| Bottom | | Front page |
Evironmental project no. 986, 2005
Background Report on Pre-validation of an OECD Springtail Test Guideline
Contents
Summary and conclusions
Sammendrag og konklusioner
1 Introduction
2 Materials and methods
3 Results and Discussion
4 Conclusion
5 References
OECD Guidelines for the Testing of Chemicals
Proposal for a New Guideline
List of participants
15 countries and 26 laboratories):
Summary and conclusions
The aim of the present project was to develop a draft proposal for an OECD test guideline for long-term toxicity-testing of Collembola (see Annex A). The draft guideline was based on two existing
standardised test methods, one for Folsomia fimetaria (Wiles & Krogh, 1998) and one for Folsomia candida (ISO, 1999), which were combined and updated.
The test on F. candida employs an asexually reproducing species typically living in the organic matter of compost heaps or pot-plants, whereas the test on F. fimetaria employs a sexually reproducing
species living in garden and agricultural soils. Accordingly for the test on F. fimetaria the effect on sexual reproduction is obtained. This latter may be seen as a more ecologically relevant endpoint than
effects on asexual reproduction, as sexual reproduction is the most frequent reproductive strategy of soil dwelling organisms. In addition, effects on the sexual reproduction of F. fimetaria would include
effects caused by chemicals that act via a disturbance of the sex-determination system in arthropods. In the present project the possibility of including a simple test for effects on the sex-determination was
investigated, but this was not successful. Given distinct life strategies (and sizes) initial studies were performed investigating possible general sensitivity differences between the two species. The results indicate
a difference in chemical sensitivity both according to species and to life-stage, but the differences could only partly be explained by size-differences.
To prepare for a ring-test of the draft test guideline three compounds were tested fipronil, 3,5-dichlorophenol and boric acid. Future test concentration ranges were identified for these compounds.
Sammendrag og konklusioner
Formålet med projektet var at udvikle et udkast til en OECD test-guideline for langtids toksicitetstest af springhaler (se Annex A). Udkastet til test-guidelinen blev baseret på to eksisterende testmetoder, en
for Folsomia fimetaria (Wiles & Krogh, 1997) og en for Folsomia candida (ISO 1999), hvilke blev kombineret og opdateret.
Testen med F. candida baseres på en aseksuel reproducerende art, der typisk lever i organisk materiale som kompost eller potteplante muld, hvorimod testen med F. fimetaria benytter en seksuelt
reproducerende art, der lever i have- og landbrugsjorde. Deraf følger, at testen med F. fimetaria vil medtage effekter på den seksuelle reproduktion, hvilket synes at være et mere økologisk relevant mål,
idet seksuel reproduktion er den mest forekommende strategi for jordlevende organismer. Ydermere vil effekter på F. fimetarias seksuelle reproduktion inkludere effekter forårsaget af kemikalier, der
virker via en forstyrrelse af kønsbestemmelses-systemet hos leddyr. I det nuværende projekt blev det undersøgt, hvorvidt det var muligt at inkludere en simpel test for effekter på kønsbestemmelsen, men
dette lykkedes ikke. Givet de to forskellige livsstrategier (og størrelser) for de to arter blev det undersøgt, om der var en generel forskel i følsomheden imellem arterne. Resultaterne indikerede en forskel i
kemikaliefølsomheden som følge af arten og som følge af livsstadiet, men forskellen kunne delvist forklares ved hjælp af størrelsesforskellen.
For at forberede en ring-test til test-guidelineudkastet blev tre kemiske stoffer testet fipronil, 3,5-dichlorophenol og borsyre. Testkoncentrations områder til brug i ringtesten blev identificeret for disse stoffer.
1 Introduction
1.1 Aims
This report provides a background report for a draft OECD test guideline for long-term toxicity to Collembola, commonly known as springtails (see Annex A). This report aim at providing a background for
the draft guideline and to discuss relevant questions related to this guideline.
Collembolans are the most numerous and widely occurring insects in terrestrial ecosystems. This is the one of the main reasons for why they have been widely used as bioindicators and test organisms for
detecting the effects of environmental pollutants and different agricultural (especially pesticide) regimes on biodiversity in soils (e.g. Paoletti et al. 1991; Crossley et al. 1992; Filser 1995; Filser et al. 1995;
Frampton 1997; Paoletti and Bressan 1996). A variety of species have been employed in terrestrial ecotoxicological test. For the present draft OECD guideline Folsomia candida and Folsomia fimetaria
have been select as previous standard tests are available (for further discussion on this see "results and discussion").
A springtail test employing the species Folsomia candida (ISO, 19993) is already in use for pesticide evaluation within Europe and USA. It is used where Environmental Agencies require an evaluation of
the soil quality, for example when evaluating the potential problems related to spreading sewage-sludge or plant protection products on agricultural land. The test is well suited as a standard-test as the test
organisms are easily cultured, the test easily accomplished and the demand for equipment is minimal. The springtail is representative for arthropods that is characterised by an aerobiontic cuticle compared to
the soft-bodied semi-aquatic organisms viz. the earthworm test. On these grounds the springtail test is recommended for development in the OECD TG in OECD Monograph no. 11 (Detailed review Paper
on Aquatic Testing methods for Pesticides and Industrial Chemicals (Paris 1998) [1]). The method is also recommended by SETAC [2] and in the EU Guidance Document for risk assessment for the soil
environment for pesticides [3], biocides and industrial chemicals [4].
An alternative to the F. candida test is a standard test with the near relative Folsomia fimetaria (Wiles & Krogh, 1998). The two species differ in their habitat choice, F. candida resides primarily in
habitats rich in organic matter such as pot-plants and compost heaps, whereas F. fimetaria is common in agricultural soils. This make the toxicity effects estimated based on F. fimetaria more applicable to
agricultural land and nature areas. Further, Folsomia fimetaria has a sexual mode of reproduction, which is in contrast to F. candida consist of females only with a parthenogenetic (asexual) reproductive
mode. It is expected that when utilising a sexually reproducing species the results will have a broader application, since the majority of species are assumed to have sexual reproduction.
The two species can appear alike from a visual point-of-view, but they differ in size with F. candida being the larger. It is unknown whether this difference is important for their general sensitivity toward
chemicals. The literature is inconclusive on whether the two species differ in sensitivity. For example, Krogh (1995) exposed the two species to dimethoate under identical conditions and observed significant
(P<0.05) differences in the EC10 values with regard to reproduction. On the other hand, no significant differences were observed for the copper EC50 values for the two species when exposed under
identical conditions (Pedersen et al 1999). Form a pure theoretical point-of-view it could be argued that the two species are likely to differ in sensitivity as the surface-to-volume ratio [this is probably
important for toxicity of chemicals that have a passive uptake] is related to the size.
As F. fimetaria have a sexual mode of reproduction it may in theory be possible to identify chemicals with sex-determination effects. There are several ways in which to identify a compound that can
influence the sex-determination, these includes biochemical assays, morphological tests and sex-ratio tests (deFur et al 1999). Since, the mode-of-action is not known a test of sex-ratio of the juvenile
outcome seem the most appropriate test. In addition, a determination of the sex-ratio of the offspring, if successful, could be a simple addition to the overall test design of the draft guideline.
There is at present no clear evidence for which compounds that primarily influence sex-determination of invertebrates. The vertebrate sex hormones have been suggested, but this is uncertain. Testosterone
has been reported to have some androgenlike activity in some crustaceans (LeBlanc 1999) and endogenous concentrations of testosterone and other vertebrate-type androgens have been reported in
Daphnia magna and Neomysis integer (LeBlanc and MacLachlan 2000, Verslycke et al 2002, 2004). Estradiol has been recorded in the silk moth Bombyx mori (Keshan and Ray 2001). However,
neither estradiol nor testosterone affected the sex-ratio in exposed Daphnia magna (Kashian and Dodson 2004). Nevertheless, two chemicals, methyl-testosterone and ethynyl-estradiol, have been
suggested as reference chemicals for evaluating potential sex-endocrine-disrupting compounds in invertebrates by the participants of "Workshop on Endocrine Disruption in Invertebrates: Endocrinology,
Testing, and Assessment (EDIETA)", held in The Netherlands in 1997 (Ingersoll et al 1999).
1.1 Aims
To support the draft guideline two main activities were pursued.
- As the two species differ with respect to ecology, reproduction biology and physiology (size). The importance of this in relation to the guideline will be reviewed and shortly discussed. These issues will
partly be pursued via literature review and through laboratory test, with emphasis on possible chemical sensitivity differences due to age/size differences between the two species.
- In connection with the test-guideline and a future ring-test it will be necessary to have positive control compounds to ensure the stability of the test-system. Hence, it is an aim to identify the future test
concentration ranges for potential positive controls. The selection of the three compounds will be based on previous ring-test programmes.
Footnotes
[1] Danish publication (sponsored by DG ENV, Danish EPA 14th Office and the Danish EPA Pesticide Research Programme)
[2] Cf. ”Test Methods to determine hazards of Sparing Soluble Metal Compounds in Soils” 2002
[3] Cf. EU Guidance Document on terrestrial Ecotoxicology Under Council Directive 91/414/EEC, SANCO/10329/2002 rev.2 final
[4] jfr. EU’s rev. TGD: ”Technical Guidance Document on Risk Assessment in support of Commission Directive 93/67/EEC on Risk Assessment for new notified substances and Commission Regulation (EC) No. 1488/94 on Risk Assessment for Existing Substances and Directive 98/8/EC of the European Parliament and of the Council Concerning the placing of Biocidal Products on the Market” (May
2002)
2 Materials and methods
2.1 Background - species identification and comparison
2.1.1 Literature search - Ecology and biology of species
2.2 Sensitivity comparison
2.2.1 General sensitivity comparison
2.2.2 Laboratory experiments - Comparison of age/size and toxicity
2.2.3 Sex-ratio effects on offspring
2.3 Identifying test concentration ranges for ring-testing
2.3.1 Test conditions
According to the aims the studies were divided in two parts. The first part deals with studies on the importance of ecological, biological and physiological for choice of species. These issues will be dealt with
though specific aspects (see 2.1). Second, to identify test-concentration ranges for future positive control compounds three compounds will be tested based on previous ring-test programmes and test
guidelines (see 2.2).
2.1 Background - species identification and comparison
2.1.1 Literature search - Ecology and biology of species
Based on a literature search and experience a short description of the ecology and biology was performed. Emphasis was put on background for choice of the species included in the guideline (see 3.1).
2.2 Sensitivity comparison
The two selected species differ in various aspects and may hence in theory differ in sensitivity to chemical toxicity. This issue was studied via two approaches.
2.2.1 General sensitivity comparison
To study the general chemical sensitivity of the species a literature survey was performed. In this review two compounds were selected for further analysis. The first compound was an choline-esterase
inhibiting pesticide, dimethoate. For this compound there was already considerable knowledge available. The second compound was a heavy metal, copper, with a more broad mode-of-action. For this
group relevant information is also available in the published literature. Hence, in the present report a comparison was made between the two species, F. fimetaria and F. candida, with regard to the toxicity
of Copper and Dimethoate. The data has been obtained via searches in international databases such as WOS, BIOSIS, SCIC and ECOTOX. Only original literature has been cited (see 3.2.1).
2.2.2 Laboratory experiments - Comparison of age/size and toxicity
One of the notable differences between the two species is the size difference. In theory the two species may differ in sensitivity to chemicals due to such a size difference, i.e. the surface
area compared to volume is larger for smaller animals (F. fimetaria), which will influence uptake chemicals taken up via outer cuticle.
In accordance, an experiment was set up to study whether the toxicity differed between the two species and to see if this could be attributed to size differences. The experiment was based on exposing a
range of age-classes (hence also size classes) to one concentration of a chemical and observing differences in mortality between the age-classes (see Table 1). Due to the limited time-span of the project it
was decided to use a compound, dimethoate, already tested for both species and to use the LC50 for Folsomia fimetaria reported by Krogh (1995) (see 3.2.2).
2.2.2.1 Test compound
- Dimethoate (purity: 400 g L-1 Cheminova Agro A/S) (CAS no. 60515)
Table 1. Age of animals in size classes employed for both Folsomia fimetaria and Folsomia candida.
