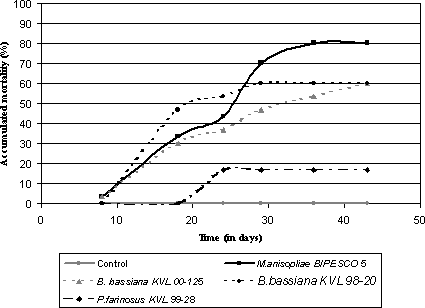
Figure 2.2: Accumulated mortality of larvae of Strophosoma spp. after subjection to entomopathogenic fungi.
2.6 Discussion
The bioassay showed that under laboratory conditions all of the tested isolates were able to infect and cause mycosis in adults of S. melanogrammum and S. capitatum even at low temperature comparable
to the temperatures found in activity periods of the adult weevils in Denmark (average mean temperature in May 11°C, June 15°C, August 16°C, September 13°C, October 10°C (DMI, 2004).
Average survival times for adults ranged between 16 and 20 days at 15°C depending on isolate. The survival time seen for S. melanogrammum and S. capitatum subjected to conidial suspensions of
entomopathogenic fungi are in the same range as reported for many other adults in the family Curculionidae. For example, Chikwenhere and Vestergaard (2001) reported median lethal times between 9 and
15 days for Neochetina bruchi Hustache when subjected to B. bassiana and incubated at 25°C and Kaaya et al. (1993) reported median lethal times from 8 days and up to more than 35 days for
Cosmopolites sordidus (Germar) when subjected to B. bassiana or M. anisopliae.
Compared to survival times usually seen for weevils treated with chemical pesticides, the survival time is much longer and no immediate effect would be expected of treatment against adult Strophosoma spp.
Nevertheless, due to an initial feeding period up to several weeks in fall and again in spring before the eggs are laid and the long period of time over which eggs are laid (see chapter 4) it is believed that a
fungal treatment in either spring or fall will have a potential to prevent population increase in the next generation. Whether adults emerging from the soil in spring or in autumn have different susceptibility to
entomopathogenic fungi so far remains unexplored.
As concerns the Strophosoma larvae, it was possible to infect them in the laboratory. Larvae were, however, not identified to species level since it is not possible to identify them in a non-destructive way
(see chapter 3), so whether both species can be infected by entomopathogens cannot be determined for sure. However, due to the following facts we believe that both species are susceptible: (1) 30 larvae
were treated for each isolate, (2) The percentage of fungal infection ranged between 60 and 80 % for most isolates tested (3) at the time of the year when larvae were collected the ratio between the two
Strophosoma species were the following year found to be approximately fifty-fifty (see chapter 4).
Other reports from studies on lethal time of coleopteran larvae have shown much difference, mostly shorter than ours. The following studies did, however, all refer to dipping of larvae in a conidial
suspension, a highly `quicker' method than ours, which more closely reflects the situation in the field upon treatment. Kershaw et al. (1999) reported LT50 values between 6 and 12 days for the curculionid
Otiorhynchus sulcatus F. treated with M. anisopliae. Kassa et al . (2002) reported a median survival time between 3.6 and 6.3 days for the curculionid Sitophilus zeamais Motschulsky subjected to B.
bassiana. White grubs in Mexico (Phyllophaga spp.) subjected to M. anisopliae and B. bassiana had a LT50 of 4.9 days and 6.1 days, respectively (Flores et al., 2002). Long average survival times
were, however, reported by Keller et al. (1999) for Melolontha melolontha L. treated with Beauveria brongniartii (Saccardo) Petch: between 22.9 and 56.4 days. Two different populations showed
different susceptibility.
2.7 Conclusions
- It was possible to implement and further develop a technique to perform bioassays with both adults and larvae of Strophosoma melanogrammum and S. capitatum. In none of the bioassays performed control mortality ever exceeded 10% and the test methods used are thus reliable.
- Beauveria bassiana, Paecilomyces farinosus and Metarhizium anisopliae were able to infect and cause mycosis in both adults and larvae of S. melanogrammum and S. capitatum.
- Average survival times (AST) for adults ranged between 16 and 20 days at 15°C depending on isolate. For larvae the AST's ranged between 20 and 30 days at 15°C for B. bassiana and M. anisopliae.
- Average survival time was highly depending on isolate and temperature.
- Results obtained in this project gave higher average survival times for Strophosoma spp. larvae than most reported data on coleopteran larvae subjected to fungi, but the method used in the present project is more closely related to the field situation than most reported results in the literature.
- Insect pathogenic fungi are, based on laboratory study, regarded as highly potential candidates for biological control of both adults and larvae of Strophosoma spp. even at lower temperatures.
3 Implementation of molecular methods for identification of Strophosoma spp. larvae
3.1 Introduction
3.1.1 The molecular methods
3.1.2 Analysis of molecular sequence data
3.2 Materials and methods
3.2.1 Extraction and amplification of DNA
3.2.2 Search in Genbank and related bases with BLAST
3.2.3 Alignments BLAST, T-Coffee and ClustalW
3.2.4 Design of Primers for the cytochrome oxidase subunits I and II
3.3 Results
3.3.1 DNA extraction from weevils
3.3.2 Weevil DNA sequences and PCR amplification
3.3.3 Search in Genbank and related bases with BLAST
3.3.4 Alignments BLAST, T-Coffee and ClustalW
3.4 Discussion
3.5 Conclusion
3.1 Introduction
Adults of the two Strophosoma species, S. capitatum and S. melanogrammum, can easily be identified based on morphologically characters alone (chapter 1). In contrast, morphological characters are
insufficient to distinguish between these species in the larval stages (Hansen, 1964; Scherf, 1964). Both larvae of S. capitatum and of S. melanogrammum are whitish, legless with chestnut-brown
sclerotised heads and for both species, the cuticle is covered by small hairs (Fig.3.1).
Detailed knowledge on life cycle, phenology and larval development is needed for a precise targetting of control measures against S. capitatum and S. melanogrammum, - knowledge which is not available
(chapter 1). In order to obtain this knowledge it is, however, a prerequisite that the two species can be distinguished during the entire life cycle.
The specific objective for this part of the project therefore was to implement molecular methods for identification of Strophosoma spp. in the larval stage leading to a detailed description of the life cycles of
each of the Strophosoma species in question.
This chapter describes briefly the background for the relevant molecular methods used and results obtained.
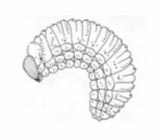
Figure 3.1: Drawing of a typical curculionid larva (Hansen, 1964).
3.1.1 The molecular methods
The identification and characterisation of insects using DNA based techniques has during the last twenty years become increasingly important. The most widely used method is polymerase chain reaction
(PCR). This method has been used for studying evolutionary ecology, species identification, population genetics, systematics and diversity.
The overall principle in any PCR reaction is as follows: First DNA is isolated, then a mixture of DNA, primer(s), Taq polymerase, dNTP, MgCl2 and buffer is mixed in reaction tubes and placed in a
thermocycler. Several cycles of (1) denaturation (93-95°C); (2) annealing (35-60°C) and (3) extension (72°C) are then repeated. After 30-40 cycles an enormous number of copies of the target DNA
have been amplified and can be monitored by electrophoresis in an agarose gel. Primers can be designed to amplify either specific or random regions of the DNA. In specific priming, two different primers
are designed so that they flank a specified target region of the DNA, one primer on each strand. The specified region between the two primers of the DNA is then amplified. Random priming involves
amplification of random segments of the entire genomic DNA. In contrast to specific priming only one primer is normally used to amplify the DNA, and it is usually shorter than the primers used for specific
priming. Because of the short length of the primer and less stringent conditions that allow mismatching, it anneals to several places of the genomic DNA. A DNA amplification product is thus generated for
each genomic region that happens to be flanked by the priming sites. Both specific and random priming molecular methods are, after some development, presumed to be able to identify the larvae of the two
Strophosoma species in question and a short introduction to the methods used and evaluated in this study is given.
3.1.1.1 Amplification of specific regions
Amplification of the ribosomal DNA (rDNA) or the mitochondrial DNA (mDNA) genes has become the most commonly used regions for specific PCR priming (Sheppard et al., 1994; Simon et al., 1994;
Cano et al., 2001; Schulenburg et al. 2001). Mitochondrial DNA genes evolve approximately 16 times faster than the nuclear rDNA and will therefore often be useful for relatively closely related species.
Amplification products of specific regions can be followed by either sequencing or restriction fragment length polymorphism (RFLP). In RFLP-PCR the amplified DNA is incubated with restriction enzymes
that bind to and cut the DNA at specific sites within or adjacent to a particular sequence known as the recognition sequence (Sambrook et al., 1989).
DNA sequences from the mitochondrial DNA genome are used with increasing frequency to estimate phylogenetic relationships among animal taxa (Simon et al., 1994). The cytochrome oxidase subunits I
and II (COI + COII) have been sequenced from several insect orders including Coleopterans (Sheppard et al., 1994; Simon et al., 1994). The COI and COII subunits can be isolated via PCR from almost
any taxon, using conserved primers.
3.1.1.2 Amplification of unspecific regions
Random amplified polymorphic DNA (RAPD) was developed in 1990 (Williams et al., 1990). It is a method for fingerprinting DNA. RAPD-PCR analysis involves amplification of random segments of
entire genomic DNA. Usually, short (10 base) oligonucleotide primers are used to amplify the DNA. A DNA amplification product is generated for each genomic region that happens to be flanked by a pair
of 10 base priming sites. One big advantage is that DNA from any organism can be amplified without any prior knowledge of its sequences. Unsatisfactory reproducibility, however, is one of the
disadvantages. Universally primed PCR (UP-PCR) is a variant of the RAPD method. UP-PCR is related to RAPD in being capable of amplifying DNA from any organism without previous knowledge of its
DNA sequences. The advantage of this technique is higher reproducibility due to PCR primer features and use of high annealing temperatures (Lübeck et al., 1998). These methods are capable of
distinguishing species and can therefore also be applied in different population studies of ecological relevance. There are several studies using RAPD-PCR within Curculionidae (Bas et al., 2000; Lewis et
al., 2001, Madeira et al., 2001). Furthermore, RAPD has for example been applied to studies concerning plant pathogenic fungus on grape (Peros and Berger, 2003), ectomycorrhizal fungi (Huai et al.,
2003), insect pathogenic fungi (Nielsen et al., 2001) and insect pathogenic bacteria (Rivera and Priest, 2003). There are, however, no reported studies on the genus Strophosoma.
3.1.2 Analysis of molecular sequence data
BLAST (Basic Local Alignment Search Tool) is a set of similarity search programs designed to explore all the available sequence databases regardless of whether the query is protein or DNA. The BLAST
programs have been designed for speed, with a minimal sacrifice of sensitivity to distant sequence relationships. The scores assigned in a BLAST search have a well-defined statistical interpretation, making
real matches easier to distinguish from random background hits. BLAST uses a heuristic algorithm that seeks local as opposed to global alignments and is therefore able to detect relationships among
sequences that share only isolated regions of similarity (Anonym, 2003).
BLAST was used to analyse the similarity in weevil DNA sequences obtained in this project and to compare these sequences with other sequences from weevils and other insects published in Nucleotide
Sequence Databases (GenBank, RefSeq Nucleotides, EMBL (European Molecular Biology Laboratory), DDBJ (DNA Data Bank of Japan).
For global multiple alignments (the entire sequences are aligned) the programs T-Coffee and CrustalW were used (Notredame et al., 2000; Gibas and Jambeck, 2001). These programmes are often used
for phylogenetic reconstruction or for illustrating conserved and variable sites within a family or the sequences analysed.
In the BLAST search and in the multiple alignments, references are given to sequence identity, which are the occurrence of exactly the same nucleic acid in the same position in two or more aligned
sequences. An E-value is calculated and indicates the number of alignments one expects to find with a score greater than or equal to the observed alignments score in a search against a random database.
This means that an e-value close to 0 indicates great similarity to the analysed sequence (Gibas and Jambeck, 2001).
3.2 Materials and methods
For development and selection of specific primer sets it was decided to:
- Compare alignments sequences of COI + COII in mDNA for S. melanogrammum and S. capitatum.
- Find consensus and variable DNA sequences between the two species and compare these sequences to DNA sequences reported in Genbank for other Curculionidae.
For selection of unspecific primers it was chosen to:
- Compare fragment pattern from UP-PCR and RAPD-PCR for S. melanogrammum and S. capitatum.
Both the amplification of specific and unspecific regions was evaluated for consistency by including S. melanogrammum and S. capitatum from several Danish localities as well as specimens from UK,
Norway and Sweden. In addition, O. singularis from Denmark was included as an outgroup.
3.2.1 Extraction and amplification of DNA
3.2.1.1 Weevil materials
DNA was isolated from adults of S. capitatum (DK, UK, SE, NO) and S. melanogrammum (DK, UK, SE,). Furthermore, Danish specimens of O. singularis were included as outliers. The weevils used
in this study were either collected alive in the field or stored specimens (air-dried or stored in 70% ethanol). In order to evaluate the methods developed, DNA was also isolated from Danish larvae of S.
capitatum, S. melanogrammum and O. singularis either stored in 70% ethanol, at –20°C or freeze-dried.
3.2.1.2 Extraction of DNA
Total genomic DNA was extracted by 1) the use of the Nucleon PhytoPure Kit (Amersham Biosciences Europe GmbH), 2) a method based on the protocol of Bulat et al. (1998) or 3) Chelating resin
(Chelex 100) (Sigma-Aldrich Co. St. Louis, USA) following the method described by Cano et al. (1993).
3.2.1.3 COI/COII amplifications and product analysis
PCR amplification was carried out for the weevil material using the primers C1-J-2797 (C1) and C2-N-3380 (C2) for amplification of part of the COI/COII subunit (Table 3.1).
Amplifications were performed in 0.2 ml PCR tubes containing a total volume of 25 µl in a solution containing 1 µl of template DNA, 250 µM of each dNTP, 0.8 µM of each primer, 1 unit Taq polymerase (Boehringer Mannheim, Germany), 2.5 µl PCR reaction buffer (100 mM Tris-HCl, 15 mM MgCl2, 500 mM KCl, pH 8.3) and 0.5 µl MgCl2 (25mM). A negative control was included in each experiment and prepared similarly but without DNA. DNA amplification was executed in a Perkin Elmer Gene Amp PCR system 9600 thermal cycler with the following PCR conditions for the COI/COII subunit: one cycle at 2 min at 94°C (denaturation), 1 min at 42°C (annealing), and 1 min at 72°C (extension) followed by 35 cycles at 1 min at 94°C, 1 min at 42°C, and 1 min at 72°C with a final cycle of 1 min at 94°C, 1 min at 42°C, and 5 min at 72°C.
PCR products (10 µl) were loaded with 1 x loading buffer (10 µl; 50 % sucrose, 0.1 % bromophenol blue, 100 mM Tris, pH 8.0) and resolved on 1.5 % 20 cm long agarose gel added ethidium bromide in 1 x TBE buffer (50 mM Tris, 50 mM Boric Acid, 1 mM EDTA) at 120 Volt for approx. 2 ½ hours. A DNA ladder (0.1-1.5 kbp, BioLabs Inc.) was loaded on each gel in order to determine the sizes of the PCR amplification products (Sambrook et al. 1989).
The PCR products obtained were in addition used for sequencing after purification with GFX PCR DNA and gel band purification kit (Amersham Biosciences, Europe GmbH). PCR products were sequenced on an ABI PRISM Genetic Analyzer (DNA Technology A/S, Denmark).
3.2.1.4 RAPD-PCR amplifications and product analysis
RAPD-PCR amplifications were performed in 0.2 ml PCR tubes containing a total volume of 25 µl in a solution containing 1 µl of template DNA, 100 µM of each of the dNTPs, 0.9 µM primer, 0.5 units of Taq polymerase (Boehringer Mannheim, Germany), 2.5 µl reaction buffer (100 mM Tris-HCl, 15 mM MgCl2, 500 mM KCl, pH 8.3 (20°C)) and 0.5 l (25 mM MgCl2). PCR reactions were executed in the same PCR machine as above but using 40 cycles with the following temperature profile: 30 s at 94°C, 1 min at 36°C, and 2 min at 72°C. A negative control was included in each experiment. Several DNA concentrations were tested in PCR in order to ensure the reliability of electrophoretic banding profiles. Three out of 15 primers gave bands, which could be used to distinguish between the two Strophosoma species. The primers (OPA-03, OPA-13, and OPA-20) were each of ten nucleotides and produced by Operon Technologies Ltd (Table 3.1). RAPD-PCR products (10 µl) were processed in the same way as described in paragraph 3.2.1.3.
3.2.1.5 UP-PCR amplifications and product analysis
UP-PCR amplifications were performed in 0.2 ml PCR tubes containing a total volume of 20 µl in a solution containing 1 µl template DNA, 100 µM of each dNTPs, about 0.5 µM primer, 1 unit of Taq polymerase (Finzym, Finland), in 1.8 µl of the reaction buffer and 2 µl MgCl2 (25 mM). Conditions for DNA amplification was 35 cycles of 94°C for 3 min, 55°C for 70 s and 72°C for 1 min, and 28 cycles of 50 s at 92°C, 70 s at 55°C, and 1 min at 72°C. A final cycle was performed of 50 s at 92°C, 70 s at 55°C and 3 min at 72°C. A negative control was included in each experiment. UP-PCR products (10 l) were processed in the same way as described in paragraph 3.2.1.3. Six universal primers were used: 3,2, AS15, AS4, L15-AS19, L21, L45 and FOK1 (DNA Technology A/S, Denmark) (Table 3.1).
