| Front page | | Contents | | Previous | | Next |
Model assessment of reductive dechlorination as a remediation technology for contaminant sources in fractured clay: Modeling tool
Appendix B Processes and modeling of TCE sequential dechlorination
B.1 Kinetics models
It seems that researchers agree on the use of Monod or Michaelis-Menten equations to simulate reductive degradation of TCE and its daughter products. It was not possible to find differences in the two kinetics models used in the literature so they will be considered to be similar in the rest of the report.
In these models, the degradation rate (mol.L-1.d-1) of the chlorinated ethenes has the following general form:

Where Ci is the concentration of the chlorinated ethene i (mol.L-1), µi is the maximum growth rate of i (d-1), X is the dechlorinating biomass concentration (cell.L-1), Y is the specific yield (cell.mol-1) and Ki is the half velocity coefficient of i (mol.L-1).
As the degradation of the TCE corresponds to production of DCE, the change in chlorinated ethenes can be described with the following equation:

Where ri is the degradation rate of the chlorinated ethene i and ri+1 is the production rate of chlorinated ethene I via the degradation of the higher chlorinated ethene.
Table B.1 - Reported values of kinetics parameters for dechlorination
Click here to see Table B.1
B.1.1 Competition between chlorinated ethenes
Some researchers have considered the competition between chlorinated ethenes for electron donors [Christ and Abriola, 2007, Chu et al., 2004, Cupples et al., 2004a, Cupples et al., 2004b, Garant and Lynd, 1998, Lee et al., 2004, Yu and Semprini, 2004 and Yu et al., 2005], among others. This competitive inhibition model is based on the assumption that a common catalyst is responsible for multiple dechlorination steps [Garant and Lynd, 1998]. Models accounting for this competition for electron donors are common in the recent literature, and it has been shown that it is possible to obtain a better fitting with a competitive than with a non-competitive model [Garant and Lynd, 1998 and Cupples et al., 2004a]. The general form for a competitive model, based on Monod kinetic is:

However the researchers do not agree on the types of competition, to be considered. In Chu et al. [2004], it has been shown that the dechlorination curves can have very different profiles depending on the inhibition considered in the model, therefore it is important to carefully consider which compounds are inhibitory in a system. Some researchers consider the competitive inhibition of all chlorinated ethenes at each dechlorination step [Cupples et al., 2004b and Garant and Lynd, 1998]. This means that TCE inhibits both DCE and VC dechlorination, DCE inhibits both TCE and VC degradation and VC inhibits both TCE and DCE degradation. Others considers that the inhibition constant of VC is very high compared to the half-velocity constant and is therefore not included in the model [Cupples et al., 2004b and Friis et al., 2007]. [Yu and Semprini, 2004 and Yu et al., 2005] suggest that the more chlorinated ethenes inhibit the degradation of the less chlorinated ethenes whereas the less chlorinated ethenes inhibit very weakly the dechlorination of the more chlorinated ethenes. The weak inhibition is therefore not included in the model. That means that TCE inhibits DCE and VC, and DCE inhibits only VC, whereas VC does not inhibit any degradation. Finally some studies consider different bacteria groups responsible for different dechlorination steps (this will be explained later), and in this case competitive inhibition occurs only between the chlorinated ethenes degraded by the same group [Lee et al., 2004]. The values of the inhibition coefficients are taken equal to the half-velocity constants in some studies [Garant and Lyng, 1998 Lee et al., 2004 Yu and Semprini, 2004 and Yu et al., 2005]. While in the others, it depends on the type of culture, used in the experiments (this will be detailed later). The reported values are presented in Table B.2.
Table B.2 - Reported values of inhibition coefficients
Click here to see Table B.2
B.1.2 Haldane inhibition
Haldane inhibition refers to the inhibition of a chlorinated compound on its own degradation rate. This phenomenon has been observed at high PCE and TCE concentrations [Yu and Semprini, 2004]. In this case, the inhibition term can be added in the equation, resulting in the following expression [Andrews, 1968]:

Where Khal,i is the Haldane inhibition constant of chlorinated ethene i.
In Yu and Semprini [2004], the model which takes into account Haldane inhibition (besides competitive inhibition) shows a better fit at high initial TCE concentration (around 4000 µM) than the competitive model only. The Haldane inhibition is applied to all chlorinated ethenes. The Haldane inhibition constants obtained from experimental data fittied with two different cultures are shown in Table B.3.
Table B.3 - Haldane inhibition constants obtained by data fitting [Yu and Semprini, 2004]
Haldane inhibition coefficient in µmol.L-1 |
culture |
PM |
EV |
K,halTCE |
900 |
900 |
K,halDCE |
6000 |
750 |
K,halVC |
7000 |
750 |
B.1.3 Limiting substrate conditions
In some studies the electron donor is simulated to be rate-limiting in the dechlorination reactions [Bagley, 1998, Christ and Abiola, 2007, Chu et al., 2004, Cupples et al., 2004a, Fennel and Gossett, 1998, Lee et al., 2004 and Yang and McCarty, 1998]. In order to take into account the rate limitation by an electron donor, the degradation rate is modified as follow:

Where CED is the electron donor (often hydrogen) concentration and KED is the electron donor half-velocity coefficient.
Other studies report the existence of a threshold concentration, which reflects the concentration under which there is no further dechlorination [Smatlak and Gossett, 1996 and Yang and McCarty, 1998]. In this case, the degradation rate can be written, [Christ and Abiola, 2007, Cupples et al., 2004a, Fennel and Gossett, 1998, Lee et al., 2004]:

Where C*ED is the electron donor threshold concentration.
In general, the values reported in literature refer to hydrogen as electron donor. These values can be found in Table B.4.
Table B.4 - Reported values for hydrogen half-velocity coefficient and threshold concentration
Hydrogen half-velocity coefficient and threshold concentration in µmol.L-1 |
|
Bagley 1998 |
Christ 2007 |
Chu 2004 |
Clapp 2004 |
Cupples 2004a |
Fennell 1998 |
Lee 2004 |
Smatlak 1996 |
Yang 1998 |
K,iPCE |
0.009 – 0.1 |
0.1 |
10 |
0.075 |
- |
0.5 |
0.5a |
0.1 |
- |
K,iTCE |
0.014 – 0.1 |
K,iDCE |
0.021 – 0.1 |
0.007 |
K,iVC |
0.017 – 0.1 |
CH* |
- |
0.002 |
- |
0.0015 |
0.0009 |
<0.0015 |
0.002 |
<0.002 |
0.002 |
B.1.4 Biomass growth
Degrading of TCE and its daughter products results in the growth of the dechlorinating bacteria. Hence ,depending on the experiment’s duration, it may not be reasonable to assume a constant biomass concentration. Therefore numerous studies take into account biomass growth during dechlorination. In this case, the change in biomass over time is given by:

Where X is the dechlorinating biomass concentration (cell.L-1), ri is the degradation rate of the chlorinated ethene i (mol.L-1.d-1), Y is the specific yield (cell.mol-1) and kd is the decay constant of the dechlorinating microorganisms (d-1).
The decay constant depends on the type of culture and the experiments/field conditions. The reported values are summarized in Table B.5.
Table B.5 - Reported values of decay rate in d-1
Click here to see Table B.5
VC degradation is assumed to occur cometabolically in some studies (PM culture does not grow on VC dechlorination) and therefore is not associated with biomass growth [Fennel and Gossett, 1998, Yu and Semprini, 2004 and Yu et al., 2005]. Finally, as explained previously, two dechlorinating groups are sometimes considered in the literature [Bagley, 1998, Christ and Abriola, 2007, Clapp et al., 2004]: one for PCE transformation to DCE via TCE and the other for DCE degradation to ethene via VC. In this case, each group grows on the specific chlorinated ethenes degradation.
B.1.5 Other competitive processes
Apart from competitive inhibition between chlorinated ethenes for electron donor, other competitive processes have been taken into account in the different studies. For example the competition for electron donor by other anaerobic microorganisms (than dechlorinating bacteria) can influence PCE/TCE dechlorination. If such microorganisms are present in the simulated system, they will also consume electron donor for growth. The hydrogenotrophic methanogenic group is often included in the studies to simulate competition among bacteria for hydrogen [Bagley, 1998, Chu et al., 2004, Clapp et al., 2004, Fennell and Gossett, 1998 and Lee et al., 2004]. In this case, the following equation, corresponding to bacteria growth, has been implemented in the model:

Where Xmeth is the concentration of methanogens microorganisms (cell.L-1), Ymeth is the specific yield (cell.mol-1), kmeth is the maximum rate of H2 utilization (mol.cell-1.d-1), CH is the hydrogen concentration (mol.L-1), CH*meth is the threshold for H2 use by hydrogenotrophic methanogens (mol.L-1), KH, meth is the half-velocity coefficient for H2 use by hydrogenotrophic methanogens (mol.L-1) and kd, meth is the decay constant (d-1).
The reported values of the different coefficients for methanogens are summarized in Table B.6.
Table B.6 - Reported values for methanogens
Reported kinetics properties of methanogens |
|
Unit |
Bagley 1998 |
Christ 2007 |
Clapp 2004 |
Fennell 1998 |
Lee 2004 |
Smatlak 1996 |
Yang 1998 |
Ymeth |
mgVSS.µmol-1 |
7.6*10-4 |
0.002 |
9.7*10-4 |
0.001 |
0.0014 |
- |
- |
kmeth |
µmol.(mgVSS.d)-1 |
163 |
1500 |
346 |
960 |
1500 |
kd,meth |
d-1 |
- |
0.05 |
0.015 |
0.024 |
0.05 |
C*H,meth |
µmol.L-1 |
- |
0.1 |
0.033 |
0.008 |
0.011 |
0.011 |
KH,meth |
µmol.L-1 |
0.96 |
0.5 |
6.1 |
0.5 |
0.5 |
1 |
- |
Finally, other types of bacteria can also compete for hydrogen, such as sulfate and iron reducers [Heimann et al., 2005], via the following redox reactions:

B.1.6 Fermentation process
The fermentation process, corresponding to the conversion of substrate into hydrogen, can be added in the model. Different substrates can be used to release hydrogen under anaerobic conditions, such as ethanol [Bagley, 1998 and Fennell and Gossett, 1998], pentanol [Christ and Abriola, 2007], butyric acid [Fennell and Gossett, 1998], propionic acid [Fennell and Gossett, 1998 and Yang and McCarty, 1998], lactic acid [Fennell and Gossett, 1998], glucose [Lee et al., 2004], benzoate [Yang and McCarty, 1998].
Examples of fermentation reactions are given below:
- Fermentation to acetate and H2
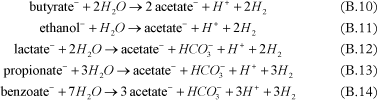
- Fermentation to propionate and acetate


Depending on the primary substrate, the fermentation step is simulating using a combination of these equations.
The rates of the different reactions are assumed to follow Monod kinetics. These reactions occur only when they are thermodynamically feasible (i.e when Gibbs free energy of the reaction is negative). This means that hydrogen concentration has to be low enough to allow fermentation to occur. The fermentation step is simulated in Fennell and Gossett [1998] with the following equation:

Where kdonor is the maximum specific rate of donor degradation (mol.cell-1.d-1), Xdonor is the donor fermenting biomass concentration (cell.L-1), KS, donor is the half-velocity coefficient for the donor (mol.L-1), S is the donor concentration (mol.L-1), S* is the hypothetical donor concentration that would result in ΔGrxn = ΔGcritical. The term S – S* allows the reaction only if it is thermodynamically feasible. However it was shown that substituting (S – S*) by S has a little impact on the results of the model in Fennell and Gossett [1998]. In the same way, the reaction rate in Christ and Abriola [2007] does not take S* into account.
The amount of hydrogen (or acetate) produced during fermentation step can be calculated with the stoichoimetric coefficients of reactions to . However it is difficult to relate hydrogen production directly to dechlorination because the produced hydrogen is consumed by different bacteria groups; and the dechlorinating biomass is only one of them.
The growth of the fermenting biomass can also be taken into account, as well as the consumption of acetic acid by methanogens [Bagley, 1998].
![Figure B.1 – Schematic overview of anaerobic dechlorination and interaction with fermentative, reductive and methanogenic bacteria from [Jørgensen et al., 2005]](images/s082.gif)
Figure B.1 – Schematic overview of anaerobic dechlorination and interaction with fermentative, reductive and methanogenic bacteria from [Jørgensen et al., 2005]
B.1.7 Conclusion
Anaerobic dechlorination consists of many different processes which interact with each other to form a complex biochemical system, especially taking into consideration the fermentation step, which leads to hydrogen production. These different processes have been studied to various degrees in the literature and there is not any single paper, which takes into account this entire system, from dechlorination to redox condition and substrate fermentation. Typically some processes (such as electron donor limitation and fermentation mainly) are disregarded in order to simplify the model formulation.
| Front page | | Contents | | Previous | | Next | | Top |
Version 1.0 July 2009, © Danish Environmental Protection Agency
|