Size class |
1 |
2 |
3 |
4 |
5 |
6 |
7 |
8 |
9 |
Age/days |
0-2 |
3-5 |
6-8 |
9-11 |
12-14 |
15-17 |
18-20 |
21-23 |
24-26 |
Hence, the mortality after exposure for one week to dimethoate 2 mg kg-1 and control was tested for 9 size/age-classes of Folsomia fimetaria and Folsomia candida in 5% OECD soil. They were fed 15
mg Baker's yeast in the beginning of the experiment. Ten individuals were added to each replicate container and extracted after 1 week. There were 2 replicates per species and size-class and treatment.
2.2.2.2 Test soil, counting and statistics
See section 2.2.1.3-2.2.1-5
2.2.3 Sex-ratio effects on offspring
Experiments were conducted to see if it was possible to include offspring sex-ratio as an effect parameter in the draft guideline. To study this adult Folsomia fimetaria were exposed for 42 days to soil
contaminated with two potential sex-ratio disrupting compounds, ethynyl-estradiol and methyl-testosterone. The experiment was continued for a total of 42 days to allow the first clutch of the juveniles to
become adult (Table 2). Allowing the first clutch of the juveniles to become adult made it potentially possible to identify the sexes on the offspring. The maximum concentrations used were 1000 mg a.s. kg-1
soil corresponding to the limit-testing for plant protection products (see 3.2.3).
Since the compounds may bind to the soil particles and hence not be available to the organism a water- exposure test was conducted to ensure exposure. Hence, adult Collembola were exposed for 48
hours to 12 mg testosterone L-1 or 2 mg estradiol L-1 after which the adults were allowed to produce eggs over a period of 7 days. The hatched juveniles were allowed to growth for 23 days prior to size
measuring.
Table 2. Test conditions of the sex-determination toxicity tests with Folsomia candida.
Test species |
Folsomia fimetaria |
Life stage |
23-26 days (synchronised) |
Exposure duration |
42 days |
Test volume |
30 g wet weight |
Temperature |
20°C |
Light cycle |
16:8 light/dark |
Feeding |
every 14 days |
Initial organisms per replicates |
20 |
2.2.3.1 Test compounds
- Methyl-testosterone (purity: 97%, Sigma Aldrich) (CAS no. 58-18-4)
- 17-βEthynyl-testosterone (purity: 85%, Sigma Aldrich) (CAS no. 57-63-6)
2.2.3.2 Test soil, counting and statistics
See section 2.2.1.3-2.2.1-5
2.3 Identifying test concentration ranges for ring-testing
Experiments were performed in order to identify the test concentrations used in a future ring-test programme and to identify test concentrations used for a positive control. The experiments included three
compounds fipronil, 3,5-dichlorophenol and boric acid. The experiments were performed according to conditions stated in table 3.
2.3.1 Test conditions
2.3.1.1 Test organisms
The Collembolans Folsomia fimetaria and Folsomia candida (Collembola, Isotomidae) were used as test organisms. For fipronil and 3,5-dichlorophenol tests were performed with F. fimetaria and for
boric acid tests were performed with both species.
Cultures of these species were kept on moist substrate of plaster of Paris and pulverised activated charcoal (as described in the guideline). Granulated yeast was added weekly as a food source. The cultures
were maintained at the Department of Terrestrial Ecology, National Environmental Research Institute.
Table 3: Test conditions of the range determination toxicity tests with Folsomia candida and Folsomia fimetaria.
Test species |
Folsomia candida |
Folsomia fimetaria |
Life stage |
10-12 days (synchronised) |
23-26 days (synchronised) |
Exposure duration |
14/21 days |
14/21 days |
Test volume |
30 g wet weight |
30 g wet weight |
Temperature |
20°C |
20°C |
Light cycle |
16:8 light/dark |
16:8 light/dark |
Feeding |
every 14 days |
every 14 days |
Initial organisms per replicates |
10 |
20 |
2.3.1.2 Test compounds
All compound used were of analytic grade and obtained from Sigma-Aldrich (Germany) or Cheminova.
- Boric Acid (purity: 100%, Sigma Aldrich) (CAS no. 10043-35-3)
- Fipronil (purity: 97%, Sigma Aldrich) (CAS no. 1200068-37-3)
- 3,5-Dichlorophenol (DCP) (purity: 97%, Sigma Aldrich) (CAS no. 591-35-5)
2.3.1.3 Test soil
The test soil was an artificial mixed soil according to OECD guideline. Compared to the "standard" OECD soil, which has a 10% organic matter content, it was chosen in the present test to use a 5% organic
matter soil. This latter choice was made to mimic field condition and reduce binding of test chemicals. Soil composition: Organic matter - 5%; clay - 21% and sand - 74% [the clay and sand content has been
changed, compared to the original OECD soil, to keep the relative composition the same].
2.3.1.4 Counting
At the end of each experiment the animals were heat-extracted and counted with a computerised system employing a digital image processing (DIP) approach (Krogh et al. 1998). The guideline alternatively
suggests to extract the animals by flotation and to manually count the organisms floating on the surface. It was chosen in this context to use the heat extraction and the automated counting since this is
considered more precise than the flotation and manually counting.
2.3.1.5 Statistics
the data were checked for normality using a 2 test, and for homogeneity of variance by Barlett's test. Effect concentrations, EC10 and EC50, and confidence intervals were estimated by fitting a logistic
model to the data (Lacey and Mallet 1991). The formulae were reparametrized by incorporation of the EC10 and EC50 into the equation (Krogh 1995). The estimates with a 95% confidence interval were
performed with the SAS procedure PROC NLIN (Enterprise Guide 1.3)
3 Results and Discussion
3.1 Background
3.1.1 Ecology and Biology of Species
3.1.2 Ecotoxicology
3.1.3 Species inclusion in draft guideline
3.2 Sensitivity of species
3.2.1 Ecotoxicology - General sensitivity differences between the two species
3.2.2 Age- and size-toxicity relationships using dimethoate
3.2.3 Additional differences: Sex-ratio of off-spring
3.3 Test concentration ranges of possible ring-test compounds
3.3.1 Fipronil
3.3.2 3,5-dichlorophenol
3.3.3 Boric acid
3.3.4 Proposed test ranges for a ring-test
3.1 Background
3.1.1 Ecology and Biology of Species
The following summary is based on the chapter written by Wiles and Krogh (1998). The Collembola, commonly known as springtails, are the most numerous and widely occurring insects in terrestrial
ecosystems. This is one of the main reasons why they have been widely used as bioindicators for detecting the effects of environmental pollutants and different agricultural (especially pesticide) regimes on
biodiversity in soils (e.g. Paoletti et al. 1991; Crossley et al. 1992; Filser 1995; Filser et al. 1995; Frampton 1997; Paoletti and Bressan 1996, Fountain and Hopkin 2004 a, b). A wealth of literature exists
concerning the systematics, evolution, physiology, behaviour and ecology of these organisms (see Hale 1967; Wallwork 1970; Fjellberg 1985; Eisenbeis and Wichard 1987; Greenslade 1991; Christiansen
1990; Curry 1994 and Hopkin 1997).
Population densities of Collembola commonly reach 105 m-2 (e.g. Burges and Raw 1967; Peterson and Luxton 1982) in soil and leaf litter layers in many terrestrial ecosystems. Because of their small size
however, adults typically measure 0.5 - 5 mm long, their contribution to total soil animal biomass and respiration is low, estimated at being between 1 and 5% (see Petersen 1994). Their most important role
may therefore be as potential regulators of processes through microbivory and microfauna predation. Springtails are prey animals for a wide variety of endogeic and epigeic invertebrates, such as mites,
centipedes, spiders and carabid and rove beetles. The role of Collembola in humus formation is not well known, but it is commonly accepted that they may contribute significantly to the decomposition
processes on acidic stands, where earthworms and diplopods are absent.
3.1.2 Ecotoxicology
Collembola possess many attributes of the ideal experimental organism for ecotoxicological research. They are ecologically important, widely distributed, often highly abundant, easily sampled in the field, can
be cultured or maintained in the laboratory and have a relatively rapid life-cycle with a high reproductive rate (Spahr 1981). They lend themselves to studies over a wide range of spatio-temporal scales
including field monitoring of population trends (e.g. Filser 1995), field bioassays (e.g. Wiles and Frampton 1996), meso- and microcosm studies (e.g. Parmelee et al. 1993) and a wide variety of laboratory
investigations (e.g. Crommentuijn et al. 1993; Krogh 1995, Scott-Fordsmand et al 2000; van Gestel and Koolhaas 2004, da Luz et al 2004). They have been used in studies to investigate the environmental
effects of a wide range of environmental contaminants.
One of the earliest laboratory studies was undertaken by Sheals (1956) who studied effects of organochlorine compounds on microarthropod communities and used supplementary tests with filter paper to
screen for species differences in susceptibility to DDT. In a later study Scopes and Lichtenstein (1967) used 6 week old F. fimetaria in an acute test, also using exposure through filter paper. Their results
showed that the LD50 for adults was greater than twice the LD50 for juveniles, a fact that has since been used to explain interspecific differences between collembolan species of different sizes. In 1972
Thompson and Gore described an acute test on filter paper and in soil for a sexual population of F. candida. They were one of the first authors to promote the use of F. candida as a test species for
laboratory bioassays of pesticides. A large number of laboratory studies with Collembola followed in the 1970's, 80's and early 90's. In these studies a range of ten to fifteen different species of Collembola
were used, of which four species have been by far the most common; Folsomia candida, Folsomia fimetaria, Onychiurus armatus (Protaphorura armata), Orchesella cincta. Some of the first
standard acute toxicity tests with Collembola were suggested by Huang (1992) and Kiss and Bakonyi (1992) and more recently by Houx et al. (1996).
The first initiative to develop a standard sub-lethal test was taken at the Institute for Chemical Examination of the German Federal Biological Research Centre for Agriculture and Forestry. A description of
the background for the development of the resulting draft test method of sub-lethal testing with F. candida has been given by Riepert and Kula (1996). The development of a test with F. fimetaria was
started in Denmark in 1990 in a project on side-effects of pesticides funded by the Danish Ministry of the Environment (Løkke 1995). The choice of F. fimetaria was based on its wide distribution in
Danish agricultural soil and the ease with which it can be cultured in the laboratory (Petersen and Gjelstrup 1995). The procedures for culturing and conducting tests with F. fimetaria were described in
Krogh and Pedersen (1995) and Krogh (1995). Based on the above consideration these two species, F. fimetaria and F. candida, were chosen for the draft OECD guideline.
3.1.3 Species inclusion in draft guideline
The draft OECD guideline proposal includes a choice between two species (see Annex A). The most prominent reason for choosing a particular species for the toxicity test is the reason of relevance to the
compartment or life-characteristic which this toxicity study is supposed to represent, e.g. use a species present in agricultural soils when the test is used for agricultural risk assessment.
F. fimetaria is widely distributed and common in several soil types ranging from sandy to loamy soils and from mull to mor soils. It has been recorded in agricultural soils in Denmark (Christensen et al.
1987; Krogh 1994; Petersen and Gjelstrup 1995), Sweden (Andrén and Lagerlöf 1983), Finland (Huldén 1984), and Germany (Heimann-Detlefsen et al. 1994). It has an omnivorous feeding habit,
including fungal hyphae, bacteria, protozoa and detritus in its food. It interacts through grazing with infections of plant pathogenic fungi (Ulber 1983) and may influence mychorrhiza, as is known to be the
case for F. candida.
Folsomia fimetaria has a sexual mode of reproduction, whereas F. candida consists of females only with a parthenogenetic (asexual) reproductive mode. It is expected that when utilising a sexually
reproducing species the results will have a broader application, since the majority of species are assumed to have sexual reproduction.
F. candida is widely distributed throughout Europe. Although it is not common in most natural soils, it often occurs in very high numbers in humus rich sites. It is a blind, unpigmented collembolan which
reproduces parthenogenetically. It has a well developed furca (spring organ) and an active running movement and springs readily if disturbed.
3.2 Sensitivity of species
3.2.1 Ecotoxicology - General sensitivity differences between the two species
When deciding which of the two species have to be tested a relevant question is whether one of the two species proposed in the guideline can be assumed generally more sensitive than the other species.
There has hitherto been few studies dealing with differences in sensitivity between F. fimetaria and F. candida, and it is unknown whether this difference is important for their general sensitivity toward
chemicals. To study this a literature was performed and an array of experiments performed (see also 3.2.2).