Table 3.1 List of primers used in this study.
Primer name |
Sequence (5’?- 3') |
Reference |
C1 |
CCT CGA CGT TAT TCA GAT TAC C |
Erney et al., 1996 |
C2 |
TCA ATA TCA TTG ATG ACC AAT |
Erney et al., 1996 |
OPA-3 OPA-13 |
AGT CAG CCA C CAG CAC CCA C |
Operon Technologies Operon Technologies |
OPA-20 |
GTT GCG ATC C |
Operon Technologies |
3,2 |
TAA GGG CGG TGC CAG T |
Lübeck et al., 1998 |
L15-AS19 |
GAG GGT GGC GGC TAG |
Lübeck et al., 1998 |
L21 |
GGA TCC GAG GGT GGC GGT TCT |
Lübeck et al., 1998 |
L45 |
GTA AAA CGA CGG CCA GT |
Lübeck et al., 1998 |
AS4 |
TGT GGG CGC TCG ACA C |
Lübeck et al., 1998 |
AS15 |
GGC TAA GCG GTC GTT AC |
Lübeck et al., 1998 |
FOK1 |
GGA TGA CCC ACC TCC TAC |
Lübeck et al., 1998 |
3.2.2 Search in Genbank and related bases with BLAST
At the web site http://www.ncbi.nlm.nih.gov/BLAST/ the “Standard nucleotide-nucleotide BLAST [blastn]” or “MEGABLAST” search programme was chosen to compare the known weevil sequences
against sequences in Genbank.
3.2.3 Alignments BLAST, T-Coffee and ClustalW
Pairwise BLAST (http://www.ncbi.nlm.nih.gov/blast/bl2seq/bl2.html) was used to compare the similarity between two known weevil sequences. The programme `blastn' was used and the parameter for a match was 1 and –2 for a mismatch. Multiple sequence alignments were done for the weevil sequences of good quality. The program T-Coffee (http://www.ch.embnet.-org/software/TCoffee.html) (Notredame et al., 2000) and the program ClustalW (http://clustalw.genome.ad.jp/sit-bin/nph-clustalw) were used.
3.2.4 Design of Primers for the cytochrome oxidase subunits I and II
After alignments of the weevil COI and COII sequences, consensus and variable DNA sequences were found. One primer from the consensus Strophosoma DNA sequences was designed by using the
program Primer 3 (http://www-genome.wi.mit.edu/cgi-bin/primer/-primer3_www.cgi) (Rozen and Skaletsky, 2000). Then different reverse primers were designed from the regions varying between S.
melanogrammum and S. capitatum. Furthermore, primers were designed so none of the primers should be able to align to DNA sequence of O. singularis. Because of high AT-content in the weevil DNA
sequences the Tm temperature was lowered in the PCR reactions with these primers compared to normal standard conditions.
3.3 Results
3.3.1 DNA extraction from weevils
DNA was isolated from adults, small larvae and large larvae of Strophosoma. However, depending of the previous storage history as well as the DNA extraction method used different qualities of DNA
were obtained (Table 3.2). For specimens caught alive all three methods gave high quality DNA, while for larvae stored in ethanol best quality of DNA were obtained with the Chelex-100 method (Table
3.2). Subsequently this method was the predominant method used for isolation of DNA from larvae.
Table 3.2 DNA qualities obtained by three different extraction methods.
|
Adult beetles |
Large larvae |
Small larvae |
DNA extraction method |
Alive |
Freeze-dried |
Ethanol (70%) |
Air-dried |
Freeze-dried |
-20°C |
Ethanol (70%) |
Freeze-dried |
Ethanol (70%) |
1) Nucleon PhytoPure kit |
good |
Good |
medium - good |
none-good |
good |
medium –good |
good |
poor -good |
poor - medium |
2) Bulat et al., 1998 |
good |
|
medium - god |
medium - good |
poor |
none |
|
|
medium |
3) Chelex 100 |
good |
|
none -medium |
none |
|
good |
good |
medium |
good |
3.3.2 Weevil DNA sequences and PCR amplification
3.3.2.1 COI/COII regions PCR products
Weevil DNA sequences from partly sequences of the COI + COII subunits were obtained by PCR. With primers C1 and C2 one band was obtained at approx. 590 bp (Fig.3.2). However, not all DNA
samples produced a PCR-product.
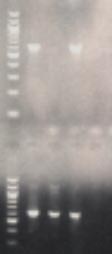
Figure 3.2. Five PCR products from the COI + COII subunits of S. melanogrammum and S. capitatum.
3.3.2.2 RAPD-PCR and UP-PCR products
The RAPD-PCR primers OPA 3, OPA 13 and OPA 20 were able to identify the two Strophosoma species and O. singularis, giving three different band profiles (figure 3.3). The same could be proven
with the UP-PCR primer L15/AS19 (figure 3.3).
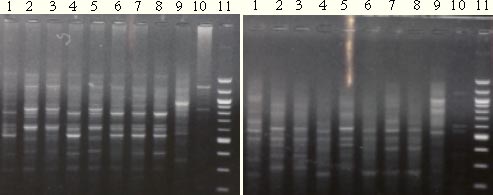
Figure 3.3. Left: RAPD-PCR products (primer OPA 20). Right: UP-PCR products (Primer L15/AS19). Same DNA have been used in both gels, lane 1, 4, 7 = S. melanogrammum, lane 2 and 5 = S. capitatum, Lane 3 and 6 = mix of the two Strophosoma species, lane 8 = unidentified larva, lane 9 = O. singularis, lane 10 without DNA and lane 11= 100bp ladder.
3.3.3 Search in Genbank and related bases with BLAST
3.3.3.1 COI and COII sequences
Similarity between sequences obtained from S. melanogrammum, S. capitatum and O. singularis to other Curculionidae was especially found from the last part of the sequence (approx. 320 – 590 bp) that is part of the COII gene. Identities at 87% for a 234 bp sequence with E-value at 4 x 10-68 were found as the best match to the weevil, Brachyderes rugatus Wollaston. A DNA sequence in Genbank was also found of COI for S. melanogrammum (from Sweden) with the accession number AY196875, but no similarity was found to the known sequences of Strophosoma species when aligned. In the Genbank an O. singularis (AY196874) sequenced COI subunits was found, but no similarity was found to the known O. singularis sequence from this project.
3.3.4 Alignments BLAST, T-Coffee and ClustalW
3.3.4.1 COI and COII sequences
Great similarities at 95 to 99% identity were found within each of the two Strophosoma species (Table 3.3), while between the two species only similarities at 86 to 90% occurred. Less similarity was found
when compared to O. singularis (78 – 80%) (Table 3.3).
Table 3.3. Similarities between sequences obtained from own Strophosoma spp. and Otiorhynchus singularis by Pairwise BLAST.
Primer used |
Weevil species and country of origin |
Identities (%) |
E-values |
Seq. length compared (bp) |
CO1 |
S.mel (DK) – S.cap (DK1) |
86 |
0 |
Total (587) |
S.mel (DK) – S.cap (DK2) |
86 |
0 |
563 |
S.mel (DK) – S.mel (UK) |
95 |
0 (2 gab) |
Total (585) |
S.cap (DK1) – S.cap (DK2) |
95 |
0 (1 gab and 1 bp) |
Total (580) |
S.cap (DK1/2) – S.mel (UK) |
86/88 |
0/0 |
507/507 |
S.mel (DK) – O.sing (DK) |
80 |
10-105 |
488 |
S.cap (DK1) – O.sing (DK) |
78 |
10-111/3x10-91 |
574 |
S.mel (UK) – O.sing (DK) |
80 |
10-115 |
530 |
3.3.4.2 Design of Primers
A primer from the consensus Strophosoma DNA sequences around 120 bp and different reverse primers from the end of the sequences with less similarity between the two species were designed (Table
3.4). The optimal PCR conditions for the new primers were found to be 35 cycles of 30 s at 94°C, 45 s at 55°C, and 45s at 72°C ending with one cycle of 30 s at 94°C, 30 s at 55°C, and 5 min at 72°C.
When using the primer combination (Start Stroph. + End-sc4+End-sm3), a PCR product of approx. 250 bp and 350 bp were produced for S. capitatum and S. melanogrammum, respectively (figure
3.4). When using the End-sc2 primer instead of End-sc4 the length of the PCR product for S. capitatum was approx. at 300 bp.
Table 3.4. Sequences of designed oligos primer
Primer name |
Primer sequence 5’- 3’ |
Species preference |
Start Stroph. |
AAT AGT GCA TTG GAC TTA AAC C |
Both species |
End-sm1 |
CCC TTC TAA TAG GTA TCG GTG AAT |
S. melanogrammum |
End-sc1 |
GGG GAG GGC AAT TAA GAT |
S. capitatum |
End-sm2 |
CCC TTC TAA TAG GTA TCG GTG AAT A |
S. melanogrammum |
End-sc2 |
GGG GAG GGC AAT TAA GAT A |
S. capitatum |
End-sm3 |
GGC TTT AAT TGA AAT TAT AGG A |
S. melanogrammum |
End-sc3 |
CCC CAT TGA TGA CAA ATA AC |
S. capitatum |
End-sc4 |
TTC AAT TAA TTG TCC TTC CAG |
S. capitatum |
Click here to see Figure 3.4
Figure 3.4. Agarose-gel with PCR-products from adult S. melanogrammum (first arrow) and S. capitatum (second arrow) plus unidentified Strophosoma spp. larvae. DNA from S. melanogrammum and S. capitatum gave a band at approx. 350 bp and 250 bp, respectively, using the primer combination Start Stroph. + End-sc4+End-sm3
Using the primer combination (Start Stroph. + End-sc2+End-sm3) or (Start Stroph. + End-sc4+End-sm3) it was possible to identify the Strophosoma larvae species. Selected Strophosoma sp. and O.
singularis DNA samples were also tested by RAPD-PCR or UP-PCR and gave results consistent with the results obtained by the primer combination Start Stroph. + End-sc2+End-sm3 and Start Stroph.
+ End-sc4+End-sm3.
3.4 Discussion
Successful extraction and high quality of DNA is a prerequisite to obtain molecular data. Preserved specimens offer a challenge, and in studies of for example plants, the quality of DNA from herbarium
specimens can often be poor (Drabkova et al., 2002). The possibility to include alcohol preserved specimens is important when considering soft and fragile larvae like Strophosoma larvae. We managed to
obtain DNA from both individuals sampled alive and from some specimens preserved in alcohol. In the latter case, however, the quality of the DNA was not always sufficiently good for analysis. In a study
on fungal pathogens in insects, Thomsen and Jensen (2002) included alcohol-preserved specimens and found that DNA could also be extracted in many cases.
PCR methods have been used recently to sort out taxonomic problems within Curculionidae. Roehrdanz (2001) studied variation in the species Anthonomus grandis Boheman and could confirm specific
geographical distributions of haplotypes. Peng et al. (2002) developed a tool to distinguish laboratory colonies of the closely related species Sitophilus oryzae (L.) and S. zeamais Motschulsky. A situation
very similar to ours was studied by Weathersbee et al. (2003) who studied indistinguishable egg masses on two pest curculionids in Florida and designed primers useful to assist in the assessment of the pest
status of each species.
Our method basically confirmed the phenotypic characters available to distinguish adult specimens and validated that S. melanogrammum and S. capitatum are two valid taxonomic units (species). We
were successful to separate larvae also by PCR giving for the first time a possibility to determine larvae of Strophosoma species, which have no clear phenotypic species characters. The phenological data
obtained in our field studies are therefore also validated giving much more evidence to these studies.
3.5 Conclusion
- It was possible to amplify and get sequences of part of cytochrome oxidase subunits I and II (COI + COII) in mDNA for the two related species of the weevil from the genus Strophosoma and of the species O. singularis.
- Consensus was found within each species of Strophosoma and specific primers were designed for both S. melanogrammum and S. capitatum.
- When the primers were tested experimentally the combination of the three primers (Start Stroph., End-sc2 and End-sm3 or Start Stroph., End-sc4 and End-sm3) gave the best results in identifying the Strophosoma spp. larvae.
- It can be concluded that it thus was possible to implement molecular methods for identification of Strophosoma spp.
- The method can, with further modification, be used for similar studies within the family Curculionidae.
4 Biology of Strophosoma melanogrammum and S. capitatum
4.1 Feeding periods of adult weevils
4.1.1 Materials and methods
4.1.2 Results
4.2 Larval drop from the canopy
4.2.1 Materials and methods
4.2.2 Results
4.3 Dispersal of Strophosoma adults and larvae
4.3.1 Materials and methods
4.3.2 Results
4.4 Development time of S. melanogrammum and S. capitatum
4.4.1 Materials and methods
4.4.2 Results
4.5 Discussion
4.5.1 Life cycle
4.6 Conclusions
Any control measure, chemical or biological, rests on detailed knowledge about the biology and ecology of the target insects. Occurrence, activity, feeding periods and life cycle of the pest will inevitably
affect the success of treatment, and therefore a description of these elements and of the factors influencing them is prerequisite in the development of control methods and strategies.
In spite of the incontrovertible status of S. melanogrammum and S. capitatum as insect pests in European forestry, knowledge of the biology of these weevil species is very sparse. Most investigations have
dealt with S. capitatum as this is the more important one in European forestry whereas S. melanogrammum, which appears to be of major interest in Denmark, is only little studied.
Knowledge is more or less confined to the feeding periods during which the damage on trees takes place. The activity studies all agree in showing that both Strophosoma species have two feeding periods
per year: One begins in early spring and lasts for a couple of months. This feeding precedes oviposition, which takes place after about three weeks of maturation feeding on the foliage (Urban, 1999). The
next feeding period occurs in late summer and autumn, when a new generation of adult beetles emerges from the soil (Grimm, 1973; Schauermann, 1973; Sedlag and Kulicke, 1979; Bejer, 1989; Palm,
1996; Thorbek and Ravn, 1999; Urban, 1999; Harding et al., 2002).
Until now, it has generally been believed, although never documented, that the eggs are laid in the soil (Kleine, 1911; Balachowski, 1963; Grimm, 1973; Schauermann, 1973; Sedlag and Kulicke, 1979;
Bejer, 1989; Palm 1996). Reissig (1928), however, mentioned wavy hair grass, Deschampsia flexuosa (L.) Trin. and Hylocomium mosses as oviposition sites and, based on laboratory observations,
Breese (1948) suggested that eggs also can be laid above ground in cracks of the bark and below budscales. In laboratory experiments, oviposition in soil has never been observed (Breese, 1948; Urban,
1999; Frølander and Nielsen, unpubl.). Recent investigations connected to this project have demonstrated that Strophosoma eggs are deposited in the canopy of A. procera and Picea abies (Karst.) below
budscales and at the base of the needles. The first instar larvae then drop to the ground for further development in the soil (Harding et al., 2002). Egg densities were highest in the top of the trees (Harding,
unpubl.). These observations, however, did not specify this oviposition behaviour for both Strophosoma species as neither eggs nor larvae can be identified to species level based on morphological
characters.
In the literature the development time, i.e. the period from the eggs are laid until the adults emerge, varies between 3 months and 1½ year. Schauermann (1973) reports a development of 12 – 15 months,
Sedlag and Kulicke (1979) 17 months; Schindler (1974), Urban (1999) and Thorbek and Ravn (1999) describe the period to last only 3-5 months. Palm (1996) reports that larvae of S. melanogrammum
are occasionally found in the soil during winter, indicating that this species may have the possibility of developing from eggs to adults in either 3-5 or 15-17 months. In forest zoology handbooks and other
extension service tools for management of forest pests, there is, however, a consensus of opinion that both Strophosoma species are univoltine, stating that the offspring of the beetles feeding and ovipositing
in spring emerge and feed in the autumn the same year. Hence, chemical control of the weevils in spring is in general anticipated to have a significant effect not only at the time of spraying, but also on the
population levels and, in consequence, on the damages in autumn.
In this project the investigations have focussed on the following elements of the biology of S. melanogrammum and S. capitatum as these are of main relevance to control strategies. Further, they will
altogether give the first description of the life cycle of each of the two weevil species:
- Feeding periods of adult weevils
- Larval drop from the canopy
- Dispersal of Strophosoma adults and larvae
- Development time of S. melanogrammum and S. capitatum
4.1 Feeding periods of adult weevils
4.1.1 Materials and methods
The activity of the adult weevils was recorded from spring 2001 until autumn 2003 in a greenery stand of A. procera established in 1983 in “Storskoven”, Bidstrup, Odsherred State Forest District. The
activity was investigated by 30 evenly distributed emergence traps recording adult beetles emerging from the soil to feed in the vegetation. Each emergence trap caught weevils from an area of 1/8 m² (Fig.
4.1). The traps were emptied weekly, and the weevils were counted and determined to species.
