| Bottom | | Front page |
Environmental Project no. 943, 2004
Evaluation of health hazards by exposure to BAM (2,6-Dichlorobenzamide) and risk characterisation of drinking water exposure
Contents
Preface
Sammenfatning og konklusion
1 General description
2 Toxicokinetics
3 Human toxicity
4 Toxicity, animal data
5 Regulations, limit values
6 Summary
7 Evaluation
8 Risk characterisation, drinking water exposure
9 References
Preface
The present report was prepared for the Danish Environmental Protection Agency by the Danish Toxicology Centre. The aim of the report was to make a health based risk assessment of BAM in drinking
water because of recent and frequent findings of BAM in water supply wells.
A steering committee followed the project and the final report has been elaborated in accordance to advise and comments made in the steering group at a meeting in August 2003 where a draft of the report
was discussed.
Participants of the meeting were:
Lis Keiding, The National Board of Health
Anders Carlsen, Medical Officer of Health
Elsa Nielsen, Danish Veterinary and Food Administration
Karl-Heinz Cohr, Danish Toxicology Centre (consultant)
Flemming A. Simonsen, Danish Toxicology Centre (consultant)
Nina Sørup Hansen, Danish Environmental Protection Agency
Christina Ihlemann, Danish Environmental Protection Agency
Poul Bo Larsen, Danish Environmental Protection Agency (chairman)
Pia Juul Nielsen, Danish Environmental Protection Agency
Susanne Rasmussen, Danish Environmental Protection Agency
Sammenfatning og konklusion
Baggrund
BAM (2,6-dichlorbenzamid) stammer fra herbiciderne Prefix og Casoron og er et nedbrydningsprodukt fra aktivstofferne dichlobenil (2,6-dichlorbenzonitril) og chlorthiamid (2,6-dichlor(thiobenzamid)) i
disse ukrudtsmidler. Midlerne har været brugt i perioden 1965 til 1997. Disse er nu forbudt, men BAM forekommer i stigende mængder i drikkevandsboringer. Formålet med denne rapport er at vurdere
BAM's toksicitet og at udarbejde en risikokarakterisering, som kan benyttes i forbindelse med vurdering af forurenede drikkevandsboringer.
Metode
Pesticidrevurderingsrapporter for dichlobenil fra Miljøstyrelsen samt fra U.S. Environmental Protection Agency dannede udgangspunkt for vurderingen af BAM's toksicitet. Supplerende litteratur blev
indhentet efter en datasøgning i en lang række relevante databaser: Toxcenter (Chemical Abstracts Service), Toxline (National Library of Medicine), Medline (National Library of Medicine), Embase
(Elsevier Science B.V.), Biosis (Biological Abstracts), IPA (American Society of Health-System Pharmacists), SciSearch (Thomson ISI), FSTA (International Food Information Service), ISTP/ISSHP
(Thomson ISI) og ISTPB (Thomson ISI). Originallitteratur blev så vidt muligt benyttet til dokumentation af kritiske effekter.
Dokumentationen er generelt baseret på ældre studier, som blev udført for over 30 år siden og ikke i henhold til gældende guidelines og retningslinier for god laboratoriepraksis (GLP). Der er således ofte
tale om ældre data, der ikke lever op til nutidens standard. Trods visse mangler i forsøgsdesign og udførelse vurderes forsøgene dog at være udført på en måde, der gør dem anvendelige til en
risikovurdering.
Fysisk-kemiske data
Rent BAM er et fast krystallinsk stof med et smeltepunkt på omkring 200 °C. Stoffet er vandopløseligt (2,7 g/l) og har en lav n-octanol-vand-fordelingskoefficient (0,77). Damptrykket er beregnet fra ca. 3,5
x 10-4 Pa til 4,4 x 10-3 Pa.
Forekomst
BAM har ingen kendt industriel anvendelse. BAM er et pesticidnedbrydningsprodukt, der hovedsageligt dannes i jorden. Herfra udvaskes det til grundvandet, hvor det anses for at være stabilt. Man har i
drikkevandsboringer fundet BAM i en gennemsnitskoncentration på 0,318 µg/l. De højeste koncentrationer, som blev målt, var i 14 µg/l i 2002 og 560 µg/l i perioden 1992-2001.
Indtagelse af BAM via drikkevandet anses for at være den mest sandsynlige humane eksponeringsvej. Da dichlobenil ikke (eller kun i ubetydelige mængder) nedbrydes til BAM i dyr eller mennesker, anses
indtagelse af dichlobenil ikke at bidrage til BAM eksponeringen.
Toksikokinetik
Efter indgift via munden optages BAM let fra mave-tarmkanalen. 48 timer efter en oral indgift til rotter, var over 60 % af dosis udskilt igen via urinen og 15 % via fæces. Der finder en udpræget
entero-hepatisk cirkulation sted. En del udskilles uomdannet i urinen sammen med en række metaboliter fremkommet ved glutation-konjugation og hydroxylering af den aromatiske ring. Der er ikke fundet
fælles metaboliter med dichlobenil.
Toksicitet
Den akutte giftighed af BAM anses for at være lav til moderat med orale LD50 –værdier på mellem 1144 mg/kg legemsvægt (lgv) og 2330 mg/kg lgv for mus og rotter.
I nogle nyere undersøgelser fra omkring 1990 fandt man, at det olfaktoriske epithel og specielt de Bowman'ske kirtler i næsen på mus og rotter var særligt følsomme overfor BAM. Dette understøttes af
autoradiografiske undersøgelser med radioaktivt mærket BAM, som viste en tilbageholdelse af radioaktivitet lokaliseret til de Bowman'ske kirtler. Milde reversible effekter i form af reduceret PAS-farvning
af de Bowman'ske kirtler blev observeret efter en enkelt intraperitoneal dosering med 25 eller 50 mg BAM/kg lgv til mus. Dette var mest udpræget i relation til den dorsale næsehule. Tyve dage efter
indgiften var PAS-farvningen normal. En reduceret PAS-farvning indikerer en nedsat forekomst af farvbart materiale så som glykogen, mucin, og glycoproteiner, og dermed en nedsættelse eller ændring af
cellefunktionen. Ved en dosis på 100 mg/kg lgv fandtes en klar beskadigelse af olfaktoriske epithel og de Bowman'ske kirtler. Det vides ikke hvordan gentagne doseringer af BAM vil påvirke
næseslimhinden, og om disse fund er prædikative for mennesker. En akut NOAEL (”no observed adverse effect level”) på 50 mg/kg er foreslået for dette studie, da den reducerede PAS-farvning ikke anses
for ”adverse”.
Undersøgelser af mutagene egenskaber (Ames test, micronucleustest, UDS test) har ikke vist tegn på mutagen effekt. BAMs kræftfremkaldende egenskaber er ikke fuldt undersøgt. Da BAM ikke anses for
at være mutagent, vil en potentiel kræftfremkaldende effekt være baseret på en non-genotoksisk mekanisme, og en tærskelværdi (NOAEL) vil således kunne forventes. BAM anses ikke for at være
reproduktionstoksisk, men heller ikke denne effekt er fuldt undersøgt.
Den toksikologiske profil af BAM viser nogen lighed med dichlobenils på trods af begrænset datamateriale og ældre undersøgelser af utidssvarende og utilfredsstillende kvalitet. Leveren synes at være det
kritiske målorgan for begge stoffer. Stofferne kan muligvis virke tumor-promoverende via en non-genotoksisk mekanisme sekundært til en organskadende effekt. Begge stoffer har affinitet til næseslimhinden,
hvor dichlobenil dog er klart mere toksisk potent overfor det olfaktoriske epithel og de Bowman'ske kirtler. BAM og dichlobenil har ikke fælles metaboliter in vivo men har formentlig fælles metaboliserende
enzymsystemer. For dichlobenil er ”Nul-effekt-niveauet” (NOAEL) angivet til 1.25 mg/kg/dag i et 2-års fodringsstudie med hunde, og det laveste effekt-niveau (LOAEL, ”lowest observed adverse effect
level”) var 8.75 mg/kg/dag baseret på lever-forandringer. Disse værdier er sammenlignelige med et andet 2-års studie, hvor hunde fik BAM opblandet i foderet. NOAEL i BAM studiet var 4.5 mg/kg/dag,
og LOAEL var 12.5 mg/kg/dag baseret på nedsat tilvækst hos både hanner og hunner og på forøget relativ levervægt hos hanner.
Kritisk effekt
NOAELs fra de fundne toksikologiske undersøgelser af kortere eller længere varighed ligger generelt i samme størrelsesorden (se tabel 5). Den kritiske effekt af BAM kommer generelt ikke til udtryk som
en specifik systemisk toksicitet, og LOAELs er ofte baseret på reduceret tilvækst. Baseret på den samlede toksikologiske datamængde foreslås en NOAEL spændvidde på 2.5 – 6 mg/kg lgv/dag til
risikokarakteriseringen.
Eksponeringsniveau
Indtagelse via drikkevandet anses for den mest relevante eksponeringsvej for BAM. En voksen person på 70 kg drikker ca. 2 l vand pr. dag (svarende til 0,03 l/kg lgv/dag), og børn i aldersgruppen 1 – 10
år drikker ca. 0.08 l/kg lgv/dag.
Hvis drikkevandet indeholder 0.1 µg BAM/l, som er den administrative grænseværdi for drikkevand, vil den teoretiske indtagelse være 0.003 µg BAM/kg lgv/dag for voksne og 0.008 µg/kg lgv/dag for børn
(tabel 6, gengivet nedenfor).
Table 6. Theoretical intake of BAM via drinking water
Water concentration of BAM |
Theoretical intake of BAM for an adult person weighing 70 kg |
Theoretical intake of BAM for 1 – 10 years old children |
0.1 µg/l |
0.003 µg/kg bw/day |
0.008 µg/kg bw/day |
1.0 µg/l |
0.03 µg/kg bw/day |
0.08 µg/kg bw/day |
10.0 µg/l |
0.30 µg/kg bw/day |
0.80 µg/kg bw/day |
14 µg/l |
0.42 µg/kg bw/day |
1.12 µg/kg bw/day |
560 µg/l |
16.8 µg/kg bw/day |
44.8 µg/kg bw/day |
Sikkerhedsmargin
Sikkerhedsmarginen, MOS (”Margin of Safety”), beregnes som forholdet mellem NOAEL og den humane BAM indtagelse:
MOS = NOAEL / BAM indtagelse
Den estimerede MOS er væsentlig større end 1000 i de fleste tilfælde (tabel 7 gengivet nedenfor).