With regard to the literature study two compounds were selected, i.e. the specific acting dimethoate (acetylcholinesterase inhibitor) and the toxicologically more broad acting metal Copper. Based on the
literature data for the two compounds no differences in sensitivity between the two species could be detected (see Table 4).
For dimethoate the mean EC50 for F. fimetaria was 1.8 [±1.1] dimethoate kg-1 and for F. candida it was 4.0 [±2.6] dimethoate kg-1, but a t-test showed no significant difference (P>0.05). As observed the means were more than 2-fold different, although not significant, which indicate a possible trend in F. fimetaria being more sensitive. This is in line with the observation by Krogh (1995) who exposed the two species under identical conditions and observed significant (P<0.05) differences in the EC10 values with regard to reproduction. They observed that for F. fimetaria the EC50 (reproduction) was 0.3 [0.14-0.47, 95% C.I.] mg kg-1 soil and for F. candida the EC50 (reproduction) was 0.5 [0.43-0.57, 95% C.I.] mg kg-1 soil. Significant differences were also observed on the mortality level, with F. fimetaria being the most sensitive. However, curiously the current laboratory data (see Fig 1) indicate that F. candida is more sensitive than F. fimetaria on a mortality level.
Studying the pooled literature data (Table 3) in detail reveals that much of the variation between the different reporting can probably be explained by differences in experimental conditions, such as
differences in the soil organic matter content. Differences in the exposure conditions, such as variations in soil type, may render the "bioavailable" fraction different under such conditions. To eliminate
differences due to exposure condition (especially soil type) the soil-water concentration could be calculated for KOW (Martikainen and Krogh 1999). Calculating the soil-water concentration by the
equation reported by Martikainen and Krogh (1995) it is observed that the ECx values are relatively closer for the two species, when expressed on mg dimethoate L-1 soil water (see Table 4) than when
based on soil concentrations.
Table 4. The EC50 values (reproduction) for Copper and dimethoate exposure of Folsomia fimetaria and Folsomia candida based on studies reported in the literature and recalculated to soil
water concentrations after Martikainen and Krogh 1999).
REFERENCE |
SPECIES |
COMPOUND |
EC50-soil (mg kg-1) |
Average (stdev) |
EC50-soil-water (mg L-1) |
Average (stdev) |
Sandifer and Hopkin (1996) |
Folsomia candida |
Copper |
700 |
|
|
|
Sandifer and Hopkin (1996) |
Folsomia candida |
Copper |
710 |
|
|
|
Sandifer and Hopkin (1996) |
Folsomia candida |
Copper |
1480 |
|
|
|
Sandifer and Hopkin (1997) |
Folsomia candida |
Copper |
700 |
|
|
|
Sandifer and Hopkin (1997) |
Folsomia candida |
Copper |
640 |
|
|
|
Pedersen et al (2000) |
Folsomia candida |
Copper |
657 |
|
|
|
Herbert et al (2004) |
Folsomia candida |
Copper |
813 |
|
|
|
Scott-Fordsmand et al (1997) |
Folsomia fimetaria |
Copper |
129 |
|
|
|
Pedersen and van Gestel (2001) |
Folsomia fimetaria |
Copper |
1244 |
|
|
|
Pedersen et al (2000) |
Folsomia fimetaria |
Copper |
519 |
|
|
|
Scott-Fordsmand et al (2000) |
Folsomia fimetaria |
Copper |
994 |
|
|
|
Martikainen (1996) |
Folsomia candida |
Dimethoate |
6.3 |
|
7,5 |
|
Martikainen (1996) |
Folsomia candida |
Dimethoate |
5.5 |
|
8,6 |
|
Martikainen (1996) |
Folsomia candida |
Dimethoate |
3.8 |
|
4,4 |
|
Krogh (1995) |
Folsomia candida |
Dimethoate |
0.5 |
4.0(±2.6) |
2,4 |
5.7(±2.8) |
Martikainen and Krogh (1999) |
Folsomia fimetaria |
Dimethoate |
0.8 |
|
3,7 |
|
Martikainen and Krogh (1999) |
Folsomia fimetaria |
Dimethoate |
1.7 |
|
4,8 |
|
Martikainen and Krogh (1999) |
Folsomia fimetaria |
Dimethoate |
3.2 |
|
5,2 |
|
Martikainen and Krogh (1999) |
Folsomia fimetaria |
Dimethoate |
2.5 |
|
4,6 |
|
Martikainen and Krogh (1999) |
Folsomia fimetaria |
Dimethoate |
2 |
|
6,0 |
|
Krogh (1995) |
Folsomia fimetaria |
Dimethoate |
0.3 |
1.8(±1.1) |
1,4 |
4.2(±1.5) |
For Cu the t-test comparison between the two species also exhibited no difference between the means with regard to reproduction (P>0.05). The mean for F. fimetaria was 722 [±496] Cu kg-1 and F.
candida was 814 [±299] Cu kg-1. This is in line with observations by Pedersen et al (1999) who observed no differences in the copper EC50 values for the two species when exposed under identical
conditions. As for dimethoate soil type models could be considered resolving the differences between the soil types, for example the biological ligand-model (BLM). The latter conversion was not performed
as the models are presently under development and analysis of the experiments showed that for example temperature and pH may be as important for toxicity as soil type (Sandifer and Hopkin 1996, 1997).
In conclusion no difference in toxicity between the two species could be identified, but a possible difference in toxicity may be blurred by confounding factors.
3.2.2 Age- and size-toxicity relationships using dimethoate
Folsomia fimetaria and F. candida can appear quite alike from a visual point-of-view, but they differ in size with F. candida being the larger. From a pure theoretical point-of-view it could be argued that
the two species are likely to differ in sensitivity as the surface-to-volume ratio is related to the size. Such sensitivity differences could be very relevant when choosing which species to test.
Based on an experimental study (see 2.1.2.2) growth of F. candida was faster than F. fimetaria as would be expected from previous knowledge of this species (data not shown). The toxicity decreased
with age with juveniles being most sensitive and it decreased faster for juvenile F. fimetaria than for F. candida (Fig 1). Furthermore, there is a clear indication of increased sensitivity of the reproductive
instars about age 15 and 22 days (every second instar is reproductive and occurs approx. weekly). Thus, juvenile F. fimetaria was the least sensitive to dimethoate, which is different from a previous
studying showing adult F. fimetaria to be the most sensitivity (Krogh 1995). Hence, it seems that the sensitivity is life-stage dependent as confirmed on a refined level in this study (Fig 1). In fact it is seen
that both species have very contrasting sensitivities in the days around the age for which they are selected in the draft OECD test. For example, in the F. candida test the start age should be 10-12 days, but
if the organisms tend to rather be 9-11 days than 12-14 days the may change the sensitivity two fold (see Fig 1). It is important to realise such small differences may not only be due to a selection based on
number of days (age) for the organisms, but a slight increase or drop in temperature may also cause the organisms to be in a different life-stage at the start of the test. In fact it is seen that both species have
very contrasting sensitivities around the age for with they are selected for the OECD test. For example, in the F. candida test the start age should be 10-12 days, but if the organisms tend to be 9-11 days
rather than 12-14 days this may increase the sensitivity two fold (see Fig 1). It is important to realise such small differences may not only be due to a selection based on number of days (age) for the
organisms, but a slight increase or drop in temperature may also cause the organisms to be in a different life-stage.
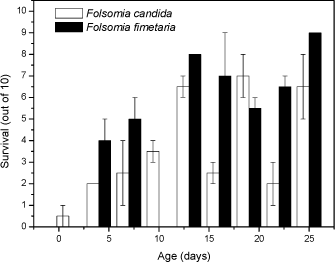
Fig 1. Survival (out of 10, mean ± standard error) of nine age-/size-classes for Folsomia candida and Folsomia fimetaria exposed for 7 days to dimethoate, 2 mg kg-1 in 5% OECD soil.
In conclusion, difference in toxicity between the species could be detected, and a pronounce life-stage toxicity dependency was present for both species.
3.2.3 Additional differences: Sex-ratio of off-spring
Given that F. fimetaria is a sexually reproducing species it is possible that compounds which introduces changes in the sex-ratio of the offspring can be identified when using this species. Measurements of
the offspring sex-ratio, if successful, would be a simple addition to, and involve a minor change of, the overall test design of the general guideline for long-term Collembola toxicity tests. Hence, based on
these considerations it was decided to attempt the sex-ratio approach.
The simplest way, and the approach taken, to identify a bias in the offspring sex-ratio was to extend the exposure period ensuring that the offspring would be of sufficient age (19-23 days) for sex
identification. Given the excessive man-power that would be required if each individual should be sexed under the microscope it was decided to use the size characteristics to class animals into sexes, this
latter could be done by employing the data produced by the digital image processing counting (see Fig 2). In performing this it is necessary to have an synchronous culture partly enabling the addition for
females and males at the beginning of the experiment, partly to ensure synchronised clutches and thus avoiding overlap of size classes. An overlap in size-classes will make sex-determination extremely
difficult by the termination of the experiment. It was decided to extend the exposure period to 42 days, which would allow for the first clutch to become adult, hence allowing for sex-ratio determination of
this.
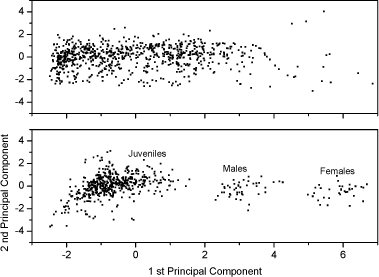
Fig 2. PCA plot of the first and second principal component scores of the original 5 dimension data: area, length, width, slimness and grey density. Lower Fig: three Folsomia fimetaria clusters can be identified: females, males and juveniles following exposure to copper (21, days of exposure, Scott-Fordsmand et al 1997). Upper Fig: No clusters can be identified following exposure to testosterone (42 days of exposure).
Two chemicals, methyl-testosterone and ethynyl-estradiol, are suggested as reference chemicals for evaluating potential endocrine-disrupting compounds in invertebrates (Ingersoll et al 1999). There is at
present no clear evidence for whether these compounds are involved in the sex-determination of invertebrates. Testosterone has been reported to have some androgen-like activity in some crustaceans
LeBlanc (1999) and endogenous concentrations of testosterone and other vertebrate-type androgens have been reported in Daphnia magna and Neomysis integer (LeBlanc and MacLachlan 2000,
Verslycke et al 2002, 2004). Estradiol has been recorded in the silk moth Bombyx mori (Keshan and Ray 2001). However, neither estradiol nor testosterone affected the sex-ratio in exposed Daphnia
magna (Kashian and Dodson 2004). No studies have previously been reported for Collembola.
Upon counting and sizing the offspring from the conducted soil-tests it was realised that with the employed approach it was not possible to identify the sex-ratio of the offspring. The problem seemed to be
that the juvenile growth was not sufficient synchronous under the present conditions and the individual clutches overlapped, hence a "small" male from the first clutch had the same size as a "large" female from
the second clutch (see Fig 2). It could then have been resolved to identifying the sex of the individual animals, but as the tests resulted in more than 18,000 animals per test this would have been an almost
impossible task. The present result indicates that the sex-ratio identification is not applicable with the present approach (see Fig 2). Other approaches may be considered in the future.
In fact another approach was partly followed using short-term exposure to an aquatic solution. Based on aquatic solutions adult Collembola were exposed for 24 hours to 12 mg testosterone L-1 or 2,0 mg
estradiol L-1 after which the adults were allowed to produce eggs over a period of 7 days. The hatched juveniles were allowed to growth for 23 days prior to size measuring. No bias in the sex-ratio could
be identified (Fig 3). No comparable literature data was available.
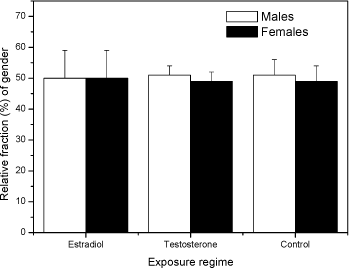
Fig 3 Relative fraction of gender Folsomia fimetaria following juvenile exposed to water, estradiol (12 mg L-1) and testosterone (2 mg L-1) following exposure of parent generation for 48 hours in an aqueous solution. Columns represent mean ± standard deviation.