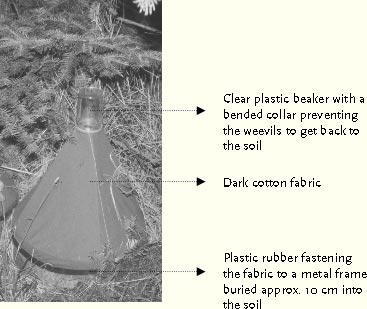
Figure. 4.1 Emergence trap for monitoring of adults of Strophosoma emerging from the soil.
4.1.2 Results
The monitoring of the emergence from the soil of S. melanogrammum and S. capitatum confirmed that both species have two feeding periods per year (Fig. 4.2).
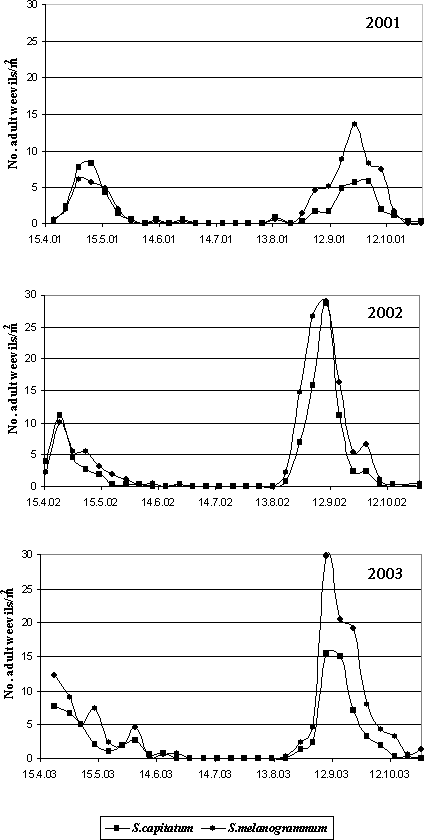
Figure 4.2. Emergence of Strophosoma melanogrammum and S. capitatum from the soil in 2001-2003 given as number of weevils per m² per week as a function of date traps were collected. Numbers were recorded by weekly counting of adult weevils in 30 emergence traps in a 20-year-old greenery stand of A. procera.
In spring, emergence for maturation feeding was initiated in early April and lasted for about 6-8 weeks. The emergence of the new generation was recorded from the beginning of August and proceeded for
another two months, peaking late August or in September. Minor differences in the pattern of emergence occurred between years: In spring as well as in autumn 2001, emergence occurred about two weeks
later than in 2002 and 2003. The two Strophosoma species were almost identical in their pattern of emergence, showing no major differences in the timing of initiation of emergence or of the peak of
emergence activity.
Population levels of S. melanogrammum were higher than of S. capitatum during all three years, except in spring 2001 (Table 4.1). Autumn densities were markedly higher than spring densities in both
species, with the exception of S. capitatum in 2001.
Table 4.1: Accumulated density (no. per m² ± SE) of Strophosoma melanogrammum and S. capitatum in the experimental greenery stand of noble fir in three successive years.
Year |
2001 |
2002 |
2003 |
|
Spring |
Autumn |
Spring |
Autumn |
Spring |
Autumn |
S. melanogrammum |
21.6±7.7 |
51.2±14.4 |
30.4±7.5 |
102.9±35.2 |
44.3±18.1 |
94.1±26.9 |
S. capitatum |
25.9±1.3 |
24.0±8.0 |
25.1±6.4 |
69.1±24.3 |
27.7±8.0 |
46.9±13.9 |
4.2 Larval drop from the canopy
4.2.1 Materials and methods
4.2.1.1 Larval behaviour
First instar larvae dropping to the ground from the oviposition site in the canopy were recorded by means of funnel traps placed below the tree crowns. The traps consisted of a plastic funnel with a diameter
of 21.5 cm, fitted into a 1 litre plastic bottle. The bottles were dug 2/3 into the soil to prevent it from falling over. 10 funnel traps were placed scattered in the A. procera stand (Fig. 4.3). The bottles were
filled with a 2 % solution of benzoic acid. The traps were emptied by changing the bottles, which were then brought to the laboratory, where the contents were filtered through a 125µm wire mesh and
preserved in 70% alcohol until Strophosoma larvae were sorted out and counted.
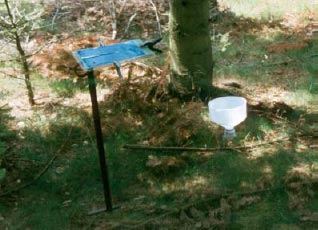
Figure 4.3. Funnel traps and sticky traps for recording of first instar larvae of Strophosoma dropping to the ground from the canopy.
The activity was recorded weekly during summer until early November. In 2001 recording was, however, not initiated until early July as the observation of oviposition in the canopy was not made before that
time. In 2002 recording started in early June, in 2003 in mid May.
The activity of the larvae dropping from the canopy was in 2001 and 2002 also recorded by means of sticky traps. By 10 trees, a 514 cm² blue sticky trap was placed horizontally at 1.5 m's height below
the crowns next to the funnel traps (Fig. 4.3). The traps were changed weekly. The sticky traps were wrapped in cling film and taken to the laboratory. Larvae adhering to the traps were counted under
microscope. Both recording methods were effective; as they, however, showed a similar pattern of activity, only data from funnel traps are presented here.
4.2.1.2 Species identification of larvae
In 2003, a fraction of the first instar larvae collected by funnel traps were identified to species by PCR analysis according to the method described in Chapter 3. At each date during the period June 17 – July
30, 10 larvae were randomly selected for analysis. However, at June 24, during the peak of activity, 46 larvae were analysed for identification. For the remaining period, bulks of 10 larvae or less were
analysed and only qualitative occurrence in the sample was recorded.
4.2.2 Results
4.2.2.1 Larval behaviour
First instar larvae of Strophosoma drop to the ground during summer and early autumn (Fig. 4.4). The activity has a very distinguished peak lasting 2-3 weeks and then abruptly declining to insignificant
levels. A few larvae still fall to the ground during the whole summer period, but this part of the larval population is of minor importance compared to the peak. The time of maximal activity varied only slightly
between years, in 2002 peaking around June 18 – 25, in 2003 June 17 – July 1. In 2001 the peak may not have been recorded, but high levels were recorded during the first two weeks of July.
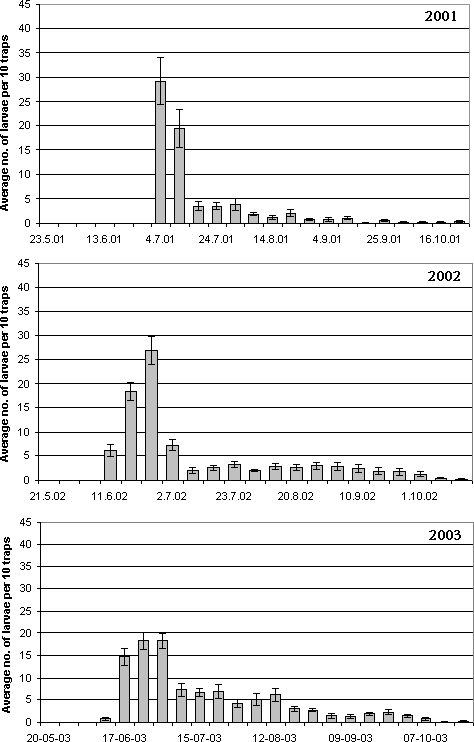
Figure 4.4. Activity of first instar Strophosoma larvae dropping from the canopy in a greenery stand of noble fir 2001-2003 . Activity is recorded as means of larvae trapped per funnel trap per week (± SE).
The density of Strophosoma larvae dropping through the vegetation to seek to the soil was very high, up to several thousand larvae per m² (Table 4.2). Local densities exceeded more than 4000 larvae per
m² (data not shown). The densities showed great variation between years, and showed an increase during the experimental period.
Table 4.2: Accumulated density (no. per m² ± SE) of first instar Strophosoma larvae dropping from the canopy in a greenery stand of noble fir. Densities were estimated from numbers of larvae recorded in funnel traps.
Year |
2001 |
2002 |
2003 |
No. of Strophosoma larvae ± SE |
1895.4±876.0 |
2418.9±469.5 |
2843.2±626.3 |
4.2.2.2 Species identification of larvae
PCR analysis showed that larvae of both Strophosoma species drop to the ground from the canopy. Also, both species occurred in the traps during the whole period (Table 4.3). Absence of S. capitatum
in samples in October may be due to a small number of specimens analysed (6 individuals).
Table 4.3. Occurrence of Strophosoma melanogrammum and S. capitatum in funnel traps recording larvae dropping to the ground from the foliage. Figures in parentheses indicate numbers of specimens identified.
Occurrence of Strophosoma species |
Date |
No. examined |
S. melanogrammum |
S. capitatum |
17-06-03 |
10 |
+ (6) |
+(1) |
24-06-03 |
46 |
+ (33) |
+(9) |
01-07-03 |
10 |
+ (7) |
+(1) |
08-07-03 |
10 |
+ (9) |
+(1) |
15-07-03 |
10 |
+ (8) |
+(1) |
22-07-03 |
10 |
+(9) |
- |
30-07-03 |
10 |
+(4) |
+(1) |
05-08-03 |
10 |
+(6) |
+(3) |
12-08-03 |
10 |
+ |
- |
19-08-03 |
10 |
+(5) |
+(2) |
26-08-03 |
10 |
+ |
+ |
02-09-03 |
7 |
+ |
+ |
09-09-03 |
6 |
+ |
- |
16-09-03 |
10 |
+ |
+ |
23-09-03 |
10 |
+ |
+ |
30-09-03 |
7 |
+ |
+ |
07-10-03 |
4 |
+ |
- |
21-10-03 |
2 |
+ |
- |
4.3 Dispersal of Strophosoma adults and larvae
4.3.1 Materials and methods
4.3.1.1 Dispersal of adults
482 adult S. melanogrammum collected in the field were marked with red nail polish in the laboratory and released on June 6, 2002, around a tree trunk of a 2½ meter high A. procera. On day 7 and 28
after the release of the weevils, the infested tree and surrounding trees were monitored for marked individuals by beating the branches over a white tray. Marked individuals captured were counted.
4.3.1.2 Dispersal of larvae
The possibility of dispersal of the weevil population by first instar larvae when they leave the canopy was investigated by two methods: a) sticky traps placed at crown level, and b) water traps below crown
level outside the stand.
Trapping by sticky traps was preliminarily tested in 2002: During the peak of larval activity, three 9-meter poles were raised within the greenery stand but without physical contact with the canopy. At 5, 6, 7,
and 8 m's height, respectively, a 514 cm² sticky trap was fastened vertically. After two weeks, the sticky traps were taken down and analysed for Strophosoma larvae as described in paragraph 4.2.1.1. In
2003, the 3 poles were raised at different distances from the stand trees: i) within the stand but without contact with the canopy, ii) within a glade in the stand, at least 5 m from the nearest trees, and iii) in an
open area ca. 10 m from stand edges of noble fir. Sticky traps were placed at an angle of 105° to the pole to minimise turbulence. One set of traps was fit up on June 17 and taken down after one week; a
second trapping period elapsed from July 1 to 15.
In 2003 7 water traps were placed on the ground along the centre of a wide track within the greenery stand. These traps were circular plastic containers (diameter 15 cm, depth 9 cm) with a 2% solution of
benzoic acid and detergent (soap). No traps were closer to the stand trees than 2 m. Another 3 traps were placed in the glade close to the pole with sticky traps. The total trapping area thus was ca. 0.35
m². Further, in the open area and close to the sticky trap pole, water traps were placed on a platform elevated 3 m above the vegetation: 8 circular containers (diameter 45 cm, depth 30 cm) each with a
trapping area of ca. 0.16 m² and 31 plastic containers with a trapping area of 0.035 m² each. The total trapping area on the platform thus was approx. 2.37 m². All water traps were put out on July 1 and
emptied twice at weekly intervals. The contents of the traps were poured into plastic cans and treated as described for funnel traps in 4.2.1.1.
4.3.2 Results
4.3.2.1 Dispersal of adults
After seven days, 23% (109) of the released individuals were recaptured. The majority was recaptured in the tree around which the weevils were released and mainly in the top of the tree; however, marked
individuals were recaptured up to 3 m away. After 28 days, 34 specimens (7%) were recaptured. The majority of weevils were again caught in the top of the tree of release, but individuals were recaptured
at a distance of 7.5 meters.
4.3.2.2 Dispersal of larvae
In 2002, only one larva was found on the sticky traps. In 2003, a total of 5 larvae were found on the sticky traps during the first period, of these 4 were found in the dense vegetation – three at 5 m and one
at 7 m, and only one larva adhered to the traps in the open area. During the second period, six larvae were captured by the sticky traps, again five within the dense stand and only one in the open area. None
of the water traps in the open area collected any dispersing larvae. However, in the track and glade within the stand, a total of 17 larvae were found in 2003 (nine on July 8, 2002 and eigth on July 15,
2002). The eigth larvae captured on July 15, 2002 were analysed by PCR and only S. melanogrammum was found.
4.4 Development time of S. melanogrammum and S. capitatum
4.4.1 Materials and methods
Strophosoma larvae were collected from the soil of the greenery stand at irregular intervals, during late autumn (November 26, 2002), spring (April 8, 2003) and early summer (May 27 and June 25, 2003).
In the field, the soil was carefully examined, and larvae and pupae were sorted out, transferred to vials with soil and taken to the laboratory, for further examination. A total of 79 larvae were collected. As
investigations had demonstrated that the width of the head capsule and body length were linearly correlated in Strophosoma (data not shown) as is known from other insect larvae (Kéler, 1952), the width
of the head capsule was used for classification of larval stage. The larvae were identified to species by PCR according the method described in Chapter 3. In addition, the soil was searched for the presence
of pupae in August 2002 and in April and May 2003.
4.4.2 Results
Pupae were only found in the soil in August. At all other sampling dates, only larvae were found. Of the 79 larvae collected, 69 were successfully identified by PCR. This analysis showed that larvae of both
Strophosoma species are present in the soil throughout the year (Fig. 4.5). In late autumn (November, 2002), medium sized larvae of S. melanogrammum were found with an average width of the head
capsule of 0.71 ± 0.12 mm. In spring (April 8, 2003), the majority of larvae were still in the same stage of development, but some had developed into the next stage. In May and June 2003, the S.
melanogrammum larvae had all entered their last stage of development (width of head capsule: 0.96±0.05 mm). For S. capitatum, larval sizes were very different in late autumn, ranging between 0.3 and
1.08 mm. In spring, only few larvae were found, but in early summer all larvae had developed into the last stage, having a head capsule size of 0.99±0.13 mm. There was thus a tendency that larvae of S.
capitatum were a little larger than larvae of S. melanogrammum, but differences were not statistically significant.
Click here to see Figure 4.5
Figure. 4.5. Size of larvae, measured as width of head capsule, of Strophosoma melanogrammum (left) and S. capitatum (right) collected at different times of the year from the soil of a greenery stand of Abies procera.
4.5 Discussion
The period of emergence observed in this investigation is consistent with previous investigations of S. capitatum (Sedlag and Kulicke, 1979) and S. melanogrammum (Thorbek, 1998; Frølander unpubl.).
The feeding period is, however, longer than the emergence period, as the adult weevils spend at least 3 weeks feeding prior to oviposition (Urban, 1999). Damages from adults feeding on the foliage thus
develop from mid April to early – mid June and again during autumn, August – early October. In warm autumns, extensive feeding may continue during October and even November (S. Harding, personal
observation) and also in early winter active weevils can be found in the canopy if temperatures are sufficiently high (Parry, 1983; Thorbek, 1998). The higher population levels in autumn than in spring were
also documented by Grimm (1973) in Germany and by Thorbek (1998) and Frølander (unpubl.) for S. melanogrammum in Denmark, but appear not to be a general pattern in Central Europe for either
species (Sedlag and Kulicke, 1979). This may indicate that the life cycle may vary within the area of distribution.
Although hypothesised from laboratory experiments that oviposition may not take place in the soil (Breese, 1948; Urban, 1999), the occurrence of the eggs and first instar larvae in the canopy of the
greenery plantation and the falling down of the larvae through the canopy and the ground vegetation have not been documented before. This new knowledge is of great importance to the development of a
control strategy as it makes it possible to target directly the microbial control against the small larvae when they drop to the ground. The application method must ensure that the larvae get in contact with the
conidia of the insect pathogenic fungus. This will be possible by a treatment of the soil surface timed immediately prior to the period when the larvae pass through. In this connection, it is noteworthy that the
peak of this activity is very narrow in as much as feeding and oviposition periods are very prolonged; this has the practical implication that optimal soil treatment can be timed to early June.
Knowledge about dispersal is relevant in relation to the ability of the weevils to spread a pathogen within the population. As the wings of Strophosoma spp. are fused, walking is the most likely strategy of
dispersal. The adults are capable of dispersing by walking up to several meters per week, but although marked individuals were recaptured 7.5 m from the point of release, dispersal over long distances is
hardly possible, which was also indicated by preliminary studies by Skov (2000). Horizontal movement of the larvae in the soil has been documented by Schauermann (1973) but is unlikely to amount to
more than a few meters. Wind dispersal over long distances by the small larvae dropping to the ground was near hand, as this strategy is well known in eg. Lymantriidae and Adelgidae, but our results do not
lend support to this hypothesis. From our studies of dispersal it must be concluded that the ability of S. capitatum and S. melanogrammum to disperse is rather restricted compared to most other pest
insects.