Table 7. Margin of safety (MOS)
Water concentration of BAM |
MOS an adult person weighing 70 kg |
MOS for 1 – 10 years old children |
0.1 µg/l |
8.3 x 105 – 2.0 x 106 |
3.1 x 105 – 7.5 x 105 |
1.0 µg/l |
8.3 x 104 – 2.0 x 105 |
3.1 x 104 – 7.5 x 104 |
10.0 µg/l |
8.3 x 103 – 2.0 x 104 |
3.1 x 103 – 7.5 x 103 |
14 µg/l |
6.0 x 103 – 1.4 x 104 |
2.2 x 103 – 5.4 x 103 |
560 µg/l |
1.5 x 102 – 3.6 x 102 |
55.8 – 134 |
Konklusion
I forbindelse med at denne risikokarakterisering og dens MOS-beregninger efterfølgende benyttes i ved konkret risikohåndtering skal der endvidere tages hensyn til følgende:
Ved information og vurdering af tilfælde, hvor personer har været udsat for de ovennævnte niveauer, kan vurdering af de ovennævnte MOS-værdier direkte anvendes som udgangspunkt ved vurdering af
evt. sundhedsmæssige konsekvenser.
I forbindelse med at opretholde et tilstrækkeligt beskyttelsesniveau og med at udarbejde information til personer, der fortsat anvender vand med et vist niveau af BAM, skal der desuden tages hensyn til
sundhedsforebyggende- og politisk-administrative forhold.
For fremtidig brug af boringerne må der således tages hensyn til mulige fluktationer i BAM-niveauerne samt en evt. risiko for indsivning at øvrige forurenende stoffer. Dette skal ske således, at der i videst
mulig udstrækning tages hensyn til den overordnede politiske målsætning om at drikkevand skal være rent og fri for forurening.
Vurdering om fortsat anvendelse og udformning af evt. fremtidige dispensationer i forbindelse hermed vil således ikke alene være knyttet til den ovenstående sundhedsmæssige/ toksikologiske
risikokarakterisering. Vurderinger skal foretages ud fra en samlet afvejning af de ovennævnte aspekter således at både de vandforsyningsmæssige forhold og beskyttelse af befolkningens sundhed tilgodeses.
1 General description
1.1 Identity
1.2 Physical / chemical properties
1.3 Production and use
1.4 Environmental occurrence
1.5 Environmental fate
1.6 Human exposure
1.1 Identity
Name: |
2,6-Dichlorobenzamide |
Molecular formula: |
C7H5Cl2NO |
Structural formula: |
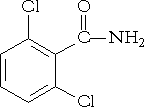 |
Molecular weight: |
190.03 |
CAS-no.: |
2008-58-4 |
Synonym: |
BAM |
1.2 Physical / chemical properties
Description: |
BAM is a rhombic crystalline solid. (BEILSTEIN, 2003) |
Purity: |
The purity of BAM ranges from 97 – 98 % (chemical catalogues). |
Boiling point: |
334.97 °C (estimated, adapted Stein and Brown Method) (U.S.Environmental Protection Agency, 2000) |
Melting point: |
The melting point ranges from 195 to 203 °C (BEILSTEIN, 2003) |
Density (crystal): |
1.44 g/cm³ (BEILSTEIN, 2003) |
Vapour pressure: |
3.26 x 10-5 mmHg (≈ 4.35 x 10-3 Pa) (estimated) (25 °C) (PHYSPROP, 2003)
1.19 x 10-5 mmHg (≈ 1.59 x 10-3 Pa) (estimated, Mackay Method) (25 °C) (U.S.Environmental Protection Agency, 2000)
4.75 x 10-6 mmHg (≈ 6.33 x 10-4 Pa) (estimated, modified Grain Method) (25 °C) (U.S.Environmental Protection Agency, 2000)
2.65 x 10-6 mmHg (≈ 3.53 x 10-4 Pa) (estimated, Antoine Method) (25 °C) (U.S.Environmental Protection Agency, 2000) |
Concentration of Saturated vapours: |
3.49 x 10-3 – 4.29 x 10-2 ppm (estimate based on vapour pressure) |
Vapour density: |
No data available |
Conversion factor: |
7.77 (25 °C) |
Flash point: |
115.0 +/- 44.2 °C (estimated) (REGISTRY, 2003) |
Flammable limits: |
No data available |
Autoignition temp.: |
No data available |
Solubility: |
Water (experimental): 2.7 g/l (20-25 °C) (Geyer et al., 1981) |
LogPoctanol/water: |
0.77 (experimental) (Nakagawa et al., 1992) |
Henry's constant: |
1.22 x 10-9 (atm x m³)/mole (estimated) (25 °C) (PHYSPROP, 2003) |
Koc: |
A Koc of 30 was measured for BAM on activated sludge (HSDB, 2002) |
pKa-value: |
No data available |
Stability: |
No data available |
Incompatabilities: |
No data available |
Odour threshold, air: |
No data available |
Odour threshold, water: |
No data available |
1.3 Production and use
BAM is a soil metabolite of the herbicides chlorthiamid (2,6-dichlorothiobenzamide) and dichlobenil (2,6-dichlorobenzonitrile), which were on the Danish market in the periods 1965 – 1980 and 1970 –
1996, respectively (Clausen et al., 2002, Elkjær et al., 2002).
There are no records on industrial preparation or use of BAM. Formerly, it may have been used in the synthesis of dichlobenil (HSDB, 2002). Chemical suppliers market the compound for research and development
purposes only.
1.4 Environmental occurrence
In recent investigations dichlobenil was found in soil samples in more than half of the investigated sites in concentrations of typically up to 100 µg dichlobenil/kg soil (Elkjær et al., 2002). Small amounts of BAM were also
detected. As it is assumed that the dichlobenil (and chlorthiamid) have not been used since 1997, this indicates that dichlobenil is still degraded and that BAM continues to infiltrate from the surface.
During the period 1992-2001 BAM was found in 22 % of all water samples analysed for BAM from water supply wells (Jørgensen, 2002). The threshold limit for pesticides in drinking water (0.1 µg/l) had been exceeded in
7.2 % of the cases. The mean and median concentration was 0.318 and 0.040 µg/l, respectively, and the highest concentration detected was 560 µg/l. A recent study concluded that in 2002 BAM was
encountered in 34 – 38 % of small water supply wells in four Danish counties (Brüsch, 2002). The highest concentration encountered was 14 µg/l.
1.5 Environmental fate
BAM is the major metabolite of dichlobenil.
If released to air, an estimated vapour pressure of 3.3 x 10-5 mm Hg at 25 °C indicates BAM will exist in both the vapour and particulate phases in the ambient atmosphere (HSDB, 2002). Vapour-phase BAM will be
degraded in the atmosphere by reaction with photochemically produced hydroxyl radicals; the half-life for this reaction in air is estimated to be 5.6 days. Particulate-phase BAM will be removed from the
atmosphere by wet and dry deposition.
If released to soil, BAM is expected to have very high mobility based upon a Koc of 30 (HSDB, 2002). Thus, BAM is expected to leach into groundwater through soil, and this has been confirmed in samples from water
supply wells analysed for BAM (Jørgensen, 2002).
Volatilization of BAM from moist soil surfaces is not expected to be an important fate process based upon an estimated Henry's Law constant of 1.2 x 10-9 (atm x m³)/mole (HSDB, 2002). Using a soil grab sample,
0.4%, 0.9%, 3%, and 6.1% of BAM (initial concentration 1 mg/l) degraded after 4, 12, 51, and 110 days of incubation, respectively, which suggests that BAM does not biodegrade rapidly in soil (HSDB, 2002).
If released into water, BAM is not expected to adsorb to suspended solids and sediment based upon the Koc (HSDB, 2002).
A BCF of 10 for Golden Ide fish suggests that bioconcentration in aquatic organisms is low (HSDB, 2002).
BAM does not biodegrade rapidly in water (HSDB, 2002). Hydrolysis is not expected to occur due to the slow rate of reaction for amide functional groups.
1.6 Human exposure
Occupational exposure to BAM may have occurred through inhalation and dermal contact with this compound at workplaces where BAM was produced or used (HSDB, 2002).
Ingestion of food and drinking water containing BAM is considered the most relevant source of exposure for the general population (HSDB, 2002).
Potential residues of dichlobenil in food crops, e.g. from imported fruits, are not likely and will not contribute to BAM exposure, as dichlobenil is not converted to BAM in vivo. Residues of BAM in
imported fruit from herbicide treated orchards are theoretically possible but are considered to be insignificant, because of the limited use of dichlobenil on edible crops. No analytical data are available
because the Danish Veterinary and Food Administration (DVFA) does not analyse for BAM or dichlobenil during their routine inspection for residues of pesticides.
Dermal exposure to BAM contaminated drinking water (e.g. by taking a bath) is a possible route of exposure. Although no information is available on dermal absorption of BAM, the dermal contribution of
bioavailable BAM is considered insignificant compared to that of the oral route. DermWin v1.42 is a model, which estimates the dermal permeability coefficient and uptake (U.S.Environmental Protection Agency, 2000). If the water contains 500 µg
BAM/l, if an adult person weighs 70 kg (equivalent to 1.8 m² surface area), and if a bath takes 15 min, then the theoretical dermal uptake is about 0.015 – 0.09 µg BAM/kg body weight (bw), or about 0.1 –
0.6 % of the oral uptake via drinking water (Table 1).
A low vapour pressure indicates a very low risk of inhalation of BAM.