3.3 Test concentration ranges of possible ring-test compounds
In connection to the test-guideline and a ring-test programme "standardized" test compounds should be available. Such compounds can be selected on a wide range of criteria, such as how non-toxic it is to humans, how easy it is to store etc. In the present project three compounds were tested, fipronil, 3,5-Dichlorophenol and boric acid. The three compounds were selected on basis of the inclusion in similar test programmes. Two of these compounds, fipronil and 3,5-DCP are currently used in the Copepod ring-test and included here upon the request from the Danish Environmental Protection Agency. Boric acid is suggested as positive control in soil test in North America. Previously, dimethoate has been suggested as a positive control in Collembola tests, but considering that this compound may be taken off the marked it is suggested to avoid using this.
As the above sensitivity studies indicated that the difference between the two species would be less than what is important for determining future test ranges, it was decided to test two of the compounds on
F. fimetaria only. In other words, the F. fimetaria test ranges is supposed also to cover the F. candida test ranges. For boric acid both species were tested, confirming that the test range covering F.
fimetaria also covered F. candida.
3.3.1 Fipronil
Fipronil has been suggested as a positive control in the Copepod ring-test programme. It is an insecticide, mainly used on pets for flea elimination (for a detailed review on this see Tingle et al 2003). As such
it should be highly toxic to arthropods, as also confirmed with the present results. For F. fimetaria exposed through the soil fipronil had EC10 and EC50 (based on nominal concentrations) values of 0.58
mg fipronil kg-1 and 1.89 mg fipronil kg-1, respectively. Based on these tests, the ring-test test ranges should be 0-10 mg fipronil kg-1 (see Fig 4).
Many data are available with regard to effects of fipronil on invertebrates, but few with soil exposed organisms. Soil toxicity data were only available for earthworms showing LC50 values above 8550 mg
kg-1 (Mostert et al 2002). As fipronil is an insecticide it is of little surprise that it is relatively non-toxic to earthworms. No soil toxicity data were found for Collembolans.
In conclusion: Based on these tests, the ring-test test ranges should be 0-15 mg kg-1 for fipronil.
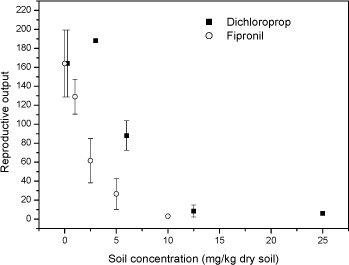
Fig 4. Number of juveniles (mean ± standard deviation) Folsomia fimetaria, following exposure of adult Collembola to fipronil or 3,5-chlorophenol for 21 days.
3.3.2 3,5-dichlorophenol
3,5-Dichlorophenol (3,5-DCP) has been suggested as a positive control in the Copepod ring-test programme. For F. fimetaria exposed through the soil 3,5-DCP had EC10 and EC50 (based on nominal
concentrations) values of 3.07 mg 3,5-DCP kg-1 and 5.53 mg 3,5-DCP kg-1, respectively (see Fig 5). Many data are available with regard to chlorophenol effects on invertebrates, but no 3,5-DCP
toxicity data for soil organisms were available. For F. candida Weigmann et al (1985) reported an LC50 value (24 hr exposure) for PCP at 32 ppm. Being weak acids, chlorophenol dissociate at a given
pH in accordance with their pKa values. Hence, it can be inferred that the solubility alters much with environmental changes in pH if the pKa is close to the environmental pH. The pKa of 3,5-DCP is 8.54
indicating that at a soil pH around 6 (as in the present test) more than 99% is on the non-ionized state.
In conclusion: Based on these tests, the ring-test test ranges should be 0-15 mg 3,5-DCP kg-1 using the reproduction test.
3.3.3 Boric acid
Boric acid is suggested as positive control in North America and has been used in many plant toxicity studies. The toxicity of boric acid was studied for both species, first in a short-term test (F. fimetaria)
and second in a reproduction study (F. fimetaria and F. candida). According to the guideline the two test should last 21 and 28 days respectively. It was, however, chosen to use a 21-day exposure for
both species in order to be able to compare the sensitivity between the two species.
The present studies revealed LC10 and LC50 values of 394 mg boric acid kg-1 and 648 mg boric acid kg-1 for F. fimetaria in a 14-day experiment (see table 5). Based on this it was decided to run the
reproduction test with concentrations ranging for 0 to 1000 mg boric acid kg-1 for both species. In the reproduction test, which lasted 7 days more than the preliminary test, the mortality proved higher
resulting in lower LCx values. The LC10 was below 200 mg boric acid kg-1 for both species. Applying dose-response modelling the EC10 and EC50 values were 24 and 117 mg boric acid kg-1 for F.
fimetaria and 22 and 111 mg boric acid kg-1 for F. candida. The latter ECx values should be considered with great care since no test concentration below 200 mg boric acid kg-1 was employed. Time
did not allow for a repetition of the experiment, besides the experiment is valid for a test-range identification. Becker-van Slooten (2004) reports ECx values corresponded with results obtained in the
present study (see Table 5).
Table 5. LCx and ECx concentrations (mg kg-1) for Folsomia fimetaria (14-days and 21-day) and Folsomia candida (21-day) exposed to boric acid in soil.
Reference |
Species |
LC10 |
LC50 |
EC10* |
EC50* |
14-day test |
|
|
|
|
|
This report |
F. fimetaria |
394
[225-563] |
648
[477-819] |
|
|
Becker van Slooten (2004) |
F. candida |
|
800
[633-1012] |
|
|
21-day test |
|
|
|
|
|
This report |
F. fimetaria |
< 200 |
>200 |
24±1
[21-26] |
117±3
[107-1289] |
This report |
F. candida |
<200 |
<200 |
22±1
[21-229] |
111±3
[103-121] |
28-day test |
|
|
|
|
|
Becker van Slooten (2004) |
F. candida |
|
|
|
147
[137-156] |
* Effect concentrations, EC10 and EC50, and bootstrapping intervals (equivalent to 95 % confidence intervals, ICP Nordberg-King, 1993). The studies by Becker van Slooten et al. (2004) is based on a
10% OECD soil
In conclusion: Based on the given data the following test concentration ranges are suggested for a ring-test: 0 to 300 mg boric acid kg-1 dry soil. The EC50 (reproduction) should be in the range of 100-150
mg boric acid kg-1 and the LC50 needs to be better defined in the ring test.
3.3.4 Proposed test ranges for a ring-test
Based on the three above tests, the ring-test test ranges should be 0-10 mg kg-1 for fipronil, 0-15 mg kg-1 for 3,5-dichlorophenol and 0-300 mg kg-1 for boric acid using reproductive output as a test
endpoint.
4 Conclusion
Searching the literature a variety of collembolan species have been used in terrestrial ecology. For the draft OECD guideline two collembolan species, Folsomia fimetaria and Folsomia candida, were
chosen as these two species have been much used in terrestrial ecotoxicology and standard tests are available. The two selected species represents two ecological and biological approaches. F. candida's
habitat is primarily high organic soils, whereas F. fimetaria is present in most agricultural soils. F. candida is parthenogenetic (asexual) reproducing, whereas F. fimetaria is sexually reproducing as are
most other species. It was attempted to include juvenile sex-determination as an additional endpoint for the test, but due to overlapping juvenile clutches (within one test) this was not possible. Given the
differences between the two species these were reviewed with respect to differences in sensitivity to chemicals, with particular emphasis on possible alterations caused by differences in their size. Neither the
literature study nor the laboratory studies resulted in conclusive differences in sensitivity between the species. A comparison in size-class related differences between the two species revealed that there was a
slight difference in sensitivity for animals of the same size, but a pronounced stage related difference in toxicity. Hence, the life-stage at which the organisms are exposed determines the sensitivity of the test.
In connection with the test guideline (and a ring-test) it will be necessary to have a positive control to ensure the stability of the test-system. Three compounds were tested in order to identify future test
ranges. The compounds were fipronil, 3,5-dichlorophenol and boric acid. The tests performed with these chemicals indicated that the test range for fipronil should be 0 to 10 mg fipronil kg-1, for
3,5-Dichlorophenol 0 to 15 mg 3,5-dichlorophenol kg-1 soil and for Boric acid 0 to 300 mg boric acid kg-1.
5 References
Andrén O and Lagerlöf J (1983) Succession of soil microarthopods in decomposing barley straw. In New Trends in Soil Biology. Proc VI Intl Coll Soil Zool, Lebrun Ph, André HM, De Medts A,
Gregoire-Wibo and Wauthy G (eds), Louvain-la-Neuve (Belgium), August 30-September 2, 1982, I. Dieu-Brichart, Ottignies-Louvain-la-Neuve, pp. 644-646.
Becker-van Slooten K, Campiche S, Feisthauer N, Stephenson G, Tarrasellas and Scroggings R (2004) Research in support of quality assurance requirements for new soil toxicity tests with Collembola.
Poster presented at SETAC 2004, Prague, Czeck.
Burges A and Raw F (eds) (1967) Soil Biology, Academic Press Inc., London.
Christiansen KA (1990) Insecta: Collembola. In Soil Biology Guide, Dindal DL (ed), John Wiley & Sons, New York, pp. 965-995.
Christensen BT, Boye Jensen M and Søndergård Klausen P (1987b) Springtails and mites (microarthropods) in different soil types with continuous spring barley and mulching of straw (In Danish
with an English summary), Statens Planteavlsforsøg, Tidsskrift for Planteavls Specialserie, Beretning nr. S 1884, Copenhagen.
Curry JP (1994) Grassland Invertebrates: Ecology, Influence on Soil Fertility and the Effects on Plant Growth, Chapman and Hall, 437 pp.
Crommentuijn T, Brils J and Van Straalen NM (1993) Influence of cadmium onnlife-history characteristics of Folsomia candida (Willem) in an artificial soil substrate. Ecotoxicol. Environ. Safety 26:
216-227.
Crossley DA, Mueller BR and Perdue JC (1992) Biodiversity of microarthropods in agricultural soils: relation to processes. Agricul. Ecosyst. Environ. 40: 37-46.
deFur PL, Crane M, Ingersoll C and Tattersfield L (1999) Endocrine disruption in invertebrates: Endocrinology, testing, and assessment. SETAC A technical publication.
Eisenbeis G and Wichard W (1987) Atlas on the Biology of Soil Arthropods, Springer Verlag, Berlin, 437 pp.
Filser J (1995) Collembola as indicators for long-term effects of intensive management. Acta Zool. Fennica 196: 326-328.
Filser J, Fromm H, Nagel RF and Winter K (1995) Effects of previous intensive agricultural management on microorganisms and the biodiversity of soil fauna. Plant and Soil 170: 123-129.
Fjellberg A (1985) Recent advances and future needs in the study of Collembola biology and systematics. Quaestiones Entomologicae 21: 559-570.
Fountain MT and Hopkin SP (2004a) Biodiversity of Collembola in urban soils and the use of Folsomia candida to assess soil 'quality'. Ecotox. 13:555-572.
Fountain MT and Hopkin SP (2004b) A comparative study of the effects of metal contamination on collembola in the field and in the laboratory. Ecotox. 13:573-587.
Frampton GK (1997) The potential of Collembola as indicators of pesticide usage: evidence and methods from the UK arable ecosystem. Pedobiologia 41:179-184.
Greenslade PJ (1991) Collembola (Springtails). In The Insects of Australia: A textbook for students and research workers, 2nd Edition, Division of Entomology, CSIRO, Melbourne University Press,
Melbourne, pp. 252-264.
Hale WG (1967) Collembola. In Soil Biology, Burgess A and Raw F (eds), Academic Press, London, pp. 397-411.
Heimann-Detlefsen D, Theiss S and Heimbach U (1994) Auswirkungen Unterschiedlich Intensiver Bewirtschaftungsintensitäten auf die Collembolenfauna des Ackerbodens. In: Bartels G and Kampmann T
(eds). Auswirkungen von Langjährigen Einsatzes von Pflanzenschutzmitteln bei unterschiedlichen Intensitätsstufen und Entwicklung von Bewertungskriterien. Mitteilungen aus der Biologischen Bundesanstalt
für Land- und Forstwirtschaft. Berlin Dahlem. Blackwell Scientific Publishers Heft 295: 230-273.