By sampling of larvae throughout the year, it was documented that an exclusively univoltine development as suggested by Schindler (1974), Urban (1999) and Thorbek and Ravn (1999) can be totally
rejected. Univoltinism would imply either absence of larvae during winter (3-5 months development) or the occurrence of last stage larvae and pupae in the spring (12 months development), and neither was
found to be the case. It was found that both S. melanogrammum and S. capitatum can have a development time of 15-17 months from the eggs are laid in spring until emergence of the new generation in
the autumn the following year. This sequence of development is in accordance with investigations undertaken in Germany by Schauermann (1973) who reports on the only previous detailed investigation of
the development of Strophosoma spp. He found a mixture of 3rd and 4th stage larvae in the soil from September until August the following year and, being unable to discriminate between the two species,
he suggested one species to hibernate in the 3rd and the other species in the 4th larval stage. This difference was not found in the present study in which larvae of both species developed from medium sized
(3rd stage) to large (4th stage) larvae during early summer.
The results do not enable us to definitely make out if part of the populations may be able to complete development in 3-5 months. More frequent sampling during summer and higher numbers of larvae are
needed to follow in detail the exact sequence of development (cf. e.g. Axelsson et al., 1973) and any variations within populations .
The practical consequence of a 2-year life cycle is that an immediate effect of control of larvae on the population levels in autumn cannot be expected.
4.5.1 Life cycle
Our studies enable us to describe the general life cycle of the two Strophosoma species in Danish greenery plantations: Adults of both species emerge from their hibernation site in the soil in late April and
seek to the canopy of the stand trees for maturation feeding prior to oviposition. Feeding lasts approx. 3 weeks and takes place in the treetops on the leader and upper whirls (Harding, unpubl.). The eggs,
which are laid in the canopy, hatch after about 3 weeks, and 6-8 weeks after the peak of emergence the 1st stage larvae drop to the ground to complete their development in the soil. The larvae complete
another 3 stages in the soil and overwinter primarily in the 3rd larval stage. The larvae enter their 4th stage in May. Pupation takes place in late July/August and the new generation emerges from late August.
It cannot be excluded that part of the populations can develop in 3-5 months.
Clear differences have not been found in the life cycles of the two Strophosoma species. However, S. capitatum may have a greater flexibility in its development as indicated by a high variation in the size of
the larvae at a given time. It is not known whether this life cycle is general for S. melanogrammum and S. capitatum in their whole area of distribution and at different stand characteristics.
4.6 Conclusions
- Strophosoma melanogrammum as well as Strophosoma capitatum feed on greenery stands in spring (April-June) and in autumn (August-October).
- Eggs are laid in the canopy during spring. After hatching the first instar larvae seek to the soil where they complete their development.
- Peak activity of larval dropping to the ground takes place second half of June-early July.
- Adult weevils may disperse by walking within the stands, at distances of several meters per week.
- Wind dispersal of first instar larvae is no important element in the dispersal of Strophosoma populations.
- The development time can be 15-17 months for both S. melanogrammum and S. capitatum. The generation initiated in spring emerges during autumn the following year. However, S. capitatum may have a greater flexibility in its development time from egg to adult.
5 Effects of hyphomycete fungi against Strophosoma spp. in greenery plantations
5.1 Soil application of Metarhizium anisopliae against larvae
5.1.1 Materials and methods
5.1.2 Results
5.2 Soil application of Metarhizium anisopliae against ovipositing females
5.2.1 Materials and methods
5.2.2 Results
5.3 Soil application of B. bassiana and of M. anisopliae against adults emerging from the soil in spring
5.3.1 Materials and methods
5.3.2 Results
5.4 Application of tree trunks with lanes of Beauveria bassiana
5.5 Discussion
5.5.1 Control of larvae
5.5.2 Control of adult weevils
5.5.3 Prospects for development of a microbial control strategy
5.6 Conclusions
Based on the results obtained in bioassays (chapter 2) and information on the life cycle of Strophosoma spp. (chapter 4), M. anisopliae and B. bassiana were chosen for test under field conditions in a
greenery plantation. Four experiments were carried out to investigate field effects of treatment of adults and of larvae in the soil and to study the possibility of single tree treatment targeted against adults
seeking to the treetops:
- Soil application of M. anisopliae (BIPESCO 5) against larvae in the soil by spraying in summer 2000 and 2001 (performed in co-operation with the EU financed project BIPESCO).
- Soil application of M. anisopliae (BIPESCO 5) against ovipositing females of Strophosoma spp. emerging from the soil by spraying in spring 2001.
- Soil application of B. bassiana (KVL 98-20, KVL 02-04 and KVL 02-14) and M. anisopliae (BIPESCO 5) against adults of Strophosoma spp. emerging from the soil by spraying in spring 2002.
- Application of tree trunks with lanes of B. bassiana (Boverol®) in autumn 2001 against adults of Strophosoma spp. seeking to the canopy to feed.
All experiments were performed in an A. procera plantation situated at ”Storskoven”, Bidstrup, Zealand, Denmark. The plantation was established in 1983 and has for the last years suffered from damages
caused by Strophosoma spp.
5.1 Soil application of Metarhizium anisopliae against larvae
5.1.1 Materials and methods
One part of the plantation was divided into four plots each covering approximately 125 m². Untreated border zones of 4-5 m separated the plots. Two plots were treated twice with M. anisopliae in 2000
and exactly the same plots were treated again twice in the following year. In both years two plots remained untreated and served as control plots.
M. anisopliae (BIPESCO 5) was applied to the two plots on July 7 and July 19, 2000 by spraying 200 ml per m² of a M. anisopliae suspension adjusted to 5.0 x 107 conidia per ml 0.05 % Triton X-100.
M. anisopliae (BIPESCO 5) was applied to the same plots on June 26 and July 10, 2001 at the same concentrations as in 2000. In both years the final concentration sprayed on each date was thus 1 x
1014 conidia per ha. The conidia of M. anisopliae were produced by Prophyta (Germany). Two control plots were treated with 0.05 % Triton X-100 only.
The effect of the treatment on the population levels of adult weevils was assessed by emergence traps placed on the ground. Adult weevils were sampled from 15 evenly distributed emergence traps in each
plot once a week from mid July 2000 until beginning of November 2003. Each emergence trap caught weevils from 1/8 m². All weevils were counted and determined to species level.
The effect of date, plot, and treatment on the number of weevils emerging from the traps was analysed by multiple regressions. The GLM procedure in SAS v. 8.02 (SAS Institute, 1999) was used for the
analysis, density of weevils were log transformed [loge (weevils per trap + 1)] and each species was analysed separately. Date, plot and treatments were used as class variables. The full model including all
parameters and possible interactions was reduced stepwise by excluding the least significant parameter for each step but always by excluding interactions before main factors (Jensen and Skovgaard, 1995).
5.1.2 Results
5.1.2.1 Population level in 2000
In autumn, the adult weevils emerged from the soil from the beginning of August until mid November with a maximum of 21 weevils per m² per week in mid September. In autumn 2000 the accumulated
number of S. melanogrammum and S. capitatum caught in emergence traps in the treated plots was not significantly different from the number caught in the control plots (data not shown).
5.1.2.2 Population level in 2001
The numbers of Strophosoma caught in spring 2001 are shown in figure 5.1. Adults emerged from the soil from the beginning of April until mid June, reaching a maximum of 12 S. melanogrammum and 5
S. capitatum per m² per week in the control plots in the beginning of May. In treated plots the maximum of S. melanogrammum was also reached in the beginning of May by 8 S. melanogrammum per
m² per week. S. capitatum reached its maximum two weeks later by 7 individuals per m² per week. The differences in numbers between weevils caught in emergence traps in the treated plots and control
plots were, however, only slightly significant for S. melanogrammum (F 1,648 = 3.94; P = 0.0476) and not significant for S. capitatum (F 1,647 = 2.49; P = 0.1151).
In autumn, the adult weevils emerged from the soil from mid August until the beginning of November, reaching a maximum of 25 S. melanogrammum and 7 S. capitatum per m² per week in the control
plots in the end of September. In treated plots the maximum of weevils was also reached in the end of September by 10 S. melanogrammum and 4 S. capitatum. The accumulated numbers of
Strophosoma spp. caught in autumn 2001 in emergence traps were 131 per m² in control plots and 63 per m² in treated plots (Fig. 5.2). For S. melanogrammum the accumulated density of weevils in M.
anisopliae treated plots was thus reduced by 60% compared to the density in the control plots. For S. capitatum the accumulated density of weevils in M. anisopliae treated plots was reduced by 38%
compared to the density in the control plots. The accumulated numbers of both S. melanogrammum and S. capitatum caught in emergence cages in the treated plots were significantly lower than the
numbers caught in the control plots (S. melanogrammum: F 1,588 = 41.31; P < 0.0001; S. capitatum: F 1,588 = 5.28; P = 0.0220).
5.1.2.3 Population level in 2002
In spring 2002, adults emerged from the soil from the beginning of April until mid June, reaching a maximum of 24 S. melanogrammum and 7 S. capitatum per m² per week in the control plots in mid
April. In treated plots the maximum of weevils was also reached in mid April by 18 S. melanogrammum and 9 S. capitatum. For S. melanogrammum the accumulated numbers of weevils in M.
anisopliae treated plots was reduced significantly by 10% compared to the density in the control plots (Fig. 5.2) (F 1,648 = 3.94; P = 0.0476). For S. capitatum the accumulated numbers of weevils in
M. anisopliae treated plots was not reduced compared to control plots (F 1,647 = 2.49; P = 0.1151).
In autumn the adult weevils emerged from the soil from mid August until the beginning of November, reaching a maximum of 49 S. melanogrammum and 11 S. capitatum per m² per week in the control
plots in the end of September. In treated plots the maximum number of S. melanogrammum was reached one week earlier by 21 weevils per m² per week. The maximum number of S. capitatum in
treated plots was reached in mid September by 14 weevils per m² per week. The accumulated number of S. melanogrammum caught in autumn 2002 in emergence traps was 149 per m² in control plots
and 84 per m² in treated plots (Fig. 5.2), which again was significantly different (F 1,589 = 18.14; P < 0.0001). For S. capitatum the accumulated number of weevils caught was 29 per m² in control plots
and 35 per m² in treated plots (F 1,588 = 0.13; P = 0.7163).
5.1.2.4 Population level in 2003
No significant differences were seen between M. anisopliae treated and control plots neither in spring nor in autumn (Spring: S. melanogrammum: F1,589 = 0.01; P = 0.9486; S. capitatum: F1,589 = 0.03; P = 0.8567. Autumn: S. melanogrammum: F1,589 = 0.82; P = 0.3656; S. capitatum: F1,588 = 0.03; P = 0.1541), which means that no long-term effect was obtained (Fig. 5.2).
Click here to see Figure 5.1
Figure 5.1: Density (no. per m²) in 2001 and 2002 of adult S. melanogrammum (left column) and S. capitatum (right column) on the experimental field site treated with either M. anisopliae BIPESCO 5 (Treated) or 0.05% Triton x-100 (control) in summer 2000 (July 7 and July 19) and summer 2001 (June 26 and July 10) after soil application against larvae. The density was measured by weekly counting of number of weevils caught in 30 emergence traps per treatment.
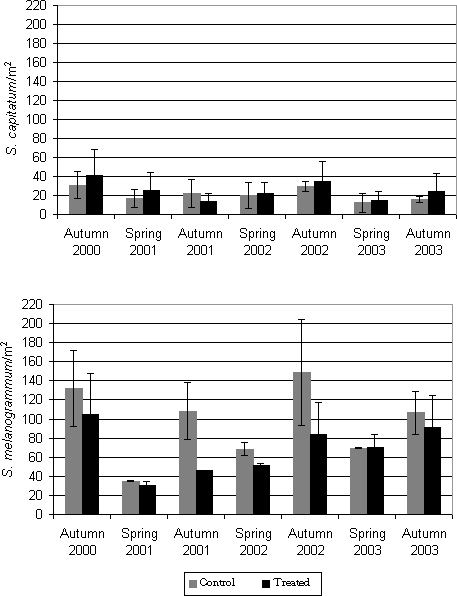
Figure 5.2: Density (accumulated numbers) in 2000, 2001, 2002 and 2003 (no. per m²) of adult S. melanogrammum (bottom) and S. capitatum (upper) on the experimental field site treated with either M. anisopliae BIPESCO 5 (Treated) or 0.05% Triton x-100 (control) in summer 2000 (July 7 and July 19, 2000) and summer 2001 (June 26 and July 10) against larvae. The density was measured by weekly counting of number of weevils caught in 30 emergence traps per treatment.
5.2 Soil application of Metarhizium anisopliae against ovipositing females
5.2.1 Materials and methods
The same A. procera plantation as used for soil application of M. anisopliae (BIPESCO 5) in summer 2000 and 2001 against larvae was chosen as field site for this experiment. Another part of the
plantation was thus divided into four new plots each covering around 125 m². Untreated border zones of 4-5 m separated the plots. Two plots were treated with M. anisopliae and two plots served as
control and were sprayed with 0.06% Triton-X 100 only.
M. anisopliae (BIPESCO 5) was applied to two plots on April 25 and May 9, 2001 by spraying 200 ml per m² of a M. anisopliae suspension adjusted to 2.3 x 107 and 5.0 x 107 conidia per ml 0.05 %
Triton X-100, respectively. The final concentration sprayed on each date was thus 4.6 x 1013 and 1 x 1014 conidia per ha. The conidia of M. anisopliae were produced by Prophyta (Germany). The two
control plots were treated with 0.05 % Triton X-100 only.
The effect of treatment on the population levels was determined as described in paragraph 5.1.1.
In order to estimate the prevalence of the applied fungus as well as the prevalence of natural infections, the weevils were sampled from traps once a week from the beginning of April 2001 until mid June
2001. The weevils were brought to the laboratory where they were counted and determined to species. Live weevils were incubated individually in plastic cups (30 ml) at 20°C and a light regime of 16:8
L:D. Each cup contained 3% water agar in the bottom to keep humidity high and a shoot of A. procera was provided as food. The mortality was recorded weekly for fours weeks, and dead weevils were
removed and placed in humid chambers and fungal infections were diagnosed based on morphological characters (Samson et al., 1988; Humber, 1997).
5.2.2 Results
5.2.2.1 Prevalence study in spring 2001
Prevalence data of fungal infections in adult S. melanogrammum and S. capitatum collected in spring 2001 are shown in Fig. 5.3. For S. melanogrammum the highest prevalence of fungal infection (90.2
%) was found on May 9, a result of the first release of the fungus, and the highest prevalence of fungal infection for S. capitatum was obtained on May 16 (78.1%). In the untreated control plots, 82
individuals of S. melanogrammum were sampled and incubated, but only one was infected by M. anisopliae while no infected individuals of S. capitatum were found. The infected individual was captured
from one of the emergence traps on the borderline to a treated plot.
Besides the applied fungus, natural infections with B. bassiana and P. farinosus and V. lecanii were observed but only at very low prevalences (below 8%).
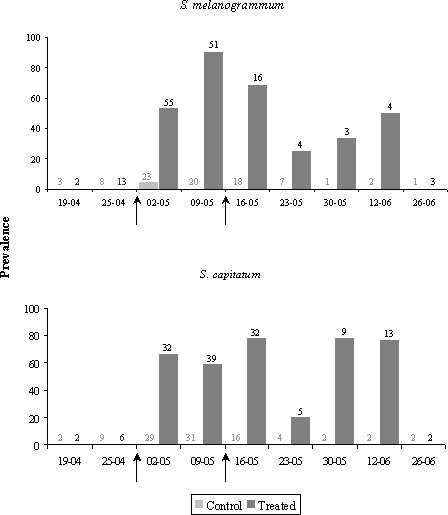
Figure 5.3: Prevalence of M. anisopliae in adult Strophosoma melanogrammum (upper graph) and Strophosoma capitatum (lower graph) populations collected from emergence traps in 2001 after treatment with Metarhizium anisopliae BIPESCO 5 (treated) or with 0.05 % Triton X-100 (control) against ovipositing females. Arrows mark application time (April 25 and May 9, 2001).
5.2.2.2 Population level in 2001
In spring adults emerged from the soil from the beginning of April until mid June (Fig 5.4). The numbers of both S. melanogrammum and S. capitatum caught from emergence traps in 2001 is shown in
figure 5.4. The number of both S. melanogrammum and S. capitatum caught from emergence traps in spring was not significantly different between the M. anisopliae treated plots and the Triton-X treated
plots (S. melanogrammum: F1,529 = 3.48; P = 0.0625; S. capitatum: F1,529 = 3.55; P = 0.0601).
In autumn, the adult weevils emerged from the soil from mid August until the beginning of November. The number of S. melanogrammum caught in emergence traps in the M. anisopliae treated plots was
again higher but not significantly, than the number caught in the control plots (F1,707 = 0.88; P = 0.3490), whereas accumulated density of S. capitatum was reduced by approximately 50% in the M.
anisopliae treated plots compared to the number caught in the control plots (F1,707 = 4.70; P = 0.0304).