Intake of contaminated drinking water is the most relevant route of exposure. Under normal conditions an adult person weighing 70 kg drinks about 2 litres of water every day, i.e. 0.03 l/kg bw/day (Miljøstyrelsen, 1992). Hot
weather and high physical activity will increase the intake. For children in the age group 1 – 10 years, a daily water intake of 0.08 l/kg bw/day can be taken as a 95-percentile (U.S.Environmental Protection Agency, 1997). If the water contains 0.1 µg
BAM/l, which is the administrative threshold limit in drinking water, the intake 0.003 µg BAM/kg bw/day and 0.008 µg/kg bw/day for adults and children, respectively (see Table 1). Exposure to the highest
concentration detected in a recent study, 14 µg/l (Brüsch, 2002), corresponds to an intake of 0.42 µg BAM/kg bw/day and 1.12 µg/kg bw/day for adults and children, respectively. Exposure to the unusual and extremely high
concentration detected in the period 1991 – 2001, 560 µg/l (Jørgensen, 2002), corresponds to an intake of 16.8 µg BAM/kg bw/day and 44.8 µg/kg bw/day for adults and children, respectively.
Table 1. Theoretical intake of BAM via drinking water
Water concentration of BAM |
Theoretical intake of BAM for an adult person weighing 70 kg |
Theoretical intake of BAM for 1 – 10 years old children |
0.1 µg/l |
0.003 µg/kg bw/day |
0.008 µg/kg bw/day |
14 µg/l |
0.42 µg/kg bw/day |
1.12 µg/kg bw/day |
560 µg/l |
16.8 µg/kg bw/day |
44.8 µg/kg bw/day |
2 Toxicokinetics
2.1 Absorption, distribution
2.2 Metabolism and excretion
2.3 Toxicological mechanisms
No human data are available and the following is based on results from animal studies.
2.1 Absorption, distribution
The metabolism and tissue distribution of 14C-labelled BAM (2,6-dichlorobenzamide-carbonyl-14C) was studied in Sprague-Dawley rats (Bakke et al., 1988a). C57B1 mice were used in autoradiography studies only. Rats
and mice received single doses of 14C-labelled BAM according to Table 2. Rats were given a single oral dose (5 mg) of labelled BAM followed by the collection of urine, bile, and faeces for metabolite
analysis. Mice were given an intravenous injection of 7 mg/kg labelled BAM and sacrificed at 10 minutes, 1, 4 and 24 hours, and 4 and 12 days for autoradiographic analysis. Juvenile rats were given an
intravenous injection of 2 mg/kg labelled BAM and sacrificed at 10 minutes, 4 and 24 hours. In addition one mouse and one juvenile rat were dosed intravenously with 7 or 2 mg/kg, respectively, of
14C-labeled BAM and killed 4 hours later, for special examination of the nasal region (microautoradiography).
Table 2. BAM metabolism and distribution studies in rats and mice (Bakke et al., 1988a)
Species, sex. |
Route and dose |
Sampling and tests |
13 rats (230-240 g), males |
Oral (5 mg 14C-labeled BAM, 0.54 µCi) |
Urine and faeces from 8 rats collected for 4 days and from 5 rats for 2 days. Animals killed and the
14C-content analysed in cadavers, selected tissues, urine and faeces. Urine analysed for metabolites |
5 rats (230-240 g), bile duct cannulated males |
Oral (5 mg 14C-labeled BAM, 0.48 µCi) |
Urine, faeces and bile collected for 2 days. Animals killed and the 14C-content analysed in cadavers,
selected tissues, urine, bile and faeces. Urine and bile analysed for metabolites |
3 rats (200-230 g), germfree males |
Oral (5 mg 14C-labeled BAM, 0.96 µCi) |
Rats kept in germfree isolator. Urine and faeces collected for 3 days. Animals killed and the
14C-content analysed in cadavers, selected tissues, urine and faeces. Urine analysed for metabolites |
2 rats (210 and 230 g), males |
Oral (mercapturic acid derivate of 14C-labeled BAM, isolated from urine
from rats dosed orally with 5 mg equivalents 14C-labeled BAM, 0.48 µCi) |
Urine collected for 2 days. Animals killed and the 14C-content analysed in cadavers, selected tissues
and urine. Urine analysed for metabolites |
Mice, females (20 g) |
Intravenous (7 mg 14C-labeled BAM/kg bw, 5 µCi) |
Animals killed after 10 min, 1, 4, and 24 hours, 4 and 12 days. Whole-body autoradiography. |
Mice, males (20 g) |
Intravenous (7 mg 14C-labeled BAM/kg bw, 5 µCi) |
Animals killed after 10 min, 4 and 24 hours. Whole-body autoradiography. |
Rats, males (75 g, juvenile) |
Intravenous (2 mg 14C-labeled BAM/kg bw, 5 µCi) |
Animals killed after 10 min, 4 and 24 hours. Whole-body autoradiography. |
1 mouse, female (20 g) |
Intravenous (7 mg 14C-labeled BAM/kg bw, 5 µCi) |
Animal killed after 4 hours. Microautoradiography of nasal region. |
1 rat, male (75 g, juvenile) |
Intravenous (2 mg 14C-labeled BAM/kg bw, 5 µCi) |
Animal killed after 4 hours. Microautoradiography of nasal region. |
BAM is extensively absorbed from the gastrointestinal tract, because a total of 85 percent of the radioactivity was found in the bile, urine, and tissues 48 hours following the oral administration of BAM to
bile duct cannulated rats (total recovery about 90 %). Non-cannulated control rats excreted much more 14C from BAM in the urine as did the cannulated rats indicating that entero-hepatic circulation was
occurring. About 13 – 23 % was excreted with the faeces. Over 60 % of the radioactivity was eliminated via the urine of non-cannulated rats after 48 hours and over 70 % after 96 hours. The excretion of
about 7 times more radioactivity in the faeces of non-cannulated compared to cannulated rats indicate that biliary metabolites were precursors to the faecal radioactivity.
Whole body autoradiography and nasal microautoradiography of rats and mice showed the chemical to be homogeneously distributed throughout the body at times between 10 minutes and 4 hours post
injection. Radioactivity appeared in the liver, tracheobronchial mucosa, esophageal mucosa, and adrenal and kidney cortices between 24 hours and 4 days post injection. A marked retention of radioactivity
was associated with the lateral nasal gland (Steno's gland) that persisted for 4 days. The radioactivity in the olfactory mucosa persisted 12 days after injection, and a marked retention of radioactivity was
observed in skeletal muscles for up to 12 days after injection.
Contrary to animals killed 10 minutes after injection much of the radioactivity of the olfactory mucosa and in the contents of the large intestine could not be extracted. The nasal microautoradiography
revealed high level of non-extractable radioactivity in Bowman's glands situated beneath the olfactory epithelium, especially in the mouse but in the rat the level also exceeded the background level.
2.2 Metabolism and excretion
Plant metabolism studies on apples and grapes indicate that BAM is the major residue after treatment with dichlobenil (U.S.Environmental Protection Agency, 1998). In addition, a small residue fraction was identified as 4-hydroxy-BAM. US EPA has
established tolerances for the combined residues of the herbicide dichlobenil and its metabolite BAM in or on (U.S.Environmental Protection Agency, 2001):
Apples and pears: |
0.5 ppm |
Blueberries, grapes, and stone fruits: |
0.15 ppm |
Blackberries, cranberries, and raspberries: |
0.10 ppm |
Today, no corresponding tolerances are available in Denmark or as harmonised maximum residue levels (MRLs) in the European Union.
BAM was not found as a metabolite or transitory intermediate of dichlobenil in ruminant or poultry studies (U.S.Environmental Protection Agency, 1998). In general, arylnitriles are metabolised to only a small extent by reactions involving the cyano group
(Griffiths et al., 1966):
Ar•CN → Ar•CO•NH2 → Ar•COOH
The extent to which this reaction occurs depends on the nature of the substituents in the benzene nucleus. Early studies with radiolabeled dichlobenil indicate that less than 2 % is metabolised in vivo to BAM
plus 2,6-dichlorobenzoic acid after oral administration to rats or goats (Bakke et al., 1988b, Griffiths et al., 1966).
As mentioned earlier the metabolism of 14C-labeled BAM (2,6-dichlorobenzamide-carbonyl-14C) was studied in Sprague-Dawley rats (Bakke et al., 1988a). The metabolism was not studied in mice. The major metabolites
formed in rats were identified by mass spectrometry and proton magnetic resonance spectrometry.
Oral doses of 14C-labeled BAM were excreted by rats as (see also Table 3):
- unchanged BAM (about 25 % in urine)
- two monohydroxy BAM's: 3-hydroxy-2,6-dichlorobenzamide (CAS No. 22818-74-2) and 4-hydroxy-2,6-dichlorobenzamide (CAS No. 7446-00-6) (about 5 % combined in urine).
- 2-chloro-5-hydroxy-6-(methylthio)benzamide (or 6-chloro-3-hydroxy-2-(methylthio)benzamide, CAS No. 117415-43-7) (about 6 % in urine)
- 2-chloro-5-hydroxy-6-[S-(N-acetyl)cysteinyl]benzamide (mercapturic acid) (or N-acetyl-S-[2-(aminocarbonyl)-3-chloro-6-hydroxyphenyl]-L-cysteine, CAS No. 117384-43-7) (about 23 % in urine)
- The metabolites isolated from urine and bile from rats dosed with BAM are also listed in Table 3. The theoretical metabolite, 2,6-dichlorobenzoic acid was not identified. Faecal metabolites were not characterised.
Table 3. Metabolites isolated from urine and bile from rats dosed with BAM showing the percentages of the oral doses excreted as each metabolite (Bakke et al., 1988a)
Metabolite |
Non-cannulated |
Bile duct cannulated |
Germ free |
|
urine |
urine |
bile |
Urine |
BAM |
25 |
16 |
13 |
16 |
Monohydroxylated BAM |
5 |
3 |
Not detected |
5 |
2-chloro-5-hydroxy-6-[S-(N-acetyl)cysteinyl] benzamide (mercapturic acid) |
23 |
9 |
(14)¹ |
25(17)² |
2-chloro-5-hydroxy-6-thiolobenzamide |
Not detected |
Not detected |
6 |
Not detected |
2-chloro-5-hydroxy-6-(methylthio)benzamide |
6 |
Not detected |
Not detected |
Not detected |
Not characterised (approx.) |
3 - 12 |
0 |
0 |
20 –22 |
1 Present as mercapturic acid pathway metabolites
2 Cysteine conjugate
Enterohepatic circulation and metabolism by intestinal microflora were involved because germ free and cannulated rats excreted neither 2-chloro-5-hydroxy-6-thiolobenzamide nor
2-chloro-5-hydroxy-6-(methylthio)-benzamide in the urine, whereas 2-chloro-5-hydroxy-6-thiolobenzamide, a presumable precursor for 2-chloro-5-hydroxy-6-(methylthio)benzamide, was excreted in the
bile. Intestinal microfloral metabolism was involved in the formation of 2-chloro-5-hydroxy-6-(methylthio)benzamide, and the mercapturic acid served as a precursor.