Herbert IN, Svendsen C, Hankard PK and Spurgeon DJ (2004) Comparison of instantaneous rate of population increase and critical-effect estimates in Folsomia candida exposed to four toxicants.
Ecotox. Environ. Safety 57:175-183.
Hopkin SP (1997) Biology of the Springtails, Insecta: Collembola, Oxford University Press, Oxford, 330 pp.
Houx NWH, Dekker A, van Kammen-Polman and Ronday R (1996) Acute toxicity test for terrestrial hazard assessment with exposure of Folsomia candida to pesticides in an aqueous medium. Arch.
Environ. Contam. Toxicol 30: 9-14.
Huang P (1992) Draft guideline on testing the effects of plant protection agents on Isotoma tigrina Nicolet (Collembola). Bulletin of the International Organization for Biological and Integrated
Control of Noxious Animals and Plants, West Palaeartic Regional Section (IOBC/WPRS) 15: 122-130.
Huldén L (1984) A check list of the Finnish insects. Small orders. Notulae Entomologicae 64: 1-29.
Ingersoll GG et al. (1999) Laboratory toxicity tests for evaluating potential effects of endocrine-disrupting compounds. In: deFur, P.L., Crane M, Ingersoll C, Tattersfield L. (1999) Endocrine disruption in
invertebrates: Endocrinology, testing, and assessment. SETAC A technical publication.
ISO (1999) Soil Quality - Effects of soil pollutants on Collembola (Folsomia candida):method for determination of effects on reproduction. No. 11267. International Organisation for Standardisation,
Geneve.
Kashian DR and Dodson SI (2004) Effects of vertebrate hormones on development and sex determination in Daphnia magna. Environ. Toxicol. Chem. 23: 1282-1288.
Keshan B and Ray AK (2001) The presence of Estradiol-17 and its specific binding sites in posterior silk gland of Bombyx mori. Gen. Comp. Endocrin. 123:23-30.
Kiss I and Bakonyi G (1992) Guideline for testing the effects of pesticides on Folsomia candida Willem (Collembola): Laboratory tests. Bulletin of the International Organization for Biological and
Integrated Control of Noxious Animals and Plants, West Palaeartic Regional Section (IOBC/WPRS) 15: 131-137.
Krogh PH (1994) Microarthropods as Bioindicators - a Study of Disturbed Populations, PhD thesis, University of Aarhus. National Environmental Research Institute, Silkeborg, Denmark, 96 pp.
Krogh PH (1995) Does a heterogenous distribution of food or pesticide affect the outcome of toxicity tests with Collembola? Ecotoxcol. Environ. Saf. 30:158-163.
Krogh PH and Bruus Pedersen M (1995) Laboratory toxicity testing with Collembola. In Effects of Pesticides on Meso- and Microfauna in Soil, Løkke H (ed) (1995), Ministry of the Environment and
Energy, Bekæmpelsesmiddelforskning fra Miljøstyrelsen, No. 8, pp. 39-58.
Krogh PH, Johansen K and Holmstrup M (1998) Automatic counting of collembolans for laboratory experiments. Appl. Soil Ecol. 7: 201-205.
LeBlanc GA (1999) Screening approaches for the evaluation of endocrine disruption in invertebrates. In Henshel DS, Black MC, Harrass MC, eds, Environmental Toxicology and Risk Assessment:
Standardization of Biomarkers for Endocrine Disruption and Environmental Assessment, Vol 8. STP 1364. American Society for Testing and Materials, Philadelphia, PA, pp 3–23.
LeBlanc GA and McLachlan JB (2000) Changes in the elimination profile of testosterone following exposure of the crustacean Daphnia magna to tributyltin. Ecotoxicol Environ Saf 45:296–303.
Lacey RF and Mallett MJ (1991) Further statistical analysis of the EEC ruing test of a method for determining the effect of chemicals on growth rate of fish. OECD Ad hoc Meeting of experts on aquatic
toxicology. WRC Medmenham, 10-12 December 1991. Room Document 3.
Løkke H (ed) (1995) Effects of pesticides on meso- and microfauna in soil, Ministry of the Environment and Energy, Bekæmpelsesmiddelforskning fra Miljøstyrelsen, No. 8, 185 pp.
da Luz TN, Ribero R, Sousa JP (2004) Avoidance test with Collembola and earthworms as early screening tools for site-specific assessment of polluted soils. Environ. Toxicol. Chem. 23:2188-2193.
Martikainen EAT and Krogh PH (1999) Effect of sol organic matter and temperature on toxicity of dimethoate to Folsomia fimetaria (Colembola:Isotomiidae)? Ecotoxcol. Environ. Safety 33:128-136.
Martikainen EAT (1996) Toxicity of dimethoate to some animal species in different soil types. Ecotoxcol. Toxicol. Chem. 18:865-872.
Mostert MA, Schoeman As, van der Merwe M (2000) The toxicity of five insecticides to earthworms of the Pheretima group, using an artificial soil test. Pest Manage. Science 56: 1093-1097.
Norberg-King TJ (1993) A liniear Interpolation Method for Sublethal Toxicity: The inhibition concentration (Icp) Approach (Version 2.0). and Dunnett Program (Version 1.5), NETAC Technical Report
03-93, U.S. Environmental Protection Agency, Duluth, MN, USA.
OECD Monograph no. 11 (Detailed review Paper on Aquatic Testing methods for Pesticides and Industrial Chemicals (Paris 1998).
Paoletti MG and Bressan M (1996) Soil invertebrates as bioindicators of human disturbance. Critical Reviews in Plant Sciences 15: 21-62.
Paoletti MG, Favretto MR, Stinner BR, Purrington FF and Bater JE (1991) Invertebrates as bioindicators of soil use. In Modern Techniques in Soil Ecology, Crossley DA Jr., Coleman DC, Hendrix PF,
Cheng W, Wright DH, Beare MH and Edwards CA (eds), Agricul. Ecosyst. Environ. 34: 305-313.
Parmelee RW, Wentsel RS, Phillips CT, Simini M and Checkai RT (1993) Soil microcosm for testing the effects of chemical pollutants on soil fauna communities and trophic structure. Environ. Toxicol.
Chem. 12: 1477-1486.
Pedersen MB, Axelsen JA, Strandberg B, Jensen J and Attril MJ (1999) The impact of a copper gradient on a microarthropod field community. Ecotoxocol. 8:467-483.
Pedersen MB, van Gestel and Elmegaard N (2000) Effects of copper on reproduction of two Collembola species exposed through soil, food, and water. Environ. Toxicol. Chem. 19:2579-2588.
Pedersen MB and van Gestel CAM (2001) Toxicity to the Collembolan Folsomia fimetaria in relation to the age of soil contamination. Ecotox. Environ. Safety 49:54-59.
Petersen H (1994) A review of collembolan ecology in ecosystem context. Acta Zool. Fennica 195: 111-118.
Petersen H and Gjelstrup P (1995) Development of a semi-field method for evaluation of laboratory tests as compared with field tests. In Effects of Pesticides on Meso- and Microfauna in Soil, Løkke H
(ed), Ministry of the Environment and Energy, Bekæmpelsesmiddelforskning fra Miljøstyrelsen No. 8, pp. 67-142.
Petersen H and Luxton M (1982) A comparative analysis of soil fauna populations and their role in decomposition processes. Oikos 39: 287-388.
Riepert F and Kula C (1996) Development of Laboratory Methods for Testing Effects of Chemicals and Pesticides on Collembola and Earthworms, Mitteilungen aus der Biologischen Bundesanstalt
für Land- und Forstwirtschaft, Berlin-Dahlem, Heft 320, Parey Buchverlag, Berlin 1996, 82 pp.
Sandifer RD and Hopkin SP (1996) Effects of pH on the toxicity of cadmium, copper, lead and zinc to Folsomia candida Willem, 1902 (Collembola) in a standard laboratory test system. Chemosphere 33:
2475-2486.
Sandifer RD and Hopkin SP (1997) Effects of temperature on the relative toxicity of Cd, Cu, Pb, abd Zn to Folsomia candida (Collembola). Ecotox. Environ. Safety 37: 125-130
Scopes NEA and Lichtenstein EP (1967) The use of F. fimetaria and Drosophila melanogaster as test insects for the detection of insecticide residues. J.Economic Entomol 60: 1539-1541.
Sheals JG (1956) Soil population studies. I. The effects of cultivation and treatment with insecticides. Bull Entomoll Research 47: 803-822.
Scott-Fordsmand JJ, Krogh PH and Weeks JM (1997) Sublethal toxicity of copper to a soil-dwelling springtail (Folsomia fimetaria) (Collembola: Isotomidae). Environ. Toxicol. Chem. 16:2538-2542.
Scott-Fordsmand JJ, Weeks JM and Krogh PH (2000) Responses of Folsomia fimetaria (Collembola: isotomidae) to copper under different soil copper contamination histories, in relation to risk
assessment. Environ. Toxicol. Chem. 19:1297-1303.
Spahr HJ (1981) Die Bodenbiologische Bedeutung von Collembolen und Ihre Eignung als Testorganismem für die Ökotoxikologie. Anzeiger für Schadlingskunde Pflanzenschutz Umweltschutz b 27-29.
Thompson AR and Gore FL (1972) Toxicity of twenty-nine insecticides to Folsomia candida: Laboratory studies. J. Econom. Entomol 65: 1255-1260.
Tingle CCD, Rother JA, Dewhurst CF, Lauer S and King WJ (2003) Fipronil: Environmental fate, ecotoxicology, and human health concerns. Rev. Environ. Contam. Toxicol. 176:1-66.
van Gestel CAM and Koolhaas JE (2004) Water-extractibility, free ion activity, and pH explain sorption and toxicity to Folsomia candida (Collembola) in seven soil-pH combinations. Environ. Toxicol.
Chem. 23: 1822-1833.
Verslycke T, Poelmans S, de Wasch K, de Brabander HF and Janssen CR (2004) Testosterone and energy metabolism in estuarine mysid Neomysis integer (Crustacea:Mysidacea) following exposure to
endocrine disruptors. Environ. Toxicol. Chem. 23: 1289-1296.
Verslycke T, De Wasch K, De Brabander F, Janssen CR (2002) Testosterone metabolism in the estuarine mysid Neomysis integer (Crustacea; Mysidacea): Identification of testosterone metabolites and
endogenous vertebrate-type steroids. Gen. Comp. Endocrinol. 126:190–199.
Wallwork JA (1970) Ecology of Soil Animals, McGraw-Hill, London, 283 pp.
Weigmann G, Papenhausen U, Kratz W, Gruttke H (1985) Die wirkung chemisher belastungen auf tier- und pflanzengesellschaften städtischer brachflächen. Specielle Berichte Berlin, Kern Forshung Anlage
Jülich, vol 296. 121-129.
Wiles JA and Frampton GK (1996) A field bioassay approach to assess the toxicity of insecticide residues on soil to Collembola. Pesticide Science 47: 273-285.
Wiles JA and Krogh PH (1998) Testing with the collembolans I. viridis, F. candida and F. fimetaria. In Handbook of soil invertebrate toxicity tests (eds Løkke H and Van Gestel CAM), pp. 131-156.
John Wiley & Sons, Ltd., Chichester.
OECD GUIDELINES FOR THE TESTING OF CHEMICALS
PROPOSAL FOR A NEW GUIDELINE
Annex 1
Annex 2
Annex 3
Annex 4
Collembola Reproduction Test (Folsomia fimetaria and Folsomia candida)
INTRODUCTION
1. This Test Guideline is designed for assessing the effects of chemicals on the reproductive output of the collembolans, Folsomia fimetaria and Folsomia candida in soil. It is principally based on a method
developed by the National Environmental Research Institute, Denmark [1] and the [2]. When more information is available it should be considered including more Collembola species in a test-programme.
2. Soil-dwelling Collembola are ecologically relevant species for ecotoxicological testing. Compared to for example earthworms the collembolans represent organisms with an exoskeleton, indicating a
different route (or at least different rate) of exposure.
3. Population densities of Collembola commonly reach 105 m-2 In soil and leaf litter layers in many terrestrial ecosystems [3,4]. Because of their small size however, adults typically measure 0.5 - 5 mm their
contribution to total soil animal biomass and respiration is low, estimated at being between 1 and 5% [5]. Their most important role may therefore be as potential regulators of processes through microbivory
and microfauna predation. Springtails are prey animals for a wide variety of endogeic and epigeic invertebrates, such as mites, centipedes, spiders and carabidae and rove beetles. Collembola contribute to
decomposition processes in soil and on acidic stands they may be the most important soil invertebrates as earthworms and diplopods are absent.