5.2.2.3 Population level in 2002
In spring 2002, adults emerged from the soil from the beginning of April until mid June, reaching a maximum of 10 S. melanogrammum and 11 S. capitatum per m² per week in the control plots in mid
April. In treated plots, the maximum number of weevils was also reached in mid April by 12 S. melanogrammum and 5 S. capitatum. The density of S. melanogrammum in the M. anisopliae treated
plots was higher than in the plot treated with Triton-X only but not significantly (F1,648 = 3.43, P = 0.0643). For S. capitatum the accumulated density of weevils in M. anisopliae treated plots was slightly
lower compared to control plots (Fig 5.5) but again the difference was not significant (F1,648 = 2.52, P = 0.1129).
In autumn the adult weevils emerged from the soil from mid August until the beginning of November, reaching a maximum of 29 S. melanogrammum and 28 S. capitatum per m² per week in the control plots in mid September. In treated plots the maximum of weevils was also reached in mid September by 26 S. melanogrammum and 17 S. capitatum. In autumn the density of both S. melanogrammum and S. capitatum were significantly lower in the M. anisopliae treated plots compared to the control plots (S. melanogrammum: F1,589 = 6.59, P = 0.0105; S. capitatum: F1,589 = 6.45, P = 0.0114). The accumulated number of S. melanogrammum caught in autumn 2002 in emergence traps was 102 per m² in control plots and 77 per m² in treated plots (Fig 5.5). For S. capitatum the accumulated number of weevils caught was 69 per m² in control plots and 46 per m² in treated plots (Fig. 5.5). Thus S. melanogrammum was reduced by 25% and S. capitatum by 33%.
5.2.2.4 Population level in 2003
In 2003 no significant differences were seen between M. anisopliae treated and control plots neither in spring nor in autumn (Spring: S. melanogrammum: F1,589 = 0.01; P = 0.9486; S. capitatum: F1,589 = 0.03; P = 0.8567. Autumn: S. melanogrammum: F1,589 = 0.82; P = 0.3656; S. capitatum: F1,588 = 0.03; P = 0.1541, which means that no long-term effect of treatment was obtained (Fig. 5.5).
Click here to see Figure 5.4
Figure 5.4: Density (no. per m²) in 2001 and 2002 of adult S. melanogrammum (left column) and S. capitatum (right column) on the experimental field site treated with either M. anisopliae BIPESCO 5 (Treated) or 0.05% Triton x-100 (control) in spring 2001 (April 25 and May 9) after soil application against ovipositing females. The density was measured by weekly counting of number of weevils caught in 30 emergence traps per treatment.
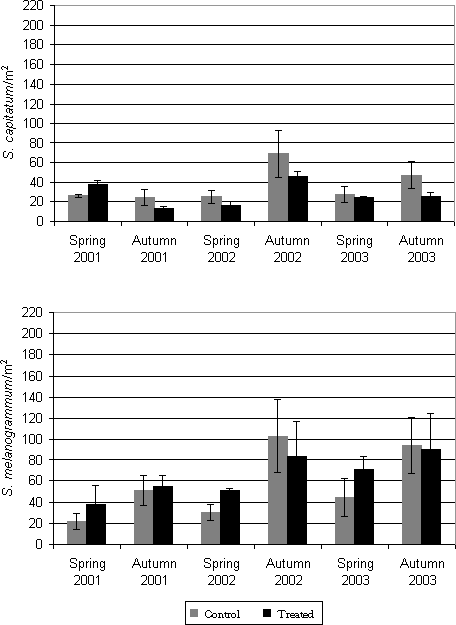
Figure 5.5: Density (accumulated numbers) in 2001, 2002 and 2003 (no. per m²) of adult S. melanogrammum and S. capitatum on the experimental field site treated with either M. anisopliae BIPESCO 5 (Treated) or 0.05% Triton x-100 (control) in spring 2001 (April 25 and May 9) against ovipositing females. The density was measured by weekly counting of number of weevils caught in 30 emergence traps per treatment
5.3 Soil application of B. bassiana and of M. anisopliae against adults emerging from the soil in spring
5.3.1 Materials and methods
The A. procera plantation used for soil application of M. anisopliae was also used for soil application of M. anisopliae (BIPESCO 5) and B. bassiana (KVL 98-20, KVL 02-04 and KVL 02-14). All the
B. bassiana isolates originated from natural infections in Strophosoma collected in Denmark.
Another part of the plantation was divided into five new plots each covering 9 m². Untreated border zones of 4-5 m separated the plots. Four plots were treated with one of the isolates and the last plot
served as control plot.
The treatments were applied to the plots on April 23, 2002 and May 7, 2002 by spraying 200 ml per m² of the fungal suspension adjusted to 5.0 x 107 conidia per ml 0.05 % Triton X-100. The final
concentration sprayed on each date was thus 1 x 1014 conidia per ha. The control plot was treated with 0.05 % Triton X-100, only.
Adult weevils were sampled from 9 evenly distributed emergence traps in each plot once a week from the beginning of April until June 2002. The weevils were counted and determined to species level.
The prevalence of the applied fungus as well as the prevalence of natural infections was determined as described in paragraph 5.2.1.
5.3.2 Results
Prevalence data for adult S. melanogrammum and S. capitatum collected in spring 2002 from the field experiment are shown in Table 5.1 (data for the entire season is pooled). For S. melanogrammum
the highest prevalence (76 %) was found in plots treated with BIPESCO 5. The highest prevalence for S. capitatum was also obtained in the plot treated with BIPESCO 5 (80%).
Table 5.1: Prevalence in adult S. melanogrammum and S. capitatum populations collected from emergence traps in 2002 after treatment with M.anisopliae (BIPESCO 5), B. bassiana (KVL 98-20, KVL 02-04 and KVL 02-14) or with 0.05 % Triton X-100 (control) on April 23 and May 7, 2002.
Isolate |
S. melanogrammum |
S. capitatum |
Control
BIPESCO 5
KVL 98-20
KVL 02-04
KVL 02-14 |
(0/18) = 0 %
(25/33) = 76%
(24/52) = 46%
(12/29) = 41%
(12/18) = 67 % |
(0/5) = 0 %
(8/10) = 80%
(10/17) = 59%
(7/13) = 54%
(2/4) = 50 % |
5.4 Application of tree trunks with lanes of Beauveria bassiana
In a preliminary experiment high infections (80 %) were initiated in Strophosoma spp. populations by treating the tree trunks with lanes covered with sporulating cultures of B. bassiana (Boverol®). The
fungus showed good survival for several months.
5.5 Discussion
Populations of Strophosoma spp. were reduced by soil application of M. anisopliae both when applied against larvae and against adults emerging from the soil to feed in the canopy. Reductions were
significant. In both experiments the main reduction of the populations was monitored in autumn the year following application, most likely due to the two-year life cycle of the weevils (chapter 4).
5.5.1 Control of larvae
No other studies have ever been reported on biocontrol of Strophosoma spp. with insect pathogenic fungi, while some experience has been obtained with other curculionid larvae. Moorhouse et al. (1993a)
used M. anisopliae to control larvae of the vine weevil Otiorhynchus sulcatus Fabr. and by a prophylactic treatment in pots in greenhouses they managed to reduce the population by 78 %. Greenhouses
have, however, a very controlled environment. Outdoor, Moorhouse et al. (1993b) managed to reduce O. sulcatus larvae by 43-62 %, but they noted a high variation in larval control (from zero to 96 %).
Quintela et al. (1998) were less successful to control larvae of Diaprepes abbreviatus L. in soil using unformulated conidia of M. anisopliae and B. bassiana. Only minimal larval mortality was found
(10.2%) and only when using B. bassiana. Even when using formulated conidia of M. anisopliae, Verkleij et al. (1992) did not obtain significant mortality in Sitona lineatus L. In comparison with for
example greenhouse trials our stand is a highly diverse environment and the obtained control of Strophosoma spp. larvae in the present investigation must be regarded as successful.
When M. anisopliae was applied in summer against the larvae, application had no effect on adult population levels in the autumn the same year. In contrast, a significant reduction of population levels was
monitored the following autumn. Further, the effect on S. melanogrammum was greater than on S. capitatum. We can hypothesize several explanations for these delayed effects and the differences
between the two species: 1) first instar larvae falling from the foliage to the ground are more easily hit by the fungus than bigger larvae developing in the soil, 2) small larvae are more susceptible to the fungus
than bigger larvae, 3) the fungus can survive in the soil and infect bigger larvae over a prolonged period of time, 4) despite the apparent similar biology of the two species small differences in larval behaviour
in the soil can prove significant for the success of microbial control.
Due to the lack of the knowledge about the process of the first instar larvae falling from the foliage to the ground at the initiation of the field experiments, the treatment of larvae was targeted against the larvae
developing in the soil. Hence, it was timed to take place mid summer. On the basis of our new knowledge, a treatment in early June would be a more optimal timing for targeting the small larvae droping to
the ground. Although the population reduction obtained in the experiment was more than 50%, it is most likely that an optimisation of timing would reduce the populations even more. More extensive trials
evaluating the timing of application are needed.
5.5.2 Control of adult weevils
Reports on microbial control of adult curculionids are only rarely found in the literature. However, Nankinga and Moore (2000) used B. bassiana to control the adult banana weevil, Cosmopolites sordidus
(Germar) and managed to reduce the population significantly, but due to the extremely different environment for banana production in contrast to greenery production in Denmark their experience is hardly
comparable with ours.
The effect of treatment of adult Strophosoma spp. was less than treatment of larvae, but still significant. Again, the main effect on the population levels was monitored 1½ year after treatment. However, S.
capitatum responded to treatment already the same year. The explanation to this difference from S. melanogrammum is not obvious: A higher degree of flexibility in the development time from egg to adult
for S. capitatum or differences in the siting or mobility of the larvae in the soil at different times of the year and hence the ability of the fungal conidia to infect one-year old larvae are possible reasons.
High prevalences of M. anisopliae were recorded in both adult weevil species. The data from the bioassays indicated that such infected adults are expected die within a couple of weeks (see chapter 2).
Thus, egg densities are likely to be reduced and the population levels after 15-17 months diminished accordingly. Further, there may have been an effect of the fungus on the first instar larvae passing through
upper soil layer two months later.
5.5.3 Prospects for development of a microbial control strategy
In accordance with the very nature of biological control in which the control agent requires a certain period of time to act on target, no immediate lethal effect on the pest population will occur as is normally
obtained by chemical pesticides. Therefore the strategy of microbial control will necessarily differ from the strategy of a traditional chemical control.
Microbial control of Strophosoma spp. in greenery plantations will primarily aim at preventing damages by a general reduction of population levels. The control can be specifically targeted either against the
larvae or the adults. Application against adults will significantly reduce the pest insect population levels the following year. However, some damages are potentially reduced already within some weeks after
treatment, as the fungus-infected adults die within a short period of time. Also, the feeding activity may be reduced by infection, but so far it remains unexplored how fungal infection influences feeding activity
of Strophosoma spp.
When treating the larvae, efficiency of control appear to be higher; however, when using this strategy no effects on damage levels can be expected until approx 1½ years after treatment. Assessment of the
effect of treatments on damage levels was outside the scope of this project but needs further examination.
Irrespective of the target stage of Strophosoma, the overall strategy of microbial control will be prophylaxis. This implies that the fungus should be applied in the stands prior to years when clipping of
greenery will take place. During the entire rotation period of the stands several applications will probably be necessary. More detailed knowledge about the behaviour and persistence of M. anisopliae in
forest soils and greenery plantations is needed to fully evaluate this strategy.
The number of prophylactic treatments could be reduced and the strategy thus refined by development of an early warning system enabling the growers to predict population development. It is well known
that population levels vary significantly between years, but knowledge about the biotic and abiotic factors determining population densities of S. melanogrammum and S. capitatum is however not yet
sufficiently available.
Due to environmental and non-target reasons (see chapter 6 and Vestergaard et al., 2003), `fungal bands' placed on tree trunks should also be considered in the future control of adult weevils. The
preliminary experiment showed high prevalences of the applied fungus in recaptured weevils and this method of application would also match the long activity period of the adult weevils. Furthermore, fungal
bands can be removed when no longer needed. Good control has been obtained with fungal bands in China against adults of the Asian long horned beetle, Anaplophora glabripennis Motschulsky (Higuchi
et al., 1997; Dubois et al., 2004). The application method needs further development for practical use. Finally, evaluation of formulated products of fungal inoculum is essential for future control of
Strophosoma spp.
5.6 Conclusions
- The Strophosoma spp. populations were significantly reduced following soil application in summer of M. anisopliae against larvae developing in the soil. The main effect was, however, not seen until
autumn the following year due to the two-year life cycle of the weevils.
- High infections with M. anisopliae in Strophosoma spp. populations were initiated in the plots where this fungus was sprayed against adults in spring.
- The Strophosoma spp. populations were significantly reduced when M. anisopliae was sprayed against ovipositing females in spring. The main effect was, however, not monitored until autumn the
following year.
- High infections with M. anisopliae and B. bassiana were initiated in the adult Strophosoma spp. populations after spring treatment.
- High infections with B. bassiana were initiated in adult Strophosoma spp. captured in the field after they had walked over `fungal bands' placed on tree trunks.
- It is concluded that both M. anisopliae and B. bassiana have a potential for future biological control of Strophosoma spp.
6 Effects of fungi on non-target invertebrates and spatio-temporal distribution
6.1 Non-target effects
6.1.1 Materials and methods
6.1.2 Results
6.2 Spatio-temporal distribution
6.2.1 Materials and methods
6.2.2 Results
6.3 Discussion
6.4 Conclusions
In general it is not possible to reduce a pest population using fungi without also affecting other invertebrates in the environment (Vestergaard et al., 2003). It is important to distinguish between the
physiological host range and the ecological host range. Usually, tests to evaluate the physiological host range of biological control agents are conducted in the laboratory, and this host range therefore
gives information on the potential non-target effects. The ecological host range is studied by sampling insects in the field after application and this gives information on the realistic non-target effects. Usually,
the ecological host range is much narrower than the physiological host range (eg. Hajek et al., 1996) and in many aspects it better reflects the impact of biological control agents on non-target invertebrates in
the ecosystem. The spatial and temporal distribution of an applied fungus will assist in an evaluation of non-target effects over time.
This chapter aims to elucidate some of the environmental effects of spraying hyphomycete fungi in a greenery plantation, in particular M. anisopliae (BIPESCO 5).
6.1 Non-target effects
In order to evaluate the effects on non-target invertebrates the most abundant insect and mite species were collected from a M. anisopliae treated A. procera plantation and incubated in the laboratory in
order to determine the infection level of M. anisopliae. Furthermore, selected isolates were characterised by means of molecular methods in order to examine whether the isolates originating from the
non-target organisms were identical with the applied isolate.
6.1.1 Materials and methods
6.1.1.1 Field site:
A 0.3 ha Abies procera plantation situated in Zealand, Denmark was chosen as field site. The plantation was established in 1983 and has for the last couples of years suffered from damages caused by
Strophosoma spp.
6.1.1.2 Application of Metarhizium anisopliae:
In year 2000, M. anisopliae (BIPESCO 5) was applied twice in two plots each of ~150 m² in a concentration of 1x1014 conidia per ha during summer (June and July) in order to control larvae of
Strophosoma spp. In year 2001, M. anisopliae was also applied twice in a concentration of 4-10x1013 conidia per ha but this time the application took place during spring (April and May) in order to
control adult Strophosoma spp. emerging after their hibernation in the soil.
6.1.1.3 Collection of non-target:
Non-targets living above the soil surface were collected either carefully by hand picking or by sweep net from control plots and from treated plots 7, 14, 75 and 277 days after application. Specimens were
incubated individually in 25 ml plastic cups at 20°C. Water and for most species also food were provided during incubation. Mortality was recorded at regular time intervals for up to eight weeks and dead
individuals were examined for symptoms of insect pathogenic fungi. All insect pathogenic fungi were determined to species level. From M. anisopliae infected individuals representative in vitro isolates were
obtained.
6.1.1.4 Characterisation of collected isolates:
For selected M. anisopliae in vitro isolates, DNA was isolated and the ITS and IGS regions were amplified in 0.2 ml PCR tubes with a total volume of 25 µl in a Perkin Elmer GeneAmp PCR system. The
amplified region was cut by restriction enzymes. Profiles were monitored by electrophoresis in 1.5% agarose gels and detected by staining with ethidium bromide. Sizes of amplification products were
measured from a photograph of the gel (for further detail on the method used se Nielsen et al., 2001).
6.1.2 Results
For non-targets sampled 7 days after application of M. anisopliae high prevalences were found (above 50%) in two insect orders and in ticks (Table 6.1) but declined to 10-20% 14 days after application
(except for target weevils and ticks). However, M. anisopliae infected ladybirds were found 277 days after application.
Table 6.1: Percentage of targets (weevils) and non-targets infected with Metarhizium anisopliae sampled from the greenery plantation at different time after application with BIPESCO 5.