The urinary metabolite profile of BAM in rats did not resemble that of dichlobenil (2,6-dichlorobenzonitrile) (Bakke et al., 1988b). The metabolic pathway(s) of dichlobenil is comparable to that of BAM, but as for the -CONH2
group of BAM the -CN group of dichlobenil is not changed. The reaction:
Ar•CN → Ar•CO•NH2 (→ Ar•COOH)
resulting in common metabolites does apparently not occur to a measurable degree. A high fraction of absorbed BAM is excreted unchanged in the urine (about 25%) whereas dichlobenil is apparently not
eliminated unchanged via the urine. Based on the metabolites identified, two general metabolic pathways may be proposed: (1) hydroxylation at the 3 or 4 position of the phenyl moiety followed by sulphation
or glucoronidation and (2) conjugation with glutathione through displacement of the chlorine atom followed by the mercapturic acid pathway.
Lactating goats were dosed with [U-phenyl]14C-BAM at a dose level of 10 ppm for five days (U.S.Environmental Protection Agency, 1998). The primary residue found in milk, kidney, fat, and muscle was unchanged BAM. The major residue found in
liver was the glutathione conjugate 6-chloro-3-hydroxy-2-mercaptobenzamide.
Laying hens were also dosed with [U-phenyl]14C-BAM at a dose level of 10 ppm for five days (U.S.Environmental Protection Agency, 1998). The primary residue found in all matrices collected was unchanged BAM.
2.3 Toxicological mechanisms
The toxicological mechanism is unknown.
3 Human toxicity
No data have been found.
4 Toxicity, animal data
4.1 Acute and short term toxicity
4.2 Long term toxicity
4.3 Reproductive and developmental effects
4.4 Mutagenic and genotoxic effects
4.5 Carcinogenic effects
Test results from studies performed over 30 years ago have been available. The tests do not fully comply with current internationally accepted guidelines, and they have not been performed in accordance
with current requirements to good laboratory practice (GLP). Despite the lacks in study design and performance, the studies, in general, appear to be well performed and are considered valid for a
toxicological assessment of BAM.
Because of lack of actually measured data default values have sometimes been used in this assessment. WHO lists some default animal weight values in a table showing approximate relation of parts per
million (ppm) in the diet to mg/kg body weight per day (WHO, 1987). The values were originally reported in 1954 (Lehman, 1954).
4.1 Acute and short term toxicity
Toxicological studies have been performed with BAM, since BAM is the major residue in plants treated with dichlobenil, and is consumed in the food supply (U.S.Environmental Protection Agency, 1998). It is not an animal metabolite.
Wistar rats (5 of each sex per group) were administered a single dose of BAM (purity not recorded) in 1% w/v aqueous tragacanth by gavage at the following doses: 1000, 2150, 4640 and 10000 mg/kg
body weight (bw) (Kemp and van der Linde, 1967). It is an old study without GLP status, and it does not fully live up to today's standard. The surviving rats were observed for 14 days. Deaths occurred 3 – 72 hours after treatment. The
acute oral LD50 value was 1470 (951 – 2270) mg/kg for males and 2330 (1430 – 3780) mg/kg for females. The symptoms began to appear 10 min after dosage and disappeared completely 24 – 28 hours
later. Symptoms from the CNS dominated the clinically appearance. At the lowest dose administered (1000 mg/kg), the symptoms included: one death, prostrate, limbs relaxed, righting reflex absent but
corneal reflex present, miosis, and rapid but shallow respiration. High doses were followed by progressive narcosis and death. No autopsy was performed.
The acute oral LD50 of BAM in mice was 1538 and 1144 mg/kg in males and females, respectively (U.S.Environmental Protection Agency, 1998).
A group of Wistar rats (5 of each sex per group) received BAM (purity not recorded) in 1% w/v aqueous tragacanth by gavage for eight consecutive days (Kemp and van der Linde, 1967). The study is not a guideline study with GLP
status. It was designed as a dose range finding study with the following dose levels: 6.25, 12.5, 25, 50, 100, 200, 400, 800 and 1600 mg/kg bw/day. Deaths occurred between day 2 and 9 and were
associated with loss of righting reflex, corneal and pain reflexes, mydriasis, shallow respiration, bradycardia and hypothermia. The subacute oral LD50 value was estimated to be 677 (410 – 1117) mg/kg for
males and 574 (363 – 907) mg/kg for females. A significant decrease in skeletal muscle tone was observed from 25 mg/kg bw/day for males and from 100 mg/kg bw/day for females. The hypotonus was
accompanied by impaired righting reflex, miosis, hypothermia, moderate analgesia and rapid but shallow respiration. These symptoms appeared about 15 min after the treatment and peaked 2 hours later.
Only partial regression of symptoms was observed at dose levels above 200 mg/kg bw/day. An increase in liver weights was observed in males at levels above 50 mg/kg bw/day. The weights of adrenal
glands were increased at 200 mg/kg bw/day in males and at 400 mg/kg bw/day in both sexes. Animals of 6.25 and 12.5 mg/kg bw/day group, as well as groups severely affected by treatment, 800 and
1600 mg/kg bw/day, were not included in the autopsy. No histopathological changes related to treatment were observed. The growth and food consumption was affected at 400 mg/kg bw/day (800 and
1600 mg/kg bw/day groups were not monitored).
Critical effect: Reduction in skeletal muscle tone
LOAEL: 25 mg/kg bw/day (males) and 100 mg/kg bw/day (females)
NOAEL: 12.5 mg/kg bw/day (males) and 50 mg/kg bw/day (females)
Wistar rats (10/sex/dose) were exposed to BAM for 13 weeks at dietary levels of 0, 50, 180, 600 or 2300 ppm (U.S.Environmental Protection Agency, 1998). The study is not performed according to current guidelines (e.g. with respect to
investigated parameters) and does not have GLP status.
Critical effects: Decreased body weight gain and food efficiency, increased blood urea nitrogen, and reduced coagulation times.
LOAEL: 600 ppm (49 mg/kg/day).
NOAEL: 180 ppm (14 mg/kg/day)
BAM (purity 97 % w/w) was administered orally to dogs (6 (control) or 4 dogs/sex/group) at dietary concentrations of 0, 100, 300 and 2000 ppm for a period of 13 weeks (Walker, 1967). The study appears to be well
performed, though not according to present guidelines (e.g. no ophthalmoscopic examination or tests for clotting potential), and it does not have GLP status. Dogs in the highest dose group lost body weight
and bodily condition after 3 – 5 weeks of exposure. Increased liver weights were observed in 300 and 2000 ppm level groups, but the effect was only statistically significant in females. The food consumption
was monitored but not stated in the report. The high dose females had a decrease in serum urea and an increase in serum alkaline phosphatase activity and 2-globulin after 13 weeks exposure. Liver function
tests carried out in control and high dose groups did not show any difference between the two groups.
Critical effect: Increased liver weights
LOAEL: 300 ppm (approximately 7.5 mg/kg bw/day (WHO, 1987)).
NOAEL: 100 ppm (approximately 2.5 mg/kg bw/day (WHO, 1987)).
The toxic effect of BAM was examined in the nasal passages of C57Bl mice following single intraperitoneal (ip) injections of BAM dissolved in DMSO (0(vehicle), 25, 50 or 100 mg/kg bw) (Brittebo et al., 1991). Two or three
animals from almost every dose group were killed after 8 hours, 1 day, 3 days or 20 days. No clinical signs of toxicity were observed in any of the BAM treated mice. After administration of 25 and 50
mg/kg bw there was an indication of disturbed function of the Bowman's glands (decreased PAS-staining) in the dorsal meatus (not lateral) at the 8 hour and 1 day killings, but not after 3 days (50 mg/kg
only). Twenty days after the 25 and 50 mg/kg ip injections, the PAS-staining intensity of the Bowman's gland was similar to the controls. At 100 mg/kg necrosis of the Bowman's glands and the
neuroepithelium was observed, especially in the dorsomedial aspects of the olfactory region at the 1 day and 3 days killings. There was however signs of restitution of the olfactory epithelium and lamina
propria (with Bowman's glands) 3 days after 100 mg/kg, but no animals were observed for a longer period at the 100 mg/kg dose level. Debris was observed in the nasal passages following ip exposure to
100 mg/kg. No lesions were observed in other parts of the nasal cavity including the respiratory or squamous mucosa in the lateral nasal glands, maxillary nasal glands or vomeronasal organ, and there were
no morphological lesions observed in the liver. It was proposed that the lesions are due to a local cytochrome P450-dependent activation and that the more extensive toxic effects of chlorthiamid and
dichlobenil in the olfactory mucosa are mediated by common or closely related metabolites, different from those of BAM.
Critical effect: Necrosis of the Bowman's glands and the neuroepithelium<
LOAEL: 100 mg/kg bw (ip)
NOAEL: 50 mg/kg bw (ip)
LOEL: 25 mg/kg bw (ip) (decreased PAS-staining)
NOEL: Could not be established because of decreased PAS-staining of Bowman's glands in the dorsal meatus, an apparently reversible effect.
4.2 Long term toxicity
In a combined chronic toxicity/carcinogenicity study BAM (purity unknown) was given to Crl CD rats (35/sex/dose) for 106-107 weeks at dietary levels of 0, 60, 100, 180 or 500 ppm (U.S.Environmental Protection Agency, 1998, Wheldon et al., 1971). The study appears
to be well performed, though not according to current guidelines (too small group size, incomplete haematology, serum chemistry, urine analysis and histopathology), and it does not have GLP status.
Although the study was reported as a combined chronic toxicity/carcinogenicity study, a group size of 35/sex/dose is too small (min. 50/sex/dose) for an adequate evaluation of carcinogenicity.
