| Front page | | Contents | | Previous | | Next |
Survey of Estrogenic Activity in the Danish Aquatic Environment
8 Discussion of the results
8.1 Sources of estrogens
8.1.1 Wastewater treatment plants
8.1.2 Drains from manure treated fields
8.1.3 Other open land sources
8.1.4 Geographical variation
8.1.5 Seasonal variation
8.2 State of the aquatic environment
8.2.1 General state of the aquatic environment
8.2.2 Streams receiving WWTP effluents
8.2.3 Reference streams and lakes
8.2.4 Geographical variation
8.2.5 Seasonal variation
8.3 Effect levels of estrogens in freshwater environments
8.4 Occurrence of the synthetic hormone Ethynylestradiol
8.4.1 Samples with non-steroid estrogenic activity
8.1 Sources of estrogens
8.1.1 Wastewater treatment plants
Influent estrogenic activities and steroid estrogen concentrations were measured once (summer) at each of the 12 WWTPs (out of a total of 36 plants) that were selected for closer examination. The levels,
reported as E2-equivalents (EEQ), varied somewhat but stayed in most cases within a range of 20-90 ng EEQ/L (with one extreme result at 385 ng EEQ/L). It has not been possible to correlate the
concentrations with size or character of the catchment (to the extent that this has been known).
The effluent quality with regard to steroid estrogens was, however, clearly dependent on the applied process technology, and the importance of the activated sludge systems was evident (categories A and
B). Trickling filters and simple retention tanks (categories C and D) did not contribute much to removal of estrogenic activity. That the Cat. D plants appear to perform slightly better than the Cat. C plants is
considered purely incidental but might be associated with the fact that the influent levels at the two category D-plants were very low.
These findings are in line with the results obtained in a German investigation at 16 municipal WWTPs (Körner et al., 2001; Spengler et al., 2001), a Swedish survey of WWTP effluents from 20 treatment
plants representing a range of technologies (Svenson et al. 2003), and the information on estrogens in WWTP effluents presented in the review report on feminisation of fish prepared by Christiansen et al.
(2002) for the Danish EPA.
It appears that the process technology needs not to be very advanced in terms of requirements to investments and costs of operation in order to produce fine results. In this study, the effluents from reed beds
(category E) and, in particular, biological sand filters (category F) contain very low levels of estrogenic activity.
It has previously been demonstrated by researchers in Japan that a sand filter installed for (pilot-scale) tertiary treatment of a WWTP effluent did not significantly reduce effluent estrogenicity (cfr.
Christiansen et al. 2002). This is, however, not regarded contradictory to the high removal efficiency of biological sand filters observed in this study since a traditional sand filter for tertiary treatment has a
very short hydraulic retention time (HRT) and practically exclusively act as a physical filter, while the biological sand filter treats influent and not effluent, has a much higher HRT and contains an active
microbial biomass, which transforms organic matter, nutrients and many specific substances.
No correlation with the general performance parameters from each of the sampled WWTPs (effluent quality in terms of COD, BOD, SS, N and P) could be revealed. This does not necessarily mean that
such correlations do not exist but apparently measurements in the same samples as the estrogens are required to elucidate them - it is not enough to compare with average general performance data as was
done here.
Stormwater overflow episodes were not investigated in this study because it is possible to predict discharge concentrations from influent data and typical dilution factors of the system in question. As a rule of
thumb a dilution factor of 5 can often be used, which, if using the influent levels observed, implies that the level of estrogenic activity in stormwater overflows typically will be about 5-20 ng/L.
8.1.1.1 Steroid estrogens and their forms in wastewater
The relation between the free estrogens and the total estrogens (free and conjugated estrogens) are shown in Figure 8.1 and Figure 8.2. The full drawn lines indicate where measurements of total and free
estrogens are equal and an almost full agreement between free and total estrogens was observed. Thus, in general free estrogens make up 90-100% of the total estrogens in the samples. The samples with
conjugated species reveal themselves by occurring above the line of unity.