Non-target |
|
Days after treatment with M. anisopliae |
Control |
7 |
14 |
75 |
277 |
INSECTA |
|
|
|
|
|
Psocoptera (bark lice) |
- |
(0/40) = 0% |
- |
- |
- |
Hemiptera |
|
|
|
|
|
Cicadellidae (leafhoppers) |
(0/51) = 0% |
(3/4) = 75% |
(5/44) = 11% |
(0/60) = 0% |
- |
Miridae (capsid bugs) |
(0/117) = 0% |
(33/64) = 52% |
(9/47) = 19% |
(2/82) = 2% |
- |
Pentatomidae (shield bugs) |
(0/1) = 0% |
(4/8) = 50% |
(0/1) = 0% |
(0/2) = 0% |
- |
Coleoptera |
|
|
|
|
|
Coccinellidae (ladybirds) |
(1/97) = 1% |
- |
- |
- |
(3/30) = 10% |
Carabidae (ground beetles) |
(0/6) = 0% |
- |
(1/6) = 16% |
- |
- |
Curculionidae (target weevils) |
(1/103) = 1% |
(50/87) = 57% |
(69/90) = 77% |
- |
- |
ARACHNIDA |
|
|
|
|
|
Ixodidae (ticks) |
(0/29) = 0% |
- |
(38/67) = 57% |
- |
- |
Opiliones |
- |
(1/16) = 6% |
- |
- |
- |
The ITS and IGS fragment pattern confirmed that the genotype isolated from infected non-targets was the same as the isolate applied (Fig. 6.1).
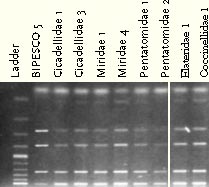
Figure 6.1: IGS fragment pattern of Metarhizium anisopliae isolates originating from non-targets collected after spraying with BIPESCO 5
6.2 Spatio-temporal distribution
6.2.1 Materials and methods
6.2.1.1 Soil samples:
All soil samples were taken with a cylindrical soil sampler with an inner diameter of 3 cm. Samples were taken in three depths (0-5; 5-15 and 15-25 cm) both before and after application of M. anisopliae in
an A. procera plantation in spring 2001. Three soil samples per treatment were taken 0, 13, 89, 180 and 418 days after application of entomopathogens. Soil samples were stored at 5°C and constant dark
until analysis.
6.2.1.2 Isolation of entomopathogens from Metarhizium anisopliae treated soil:
The density of M. anisopliae conidia was determined by plating soil suspensions onto Metarhizium selective medium medium (SDA with 0.5 ml l-1 dodine, 50.0 ppm Chloramphenicol, 50.0 ppm
Streptomycin sulphate) using the following protocol: 1 g of soil was whirl mixed for 30 sec. in tests tubes added 9 ml of 0.05% Triton X-100. 100 l suspension or 100 l of a diluted suspension was
distributed with a Drigalsky spatula onto a petri-dish with the selective medium. Plates were incubated at 23°C for 3-5 days and colony-forming units (cfu) of M. anisopliae were counted. Three replicates
per sample were prepared.
6.2.2 Results
Before spraying with M. anisopliae the fungus did not occur naturally at detectable levels in the plantation soil. After spraying with M. anisopliae the fungus was solely found in the plots where the fungus
was applied. M. anisopliae could be detected in the soil for at least 418 days after the application. The highest concentration measured was 1x105 cfu per g of dry soil in 0-5 cm's depth just after spraying
of M. anisopliae. From 89 days after first spraying until 418 days after the first spraying the concentration of M. anisopliae in the upper layer remained around 1x104 cfu per g of dry soil. In the deeper
layer of soil the concentration of M. anisopliae remained lower than in the upper layer throughout the season and except from just after the first spraying the concentration never exceeded 1x103 cfu per g of
dry soil.
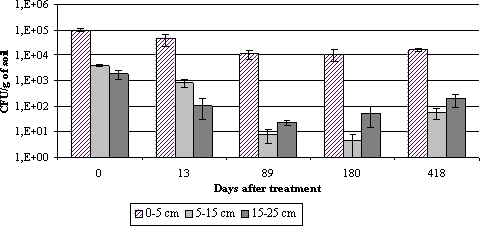
Figure 6.2: Density of Metarhizium anisopliae (cfu's per g dry soil) in soil collected from the experimental field site after treatment with M. anisopliae (BIPESCO 5) in spring 2001.
6.3 Discussion
M. anisopliae has a wide natural host range and the fungus has been reported from many insect orders (extensive review by Vestergaard et al., 2003). In studies on the physiological host range of specific
isolates, transmissions between insect orders are commonly reported (James and Lighthardt, 1994; Danfa et al., 1999; Vestergaard and Eilenberg, 1999; Vestergaard et al., 2003). The natural infection of
fungi in Carabids seems to be low: It was found to be around 2% in Danish lucerne and cabbage fields (Steenberg et al., 1995; Vestergaard and Eilenberg, 2000). Data from the greeneries support these
data. Upon application in a lucerne field, M. anisopliae infected several Staphylinid and Carabid genera (Vestergaard and Eilenberg, 2000). It is, however, questionable if there are any population effects on
non-targets. In Finland, where M. anisopliae was sprayed onto an oilseed rape field, no difference in the number of five dominant carabid species compared to the control was found (Zeng et al., 2001).
It is also important to keep in mind that most of the non-targets infected by M. anisopliae have a shorter lifespan than the target Strophosoma spp. Therefore the populations of non-targets will recover
much faster than the target. Also, some non-target effects can prove useful. Our finding that M. anisopliae had a significant effect on ticks, Ixodes ricinus, can actually be the first step towards the use of the
fungus against this pest in Europe. In North America, a commercial product based on M. anisopliae was in summer 2003 launched for control of tick species occurring there (E. Butts, pers.comm.).
It is noteworthy that M. anisopliae was not found in the soil in the control plots in the plantation. In a Danish study in Abies plantations of antagonists to the needle miner, Epinotia fraternata,
Münster-Swendsen (1989) reported the occurrence of Paecilomyces farinosus in the hibernating larvae. In our study this fungus was also found naturally occurring in the stand, while M. anisopliae may
occur, but at levels below the detection limit. This is opposite to the findings in agricultural fields. Here, Vestergaard and Eilenberg (2000) reported natural levels up to 300 cfu per g soil. In Finland, Vänninen
et al. (2000) reported natural levels even up to 9000 cfu of M anisopliae per g soil, also in agricultural soils. It seems thus that M. anisopliae is not a significant fungus in natural greenery systems, but this
needs further verification.
In our studies we found non-target effects even 277 days after application and the fungus was present in the soil 418 days after application. In agricultural soils in Denmark (Vestergaard and Eilenberg, 2000)
and Finland (Vänninen et al., 2000) significantly higher levels of M. anisopliae were found in treated plots compared to untreated 4 and 3 years after application, respectively. In a study from Australia it
was found that three years is the maximum period for M. anisopliae to provide control in the soil after application (Milner et al., 2003). Importantly, the authors concluded that rainfall and soil type had
negligible effects on the persistence of the fungus. In Tasmania, results from grassland indicate that applied M. anisopliae persists for more than seven years (Rath et al., 1997).
It is still debatable, whether a long persistence of an applied fungus is simply due to 1) a long survival of single conidia, 2) a saprophytic growth and sporulation, or 3) a multiplication in hosts. Rath et al.
(1997) hypothesized the long persistence in their studies to be due to a multiplication in the host. Whether or not a long persistence should be regarded as an advantage or not depends on the type of control
wanted. In case of a wish to obtain long-term protection, a long persistence of the biocontrol agent should be regarded as a positive feature.
6.4 Conclusions
- M. anisopliae infected a range of non-targets in the field after its release but the effect on the population development is assumed to be limited.
- Before spraying with M. anisopliae the fungus did not occur naturally in the plantation soil. After spraying with M. anisopliae the fungus was only found in the plots where the fungus was applied.
- M. anisopliae could be detected in the soil for at least 418 days after the application. The highest concentration measured was 1x105 cfu per g of dry soil in 0-5 cm's depth just after spraying of M.
anisopliae.
- The concentration of M. anisopliae in the upper layer remained around 1x104 cfu per g of dry soil during a long period after treatment. This concentration corresponds to the concentration of M. anisopliae, which can be found in agricultural fields.
- To minimise the environmental effects, the time and place of application should be precisely targeted.
- Long-term non-target effects should be studied more extensively in different stands.
7 Discussion and Conclusions
7.1 Population studies
7.2 Molecular characterization
7.3 Bio-assays and strain selection
7.4 Field releases and biocontrol experiments
7.5 Non-target effects
7.6 Conclusions
In the preceding chapters a discussion on the specific obtained results were included and will not be repeated here. Below is a brief discussion on the more general, conceptual elements of the methods in the
studies performed.
7.1 Population studies
Population ecology is a core element in the discipline ecology, and insect population ecology has over the past been subjected to many studies, and results have been compiled in classical books like Price
(1975). The classical methods for population studies include method for absolute and relative population estimates (Southwood, 1980). In this book, which has been published in many editions, standard
devices for such studies are described. Our emergence traps and funnel traps are a well-know device for absolute population estimates and our design for the traps were based on the recommendations in
Southwood (1980). Methods for absolute population estimates are to be preferred over relative estimates, whenever possible, since the results from the former can be calculated to an estimate on number of
individuals per surface square unit. The present project managed to obtain such data, which are very valuable to understand the phenology and development of Strophosoma species.
The knowledge and understanding of pathogen-insect interaction is generally not as well elucidated as the interactions between insect populations and predators and parasitoids. Examples of the latter
interactions have to a much greater extend been adopted in general textbooks on ecology (for example Begon et al. (1995). The methods employed for the assessment of fungus prevalence in our studied
insect populations were based on the conceptual framework defined by Fuxa and Tanada (1987). In their book, the interaction between insect pathogens and insect populations are seen in an
epidemiological context, defining the terms `prevalence' and `incidence' for insect pathology. The present project thus gave data on the prevalence on fungal diseases in the insect populations in a correct
context.
7.2 Molecular characterization
The use of DNA-based methods for characterization and identification of a wide range of organisms is a rapidly developing area, and every year, new methods or modification of existing methods become
available. The importance of genetic studies in biological control is outlined in Ehler et al. (2004), although none of the cases in their book are from insect-pathogen interaction studies. We used molecular
methods in two ways: 1) in order to validate the phenological data on the two included Strophosoma species, 2) in order to evaluate the effects and the persistence of a released fungus.
With respect to 1), this project managed to validate the phenological data, thus allowing for the first time a more full understanding of the life cycle of S. melanogrammum and S. capitatum. It can be
expected in the future that DNA-based methods will be used to a much greater extent in studies of species complexes in insects, and this study is a valuable contribution to the studies in the family
Curculionidae.
With respect to 2), the fungus M. anisopliae was not present at detectable level in the A. procera stands used in this study before the application. To some extent, the site could therefore be regarded as an
`agent free' environment. This gave the advantage that our characterization did not aim to discriminate between a range of natural genotypes and the applied genotypes, but solely aimed to verify that the
applied genotype was the causal agent. We managed to do this, and especially as concerns non-target effects, it is desirable that such verification is possible.
7.3 Bio-assays and strain selection
Our approach to perform bio-assays with insect pathogenic fungi was based on the classical concept (Lacey, 1997a): first, the pathogenicity of the fungus isolates was proven. Then, quantitative bio-assays
were performed in order to discriminate between the levels of virulence. Virulence is `the disease producing power of a microorganism' (Lacey, 1997a), and is in most cases calculated from data on either
dose-response relationships or experiments providing data on the lethal time or survival time. We used both approaches and obtained a set of data sufficient to select the best isolate on these premises. It
should, however, be stressed that the most virulent isolate in laboratory studies is not necessarily the isolate with the best ecological fitness.
The next step is then either to go straight to the field or to add semi-field experiments to obtain information about ecological fitness. In the present project it was decided to go directly from laboratory
experiments to field experiments, although additional laboratory experiments were carried out after the initiation of the field experiments. In practice, it is almost never possible to `wait' for all needed
information before the field experiments to test the ecological fitness. In this case, a perennial cropping system and pest insects with an expected life cycle of more than one year made it indispensable to
postpone field experiments. The selected and later applied strain of M. anisopliae, BIPESCO 5, was known for its high ecological fitness in other cropping systems, so it was expected that this strain would
be ecologically fit also in our system. The outcome of the field experiments confirmed this hypothesis.
7.4 Field releases and biocontrol experiments
The studies on efficacy after field releases were based on the expectation of an inundative effect. Inundation biological control is performed when large amounts of an agent are released with the expectation
that control will be achieved with the released organisms themselves (Eilenberg et al., 2001). Inundation is thus complementary to inoculation biological control. In the case of inoculation, low amounts of an
agent are released with the expectation that the released organisms will multiply. In many cases, however, the final outcome of an inundation biocontrol seems to be an additive result of the `real' inundation
and some inoculation effects so these two strategies are often together listed as augmentation (Hajek, 2004). In the field experiments performed under the present project were done by inundation.
Further studies may show if there in addition to the inundation effects are also inoculative effects, for example by production of conidia from infected hosts, which result in infection of uninfected hosts.
Hand-operated sprayer for application of insect pathogenic fungi has been used in many earlier described studies aiming to test field efficacy of insect pathogens (Lacey and Kaya, 2000), in relatively small
areas of application. The application of an aqueous solution of conidia by one person by a hand-operated sprayer (Bateman et al., 2000) allows a relatively precise dosage. The environment at the field sites
used in the resent studies was an uneven ground surface, with stems and decaying twigs in clusters (major clusters of twigs were, however, removed before application). Much care was therefore needed to
ensure that spraying was exact in dosage and distribution. The data on density of the M. anisopliae from sprayed plots indicates that we managed to do this. Obviously, large-scale experiments and routine
usage require a further development of the application technique.
The assessment of efficacy made benefit of the data from the population studies. As a basic rule, efficacy by an applied insect pathogen should be based on both 1) prevalence assessments, 2) population
estimates of targets, 3) crop yield and/or quality. In the present project, data on 1 and 2 were obtained, while the project did not aim to assess 3). Additional studies are needed to obtain such data.
7.5 Non-target effects
Nowadays, studies on non-target effect are regarded as an integral element in biocontrol studies, both due to the demands from authorities before a registration in EU is possible (Anonym, 2003a) and due to
the intention of developing biological control as environmentally sound plant protection.
It is essential to discriminate between the ecological and physiological host range (see chapter 6 on non-target effects). The present study aimed to pay full attention to the ecological host range. The most
used method for ecological non-target studies is based on the collection of selected non-target species followed by incubation in the laboratory and diagnosis of possible pathogens in case of death of the
incubated non-target (Hokkanen and Hajek, 2003). Results obtained in this project documented that the applied fungus was able to cause infections in non-target arthropods. The ecological host range thus
gave valuable data on the expected events in a field situation. However, even though infection was seen in the sampled non-target arthropods the effect of the population development of these non-targets is
not known, since assessment of population effects would require more laborious studies than the resources available in this study. A general drawback of field studies is, however, that the sampling of
non-targets depends on the practical circumstances: only if a sufficient number of a specific non-target species is available, such data are achievable. If non-target studies are to be performed at a number of
localities it cannot be guaranteed that the same non-target species can be collected on each site. The same is to be said about studies on the same locality over several years.
In recent years, the attention to the so-called indirect effects of non-targets has increased. The basic assumption is that the direct effect on non-target species is not the only expected population effect. If a
non-target species decreases in numbers, natural enemies of that species will also decrease etc. (Wajnberg et al., 2001). We did not address such questions in our studies; even though they can potentially
be present.
7.6 Conclusions
The aim of the projects was to clarify the potential of insect pathogenic fungi for microbial control of Strophosoma spp. The conclusions in the chapters can be summarized as follows:
- Isolates of the fungi Metarhizium anisopliae, Beauveria bassiana, Paecilomyces farinosus and Verticillium lecanii were all pathogenic to Strophosoma melanogrammum and S. capitatum.
- Based on virulence studies in the laboratory, one isolate of M. anisopliae was selected for field studies.
- Molecular methods were successfully implemented to assist in the identification of Strophosoma spp. larvae as a prerequisite in the elucidation of the life cycles of Strophosoma melanogrammum and S. capitatum.
- Adults of both S. melanogrammum and S. capitatum feed on the greenery in spring and autumn.
- Eggs of S. melanogrammum and S. capitatum are deposited in the canopy and the small larvae drop to the ground to complete their development to adults. This behaviour makes it possible to specifically target a microbial control towards the small larvae.
- Both Strophosoma species have a development time of 15-17 months,
- although S. capitatum may have a greater flexibility in its development time from egg to adult.
- Field application of M. anisopliae in summer against larvae of Strophosoma spp. resulted in population reductions the following year.
- It was also possible to obtain a reduction of population levels by treating the adult Strophosoma spp. during spring prior to reproduction.
- The applied fungus, M. anisopliae, was able to infect non-target coleopterans, hemipterans and ticks in the field plots.
- M. anisopliae BIPESCO 5 persisted in the soil for more than one year after application.
- In overall, the potential of microbial control of Strophosoma spp. in Danish A. procera stands using insect pathogenic fungi is regarded as high.
- We suggest, based on the present knowledge, the future strategy of application is a prophylactic treatment of the greenery stands prior to harvest of the decoration green.