The dietary levels were equivalent to an average BAM intake of 0, 2.2, 3.6, 6.5, or 19 mg/kg bw/day in males and 0, 2.8, 4.7, 8.5, or 25 mg/kg bw/day in females calculated from nominal dietary
concentrations over 106-107 weeks. When correlated for mean analysed concentrations, the average BAM intake was 0, 2.0, 3.4, 6.0, or 17 mg/kg bw/day in males and 0, 2.6, 4.4, 7.9, or 23 mg/kg
bw/day in females. As usual in this kind of studies, the dosages (based on mg/kg bw/day) fell during the first 26 - 30 weeks to about one-third of their initial values, and thereafter remained essentially
constant.
At the highest dose level a statistically significant decrease in mean body weight gains was observed in both males and females (11 % and 20 % less than controls, respectively, at week 52). Food
consumption and food conversion efficiency of females was marginally reduced at the highest dose level.
No clinical findings or macroscopic changes were considered related to treatment. The relative liver weights were increased in a dose-related manner in females and at the highest dose level in males. The
effect was only statistically significant for the females at the highest dose level, and this was consistent with greater degree of histological changes (slightly increased severity of fat deposition) in the livers of
females. There were no treatment-related changes in liver enzyme activities. A non-progressive depression of packed cell volume, erythrocyte counts and haemoglobin was observed in high dose males and
female (not examined for low or intermediate dose groups). BAM produced an increased incidence of hepatoma in females at 500 ppm. The frequency of rats bearing hepatoma of the rats subjected to liver
histology was 7.4, 0, 6.3, 7.7 and 2.9 % for males and 0, 0, 0, 3.1 and 14.3 % for females in treatment groups 0, 60, 100, 180 and 500 ppm, respectively (Table 4, section 4.5). No hepatocarcinoma was
found. The U.S. EPA determined that the high dose female rats demonstrated an increased incidence of hepatoma (14 %), which was of borderline significance (p< 0.049). However, a histopathological
examination of livers from the rats needs to be reevaluated before a formal determination can be made on the carcinogenic potential of BAM. In addition the group size appears to be too small for an
adequate evaluation of carcinogenicity.
Critical effect: Decreased body weight gain in males and in females.
LOAEL was set at 19 (17 based on analysed feed data) mg/kg/day.
NOAEL was 6.5 (6.0 based on analysed feed data) mg/kg/day.
In a chronic toxicity study in dogs, BAM (purity 97 % w/w) was fed to beagle dogs 4/sex/dose for two years at dietary levels of 0 (control), 60, 100, 180, or 500 ppm (approximately 0, 1.5, 2.5, 4.5, or
12.5 mg/kg/day) (U.S.Environmental Protection Agency, 1998, Wilson and Thorpe, 1971). The study report is very brief. It is not performed according to present guidelines (no food consumption data, incomplete serum chemistry study, and no ophthalomological examination),
and it does not have GLP status. Decreased body weight gain in males (58 % of controls at 2 years) and in females (29% of controls at 2 years) was observed at 500 ppm. At the 500 ppm level, the males
had an increased relative liver weight. The females had reduced relative kidney and liver weights at and above 60 ppm, but this was not considered related to treatment.
Critical effect: Decreased body weight in both sexes and increased relative liver weight in males.
LOAEL: 12.5 mg/kg/day
NOAEL: 4.5 mg/kg/day
4.3 Reproductive and developmental effects
New Zealand white rabbits (16/dose group) were given BAM at dosing levels of 0, 10, 30, or 90 mg/kg/day by oral gavage on gestational days (GDs) 7 – 19 (U.S.Environmental Protection Agency, 1998). Maternal toxicity was observed at 30 and 90
mg/kg/day. Increased moribundity was observed (2 animals in both the middle and high dose groups compared to 0 in the control). At 90 mg/kg/day, the body weight decreased non-significantly (93%-95%
of control) on GDs 13 - 19; weight gain decreased significantly during the dosing period; food consumption decreased significantly (51% of controls) during the dosing period; and the number of abortions
increased (3 animals compared to 0 in the control). Post-dosing, compensatory body weight and food consumption increases above controls were observed.
Developmental toxicity was observed at 90 mg/kg/day as a non-significant decrease (94% of controls) in foetal body weight, which was outside the historical control range. BAM was not teratogenic in this
study.
Critical maternal effect: Increased mortality
Critical foetal effect: Decrease in foetal body weight and body weight gain
LOAEL, maternal: 30 mg/kg/day
LOAEL, foetal: 90 mg/kg/day
NOAEL, maternal: 10 mg/kg/day
NOAEL, foetal: 30 mg/kg/day
In a three generation reproduction study with two litters per generation, BAM (purity 99.5 %, phenol 0.1%) was given to 10 male and 20 female Long-Evans rats per dose group at dietary levels of 0, 60,
100, or 180 ppm (equivalent to about 0, 3-6, 5-10, or 9-18 mg/kg bw/day (WHO, 1987)) (Hine, 1970, U.S.Environmental Protection Agency, 1998). The study is not performed according to current guidelines (e.g. no diet analysis or food consumption data) and does not have
GLP status. The study appears to be well performed, but individual animal data could not be provided. A significant decrease in weights of weanlings (85 – 88 % of the control animals at day 21 only for
F2a, F3a and F3b generations) was observed at the highest dose level. The terminal weight of the last parent generation (F2b) females was significant less at the highest dose level compared to the controls
(94% of the control animals). The F3b weanlings were autopsied, and at 180 ppm level the relative kidney weight of females and relative liver weights of both sexes were increased. The 100 ppm females
also had a marginal, but statistically significantly increased relative liver weight.
Critical effect: Increased relative liver weight
LOAEL: 100 ppm (5-10 mg/kg/day)
NOAEL: 60 ppm (3-6 mg/kg bw/day)
4.4 Mutagenic and genotoxic effects
Three mutagenicity studies have been assessed by the US EPA and reported in the Reregistration Eligibility Decision (RED) document for dichlobenil. No details are available. No other mutagenicity studies
have been identified for BAM.
“BAM was negative for inducing reverse gene mutation (his- to his+) in TA strains of Salmonella typhimurium exposed, in the absence and presence of mammalian metabolic activation (rat S9 mix), up to
5000 µg/plate” (U.S.Environmental Protection Agency, 1998).
“BAM was negative for inducing repair of DNA damage as measured by unscheduled DNA synthesis (UDS), as determined by net nuclear silver grain count in primary rat hepatocytes, exposed up to
cytotoxic doses (1000 µg/ml)” (U.S.Environmental Protection Agency, 1998).
“A mouse micronucleus assay using a single dose of BAM (250 mg/kg) was negative. This dose was selected based on a single dose study in which the group of mice treated at 250 mg/kg displayed mild
neurotoxic effects (lethargy and ataxia); the group treated at 500 mg/kg were severely affecte (becoming comatose), and the groups treated at 1000 mg/kg and higher died in extremis “ (U.S.Environmental Protection Agency, 1998). The route was not mentioned.
4.5 Carcinogenic effects
As mentioned above, a combined chronic toxicity/carcinogenicity study has been performed with BAM. It was administered to Crl CD rats (35/sex/dose) for 106-107 weeks at dietary levels of 0, 60, 100,
180 or 500 ppm (Wheldon et al., 1971). The frequency of rats bearing hepatoma of the rats subjected to liver histology was 7.4, 0, 6.3, 7.7 and 2.9 % for males and 0, 0, 0, 3.1 and 14.3 % for females in treatment groups 0, 60,
100, 180 and 500 ppm, respectively (Table 4). No hepatocarcinoma was diagnosed, or clear distinction between hepatocellular preneoplastic foci, adenomas and carcinomas was not made. Not all animals
were subjected to liver histology. The incidence of this common tumour type among historical controls of this particular strain of rat has not been reported. The study is inadequate (e.g. the group size is too
small) for a full evaluation of carcinogenicity.
As part of the review process of US EPA, clarification of the tumour types and data on the stability/homogeneity of the test compound was requested (U.S.Environmental Protection Agency, 1998). This information was submitted to US EPA. With this
additional data from reclassified liver slides, the Agency determined that the high dose female rats demonstrated an increased incidence of adenomas (14%), which was of borderline significance (p< 0.049).
However, a formal US EPA assessment of the carcinogenic potential of BAM awaits peer review results of the histopathological examination of livers from the rats.
Table 4. Hepatoma
Dosage (ppm) |
Males |
Females |
|
No. of rats subjected to liver histology (*) |
No. of rats with hepatoma (*) |
Pct. of rats with hepatoma (*) |
No. of rats subjected to liver histology (*) |
No. of rats with hepatoma (*) |
Pct. of rats with hepatoma (*) |
0 |
27 (7) |
2 (1) |
7.4 (14.3) |
26 (8) |
0 (0) |
0 (0) |
60 |
29 (10) |
0 (0) |
0 (0) |
28 (10) |
0 (0) |
0 (0) |
100 |
32 (10) |
2 (1) |
6.3 (10) |
28 (10) |
0 (0) |
0 (0) |
180 |
26 (10) |
2 (0) |
7.7 (0) |
32 (10) |
1 (0) |
3.1 (0) |
500 |
35 (15) |
1 (0) |
2.9 (0) |
35 (20) |
5 (4) |
14.3 (20) |
* Rats sacrificed at 107 weeks only
5 Regulations, limit values
The threshold limit in drinking water of each individual pesticide or their relevant metabolites, degradation and reaction products is 0.1 µg/l (Council of the European Union, 1998). The sum of all individual pesticides detected and quantified in the
monitoring procedure should not exceed 0.5 µg/l.
BAM is listed on the following international/national lists (CHEMLIST, 2003):
- EINECS (EINECS No.: 217-918-4) - Annex to Official Journal of the European Communities, 15 June 1990
- SWISS (SWISS No.: G-4611) - Giftliste 1 (List of Toxic Substances 1, as amended in 2003). Toxic Category 4 (“acute oral lethal dose of 500 - 2000 mg/kg”).
- AICS - Australian Inventory of Chemical Substances, June 1996 Ed.