F. fimetaria has a worldwide distribution and is common in several soil types ranging from sandy to loamy soils and from mull to mor soils. It has been recorded in agricultural soils all over Europe [6]. It has
an omnivorous feeding habit, including fungal hyphae, bacteria, protozoa and detritus in its food. It interacts through grazing with infections of plant pathogenic fungi [7] and may influence mychorrhiza, as is
known to be the case for F. candida. It is a sexually reproducing species.
F. candida is worldwide distributed. Although it is not common in most natural soils, it often occurs in very high numbers in humus rich sites. It is a blind, unpigmented collembolan, which reproduces
parthenogenetically. It has a well-developed furca (jumping organ) and an active running movement and jumps readily if disturbed. It's a parthenogenetic species.
PRINCIPLE OF THE TEST
4. Synchronous adult Collembola are exposed to a range of concentrations of the test substance mixed into an artificial soil (or alternative soil). The test scenario can be divided into two steps:
4.a A range-finding test, in case no sufficient information is available, in which mortality is the main endpoint assessed after two weeks exposure
4.b A definitive reproduction test in which the total number of juveniles produced by parent animal and the survival of parent animals are assessed. The duration of this definitive test is three
(F. fimetaria) /four (F. candida) weeks.
The toxic effect of the test substance on the reproductive output of the animals is expressed as ECx (e.g. EC10, EC50) by using a sigmoid-regression model to estimate the concentration that would cause x
% reduction in reproductive output.
INFORMATION ON THE TEST SUBSTANCE
5. The following information relating to the test substance should be available to assist in the design of appropriate test procedures:
- water solubility
- the log Kow,
- the soil water partition coefficient
- the vapour pressure of the test substance should preferably be known.
- Additional information on the fate of the test substance in soil, such as the rates of photolysis and hydrolysis is desirable.
6. This Guideline can be used for water soluble or insoluble substances. However, the mode of application of the test substance will differ accordingly. The Guideline is not applicable to volatile substances,
i.e. substances for which the Henry's constant or the air/water partition coefficient is greater than one, or substances for which the vapour pressure exceeds 0.0133 Pa at 25°C.
VALIDITY OF THE TEST
7. The following criteria should be satisfied in the controls for a test result to be considered valid:
7.a Adult mortality should not exceed 20% at the end of the range-finding test and after the first three/four weeks of the reproduction test.
7.b F. candida: assuming that 10 adults per vessel were used in setting up the test, an average of at least 100 juveniles per vessel should have been produced at the end of the four-week
test.
7.c F. fimetaria: assuming that 20 adults (10 females and 10 males) per vessel were used in setting up the test, an average of at least 200 juveniles per vessel should have been produced at
the end of the three-week test.
7.d The coefficient of variation of juveniles should be less than 25% at the end of the reproduction test.
Where a test fails to meet the above validity criteria the test should be terminated unless a justification for proceeding with the test can be provided. The justification should be included in the test report.
REFERENCE SUBSTANCE
8. A reference substance must be tested at its EC50 concentration for the chosen test soil type either at regular intervals or possibly included in each test to verify that the response of the test organisms has
not changed significantly over time and that the test system is responding at is normal level. A suitable reference substance is -TO BE DECIDED-, which has been shown to affect survival and reproduction
of Collembola [8, 9].
DESCRIPTION OF THE TEST
Equipment
9. Containers capable of holding 30 gram of soil are suitable test vessels. The material should either be glass or inert plastic (non-toxic). The vessels should have a cross-sectional area allowing the soil depth
to be between 3-4 cm. The vessels should have transparent lids (e.g. glass or polyethylene) that are designed to reduce water evaporation whilst allowing gas exchange between the soil and the atmosphere.
The container should be at least partly transparent to allow light transmission.
10. Normal laboratory equipment is required, specifically the following:
- drying cabinet;
- stereomicroscope;
- pH-meter and photometer;
- suitable accurate balances;
- adequate equipment for temperature control;
- adequate equipment for humidity control (not essential if exposure vessels have lids);
- incubator or small room with air-conditioner;
- tweezers, hooks or loops.
Preparation of the artificial soil
11. The artificial soil is prepared according to OECD guideline 207 [10] and consist of the below components. It is highly preferable to use, in addition to the OECD soil, a natural standard soil such as Lufa
Speyer. With regard to the artificial soil its recommended composition is as follows (based on dry weights, dried to a constant weight at 105°C):
- 5 or 10% sphagnum peat, air-dried and finely ground (a particle size of 2 ± 1 mm is acceptable); it is recommended to check that a soil prepared with a fresh batch of peat is suitable for
containing the Collembola before it is used in a test. It is recommended to measure the C/N ratio, pH and CEC of the peat.
- 20% kaolin clay (kaolinite content preferably above 30%);
- approximately 0.3 to 1.0% calcium carbonate (CaCO3, pulverized, analytical grade) to obtain a pH of 6.0 ± 0.5; the amount of calcium carbonate to be added may depend principally on
the quality/nature of the peat;
- approximately 69% air-dried industrial sand (depending on the amount of CaCO3 needed), predominantly fine sand with more than 50% of the particles between 50 and 200 microns.
It is advisable to demonstrate the suitability of the test soil for use in the test and for achieving the test validity criteria before using the soil in a definitive test
11. The dry constituents of the soil are mixed thoroughly (e.g. in a large-scale laboratory mixer). This should be done at least one week before starting the test. The mixed soil should be stored for two weeks
in order to equilibrate/stabilise the acidity. For the determination of pH a mixture of soil and 1 M potassium chloride (KCl) or 0.01 M calcium chloride (CaCl2) solution in a 1:5 ratio is used (according to
Annex 3). If the soil is more acidic than the required range, it can be adjusted by addition of an appropriate amount of CaCO3. If the soil is too alkaline it can be adjusted by the addition of more of the
mixture comprising, but excluding the CaCO3.
12. The maximum water holding capacity (WHC) of the artificial soil is determined in accordance with procedures described in Annex 2. At the start of the test, the pre-moistened soil is divided into portions
corresponding with the number of test concentrations (and reference substance where appropriate) and controls used for the test. The moisture content is adjusted to about 50% of the maximum WHC
(corresponding to 50 ± 10% moisture dry mass) by the addition of the test substance solution and/or distilled or de-ionised water. However, moisture content should be optimized to attain a loose porous
texture to allow animals to enter into the pores. The weight of the containers is determined at the beginning, in the middle and at the end of the test.
Selection and preparation of test animals
13. The recommended test species are Folsomia fimetaria and Folsomia candida. These two species are some of the most accessible species of Collembola, with specimens sizes of up to 2.5 mm in
length. These collembolans are culturable and commercially available.
13.a Preparation of culturing substrate
The culturing substrate is plaster of Paris (calcium sulphate) with activated charcoal. This provides a moist substrate, the function of the charcoal being to absorb waste gases and excreta [11,
12]. Different forms of charcoal may be used to facilitate observations of the Collembola. For example, powdered charcoal is used for F. candida and F. fimetaria (producing a black/grey
plaster of Paris).
Substrate constituents:
20 ml of activated charcoal
200 ml of distilled water
200 ml of plaster of Paris
or
50 g of activated pulverized charcoal
260-300 ml of distilled water
400 g plaster of Paris.
13.c Breeding
Collembolans are held in Petri dishes (90 mm x 13 mm) with the bottom covered by a 0.5 cm layer of plaster of Paris/charcoal substrate. They are cultured at 20 ± 1°C at a light:dark cycle of
12:12 hours.
Every time the Petri dishes are 4-8 weeks old, it is necessary to transfer the animals to Petri dishes with newly prepared plaster of Paris/charcoal substrate, and they will start producing eggs
again.
Cultures are kept in an incubator at a temperature of 20±0.5°C, under a 16:8 hours light regime (<1000 Lux). Containers are kept moist at all times. Any dead individuals should be removed
from the containers immediately, as should any stale food. Stock cultures of both species should be periodically moved (e.g. every 2-3 months) to fresh plaster of Paris.
13.d Food source
Granulated dried baker's yeast is used as the principal food supply for F. candida. Fresh food is provided either once or twice a week, to avoid spoilage by fungal growth. It is placed on
filter paper discs, which are removed together with the food when necessary. The mass of baker's yeast added should be tailored to the size of collembolan population, but as a general rule
10-30 mg is sufficient.
14. The animals used in the tests are adult individuals. Synchronisation of the breeding culture is necessary, especially so for the F. fimetaria.
14.a Synchronising and culturing F. fimetaria.
1) Prepare Petri dishes with a 0.5 cm layer of plaster of Paris/charcoal substrate.
2) For egg laying transfer 150-300 adult F. fimetaria from a 4-8 weeks old substrate to the containers and feed with 15 mg baker's yeast.
3) Keep the culture at 20±1°C (means should be 20°C) and a light:dark cycle 12:12 hours (<1000 Lux).
4) After 9 days the eggs are carefully collected with a needle and spatula and moved to an “egg-paper” (small pieces of filter paper dipped in plaster of Paris/charcoal
substrate) which is placed in a fresh Petri dish with plaster of Paris/charcoal substrate. It is important that the egg-paper and substrate are humid, or else the eggs will
dehydrate.
5) Eggs and hatched juveniles are cultured in same manner as the adults.
6) After three days most of the eggs on the egg-paper have hatched, and the juveniles tend to stay under the egg-paper.
7) To have evenly aged juveniles the egg-paper with unhatched eggs is removed from the Petri dish with a tweezer. The juveniles stay in the dish. Age of the juveniles are 0-3
days.
8) Label the container with date of hatching and provide baker's yeast.
14.b. Synchronising and culturing F. candida.
1) Prepare new breeding containers with a 1 cm layer of plaster of Paris.
2) Transfer several hundred adult Collembola from stock cultures into each container, and supply with baker's yeast.
3) Keep the culture at 20±1°C (means should be 20°C) and a light:dark cycle 12:12 hours (<1000 Lux).
4) Remove adults after 24 to 48 hours.
5) Eggs and hatched juveniles are cultured in same manner as the adults.
6) Observe daily and record date of hatching. Provide food immediately after hatching.
7) After three days most of the eggs on the egg-paper have hatched, and the juveniles tend to stay under the egg-paper.
8) To have evenly aged juveniles the egg-paper with unhatched eggs is removed from the Petri dish with a tweezer. The juveniles stay in the dish. Age of the juveniles are 0-3
days.
9) Label the container with date of hatching and provide baker's yeast.
15. Other Collembolan species may also be suitable, e.g. I. viridis or O. folsomi. If other species of Collembola are used, they must be clearly identified and the rationale for the selection of the species
should be reported.
Preparation of test concentrations
16. Two methods of application of the test substance can be used: mixing the test substance into the soil or application to the soil surface. The selection of the appropriate method depends on the purpose of
the test. In general, mixing of the test substance into the soil is recommended. However application procedures that are consistent with normal agricultural practice may be required (e.g. spraying of liquid
formulation or use of special pesticide formulations such as granules or seed dressings).
Mixing the test substance into the soil
Test substance soluble in water
17. A solution of the test substance is prepared in deionised water in a quantity sufficient for all replicates of one test concentration. Each solution of test substance is mixed thoroughly with one batch of
pre-moistened soil before being introduced into the test vessel.
Test substance insoluble in water
18. For chemicals insoluble in water but soluble in organic solvents, the test substance can be dissolved in the smallest possible volume of a suitable vehicle (e.g. acetone). Only volatile solvents should be
used. When such vehicles are used, all test concentrations and an additional control should contain the same minimum amount of the vehicle. The vehicle is sprayed on or mixed with a small amount, for
example 2.5 g, of fine quartz sand. The vehicle is eliminated by evaporation under a fume hood for at least one hour. This mixture of quartz sand and test substance is added to the pre-moistened soil and
thoroughly mixed after adding an appropriate amount of de-ionised water to obtain the moisture required. The final mixture is introduced into the test vessels.