8 Perspectives
8.1 Biological control in Danish greenery and Christmas tree plantations
8.2 The status of insect pathogenic fungi in biological control
8.3 The grower's situation
8.4 Occupational health effects
8.5 Consumers' preferences
8.6 The final societal evaluation
8.1 Biological control in Danish greenery and Christmas tree plantations
Denmark has a long tradition for biological control. Approximately 50 organisms are commercially available for growers, especially for use in glasshouses (Eilenberg et al., 2000). Outdoor biological control
is more limited and there is no long tradition for biological control in greenery and Christmas tree plantations. Since 1993, microorganisms for use as biological control agents must pass the approval in EU.
Very few organisms have, however, passed so far due to the laborious work related to such an approval and not due to rejection because of detrimental effects. The most used microorganisms for biological
control are thus still used by dispensation (A. Fjeldsted, pers.com.). M. anisopliae is commercially available in Europe against curculionid beetles from the genus Otiorhynchus, by dispensation. A
development towards a product against Strophosoma spp. requires an approval from EU. The fungus is already used and much information on for example non-target effects and health effects is available in
Europe and elsewhere. It is therefore reasonable to assume that it will be possible to have the fungus (or the other tested fungi) approved for commercial use.
It is, however, important that the application of a biocontrol agent is optimized in order to avoid significant non-target effects on other arthropods. In the case of microbial control of Strophosoma spp. larvae
with M. anisopliae in greenery plantations, a precise targeting at the first instars larvae during the process of dropping to the ground would improve control leading to a lower effective dose and consequently
a reduction of adverse side effects. Concerning control of adult Strophosoma spp. `fungal bands' placed on tree trunks should also be considered in the future control since such bands only expose
arthropods on the tree trunks and not those on the ground. Furthermore, the fungal bands can be removed when they are not needed for control thus decreasing the exposure time for the non-target.
However, for both strategies outlined here additional studies are needed with special attention to long-term non-target effects using the ecological host range as the measure.
8.2 The status of insect pathogenic fungi in biological control
The main insect pathogenic fungi in biological control are from the genera Metarhizium, Beauveria, Paecilomyces and Verticillium (Ingliss et al., 2001). They are used worldwide and for example M.
anisopliae is widely used in United States (Anonymous, 2004), Africa (Ekesi and Maniania, 2003) and Latin America (Alves et al., 2003). M. anisopliae is used against a variety of pests from several
insect orders. The potential broad target host range is needed, since many insect species are `local' and without sufficient economic importance for a full product development. The company Prophyta, who
produced our M. anisopliae, agrees that the European `Strophosoma market' is too small to be immediately commercially interesting if a fungus product should be developed to target only Strophosoma
species (P. Lüth, pers.com.). From a company point of view, M. anisopliae is nevertheless attractive because most technology needed for production is known and because of a potential big range of target
species. We recommend that economic analysis on the costs for developing fungi for biological control in greeneries should be analysed in detail.
8.3 The grower's situation
The production of greenery and Christmas trees has both a high export value and a high national value. Also, there is a political intention to minimize the use of chemical pesticides. Growers will probably
easily adopt biological control options, provided the methods work and provided that standard technology can be used or, at least that some easily adoptable new technology can be used. The main method
for application of conidial suspension was based on a personal spray device, which is familiar to growers. The grower attitudes to new technologies are that they are willing to pay an additional cost of 10 –
25 % compared to the conventional (K. Østergaard, pers. com.). It is therefore assumed that a biocontrol product can be accepted even if it may cost more than conventional products. The growers'
economic situation is, however, very severe and it is not to be expected that they can contribute economically significantly to the development of biocontrol products.
Also the strategy of application is important in considerations of adopting new control methods. In the case of Strophosoma spp., no immediate lethal effect on the pests occurs as with traditional chemical
products; therefore the strategy must instead be prophylactic treatment leading to reduction of subsequent population levels.
8.4 Occupational health effects
A conference held November 27, 2003 under the auspices of `Danish Centre for Biological Control' (www.centre-biological-control.dk), paid attention to potential occupational health problems using
microorganisms for biological control (Madsen et al., 2003). Growers can be exposed to large amounts of fungus spores when performing biological control with fungi, and in glasshouses this exposure is
sometimes high, yet insignificant compared with the exposure to various naturally occurring fungi in the cropping system. It is assumed that the exposure to fungi for persons performing biological control
outdoor is much less than in glasshouses. This project did not measure the exposure of the persons who applied M. anisopliae in our studies, but recommend such aspects are taken into consideration.
Personnal protection as recommended by the ministry shoul always be used when treating with biocontrol agents
8.5 Consumers' preferences
Not much is known about consumers' preferences and biological control. It is normally assumed that many consumers prefer crops without chemical agents. In Denmark many organic products are now sold
at a higher price than conventionally grown food products and this documents that consumers are willing to pay a higher price in order to avoid product treated with chemicals. However, user preferences for
organic food products are likely to differ from preferences for non-food products.. Nevertheless, in Denmark the sales of organically grown Christmas trees and greenery have increased over the years and
may point towards a future demand for such products.
However, a preliminary study some years ago in Germany reflected that consumers in general do not regard Christmas trees and greenery as `ecological products'. They were therefore not immediately
willing to pay a higher cost for pesticide free products (K. Østergaard, pers.com.).
We are not able to assess the consumers' reaction concerning biological control using fungi. Do they regard it as a nice and environmentally sustainable technology or do they regard it an unnecessary
complex and `dangerous' biotechnological solution? A very recent study from USA (Jetter and Paine, 2004) showed that urban and suburban residents are willing to pay for biological control in the
landscape. In general they preferred macroorganisms (exemplified by parasitoids) slightly to microorganisms (exemplified by Bacillus thuringiensis), while fungi were not included in the study. In this
context, knowledge about adverse side effects on the environment, for example on non-target, may be an important element in user preferences. Studies on consumers' preferences for biological control
should be initiated in Denmark, both with respect to non-food crops such as greenery and Christmas trees and with respect to food products.
It should also be noted that the perception of risk differs between scientific experts and the public (Sandøe, 2003). While the experts will evaluate the risk as `hazard X probability' the public perception is
more complex. Studies on the public perception have only to a very limited extent so far been applied to biological control studies but will indeed be relevant for evaluating the potential for future use of
biological control.
8.6 The final societal evaluation
As described above, the biological data obtained in this project, point towards a development for commercial use. However, some potential restrictions and conflicts occur, which basically can lead to three
questions: Who pays? Who benefits? Are there any unacceptable risks? These questions are relevant at a societal level and are unavoidable in the development of future biological control.
9 References
Alves, S.B.; Pereira, R.M.; Lopes, R.B. and Tamai, M.A. 2003. Use of entomopathogenic fungi in Latin America. In Upadhyay, R. (ed.): Advances in Microbial Control of Insect Pests, Kluwer
Academic, 193-211.
Anonym 2003a. Forslag til Rådets Direktiv om ændring af bilag VI til Direktiv 91/914/EØF for så vidt angår plantebeskyttelsesmidler, der indeholder mikroorganismer. Kommissionen for de Europæiske
Fællesskaber, 45 pp.
Anonym 2003b. http://www.ncbi.nlm.nih.gov/BLAST.
Axelson, B.; Larsson, S.; Lohm, U. and Persson, T. 1973. Production of larvae of Phyllobius piri L. (Col. Curculionidae) in an abandoned field. Entomologica Scandinavica 4: 21-29.
Balachowski, A. S. 1963. Entomologie appliquée a l`agriculture. Traité. Tome I. Coléoptères. Maison et Cie Éditeurs, Paris, 1391 pp.
Bas, B.; Dalkilic, Z.; Peever, T.L.; Nigg, H.N.; Simpson, S.E.; Gmitter, F.G. and Adair, R.C. 2000. Genetic relationships among Florida Diaprepes abbreviatus (Coleoptera: Curculionidae)
populations. Annals of the Entomological Society of America 93: 459-467.
Bateman, R.P.; Matthews, G.A and Hall, F.R. 2000. Ground based application equipment. In Lacey, L.A. and Kaya, H.K. (eds.): Field manual of techniques in invertebrate pathology. Application and
evaluation of pathogens for control of insects and other invertebrate pests. Kluwer Academic Publishers, 77-112.
Begon, M.; Harper, J.L. and Townsend, C.R. 1995. Ecology. Individuals, populations and communities. Second edition, Blackwell Science, 945 pp.
Bejer, B. 1989. Forstzoologi. DSR Forlag, Copenhagen, Denmark.
Breese, M. H. 1948. Notes on the oviposition site and methods of repro-duction of the weevils Strophosomus melanogrammus (Forster) (Col.). Proceedings from the Royal Entomological Society of
London. 23: 62-65.
Bulat, S. A., Lübeck, M., Mironenko, N., Jensen, D.F .and Lübeck, P. S. 1998. UP-PCR analysis and ITS1 ribotyping of strains of Trichoderma and Gliocladium. Mycological Research 102:
933-943.
Cano, R.J; Poinar, H.N.; Pieniazek, N.J; Acra, A and Poinar Jr., G.O. 1993: Amplification and sequencing of DNA from a 120-135 million-year-old weevil. Nature (London) 363: 536-538.
Chikwhenhere, G.P. and Vestergaard, S. 2001. Potential effects of Beauveria bassiana (Balsmo) Vuillemin on Neochetina bruchi Hustache (Coleoptera: Curculionidae), a biological control agent of
water hyacinth. Biological Control 21: 05-110.
Danfa, A.; van der Valk, H.C.H.G. 1999. Laboratory testing of Metarhizium spp. and Beauveria bassiana on sahelian non-target arthropods. Biocontrol Science and Technology 9: 187-198.
DMI 2004. http://www.dmi.dk/index/danmark/vejrarkiv.
Drabkova, L.; Kirschner, J. and Vlcek, C. 2002. Comparison of seven DANN extraction and amplification protocols in historical herbarium specimens of Juncaceae. Plant Molecular Biology Reporter 20:
161-175.
Dubois, T.; Hajek, E. A.; Jiafu, H. and Li, Z. 2004. Evaluating the efficiency of entomopathogenic fungi against the Asian long horned beetle, Anaplophora glabripennis (Coleoptera: Cerambycidae), by
using cages in the field. Environmental Entomology 33: 62-74.
Ehler, L.E.; Sforza, R. and Mateille, T. (eds.) 2004 . Genetics, evolution and biological control. CABI Publishing, 260 pp.
Ehnström, B. 1985. Ögonviveln och tallbarrgallmyggan- två barrskadegörere på tall. Sveriges Skogvårdsforbunds Tidsskrift 2: 51-55.
Eilenberg, J.; Enkegaard, A.; Vestergaard, S.; Jensen, B. 2000. Biological control of pests on plant crops in Denmark: Present status and future potential. Biocontrol Science and Technology 10:
703-716.
Eilenberg, J.; Hajek, A.E.; Lomer, C. 2001. Suggestions for unifying the terminology in biological control. Biocontrol 46: 387-400.
Eilenberg, J., Nielsen, C., Vestergaard, S., Harding, S., Frølander A., and Augustyniuk, A. 2003. Biological control of weevils (Strophosoma spp.) in Danish greenery plantations. IOBC/WPRS
Bulletin 26: 55-58.
Ekesi, S. and Maniania, N.K. 2003. Metarhizium anisopliae: an effective biological control agent for the management of thrips in horti- and floriculture in Africa. In Upadhyay, R. (ed.): Advances in
Microbial Control of Insect Pests, Kluwer Academic, 165-180.
Erney, S. J.; Pruess, K.P.; Danielson, S.D. and Powers, T.O 1996. Molecular differentiation of Alfalfa Weevil strains (Coleoptera: Curcurlionidae). Annals of the Entomological Society of America 89:
804-811.
Flores, A.G.; De la Rosa, W.; Rojas, J.C. and Castro-Ramirez, A.E. 2002. Evaluation of Beauveria bassiana and Metarhizium anisopliae (mitosporic) against species of the `white brub complex' in the
South of Mexico. Southwestern Entomologist 27: 73-83.
Fuxa, J.R. and Tanada, Y. 1987. Epizootiology of insect diseases. John Wiley and Sons, 555 pp.
Gibas, C. and Jambeck, P. 2001. Bioinformatics computer skills. O’Reilly & Associates, Inc., CA, USA.133-214.
Goettel, M. S., and Inglis, G. D. 1997. Fungi: Hyphomycetes. In.: Lacey, L. (ed.) Manual of Techniques in Insect Pathology. Academic Press, San Diego, 409 pp.
Grimm, R. 1973. Zum Energieumsatz phytophager Insekten im Buchenwald I. Oecologia 11: 187-262.
Hajek, A.E. 2004. Natural enemies – and introduction to biological control. Cambridge University Press, 378 pp.
Hajek, A.E.; Butler, L; Walsh, S.R.A.; Silver, J.C.; Hain, F.P.; Hastings, F.L.; Odell, T.M and Smitley, D.R. 1996. Host range of the Gypsy moth (Lepidoptera: Lymantriidae) pathogen Entomophaga
maimaiga (Zygomycetes: Entomophthorales) in the field versus laboratory. Environmental Entomology 25: 709-721.
Hall, R.A. 1976. A bioassay of the pathogenicity of Verticillium lecanii conidiospores on the aphid Macrosiphonella sanborni. Journal of Invertebrate Pathology 27: 293-322.
Hansen, V. 1964. Snudebiller. BillerXXI. Danmarks Fauna 63. G.E.C Gads Forlag, Copenhagen, Denmark.
Harding, S. 1993. Gråsnuder – et aktuelt skadedyr. Skoven, No 8, 330-331.
Harding, S. and Ravn, H.P. 1999. Skovbrugets skadedyr 1998. Skoven, No. 4, 169-172.
Harding, S.; Annila, E.; Ehnström, B.; Halldorsson, G., and Kvamme, T. 1998. Insect pests in forest of the Nordic countries 1987-1990. Rapport fra Skogforskningen 3: 1-22.
Harding, S.; Nielsen, C.; Philipsen, H.; Vestergaard, S. and Martin, J. 2002. Ny viden om gråsnuder i pyntegrønt. Abstract, Skov- og Landskabskon-ferencen, 2002.
Higushi, T. Saika, T, Senda, S., Mizobata, T., Kawata, Y. and Nagai, J. 1997. Development of biorational pest control formulation against longicorn beetles using a fungus, Beauveria brongniartii
(Sacc.) Petch. Journal of Fermentation and Bioengineering 84: 236-243.
Hokkanen, H.M.T. and Hajek, A.E. (eds.) 2003. Environmental impacts of microbiological insecticides – need and methods for risk assessment. Progress in Biological Control. Kluwer Academic
Publishers, 269 pp.
Huai, W.X.; Guo, L.D.; He, W. 2003. Genetic diversity of an ectomycorrhizal fungus Tricholoma terreum in a Larix principisupprechtii stand assessed using random amplified polymorphic DNA.
Mycorrhiza 13: 265-270.
Inglis, D.G.; Goettel, M.S.; Butt, T.M. and Strasser, H. 2001. Use of Hyphomycete fungi for managing insect pests. In Butt, T.M.; Jackson, C.W. and Magan, N. (eds.): Fungi as biocontrol agents.
Progress, problems and potential. CABI Publishing, 23-69.
James, R.R. and Lighthardt, B. 1994. Susceptibility of the convergent lady beetle (Coleoptera: Coccinellidae) to four entomogenous fungi. Environmental Entomology 23: 190-192.
Jensen, J.E. and Skovgaard, I. (1995) Introduktion til SAS med statistiske anvendelser [Introduction to SAS with statistical application] (in Danish). Jordbrugsforlaget, Copenhagen, Denmark.
Jetter, K. and Paine, T.D. 2004. Consumer preferences and willingness to pay for biological control in the urban landscape. Biological Control (in press).
Jorge, S.; Pedroso, M.C.; Neale, D.B. and Brown, G. 2003. Genetic differentiation of Portuguese tea plants using RAPD markers.
Hortscience 38: 1191-1197
Kassa, A.; Zimmermann, G.; Stephan, D.; Vidal, S. 2002. Susceptibility of Sitophilus zeamais (Motsch.) (Coleoptera: Curculionidae) and Prostephanus truncates (Horn) (Coleoptera: Bostrichidae) to
entomopathogenic fungi from Ethiopia. Biocontrol Science and Technology 12: 727-736.
Kaaya, G.P.; Seshureddy, K.V.; Kokwaro, E.D. and Munyinyi, D.M. 1993. Pathogenicity of Beauveria bassiana, Metarhizium anisopliae and Serratia marcescens to the banana weevil cosmopolites
Sordidus. Biocontrol Science and Technology 3: 177-187.
Kéler, St. v. 1952. Über die Wachstumsprogression bei Pseudomenopon rowanae Kéler (Mallophaga). Beiträge der Entomologie 2: 113-119
Keller, S.; Christian, P. and Shah, P. 1999. Differential susceptibility of two Melolontha populations infections by the fungus Beauveria brongniartii. Biocontrol Scence and Technology 9: 441-446.
Keller, S.; David-Henri, A-I. and Schweizer, C. 2000. Insect pathogenic soil fungi from Melolontha melolontha control sites in the canton Thurgau. IOBC/WPRS Bulletin 23: 73-78.
Kershaw, M.J.; Moorhouse, E.R.; Bateman, R.; Reynolds, S.E.; Charnley, A.K. 1999. The role of destruxins in the pathogenicity of Metarhizium anisopliae for three species of insects. Journal of
Invertebrate Pathology 74: 213-223.