Samples taken from the open land sources and the aquatic environment (G-R) rarely contain more than 10% conjugated estrogens, and in most environmental samples almost 100% of the total estrogenic
activity can be attributed to free estrogens.
The samples taken directly in the WWTP effluents are somewhat higher in the concentration of conjugates, and often display a 20 % contribution from the conjugated species when concentrations exceed 5
ng/L. All values are included in Figure 8.1 with an expansion of the 0-12 ng/L range in Figure 8.2.
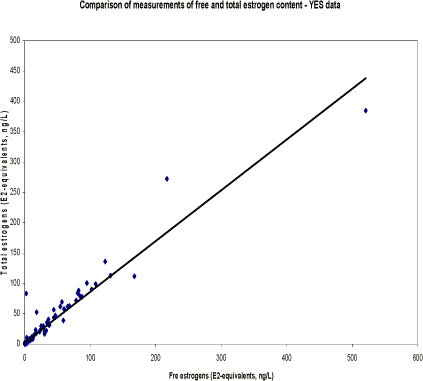
Figure 8.1 Comparison of total and free estrogens determined in YES assay.
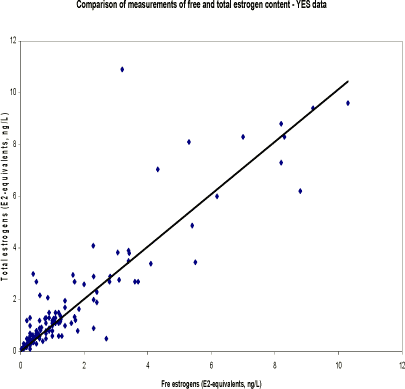
Figure 8.2 Expansion of the lower range 0-12 ng/L of the data set.
Therefore the concern that a major part of the potential aquatic estrogenic activity occurs in a form that is mobilised during deconjugation is not supported with the present data. By far the highest activity of a
water sample from the environment will be found in the free estrogenic fraction compared to the conjugated.
With regard to the chemically analysed steroid estrogens in can be concluded that in WWTP effluents E1 is in most samples the main contributor to the estrogenic activity observed followed by E2. Only
occasionally did EE2 occur at a significant level.
8.1.1.2 Environmental load of estrogens from WWTP effluents
It is relevant to consider the overall release of estrogens from anthropogenic sources to the aquatic environment and the relative importance of the various sources. For the WWTPs so many data exist that a
rough estimate of the total load and the relative share of each of the WWTP categories A-F can be made.
Table 8.1 presents such an estimate of the relative share of each WWTP category to the total emission of estrogens with WWTP effluents to fresh surface waters and coastal waters in Denmark. The
estimate is based on the median level of total estrogenic activity in the YES assay for each category (calculated as ng E2 equivalents/L) including the non-detect results, which for this purpose have been
assigned the value of 0.025 ng/L (= ½ x LOD for the YES assay). These data have then been combined with the most recent data on wastewater volumes (average of last three years, 2001-2003 = 713
mill. m3) and distribution on categories (2003 data) from the national Danish surveillance programme for the aquatic environment (Miljøstyrelsen 2004).
The total emission of estrogens with WWTP effluents to the aquatic environment in Denmark can hereby roughly be estimated to less than 3 kg E2 equivalents/year. This corresponds to about 10 % of the
calculated total excretion of estrogens by the Danish population (based on data given in Christiansen et al. 2002, p. 84).
Table 8.1 The relative contribution by category to the estimated total load of estrogenic activity from the Danish WWTPs to the aquatic environment (marine as well as freshwater). Based on
national wastewater data from 2003 (Miljøstyrelsen 2004).