Test substance insoluble in water and organic solvents
19. For substances that are poorly soluble in water and organic solvents, the equivalent of 2.5 g of finely ground quartz sand per test vessel is mixed with the quantity of test substance to obtain the desired
test concentration. This mixture of quartz sand and test substance is added to the pre-moistened soil and thoroughly mixed after adding an appropriate amount of de-ionised water to obtain the required
moisture content. The final mixture is divided between the test vessels. The procedure is repeated for each test concentration and an appropriate control is also prepared.
Application of the test substance to the soil surface
20. The soil is treated after the Collembolans are added. The test containers are first filled with the moistened soil substrate and the weighed. The test substance is applied. It should not be added to the soil
within half an hour of introducing the Collembola so as to avoid any direct exposure to the test substance by skin contact. When the test substance is a pesticide it may be appropriate to apply it to the soil
surface by spraying. The test substance should be applied to the surface of the soil as evenly as possible using a suitable laboratory-scale spraying device to simulate spray application in the field. Before
application the cover of the test container should be removed and replaced by a liner, which protects the side walls of the container from spray. The liner can be made from a test container with the base
removed. The application should take place at a temperature within ± 2°C of variation and for aqueous solutions, emulsions or dispersions at a water application rate of between 600 and 800 µl/m². The rate
should be verified using an appropriate calibration technique. Special formulations like granules or seed dressings could be applied in a manner consistent with agricultural use.
Test containers should be left uncovered for a period of one hour to allow any volatile solvent associated with the application of the test substance to evaporate.
PERFORMANCE OF THE TESTS
Test groups and controls
21. For each test concentration, an amount of test soil corresponding to 25 g dry weight is placed into the test vessel. Controls, without the test substance, are also prepared. Food is added to each vessel.
Ten (F. candida) or twenty (F. fimetaria) collembolans are randomly allocated to each test vessel. The individuals are carefully transferred into each test vessel and placed on the surface of the soil using.
For efficient transfer of the animals a low-suction air flow devise can be used. The number of replicates for test concentrations and for controls depends on the test design used. The test vessels are
positioned randomly in the test incubator and these positions are re-randomised weekly.
22. If a vehicle is used for application of the test substance, one control series containing quartz sand sprayed or mixed with solvent should be run in addition to the test series. The solvent or dispersant
concentration should be the same as that used in the test vessels containing the test substance. A control series containing additional quartz sand (2.5 g per vessel) should be run for substances requiring
administration.
Test conditions
23. The test temperature is 20 ± 2°C. To discourage collembolans from escaping from the soil, the test is carried out under controlled light-dark cycles (preferably 16 hours light and 8 hours dark) with
illumination of 400 to 800 lux in the area of the test vessels.
24. In order to check the soil humidity, the vessels are weighed at the beginning of the test and thereafter once a week. Weight loss is replenished by the addition of an appropriate amount of deionised
water. It should be noted that loss of water can be reduced by maintaining a high air-humidity (> 80%) in the test incubator.
25. The moisture content and the pH, should be measured at the beginning and the end of both the range-finding test and the definitive test. Measurements should be made in control and treated (all
concentrations) soil samples prepared and maintained in the same way as the test cultures. Food should be added at the top of the soil samples at the start of the test and after 2 weeks. The amount of food
added should be the same as that added to the test cultures.
Feeding
26. A suitable source is granulated dried Bakers's yeast, commercially available for household use.
27. At the beginning of the test and after each 14-day interval, add about 15 mg yeast to each container.
Design for the range-finding test
28. When necessary, a range-finding test is conducted with, for example, five test substance concentrations of 0.1, 1.0, 10, 100, and 1000 mg/kg (dry weight of soil). Two replicates for each treatment and
control are sufficient.
29. The duration of the range-finding test is two weeks. At the end of the test, mortality of the Collembola is assessed. A Collembola is recorded as dead if not present in the extraction. Additional
information to mortality may also be useful in deciding on the range of concentrations to be used in the definitive test.
30. The LC50 can be determined using Probit analysis. In order for an accurate determination of the LC50 performing the test it should be ensured that the number of replicates and test concentrations
matches the power requirements for the test.
Design for the definitive reproduction test
31. For determination of the ECx (e.g. EC10, EC50), an adequate number of concentrations to cause at least four statistically significantly different mean responses at these concentrations is recommended..
At least four replicates for each test concentration and four control replicates are recommended. The spacing factor should ensure that the majority of test concentrations are on the slope of the ECx curve. It
should be considered that the power requirements of the test should be maintained.
30. Number of test individuals and duration (three test designs).
30 a. For the F. fimetaria test twenty adults per test vessel should be used. Food is added to the test vessels at the beginning of the test and then after 14 days up to and including Day 21.
On Day 21 the soil samples should be extracted and counted.
30 b. For the F. candida test ten adults per test vessel should be used. Food is added to the test vessels at the beginning of the test and then after 14 days up to and including Day 28. On
Day 28 the soil samples should be extracted and counted.
Limit test
31. If no effects are observed at the highest concentration in the range-finding test (i.e. 1000 mg/kg), the reproduction test could be performed as a limit test, using a test concentration of 1000 mg/kg. A limit
test will provide the opportunity to demonstrate that the NOEC for reproduction is greater than the limit concentration whilst minimising the number of Collembola used in the test. Eight replicates should be
used for both the treated soil and the control.
Power of the test
32. For all test designs it is advised that the Type I error is set at a 5% level and the type II error is set at maximum 20%. This should be ensured for each test.
Counting animals
33. Two methods of extraction can be performed.
33.a. First method: A controlled temperature gradient extractor based on principles by MacFayden can be used [1]. The heat coming from a heathing element at the top of the extraction box
(regulated though a thermistor placed ion the surface of the soil sample). The temperature in the cooled liquid surrounding the collecting vessel is regulated through a thermistor situated at the
surface of the collection box (placed below the soil core). The thermistors are connected to a programmable controlling unit which raises the temperature according to a pre-programmed
schedule. Animals are collected in the cooled collecting box (2°C) with the bottom layer of plaster of Paris/charcoal. Extraction is started at 25°C and the temperature is increased
automatically every 12 h by 5°C. After 12 h at 40°C the extraction is finished.
33.b. Second method: After the experimental incubation period the number of juvenile Collembola present is assessed by floatation. This involves emptying the tube of soil into a 250 ml
vessel and adding approx. 200 ml of distilled water. The soil is gently agitated with a fine paintbrush to allow Collembola to float to the water surface. A small amount, approx. 0.5 ml, of
black Kentmere photographic dye may be added to the water to aid counting by increasing the contrast between the water and the white Collembola. The dye is not toxic to the Collembola.
Counting: Counts of numbers may be carried out by eye or under a light microscope using a grid placed over the floatation vessel or by photographing the surface of each vessel and later counting
Collembola on the enlarged prints or projected slides. Count may also be performed using digital image processing techniques [13].
Summary and timetable for the test
34. The steps of the test can be summarised as follows:
Purpose |
Range-finding test |
Definitive Reproduction test - 1 |
Definitive Reproduction test - 2 |
Species |
F. fimetaria/F. candida |
F. candida |
F. fimetaria |
Aim |
Mortality |
Reproduction |
Reproduction |
Time (day) |
|
|
|
-9-12
(F. candida)
-13-26
(F. fimetaria) |
Preparation of synchronous culture |
Preparation of synchronous culture |
Preparation of synchronous culture |
Day 5 or earlier |
- Prepare artificial soil (mixing of dry constituents) |
- Prepare artificial soil (mixing of dry constituents) |
- Prepare artificial soil (mixing of dry constituents) |
Day 3 |
- Check pH of artificial soil
- Measure max WHC of soil |
- Check pH of artificial soil
- Measure max WHC of soil |
- Check pH of artificial soil
- Measure max WHC of soil |
Day 2 to 1 |
- Sort Collembola for testing |
Sort Collembola for testing |
- Sort Collembola for testing |
Day 1 |
- Pre-moisten artificial soil and distribute into batches
- Prepare stock solutions
- Apply test substance if solvent required |
- Pre-moisten artificial soil and distribute into batches
- Prepare stock solutions
- Apply test substance if solvent required |
- Pre-moisten artificial soil and distribute into batches
- Prepare stock solutions
- Apply test substance if solvent required |
Day 0 |
- Prepare stock solutions
- Apply test substance
- Weigh test substrate into test vessels
- Mix in food
- Introduce Collembola
- Measure soil pH and moisture content |
- Prepare stock solutions
- Apply test substance
- Weigh test substrate into test vessels
- Mix in food
- Introduce Collembola
- Measure soil pH and moisture content |
- Prepare stock solutions
- Apply test substance
- Weigh test substrate into test vessels
- Mix in food
- Introduce Collembola
- Measure soil pH and moisture content |
Day 7 |
- Check soil moisture content |
- Check soil moisture content |
- Check soil moisture content |
Day 14 |
- Determine adult mortality
- Estimate number of juveniles
- Measure soil pH and moisture content |
- Check soil moisture content
- Feed |
- Check soil moisture content
-Feed |
Day 21 |
|
- Check soil moisture content |
-Extract all individuals
- Check soil moisture content
-Measure pH |
Day 28 |
|
- Extract all individuals
- Check soil moisture content
-Measure pH |
|
DATA AND REPORTING
Treatment of results
35. An overview is in the following but more detailed statistical guidance for analysing test results is given in the "OECD draft statistical report 2003".
36. In the range finding test, the main endpoint is mortality. Probit analysis [14] should normally be applied to determine the LC50. However, in cases where this method of analysis is unsuitable (e.g., if less
than three concentrations with partial kills are available), alternative methods can be used. These methods could include moving averages [14], the trimmed Spearman-Karber method [15] or simple
interpolation (e.g., geometrical mean of LC0 and LC100, as computed by the square root of LC0 multiplied by LC100).
ECx estimation (preferred method)
37. To compute any ECx value, the per-treatment means data are used for regression analysis (linear or non-linear), after an appropriate dose-response function has been selected [16]. Among suitable
functions are the normal sigmoid, logistic or Weibull functions, containing two to four parameters, some of which can also model hormetic responses. If a dose-response function was fitted by linear
regression analysis a significant r² (coefficient of determination) and/or slope should be found with the regression analysis before estimating the ECx by inserting a value corresponding to x% of the control
mean into the equation found by regression analysis. 95%-confidence limits are calculated according to [17].
38. Alternatively, one may express the treatment results as percentages of the control result or as percent inhibitions relative to control. In these cases, the normal (logistic, Weibull) sigmoid curve can often
be easily fitted to the results using the probit regression procedure [17]. In these cases the weighting function has to be adjusted for metric responses as given by [18]. However, if hormesis has been
observed, regression analysis should be replaced by a four-parameter logistic or Weibull function, fitted by a non-linear regression procedure [19]. If a suitable dose-response function cannot be fitted to the
data, one may use alternative methods to estimate the ECx, and its confidence limits, such as Moving Averages after Thompson [14] and the Trimmed Spearman-Karber procedure [15].
NOEC estimation (alternative method)
39. If an analysis of variance has been performed, the standard deviation, s, and the degrees of
freedom, df, may be replaced by the pooled variance estimate obtained from the ANOVA and by its degrees
of freedom, respectively – provided variance does not depend on the
concentration. In this case, use the single variances of control and treatments. Those values are usually calculated by commercial
statistical software using the per-vessel results as replicates. If pooling data for the negative and solvent controls appears reasonable rather than testing against one of
those, they should be tested to see that
they are not significantly different (for the appropriate test consider Annex 4). Further statistical analysis and inferences depend on whether the replicate values are normally distributed and are homogeneous
with regard to their variance.
41. NOEC estimation: Kolmogoroff-Smirnov's [20] and Bartlett's-test procedures [21] are used respectively for testing for normality and homogeneity of variance
homogeneity. With normally distributed
and homogeneous data, multiple t-tests such as Dunnett’s test [22, 23] or William’s test (a = 0.05,
one-sided) [24, 25] should be performed. It should be noted that, in the case of unequal
replication, the
tabulated t-values must be corrected as suggested by Dunnett and Williams.
Sometimes, because of large variation the responses do not increase/decrease
regularly. In this case Dunnett’s test does not lead
to reasonable NOEC/LOEC values and analysis by Williams-test is to be preferred.
Alternatively, Otherwise, a multiple U-test, e.g. the Bonferroni-U-test according to Holm [26], could be
used.