Kessler, P., and Nielsen, C. 2000. Standard protocol for Statistical Analysis of Bioassays in BIPESCO. 18 Months Report to the EU project 'BIPESCO' (FAIR6-CT98-4105). 12 pp.
Kirkeby-Thomsen, A., and Ravn, H.P. 1997. Skadedyr In Lundqvist, H.(ed.). Miljøvenlig Juletræsproduktion. En statusopgørelse. Pyntegrøntserien No. 2-1997. Danish Forest and Landscape Research
Institute, Hørsholm. 157 pp.
Kleine, R. 1911. Über die Verwandlung und Lebensweise des Strophosomus coryli. Fabr. von Bohutinsky. Entomologische Blätter 7: 183.
Lacey, L. A. 1997a. Manual of Techniques in Insect Pathology. Academic Press, San Diego, 409 pp.
Lacey, L. A. 1997b. Bacteria: Laboratory bioassay of bacteria against aquatic insects with emphasis on larvae of mosquito and black flies. In.: Lacey, L. A. (ed.) Manual of Techniques in Insect Pathology.
Academic Press, San Diego, 409 pp.
Lewis, K.G.; Ritland, K.; El-Kassaby, Y.A.; McLean, J.A.; Glaubitz, J. and Carlson, J.E. 2001. Randomly amplified polymorphic DNA reveals fine-scale genetic structure in Pissodes strobi
(Coleoptera: Curculionidae). Canadian Entomologist 133: 229-238.
Lacey, L.A. and Kaya, H.K. (eds.) 2000. Field manual of techniques in invertebrate pathology. Application and evaluation of pathogens for control of insects and other invertebrate pests. Kluwer Academic
Publishers, 911 pp.
LeOra Software 1987. POLO-PC a user's guide to Probit or Logit analysis. LeOra Software, Berkeley, California, USA.
Lingg, A.J and Donaldson, M.D. 1992. Biotic and abiotic factors affecting stability of Beauveria bassiana conidia in soil. Journal of Invertebrate Pathology 38: 191-200.
Lubeck, P.S.; Alekhina, I.A.; Lubeck, M.;Bulat, S.A 1998. UP-PCR genotyping and rDNA analysis of Ascochyta pisi Lib. Journal of Phytopathology 146: 51-55
Madeira, P.T.; Hale, R.E.; Center, T.D.; Buckingham, G.R.; Wineriter, S.A. and Purcell, M. 2001. Whether to release Oxyops vitiosa from a second Australian site onto Florida's melaleuca ? A
molecular approach. Biocontrol 46: 511-528.
Madsen, A.M.; Eilenberg, J.; Enkegaard, A.E.; Hendriksen, N.B.; Jensen, D.F.; Jespersen, J.B. and Larsen, J. (eds.). 2003. Abstracts: Conference on
Occupational Health Risks of Producing and Handling Organisms
for Biological Control of Pests in Agriculture. National Institute of Occupational Health, Copenhagen, Nov 27.
Mainz, H. 2003. Eksporten 2002. PS Nåledrys 45: 5-9.
Marchal, M. 1977. Fungi imperfecti isoles d'une population naturelle d'Otiorrhynchus sulcatus Fabr. (Col. Curculionidae). Revue de Zoologie Agricole et de Pathologie Vegetale 76: 101-108.
Milner, R.J.; Samson, P. and Morton, R. 2003. Persistence of conidia of Mehizium anisopliae in sugercane fields: effect of isolate and formulation on persistence over 3.5 years. Biocontrol Science and
Technology 13: 507-516.
Moorhouse, E.R.; Gillespie, A.T. and Charnley, A.K. 1993a. Application of Metarhizium anisopliae (Metsch.) conidia to control Otiorhynchus sulcatus (F.) (Coloptera, Curculionidae) larvae on
glasshouse pot plants. Annals of Applied Biology 122: 623-636.
Moorhouse, E.R.; Easterbrook, M.A.; Gillepsie, A.T. and Charnley, A.K. 1993b. Control of Otiorhynchus sulcatus (Fabricius) (Coleoptera, Curculionidae) larvae on a range of hardy ornamental
nursery stock species using the entomogenous fungus Metarhizium anisopliae. Biocontrol Science and Technology 3: 63-72.
Münster-Swendsen, M. 1989. Phenology and natural mortalities of the fir needleminer, Epinotia fraternata (Hw.) (Lepidoptera, Tortricidae). Entomologiske Meddelelser 57: 111-120.
Nankinga, C.M andMoore, D. 2002. Reduction of banana weevil populations using different formulations of the entomopathogenic fungus Beauveria bassiana. Biocontrol Science and Technology 10:
645-657.
Nielsen, C.; Sommer, C.; Eilenberg, J.; Hansen, K.S. and Humber, R.A. 2001. Characterization of aphid pathogenic species in the genus Pandora by PCR techniques and digital image analysis.
Mycologia 93: 864-874.
Notredame, C., Higgins, D. and Heringa, J. 2000. A novel method for multiple sequence alignments. Journal of Molecular Biology 302: 205-217.
Palm, E. 1996. Nordeuropas Snudebiller. 1. De kortsnudede arter (Coleoptera: Curculionidae) – med særligt henblik på den danske fauna (in Danish). Danmarks Dyreliv Bind 7. Apollo Books, Steenstrup,
356 pp.
Parry, W.H.1983. The overwintering of adult Strophosomus melanogrammus (Coleoptera: Curculionidae) in forest soils. In: Lebrun, P., André, H.M., de Medts, A., Grégoire-Wibo, C. and Wauthy,
G.(eds.): New Trends in Soil Biology. Proceedings of the VII.Intl. Colloquium of Soil Zoology. Louvain-la-neuve (Belgium). August 30-September 2, 1982. Imprimeur Dieu-Brichart,
Ottignies-Louvain-la-Neuve.
Parry, W.H.; Kelly, J.M. and McKillop, A.R. 1990.The role of nitrogen in the feeding strategy of Strophosomus melanogrammus (Forster) (Col., Curculionidae) in a mixed woodland habitat. Journal of
Applied Entomology 109: 367-376.
Peng, W.K.; Lin, H.C.; Chen, C.N. and Wang, C.H. 2002. DNA identification of two laboratory colonies of the weevils, Sitophilus oryzae (L.) and S. zeamais Motschulsky (Coleoptera: Curculionidae)
in Taiwan. Journal of Stored Products Research 39: 225-235.
Peros, J.P. and Berger, G. 2003. Genetic structure and variation in aggressiveness in European and Australian populations of the grapevine dieback fungus, Eutypa lata. European Journal of Plant
Pathology 109: 909-919.
Price, P.W. 1975. Insect Ecology. John Wiley and Sons, 514 pp.
Quintela, E.D. and McCoy, C.W. 1998. Synregistic effect of imidacloprid and two entomopathogenic fungi on the behaviour and survival of larvae of Diaprepes abbreviatus (Coleoptera: Curculionidae) in
soil. Journal of Economic Entomology 91: 110-122.
Rath, A. C., 1992. Metarhizium anisopliae for control of the Tasmanian pasture scarab Adoryphorus couloni. In: T.A. Jackson and T.R. Glare (eds), Use of Pathogens in Scarab Pest Management.
Intercept, Andover, 217-227.
Rath, A.C.; Bullard, G.K. and Allsopp, P.G. 1997. Persistence of Metarhizium anisopliae DAT F-001 in pasture soils for 7.5 years – implications for sustainable soil-pest management. In Rogers, D.J.
and Robertson, L.N. (eds.): Proceedings of the 3rd Brisbane Workshop on Soil Invertebrates, 78-80.
Ravn, H.P. 2000. Status for de vigtigste Skadevoldere- ind i det ny årtusind med og uden pesticider. Beretning, Skov- og Landskabskonferencen 2000: 98-104.
Reissig, J. 1928. Über S. coryli und obesus, die Schmerbauchrüssler. Forstwissenschaftliches Zentralblatt 50: 316-324.
Rivera, A.M.G. and Priest, F.G. 2003. Molecular typing of Bacillus thurin-giensis by RAPD-PCR. Systematic and Applied Microbiology 26: 254-261.
Robertson, J.L. and Preisler, H.K. 1992. Pesticide bioassays with Arthropods. CRC Press, Inc. London, 127 pp.
Roehrdanz, R.L. 2001. Genetic differentiation of southeastern boll weevil and thurberia weevvil populations of Anthronomus grandis (Coleoptera: Curculioinidae) using mitochondrial DNA. Annals of the
Entomology Society of America 94: 928-935.
Rozen , S. and Skaletsky, H.J. 2000. Primer 3 on the WWW for general users and for biologist programmers. In: Krawetz S., Misener S. (eds.): Bioinformatics Methods and Protocols: Methods in
Molecular Biology. Humana Press, Totowa, NJ, 365-386.
SAS Institute Inc. 1999. SAS/STAT User's Guide, Version 8, First edition, SAS Institute Inc., Cary, NC, USA.
Sambrook, J., Fritsch, E.F.and Maniatis, T. 1989. Molecular cloning: a laboratory manual. 2nd edn. Cold Spring Habor Laboratory, Cold Spring Habor.
Sandøe, P. 2003. How will the public perceive risks related to biocontrol ? In Madsen, A.M.; Eilenberg, J.; Enkegaard, A.E.; Hendriksen, N.B.; Jensen, D.F.; Jespersen, J.B. and Larsen, J. (eds.).
2003. Abstracts: Conference on
Occupational Health Risks of Producing and Handling Organisms
for Biological Control of Pests in Agriculture. National Institute of Occupational Health, Copenhagen, Nov 27, 7.
Schauermann, J. 1973. Zum Energiumsatz phytophager Insekten im Buchenwald. II. Oecologia 13: 313-350.
Scherf, H. 1964. Die Entwicklungsstadien der Mitteleuropäischen Curculioniden (Morphologie, Bionomie, Ökologie). Abhandlungen der Senckenbergischen naturforschenden Gesellschaft 506: 1-305.
Frankfurt.
Schindler, U. 1964. Zur Imaginalparasitierung forstlicher Curculionidae. Zeitschrift für angewannte Zoologie 51: 501-507.
Schindler, U. 1974. Adelognathi, Kurzrüssler. In: Schwenke, W.: Die Forstschädlinge Europas 2. band. Käfer. Verlag Paul Parey, Hamburg und Berlin.
Schulenburg, J. H. G, Hancock, J. M., Pagnamenta, A., Sloggett, J.J., Majerus, M. E. N. and Hurst, G.D.D. 2001. Extreme length and length variation in the first ribosomal internal transcribed spacer
of ladybird beetles (Co-leoptera: Coccinellidae). Molecular Biology and Evolution 18: 648-660.
Sedlag, U. and Kulicke, H. 1979. Zur Biologie, zum Schadauftreten und Bekämpfung des Dichtscuppigen Gtaurüsslers (Strophosomus capitatus; Coleoptera: Curculionidae) in Kiefernkulturen. Beiträge
für die Forstwirtschaft, Heft 2.
Sheppard, W.S., Arias, M.C. and Shimanuki, H. 1994. Determination of mitochondrial DNA haplotypes from sting remnants of the honeybee Apis mellifera (Hymenoptera:Apidae). Bulletin of
Entomological Research 84: 551-554.
Simon, C.; Frati, F.; Beckenbach, A.; Crespi, B.; Liu, H. and Flook, P. 1994. Evolution, Weighting, and Phylogenetic Utility of Mitocondrial gene sequences and a compilation of conserved polymerase
chain reaction primers. Annals of the Entomological Society of America 87: 651-701.
Skov, R. 2000. Skadevoldende snudebiller i danske bevoksninger - med særlig vægt på Strophosoma spp. Specialerapport. Afd. for Zoologi, Aarhus Universitet. 55 pp.
Steenberg, T.; Langer, V. and Esbjerg, P.1995. Entomopathogenic fungi in predatory beetles (Col.: Carabidae and Staphylinidae) from agricultural fields. Entomophaga 40: 77-85.
Southwood, T.R.E. 1980. Ecological methods with particular reference to the study of insect populations. Second edition, Chapman and Hall, 524 pp.
Szmidt, A. and Stachowiak, P. 1980. Strophosoma capitatum Deg. (Coleoptera, Curculionidae), occurrence of the beetles, food preference and damages. Prace Komisji Nauk Rolniczych i Komisji
Nauk Lesnych 50: 145-153.
The Danish Environmental Protection Agency 1998. Agreement on phasing out the use of plant protection products in public areas. Copenhagen, 3 November 1998. http://www.mst.dk/homepage/.
Thomsen, L. and Jensen, A.B. 2002. Application of nested-PCR techniques to resting spores from the Entomophthora muscae species complex: Implications for analysis of host pathogen population
interactions. Mycologia 94: 794-802.
Thorbek, P. 1998. Skadedyr i pyntegrøntproduktionen. Hvirvelløse skadedyr og deres naturlige fjender på Ædelgran. Speciale ved Københavns Universitet, Zoologisk Institut, Afdeling for
Populationsbiologi.
Thorbek, P. and Ravn, H.P. 1999. Strophosoma spp. Videnblad Pyntegrønt no. 5.5-3. Danish Forest and Landscape Research Institute, Hørsholm. 2 pp.
Throne, J. E., Weaver, D. K., Chew, V. and Baker, J. E. 1995. Probit analysis of correlated data: multiple observations over time at one concentration. Journal of Economic Entomology 88: 1510-1512.
Urban, J. 1999. Strophosoma melanogrammum Först. – A pest on young beech trees and on European mountain ash in the Zdar region (in Czech with English summary). Journal of Forestry Science 45:
64-80.
Wajnberg, E.; Scott, J.K. and Quimby, P.C. (eds.) 2001. Evaluatin indirect effects of biological control. CABI Publishing, 261 pp.
Vänninen, I.; Tyni-Juslin, J. and Hokkanen, H.M.T. 2000. Persistence of augmented Metarhizium anisopliae and Beauveria bassiana in Finnish agricultural soils. Biocontrol 45: 201-222.
Verkjeij, F.N.; Vanamelsvoort, P.A.M. and Smits, P.H. 1992. Control of the pea weevil (Sitona lineatus L.) (Col., Curculionidae) by the entomopathogenic fungus Metarhizium anisopliae in field
beans. Journal of applied Entomology 113: 183-193.
Vestergaard, S., Butt, T.M., Giillespie, A.T., Schreiter, G.and Eilenberg, J. 1995. Pathogenecity of the hyphomycete fungi Verticillium lecanii and Metarhizium anisopliae to the western flower thrips,
Frankliniella occidentalis. Biocontrol Science and Technology 5: 185-192.
Vestergaard, S. and Eilenberg, J. 1999. Lack of side effects of Metarhizium anisopliae to Western Flower Thrips (Frankliniella occidentalis) beneficials. Programme and Abstracts, Society for
Invertebrate Pathology, 32th Annual Meeting, Irvine, California, 23/8-27/8, 77.
Vestergaard, S. and Eilenberg, J. 2000. Persistence of released Metarhizium anisopliae in soil and prevalence in ground and rove beetles. IOBC/WPRS Bulletin 23: 181-186.
Vestergaard, S., Eilenberg, J. and Harding, S. 2000. Potential for microbial control of scarabs and weevils in Danish forestry. IOBC/WPRS Bulletin 23: 35-37.
Vestergaard, V.; Nielsen,C.; Harding, S. and Eilenberg, J. 2002. First field trials to control Melolontha melolontha with Beauveria brongniartii in Christmas trees in Denmark. IOBC/WPRS Bulletin 25:
51-58.
Vestergaard, S.; Cherry, A.; Keller, S. and Goettel, M. 2003. Safety of Hyphomycete fungi as microbial control agents. In: Assessment of environmental safety of biological insecticides (eds. Hokkanen,
H. and Hajek, A.) Kluwer Academic Publisher, 35-62.
Wagner, B., and Schaefer, M. 1987. Rüsselkäfer (Coleoptera: Curculionidae, Chrysomelidae) als Phytophage in einem Kalkbuchenwald. Verhandlungen der Gesellschaft für Ökologie 17: 273-255.
Weatherbee, A.A.; Bullock, R.C.; Panchal, T.D. and dang, P.M. 2003. Differentiation of Diaprepes abbreviatus and Pachnaeus litus (Coleoptera: Curculionidae) egg masses: PCR-Restiction
fragment-length polymorphism and species-specific PCR amplification of 188 rDNA products. Annals of the Entomological Society of America 95: 637-642.
Williams, J.G.K.; Kubelik, A.R.; Livak, K.J., Rfalski, J.A.; Tingey, S.V. 1990. DNA polymorphism amplified by arbitrary primers are useful as genetic markers. Nucleic Acids Research 18: 6531-6535.
Zeng, Q.Q., Hokkanen, H.M.T. and Menzler-Hokkanen, I. 2001. Field Evaluation of non-target impacts of Metarhizium anisopliae mycoinsecticide in oilseed rape ecosystem. Programme and
Abstracts, Society for Invertebrate Pathology, 34th Annual Meeting, Noordwij-kerhout, The Netherlands, 25/8-30/8, 51.
Zimmermann, G., 1993. The entomopathogenic fungus Metarhizium anisopliae and its potential as a biocontrol agent. Pesticide Science 37: 375-379.
| Top | | Front page |
Version 1.0 November 2004, © Danish Environmental Protection Agency
|