BAM is listed on the Danish Advisory List for Self-Classification of Substances with the advisory classification: Xn;R22 (“Harmful if swallowed”) (Danish Environmental Protection Agency, 2001).
BAM is not on the list of dangerous substances (Annex I of Directive 67/548/EEC). This is the case for dichlobenil (Index No. 608-015-00-X), which is classified as:
Xn; R21(”Harmful in contact with skin”)
N; R51/53 (”Toxic to aquatic organisms, may cause long-term adverse effects in the aquatic environment”)
No TDI (“tolerable daily intake”), ADI (“accepted daily intake”), MRL (“maximum residue limit”) values have been proposed by the European Commission, the Joint Expert Committee on Food Additives
(JECFA), Codex or WHO for BAM or dichlobenil.
The US EPA has established tolerances for the combined residues of the herbicide dichlobenil and its metabolite BAM in or on (U.S.Environmental Protection Agency, 2001):
Apples and pears: |
0.5 ppm |
Blueberries, grapes, and stone fruits: |
0.15 ppm |
Blackberries, cranberries, and raspberries: |
0.10 ppm |
The Agency has evaluated the chronic dietary risk associated with the consumption of the dichlobenil metabolite BAM based on tolerance level residues, 100 % crop treated data and estimated BAM
residue values for meat and milk (U.S.Environmental Protection Agency, 1998).
The RfD (“reference dose”) for BAM was determined to be 0.015 mg/kg/day based upon the NOAEL from a chronic toxicity study in dogs and an uncertainty factor of 300 (100 x 3). The uncertainty
factor of 100 accounts for the inter-species extrapolation and intra-species variability. The uncertainty factor of 3 is to compensate for the lack of an acceptable BAM reproduction study. A chronic drinking
water risk was assessed for dichlobenil.
The RfD for dichlobenil was determined to be 0.013 mg/kg/day based upon a NOAEL of 1.25 mg/kg/day from a two-year dog feeding study and an uncertainty factor of 100.
6 Summary
The documentation is mainly based on old studies performed over 30 years ago that do not fully comply with current internationally accepted guidelines and GLP. Some study reports have not been
available, and results have been cited from the Reregistration Eligibility Decision (RED) document for dichlobenil (U.S.Environmental Protection Agency, 1998).
Description
BAM is a crystalline solid with a melting point of about 200 °C. It is soluble in water (2.7 g/l), and it has a low n-octanol-water partition coefficient (0.77). The vapour pressure of BAM has been estimated
by different methods and ranges from 3.5 x 10-4 Pa to 4.4 x 10-3 Pa.
Use
BAM is a soil metabolite of the herbicides chlorthiamid (2,6-dichlorothiobenzamide) and dichlobenil (2,6-dichlorobenzonitrile), which were on the Danish market in the periods 1965 – 1980 and 1970 –
1996, respectively. BAM has no known industrial uses.
Environment
BAM has been found in water supply wells. In the period 1992-2001 about 22 % of all water samples analysed for BAM contained BAM in a mean and median concentration of 0.318 and 0.040 µg/l,
respectively. The highest concentration detected was 560 µg/l. In 2002 BAM was encountered in 34 – 38 % of small water supply wells in four Danish counties, and the highest concentration encountered
was 14 µg/l. BAM is considered stable in water. It is not expected to biodegrade or hydrolyse rapidly in water, and volatilisation from water is not expected to play a role either due to a low estimated
Henry's Law constant (1.22 x 10-9 (atm x m³)/mole.
Human exposure
Intake is considered the most important route of human exposure to BAM (i.e. primarily drinking water containing BAM). Potential dichlobenil exposure may only contribute to BAM exposure to a very
limited extent due to minor metabolic conversion of dichlobenil to BAM.
Toxicokinetics
In rats, BAM is well absorbed after oral administration. Over 60 % was eliminated via the urine and 15 % via faeces 48 hours after a single oral dose of radiolabeled BAM. The entero-hepatic circulation
plays an important role. The retention of radioactivity in the carcass was about 5 % after 96 hours. Dermal absorption of BAM has not been investigated.
Autoradiographic studies showed that a marked retention of radioactivity was associated with the nasal region (especially in the Bowman's glands) and the contents of the large intestines of rats and mice
dosed with 14C-BAM.
About 25 % of the oral dose was excreted unchanged via the urine in rats. The major route of metabolism of BAM in rats and mice involved conjugation with glutathione in a process, which also involved
hydroxylation of the aromatic ring. None of the urinary metabolites of BAM were common to the metabolites of dichlobenil found in the same species.
Human toxicity
No data available.
Animal toxicity – acute effects
Rats
The acute oral LD50 value for rats was 1470 mg/kg for males and 2330 mg/kg for females. The acute oral LD50 for mice was 1538 and 1144 mg/kg in males and females, respectively. CNS effects have
been observed: prostrate, limbs relaxed, righting reflex absent, miosis, rapid and shallow respiration, and at high doses unconsciousness. At the lowest dose administered to rats (1000 mg/kg), the symptoms
included: one death, prostrate, limbs relaxed, righting reflex absent but corneal reflex present, miosis, and rapid but shallow respiration.
The acute toxicity of BAM after dermal exposure or inhalation has not been studied.
Mice
The toxic effect of BAM was examined in the nasal passages of C57Bl mice following single ip injections (25, 50 or 100 mg/kg bw). No clinical signs of toxicity were observed in any of the BAM treated
mice. After administration of 25 and 50 mg/kg bw there was an indication of disturbed function of the Bowman's glands (decreased PAS-staining). This effect was not observed 20 days after the exposure.
At 100 mg/kg bw necrosis of the Bowman's glands and neuroepithelium was observed especially in the dorsomedial aspects of the olfactory region. No lesions were observed in other parts of the nasal
cavity or in the liver. It was proposed that the lesions are due to a local cytochrome P450-dependent activation and that the more extensive toxic effects of chlorthiamid and dichlobenil in the olfactory
mucosa are mediated by common or closely related metabolites, different from those of BAM.
Given that the decreased PAS-staining of Bowman's glands only implies a slight physiological response and does not affect the function of the nasal cavity, an acute NOAEL of 50 mg/kg may be assigned.
The LOAEL may therefore be 100 mg/kg.
For an overview of NOAEL's, LOAEL's and critical effects see Table 5.
Rats
A group of Wistar rats (5 of each sex per group) received BAM orally at the following dose levels: 6.25, 12.5, 25, 50, 100, 200, 400, 800 and 1600 mg/kg bw/day for 8 days. Deaths occurred between
day 2 and 9 and were associated with loss of righting reflex, corneal and pain reflexes, mydriasis, shallow respiration, bradycardia and hypothermia. The subacute oral LD50 value was estimated to be 677
mg/kg for males and 574 mg/kg for females. The reduction in skeletal muscle tone was the most sensitive parameter in this study. The hypotonus was accompanied by impaired righting reflex, miosis,
hypothermia, moderate analgesia and rapid but shallow respiration. These symptoms appeared about 15 min after the treatment and peaked 2 hours later. The NOAEL was 12.5 mg/kg bw/day for males
and 50 mg/kg bw/day for females.
Wistar rats (10/sex/dose) were exposed to BAM for 13 weeks at dietary levels of 0, 50, 180, 600 or 2300 ppm. The NOAEL for systemic effects was set at 180 ppm (14 mg/kg/day) and the LOAEL was
set at 600 ppm (49 mg/kg/day) based on decreased body weight gain and food efficiency, increased blood urea nitrogen, and reduced coagulation times.
Dogs
Dogs (6 (control) or 4 dogs/sex/group) were fed a diet containing 0, 100, 300 and 2000 ppm BAM for a period of 13 weeks. Dogs in the highest dose group showed reduced body weight, increased liver
weights, and in females decreased serum urea and increased serum alkaline phosphatase activity and 2-globulin. At 300 ppm, increased liver weights were observed. The NOAEL in this study was 100 ppm
(approximately 2.5 mg/kg bw/day).
Animal toxicity – Chronic effects / carcinogenicity
Rats
In a combined chronic toxicity/carcinogenicity study BAM was given to Crl CD rats (35/sex/dose) for 106-107 weeks at dietary levels of 0, 60, 100, 180 or 500 ppm. At 500 ppm the following was
observed: a decrease in mean body weight gain, increased relative liver weight (females) and slightly increased severity of fat deposition in the livers of females. The NOAEL was or 180 ppm or 6.5 (6.0
based on analysed feed data) mg/kg/day. The LOAEL was set at 500 ppm or 19 mg/kg/day (17 mg/kg/day based on analysed feed data).
BAM produced an increased incidence of hepatoma in females at 500 ppm (≈ 25(23 based on analysed female feed data) mg/kg bw/day), which was of borderline significance (p < 0.049).
Dogs
Beagle dogs (4/sex/dose) were studied in a chronic toxicity study. BAM was fed to the dogs for two years at dietary levels of 0 (control), 60, 100, 180, or 500 ppm. The NOAEL was 180 ppm or 4.5
mg/kg/day. The LOAEL was set at 500 ppm or 12.5 mg/kg/day based on decreased body weight gain in males (58 % of controls at 2 years) and in females (29% of controls at 2 years) and increased
relative liver weight in males.
Reproductive and developmental effects
Rabbits
In a teratology study, New Zealand white rabbits (16/dose group) were administered BAM at dosing levels of 0, 10, 30, or 90 mg/kg/day by oral gavage on gestational days (GDs) 7 – 19. Developmental
toxicity was observed at 90 mg/kg/day as a non-significant decrease (94% of controls) in foetal body weight, which was outside the historical control range. BAM was not teratogenic in this study. The
maternal NOAEL was set at 10 mg/kg/day, and the LOAEL was set at 30 mg/kg/day. The developmental toxicity NOAEL was set at 30 mg/kg/day, and the developmental toxicity LOAEL was set at 90
mg/kg/day.
Rats
In a three generation reproduction study with two litters per generation, BAM was given to 10 male and 20 female Long-Evans rats per dose group at dietary levels of 0, 60, 100, or 180 ppm (equivalent to
about 0, 3-6, 5-10, or 9-18 mg/kg bw/day). The NOAEL was 60 ppm (3-6 mg/kg bw/day), and a LOAEL was set at 100 ppm (5-10 mg/kg/day).