42. If a limit test has been performed and the prerequisites of parametric test procedures (normality, homogeneity) are fulfilled, the pair-wise Student t-test can be used or otherwise the
Mann-Whitney-U-test procedure [20].
40. The test report should include the following information:
40.a. Test substance:
- physical nature and, where relevant physical-chemical properties (e.g. water solubility, vapour pressure);
- chemical identification of the test substance according to IUPAC nomenclature, CAS-number, batch, lot, structural formula and purity;
- expiry date of sample.
40.b. Test species:
- test animals used: species, scientific name, source of organisms and breeding conditions.
40.c. Test conditions:
- ingredients and preparation details of the artificial soil; (minimum: C/N ratio, pH, WHC, CEC)
- method of application of the test substance (data verifying homogeneity of application);
- description of the test conditions, including temperature, moisture content, pH, etc.;
- full description of the experimental design and procedures.
40.d. Test results:
- Actual concentration of the tested compound in the test media (at minimum for control exposures)
- mortality of adult Collembola after two weeks and the number of juveniles at the end of the range-finding test;
- mortality of adult Collembola after three weeks exposure and the full record of juveniles at the end of the definitive test;
- any observed physical or pathological symptoms and behavioural changes in the test organisms;
- the LC50, the NOEC and/or ECx (e.g. EC50, EC10) for reproduction if some of them are applicable with confidence intervals, and a graph of the fitted model used for its
calculation, the slope of the dose-response curve and its standard error;
- all information and observations helpful for the interpretation of the results.
- Power of the actual test.
Deviations from procedures described in this guideline and any unusual occurrences during the test.
LITERATURE
(1) Wiles JA and Krogh PH (1998) Testing with the collembolans I. viridis, F. candida and F. fimetaria. In Handbook of soil invertebrate toxicity tests (ed. H. Løkke and C. A. M. Van Gestel), pp.
131-156. John Wiley & Sons, Ltd., Chichester.
(2) ISO (1999) Soil Quality - Effects of soil pollutants on Collembola (Folsomia candida):method for determination of effects on reproduction. No. 11267. International Organisation for Standardisation,
Geneve.
(3) Burges A and Raw F (Eds) (1967) Soil Biology. Academic Press. London
(4) Petersen H and Luxton M (1982) A comparative analysis of soil fauna popyulations and their role in decomposition processes. Oikos 39: 287-388
(5) Petersen H (1994) A review of collembolan ecology in ecosystem context. Acta Zoologica Fennnica 195: 111-118
(6) Hopkin SP (1997). Biology of the Springtails (Insecta : Collembola). Oxford University Press. 330pp (ISBN 0-19-854084-1).
(7) Ulber B (1983) Einfluss von Onychirurus fimatus Gisin (Collembola, Onychiuridae) und Folsomia fimetaria L. (Collembola, Isotomidae) auf Pythium ultimum trow. einen Erreger des Wurzelbrandes der
Zuruckrübe. In New trends in soil Biology (Lebrun Ph, André HM, De Medts A, Grégoire-Wibo, Wauthy G (Eds), Proceedings of the VI international colloquium on soil zoology, Louvain-la-neuve
(Belgium), 30 August-2 September 1982, I. Dieu-Brichart, Ottignies-Louvain.la.neuve, pp. 261-268.
(8) Scott-Fordsmand JJ and Krogh PH (2004). Background report on prevalidation of an OECD springtail test guideline. MST in press.
(9) Becker-van Slooten K, Campiche S, Feisthauer N, Stephenson G, Tarrasellas and Scroggings R (2004) Research in support of quality assurance requirements for new soil toxicity tests with Collembola.
Poster presented at SETAC 2004, Prague, Czeck.
(10) OECD (Organisation for Economic Cooperation and Development) (1984). Earthworm, Acute Toxicity Tests, Guideline No. 207. OECD, Paris.
(11) Edwards (1955) Simple techniques for raraing Collembola, Symphyla and other small soil inhabiting arthropods. In Soil Zoology (Kevan DKMcE, Ed). Butterworths, London, pp. 412-416.
(12) Goto HE (1960) Simple techniques for the rearing of Collembola and a not on the use of a fungistatic substance in the cultures. Entomologists' Monthly Magazine 96:138-140
(13) Krogh PH, Johansen K and Holmstrup M (1998) Automatic counting of collembolans for laboratory experiments. Appl. Soil Ecol. 7, 201-205.
(14) Finney DJ (1978) Statistical Method in Biological Assay. - Charles Griffin & Company Ltd, London.
(15) Hamilton MA, Russo RC and Thurston RV (1977) Trimmed Spearman-Karber Method for estimating median lethal concentrations in toxicity bioassays. Environ. Sci. Technol. 11(7), 714-719;
Correction Environ. Sci. Technol. 12(1998), 417.
(16) OECD (2003) Draft Guidance Document for on the Statistical Analysis of Ecotoxicity Data. Environment Directorate, ORGANISATION FOR ECONOMIC CO-OPERATION AND
DEVELOPMENT, Paris 2003.
(17) Finney DJ (1971) Probit Analysis (3rd ed.), pp. 19-76. Cambridge Univ. Press.
(18) Christensen ER (1984) Dose-response functions in aquatic toxicity testing and the Weibull model. Water Research 18, 213-221.
(19) Van Ewijk PH and Hoekstra JA (1993) Calculation of the EC50 and its confidence interval when sub-toxic stimulus is present. Ecotox, Environ. Safety. 25, 25-32.
(20) Sokal, R.R. and F.J. Rohlf. (1981) Biometry. The principles and practice of statistics in
biological research. 2nd edition. W.H. Freeman and Company. New York.
(21) Miller, R.G., Jr. (1986). Beyond ANOVA, basics of applied statistics. John Wiley & Sons. New York.
(22) Dunnett, C.W. (1955). A multiple comparison procedure for comparing several treatments with a control. Amer. Statist. Ass. J. 50, 1096-1121.
(23) Dunnett, C.W. (1964). New tables for multiple comparisons with a control. Biometrics 20, 482-491.
(24) Williams, D.A. (1971). A test for differences between treatment means when several dose levels are compared with a zero dose control. Biometrics 27, 103-117.
(25) Williams DA. (1972). The comparison of several dose levels with a zero dose control. Biometrics 28, 519-531.
(26) Holm, S. (1979). A simple sequentially rejective multiple test procedure. Scand. J. Statist. 6, 65-70.
ANNEX 1
The following definitions are applicable to this Guideline
LC50 (Median lethal concentration) is the concentration of a test substance that is statistically likely to kill 50% of exposed test organisms within a given time period. In this test the LC50 is expressed as a
mass of test substance per dry mass of the test soil or as a mass of test substance per unit area of soil.
LOEC (Lowest Observed Effect Concentration) is the lowest test substance concentration that has a statistically significant effect (p < 0.05) In this test the LOEC is expressed as a mass of test substance
per dry mass of the test soil or as a mass of test substance per unit area of soil. All test concentrations above the LOEC should normally show an effect that is statistically different from the control. Any
deviations from the above must be justified in the test report.
NOEC (No Observed Effect Concentration) is the highest test substance concentration immediately below the LOEC at which no effect is observed. In this test, the concentration corresponding to the
NOEC, has no statistically significant effect (p < 0.05) within a given exposure period when compared with the control.
ECx (Effect concentration for x% effect) is the concentration that causes an x% of an effect on test organisms within a given exposure period when compared with a control. For example, an EC50 is a
concentration estimated to cause an effect on a test end point in 50% of an exposed population over a defined exposure period. In this test the effect concentrations are expressed as a mass of test substance
per dry mass of the test soil or as a mass of the test substance per unit area of the soil.
Reproduction rate: Mean number of juvenile Collembola produced per a number of adults over the test period.
ANNEX 2
DETERMINATION OF THE MAXIMUM WATER HOLDING CAPACITY OF THE SOIL
The following method for determining the maximum water holding capacity of the soil has been found to be appropriate. It is described in Annex C of the ISO DIS 11268-2 (Soil Quality - Effects of
pollutants on earthworms (Eisenia fetida). Part 2: Determination of effects on reproduction (3)).
Collect a defined quantity (e.g. 5 g) of the test soil substrate using a suitable sampling device (auger tube etc.). Cover the bottom of the tube with a piece of filter paper fill with water and then place it on a
rack in a water bath. The tube should be gradually submerged until the water level is above to the top of the soil. It should then be left in the water for about three hours. Since not all water absorbed by the
soil capillaries can be retained, the soil sample should be allowed to drain for a period of two hours by placing the tube onto a bed of very wet finely ground quartz sand contained within a covered vessel (to
prevent drying). The sample should then be weighed, dried to constant mass at 105°C . The water holding capacity (WHC) can then be calculated as follows:
WHC (in % of dry mass) = 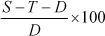
Where:
S = water-saturated substrate + mass of tube + mass of filter paper
T = tare (mass of tube + mass of filter paper)
D = dry mass of substrate
ANNEX 3
DETERMINATION OF SOIL pH
The following method for determining the pH of a soil is based on the description given in ISO DIS 10390: Soil Quality – Determination of pH (15).
A defined quantity of soil is dried at room temperature for at least 12 h. A suspension of the soil (containing at least 5 grams of soil) is then made up in five times its volume of either a 1 M solution of
analytical grade potassium chloride (KCl) or a 0.01 M solution of analytical grade calcium chloride (CaCl2). The suspension is then shaken thoroughly for five minutes and then left to settle for at least 2
hours but not for longer than 24 hours. The pH of the liquid phase is then measured using a pH-meter that has been calibrated before each measurement using an appropriate series of buffer solutions (e.g.
pH 4.0 and 7.0).
ANNEX 4
OVERVIEW OF THE STATISTICAL ASSESSMENT OF DATA (NOEC DETERMINATION)
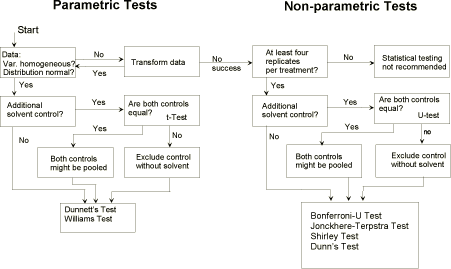
List of participants
(15 countries and 26 laboratories):
Canada:
Dr. G. Stephenson /Dr. Feisthauer, ESG International, Ontario, Canada
Dr. J. Princz, Environment Canada, Quebec, Canada
Czech:
Dr. J. Haufman, Masaryk University, Czech
Denmark:
Dr. P.H. Krogh, Danmarks Miljøundersøgelser, Danmark
Dr. J.J. Scott-Fordsmand, Danmarks Miljøundersøgelser, Danmark
France:
Prof. P. Vasseur, Faculty of Sciences, Univeristy of Metz, France
Germany:
Dr. S. Knaebe, GAB-Biotech, Germany
Dr. J. Römbke, ECT Oekotoxikologie, Germany
Dr. B.M. Wilke, Berlin University of Technology, Germany
Dr. F. Riepert, BBA, Germany
Hungary:
Prof. G. Bakonyi, Department of Zoology and Ecology, Hungary
Japan:
Prof. N. Kaneko, Yokohama National University, Japan
Nigeria:
Prof. M.A. Badejo, Obafemi Awolowo University, Nigeria.
Norway:
Dr. H. Stubberup, Jordfrorsk, Norway
The Netherlands:
Dr. C.A.M. van Gestel, Vrije Universitet, The Netherlands
Portugal:
Dr. J-P. Sousa, University of Coimbra, Portugal
Prof. A. Soares/Dr. M. Amorim, University de Aveiro, Portugal
Russia:
Dr. A Pokarzhevskii, Russian Academy of Science, Russia
Switzerland:
Dr. K. Becker van Slooten, Ecole PolyTechnique federale de Lausanne, Switzerland
Dr. M. Candolfi, RCC Ltd, Itingen, Switzerland
Thailand:
Dr. C.B. Iwai, Khon Kaen University, Thailand
United Kingdom
Dr. G. Frampton, University of Southampton, UK.
Dr. M. Riches, CEMAS,UK
Dr. M. Coulson, Syngenta, UK
USA
Dr. R. Kuperman, US Army, Edgewood Chemical Biological Center, USA
Dr. R. Lanno, University of Ohio, USA
| Top | | Front page |
Version 1.0 March 2005, © Danish Environmental Protection Agency
|