Mutagenic and genotoxic effects
BAM was negative in a bacterial reverse mutation assay, in a test for unscheduled DNA synthesis in primary rat hepatocytes, and in an in vivo mouse micronucleus assay using a single dose of BAM (250
mg/kg).
Table 5. Summary of NOAEL's and LOAEL's
Duration |
Route |
Species |
NOAEL |
LOAEL |
Critical effects |
Reference |
|
|
|
(mg/kg bw/day) |
(mg/kg bw/day) |
|
|
Single dose |
ip |
mouse |
50 |
100 |
Necrosis of the Bowman's glands and the neuroepithelium |
(Brittebo et al., 1991) |
8 days |
oral |
Rat, males |
12.5 |
25 |
Reduction in skeletal muscle tone |
(Kemp and van der Linde, 1967) |
|
|
Rat,
females |
50 |
100 |
|
|
13 weeks |
oral |
rat |
14 |
49 |
Decreased body weight gain and food efficiency, increased blood urea nitrogen, and reduced
coagulation times. |
(U.S.Environmental Protection Agency, 1998) |
13 weeks |
oral |
dog |
2.5 |
7.5 |
Increased liver weights |
(Walker, 1967) |
2 years |
oral |
rat |
6 |
17 |
Decreased body weight gain in males and in females. |
(Wheldon et al., 1971) |
2 years |
oral |
dog |
4.5 |
12.5 |
Decreased body weight in both sexes and increased relative liver weight in males. |
(Wilson and Thorpe, 1971) |
Gestation days 7 -
19 |
oral |
rabbit |
10 (maternal), 30
(foetal) |
30 (maternal), 90
(foetal) |
Maternal effect: Increased mortality
Foetal effect: Decrease in foetal body weight and body weight gain |
(U.S.Environmental Protection Agency, 1998) |
3 generations |
oral |
rat |
3 – 6 |
5 - 10 |
Increased relative liver weight |
(Hine, 1970) |
BAM versus dichlobenil
The urinary metabolite profile of BAM in rats did not resemble that of dichlobenil (2,6-dichlorobenzonitrile) (Bakke et al., 1988b). The metabolic pathway(s) of dichlobenil may be comparable to that of BAM, but neither the
-CONH2 group of BAM nor the –CN group of dichlobenil is changed. The reaction:
Ar•CN → Ar•CO•NH2 → ( Ar•COOH)
that could result in common metabolites does apparently not occur to a measurable degree. In addition, a high fraction of absorbed BAM is excreted unchanged in the urine (about 25%) whereas dichlobenil
is apparently not eliminated unchanged via the urine.
Like BAM, dichlobenil generally is of low acute toxicity. It is slightly toxic by the oral, dermal, and inhalation routes (U.S.Environmental Protection Agency, 1998).
The effect on the olfactory mucosa after a single ip injection to mice has been investigated. Necrosis of the Bowman’s glands and the neuroepithelium was observed from 12 mg dichlobenil/kg (lowest dose),
from 12 mg dichlorothiobenzamide/kg (but not 6 mg/kg), and at 100 mg BAM/kg (but not 50 mg/kg). Dichlobenil was considerably more toxic to the olfactory mucosa when compared to BAM (Brandt et al., 1990, Brittebo et al., 1991).
The critical effects identified for chronic exposure to dichlobenil were derived from a two-year dog feeding study where systemic toxicity was observed (U.S.Environmental Protection Agency, 1998). The NOAEL was 1.25 mg/kg/day. The LOAEL for
systemic toxicity was 8.75 mg/kg/day based on:
- an increase in absolute and relative liver and thyroid weights in both sexes;
- an increase in serum alanine aminotransferase in females, and serum alkaline phosphatase in both sexes;
- an increase in liver enzyme glucose-6-phosphatase and glucose-6-phosphatase dehydrogenase activity in both sexes; and
- leucocytic infiltration and fibrinoid degeneration around the central hepatic veins of both sexes.
The critical effects identified for chronic exposure to BAM was determined from a two-year dog feeding study based on decreased body weight gain in both males and females. The NOAEL of 4.5
mg/kg/day has been determined, and the LOAEL was 12.5 mg/kg/day. The liver appears to be the target organ for both BAM and dichlobenil.
Neither dichlobenil nor BAM have demonstrated mutagenic potential in a variety of mutagenicity tests (U.S.Environmental Protection Agency, 1998).
There is only limited evidence for carcinogenicity of dichlobenil that is classified like BAM by US EPA as a Group C, “possible human carcinogen” (U.S.Environmental Protection Agency, 1998). Liver tumours were observed in one out of two hamster
studies and in one study with Fisher rats. Available data for BAM suggests that its potential carcinogenicity (also liver tumours) does not exceed that of dichlobenil (and may be lower).
Dichlobenil is not considered to be specific toxic to reproduction based on two teratogenicity studies (rabbits and rats) and one two-generation study with Sprague-Dawley rats. Although not fully
investigated, this also appears to be the case for BAM.
7 Evaluation
The toxicity of BAM has not been fully investigated. Test results from studies performed over 30 years ago have been available. The tests do not comply with current internationally accepted guidelines, and
they have not been performed in accordance with current requirements to good laboratory practice. Despite the lacks in study design and performance, the studies, in general, appear to be well performed,
and for lack of any better studies they can be used for the toxicological assessment of BAM.
The skin and eye irritation potential and the skin sensitising properties of BAM have not been studied.
In more recent studies with mice and rats, the nasal cavity with the olfactory epithelia and especially the Bowman's glands has been demonstrated as a target organ for BAM toxicity. This has been supported
by autoradiographic studies where retention of radioactivity was located to the Bowman's glands. Mild reversible effects, i.e. reduced PAS-staining of Bowman's glands, were observed after a single
intraperitoneal dose of 25 or 50 mg/kg bw to mice. This effect was especially evident in the dorsal meatus, whereas PAS-positive Bowman's glands were present in the lateral aspects of the nasal cavity.
Twenty days after the administration, the PAS-staining intensity appeared normalised. PAS (periodic acid-Schiff) is a sensitive staining method that stains glycogen (and other periodate reactive
carbohydrates), mucin, mucoprotein, and glycoprotein. The reduced staining in the Bowman's glands indicates a reduced presence of stainable material (e.g. glycoproteins) in these cells. This again indicates
a disturbance of the cellular function.
When the dose was raised to 100 mg/kg bw a clear pathological effect was observed in the olfactory epithelia (undulating and necrotic) and the Bowman's glands (necrotic).
It is not known how repeated exposure to BAM will affect the nasal mucosa, as this has not been investigated in the repeated dose studies. One may suspect a potential for increased retention of BAM
(metabolites) at low dose levels with resultant destruction of the Bowman's glands and subsequent the olfactory epithelia. This may reduce the function of the nasal mucosa (e.g. reduced ability to smell and
reduced mucosal immune defence). Another unsolved question is whether the findings in rodents are predictive for humans. The pathogenesis apparently involves a local cytochrome P450-dependent
activation of BAM that may not be expressed that clearly in humans. Provided that the decreased PAS-staining of rodent Bowman's glands does not affect the function of the nasal cavity, an acute NOAEL
of 50 mg/kg may be assigned.
The carcinogenic potential of BAM has not been fully investigated. As BAM was not shown to be mutagenic, a possible carcinogenic effect may be considered mediated through a non-genotoxic mechanism
secondary to organ toxicity. A threshold for such an effect can be anticipated.
The toxicological profile of BAM does show some resemblance to dichlobenil. Despite the generally poor quality of the studies performed with BAM and dichlobenil the results indicate some common
toxicological features. The liver appears to be the target organ for both substances. The substances may promote the development of liver tumours by a non-genotoxic mechanism secondary to the organ
toxicity. They both have an affinity to the nasal mucosa. However, dichlobenil is clearly more acute toxic to the olfactory epithelium and Bowman's gland than BAM. They do not have common metabolites in
vivo but probably share metabolising enzymes. The NOAEL was 1.25 mg/kg/day in a two-year dog feeding study with dichlobenil. The LOAEL was 8.75 mg/kg/day based on liver toxicity. These values
are comparable to those in another 2-year dog feeding with BAM. In the BAM study a NOAEL of 4.5 mg/kg/day was determined, and the LOAEL of 12.5 mg/kg/day was based on decreased body
weight gain in both males and females and increased relative liver weight in males.
The NOAEL (4.5 mg/kg bw/day) from the 2-year dog feeding with BAM may be preferred as the end point of concern identified for chronic exposure instead of the NOAEL of 2.5 mg/kg bw/day from the
13 week dog study, because the NOAEL of the 2 year study was lower than the LOAEL (7.5 mg/kg bw/day) in the 13 week dog study. However, the NOAELs lie within a close range (Table 5). The
critical effect of BAM is not very clearly expressed as systemic toxicity, and the LOAELs are often based on reduced weight gain.
From the toxicological database an overall NOAEL range of 2.5 – 6 mg/kg bw/day is selected for further risk characterisation.
8 Risk characterisation, drinking water exposure
A NOAEL range of 2.5 – 6 mg/kg bw/day is selected for the human risk characterisation.
Intake of contaminated drinking water is the most relevant route of exposure to BAM.
An adult person weighing 70 kg drinks about 2 litres of water every day, i.e. 0.03 l/kg body weight (bw)/day. This is a default value, and it does not take into consideration that hot weather and high physical
activity will increase the intake. For children in the age group 1 – 10 years, a daily water intake of 0.08 l/kg bw/day can be taken as a 95-percentile (U.S.Environmental Protection Agency, 1997).
An acute dietary risk of BAM is not considered relevant.
If the water contains 0.1 µg BAM/l, which is the administrative threshold limit in drinking water, the intake of 0.003 µg BAM/kg bw/day and 0.008 µg/kg bw/day for adults and children, respectively (see Tables
1 and 6). Exposure to the highest concentration detected in a recent study, 14 µg/l (Brüsch, 2002), corresponds to an intake of 0.42 µg BAM/kg bw/day and 1.12 µg/kg bw/day for adults and children, respectively. Exposure
to the unusual and extremely high concentration detected in the period 1991 – 2001, 560 µg/l (Jørgensen, 2002), corresponds to an intake of 16.8 µg BAM/kg bw/day and 44.8 µg/kg bw/day for adults and children, respectively.
Table 6. Theoretical intake of BAM via drinking water
Water concentration of BAM |
Theoretical intake of BAM for an adult person weighing 70 kg |
Theoretical intake of BAM for 1 – 10 years old children |
0.1 µg/l |
0.003 µg/kg bw/day |
0.008 µg/kg bw/day |
1.0 µg/l |
0.03 µg/kg bw/day |
0.08 µg/kg bw/day |
10.0 µg/l |
0.30 µg/kg bw/day |
0.80 µg/kg bw/day |
14 µg/l |
0.42 µg/kg bw/day |
1.12 µg/kg bw/day |
560 µg/l |
16.8 µg/kg bw/day |
44.8 µg/kg bw/day |
The margin of safety (MOS) is calculated as:
MOS = NOAEL / BAM intake
Table 7. Margin of safety (MOS)
Water concentration of BAM |
MOS an adult person weighing 70 kg |
MOS for 1 – 10 years old children |
0.1 µg/l |
8.3 x 105 – 2.0 x 106 |
3.1 x 105 – 7.5 x 105 |
1.0 µg/l |
8.3 x 104 – 2.0 x 105 |
3.1 x 104 – 7.5 x 104 |
10.0 µg/l |
8.3 x 103 – 2.0 x 104 |
3.1 x 103 – 7.5 x 103 |
14 µg/l |
6.0 x 103 – 1.4 x 104 |
2.2 x 103 – 5.4 x 103 |
560 µg/l |
1.5 x 102 – 3.6 x 102 |
55.8 – 134 |
The estimated MOS greatly exceeds 1000 in most cases.
When using the above risk characterisation and the MOS-calculation for risk management purposes several issues should be addressed.
Regarding the information given to people who have been exposed to contaminated wells, the above calculated MOS-values can be used to express concern/no concern in relation to intake in the past.
In relation to establishing a protection level and providing adequate information for the continued use of wells containing low residue levels, several health and policy related issues should be considered as
well. When assessing the safety of different levels of the substances, the possibility of fluctuations in contamination levels as well as the possible occurrence of other contaminants that may follow together with
the residues should be considered. Further, attention should be given to the policy concerning protection of the ground water as a drinking water resource and to the overall policy for a drinking water supply
aiming at clean and unpolluted drinking water. Therefore, a possible maximum level of residues of BAM in drinking water should not be established as toxicologically acceptable levels but as levels that can
be accepted based on overall considerations taking into account the overall policy, the necessity of sufficient drinking water supply and the protection of human health.
9 References
HSDB [Hazardous Substances Data Bank]. National Library of Medicine. Last update: 6-8-2002. Access date: 6-5-2003. http://toxnet.nlm.nih.gov/cgi-bin/sis/htmlgen?HSDB.
BEILSTEIN. Beilstein-Institut zur Förderung der Chemischen Wissenschaften. Last update: 18-1-2003. Access date: 30-5-2003. http://www.cas.org/ONLINE/DBSS/beilsteinss.html.
CHEMLIST. Chemical Abstracts Service. Last update: 23-5-2003. Access date: 30-5-2003. http://www.cas.org/ONLINE/DBSS/chemlistss.html.
PHYSPROP [the Physical Properties Database]. Syracuse Research Corporation. Last update: 2003. Access date: 7-5-2003. http://esc.syrres.com/interkow/PhysProp.htm.
REGISTRY [database]. Chemical Abstracts Service. Last update: 2003. Access date: 6-5-2003. http://www.cas.org/ONLINE/DBSS/registryss.html.
Bakke JE, Larsen GL, Feil VJ, Brittebo EB, Brandt I. Metabolism of 2,6-Dichlorobenzamide in Rats and Mice. Xenobiotica 1988a;18(7):817-30.
Bakke JE, Larsen GL, Struble C, Feil VJ, Brandt I, Brittebo EB. Metabolism of 2,6-Dichlorobenzonitrile, 2,6-Dichlorothiobenzamide in Rodents and Goats. Xenobiotica 1988b;18(9):1063-75.
Brandt I, Brittebo EB, Feil VJ, Bakke JE. Irreversible Binding and Toxicity of the Herbicide Dichlobenil (2,6-Dichlorobenzonitrile) in the Olfactory Mucosa of Mice. Toxicol Appl Pharmacol
1990;103(3):491-501.
Brittebo EB, Eriksson C, Feil V, Bakke J, Brandt I. Toxicity of 2,6-Dichlorothiobenzamide (Chlorthiamid) and 2,6-Dichlorobenzamide in the Olfactory Nasal Mucosa of Mice. Fundam Appl Toxicol
1991;17(1):92-102.
Brüsch W. Statusrapport 2002: Pesticidforurenet vand i små vandforsyningsanlæg. Danmarks og Grønlands Geologiske Undersøgelse; 2002. Report number: 2002/87.
http://www.geus.dk/program-areas/water/denmark/rapporter/status_2002_pesticid_smaa_anlaeg.pdf.
Clausen L, Arildskou NP, Larsen F. Nedbrydning og sorption af dichlobenil og BAM - Litteraturopsamling samt laboratorieforsøg. Delrapport 3. 2002. Report number: Arbejdsrapport fra Miljøstyrelsen
nr. 35. http://www.mst.dk/udgiv/Publikationer/2002/87-7972-331-4/pdf/87-7972-332-2.pdf.
Council of the European Union. Directive 98/83/EC of 3 November 1998 on the Quality of Water Intended for Human Consumption. Official Journal of the European Communities 1998 Dec 5;L
330:32-54.
Advisory List for Self-Classification of Substances. Danish EPA. Environmental Project 636. Last update: 2001. Access date: 30-5-2003. http://www.mst.dk/kemi/02040300.htm.
Elkjær L, Ludvigsen L, Juhl MM, Stougaard M, Bastrup J, Baumann J et al. Pesticider og vandværker. Udredningsprojekt om BAM-forurening. Danish Environmental Protection Agency; 2002. Report
number: Miljøprojekt nr. 732. http://www.mst.dk/udgiv/Publikationer/2002/87-7972-324-1/pdf/87-7972-326-8.pdf.
Geyer H, Freitag R, V, Korte F. Relationship Between Water Solubility of Organic Chemicals and Their Bioaccumulation by the Alga, Chlorella Fusca. Chemosphere 1981;10(11-12):1307-14.
Griffiths MH, Moss JA, Rose JA, Hathway DE. The Comparative Metabolism of 2,6-Dichlorothiobenzamide (Prefix) and 2,6-Dichlorobenzonitrile in the Dog and Rat. Biochemical Journal 1966;
98(3):770-81.
Hine CH. Results of reproduction study of rats fed diets containing 2,6-dichlorobenzamide (BAM) over three generations. San Francisco, California, USA. The Hine Laboratories; 1970.
Jørgensen LF. Grundvandsovervågning 2002. Miljøministeriet og Danmarks og Grønlands Geologiske Undersøgelse; 2002 Dec. http://www.geus.dk/publications/grundvandsovervaagning/g-o-2002.pdf.
Kemp A, van der Linde HM. Preliminary toxicity studies with 2,6-dichlorobenzamide: a) Acute oral toxicity to rats. b) Range finding study in rats - daily oral application for eight days. Weesp, Holland.
N.V. Philips-Duphar; 1967 Aug. Report number: 56645/1/67. p.1-25.
Lehman AJ. Quarterly Report to the Editor on Topics of Current Interest. Association of Food and Drug Officials Quarterly Bulletin 1954;18:66.
Miljøstyrelsen. Sundhedsmæssig vurdering af kemiske stoffer i drikkevand. Miljøstyrelsen. 1992; 1. Vejledning fra Miljøstyrelsen.
Nakagawa Y, Izumi K, Oikawa N, Sotomatsu T, Shigemura M, Fujita T. Analysis and Prediction of Hydrophobicity Parameters of Substituted Acetanilides Benzamides and Related Aromatic Compounds.
Environ Toxicol Chem. 1992;11(7):901-16.
U.S.Environmental Protection Agency. Exposure Factors Handbook -. EPA/600/P-95/002Fa - Update to Exposure Factors Handbook EPA/600/8-89/043 - May 1989 ed. Vol 1 - General factors. 1997.
U.S.Environmental Protection Agency. Reregistration Eligibility Decision (RED): Dichlobenil. 1998 Oct. Report number: EPA-738-R-98-003. http://www.epa.gov/oppsrrd1/REDs/0263red.pdf.
EPI Suite [computer program]. U.S.Environmental Protection Agency. v. 3.10. U.S. Environmental Protection Agency; 2000.
U.S.Environmental Protection Agency. List of Subjects in 40 CFR Part 70 Environmental Protection, Administrative Practice and Procedure,
4-Amino-6-(1,1-Dimethylethyl)-3-(Methylthio)-1,2,4-Triazin-5(4H)-One [Metribuzin], Dichlobenil, Diphenylamine, Sulprofos, Pendimethalin, and Terbacil; Tolerance Actions. Fed Reg
2001;66(234):63192-9.
Walker AIT. The study of the oral toxicity of the "Prefix" residue 2,6-dichlorobenzamide: 13 week exposure to dogs. Tunstall Laboratory, Sittingbourne, Kent, England. "Shell" Research Limited; 1967 Feb.
Report number: R(T)-1-67.
Wheldon GH, Street AE, Heywood R, Spicer EJF, Hague PH. Effects of BAM in dietary administration to rats for two years. Huntingdon, England. Huntingdon Research Centre; 1971. Report number:
3980/71/138.
WHO. Principles for the safety assessment of food additives and contaminants in food. Geneva: World Health Organization; International Labour Organisation; United Nations Environment Programme.
1987 (Environmental health criteria; vol. 70). http://www.who.int/pcs/jecfa/ehc70.html.
Wilson AB, Thorpe E. Toxicity Studies on the "Prefix" Residue 2,6-Dichlorobenzamide: Two Year Oral Experiment with Dogs. Tunstall Laboratory; 1971. Report number: Lab Project Number: T507531;
Group Research Report: TLGR.0028.71. p.1-28.
| Top | | Front page |
Version 1.0 November 2004, © Danish Environmental Protection Agency
|