WWTP category |
Share of wastewater volume (%) |
Median estrogen level (ng/L)* |
Share of total release of estrogens (%) |
A - MBND(C) |
90,8 |
3.6 |
81.4 |
B - MBN(C) |
6,6 |
1.4 |
2.3 |
C - MB(C) |
1,4 |
27.7 |
9.7 |
D - M(C) |
0,8 |
31.5 |
6.3 |
E - Reed beds |
0,2 |
5.5 |
0.3 |
F - Biological sand filters |
0,1 |
0.23 |
0.007 |
* Based on all data (only one value per WWTP) including non-detects, for which a value of 0.025 ng/L (= ½ x LOD for the YES assay) has been used.
As can be seen from the table, the A and B categories, i.e. the most advanced treatment plants, contribute, despite their good elimination efficiency, with more than 83% of the total estrogenic activity
released from Danish WWTPs because they are responsible for more than 97% of the total effluent volume.
Category C and D plants are responsible for about 16% of the activity despite their modest 2.2% share of the total effluent volume, while the emissions from categories E and F are negligible in the overall
picture.
It should be stressed, however, that a major part of the effluent volume from the A and B plants (probably more than 70%) is released directly into the marine (coastal) environment and thereby does not
contribute to the load on the freshwater systems. The share of Category C and D effluents being discharged into the marine environment is believed to be considerable lower than for A+B, and therefore the
relative importance of these two categories in relation to the freshwater environment becomes even more pronounced.
8.1.2 Drains from manure treated fields
All mammals excrete natural estrogens and therefore, significant amounts are released from household animals (cattle, swine, and poultry). Raman et al., (2004) recently reported representative
concentrations of estrogens in animal waste in the range 1-100 µg/L. The concentration is dependent of the animal type and the manure storage procedures. When manure is spread on agricultural soil, it is an
obvious risk that the estrogens are transported to the aquatic environment. Although the substances has been shown to absorb and dissipate strongly (half-lives range from hours to a few days) in soil (Lee et
al., 2003), studies linking estrogens detected in the environment directly to animal sources (Finlay-Moore et al., 2000; Peterson et al., 2000) and the total amount of estrogens released gives reason to
believe that the steroid estrogens from farm animals may reach the surface waters. It is therefore anticipated that estrogens from household animals may reach the environment due to transport mechanisms
including binding to colloids and transport through macro-pores in the soil.
In the current project, the estrogenic activity in drainage water from fields where manure was applied in the late winter/early spring of 2004 (Cat H), was below the LOD in 4 out of 8 samples while in the
remaining 4 samples the levels were 0.1-1.1 ng/L. The sampling was carried out in the spring, shortly after application had taken place, and again in the fall (late September). Due to the few locations
sampled and only two samples taken at each site, possible pulses with higher concentrations of estrogens (as known from investigations of pesticide transport to the aquatic environment via drainage systems)
may very well have been overlooked. Thus the data must be described as inconclusive though none of the results from the testing and analysis of samples from the aquatic environment indicate that severe
estrogen contamination originating from manure applied to fields occur widespread.
8.1.3 Other open land sources
Septic tank effluents from single farmhouses (Cat. G) were expected to be quite "dirty" and they have also been shown to contain about the same estrogenicity as the influents to WWTPs in categories A-F.
Drainage water from three fields amended with sewage sludge in 2004 (Cat. I) was sampled in the spring and again in the fall (late September). All the samples contained steroid estrogens at a level above
the LOD. At two of the sites the levels were below 1 ng/L, but in one case 32 ng/L was found in the September sample while the spring sample from the same drain contained 3.8 ng/L. This result can only
be logically explained if it turns out that also effluents from one or more households are connected to the drainage system. As the drainage data from the manure treated fields also the data for the sludge
treated fields are considered inconclusive due to the few locations sampled and few samples taken.
The two samples of stormwater effluents from separate systems (Cat. J) did not contain any appreciable amounts of estrogens, and a possible low level of estrogens is probably rather due to xenoestrogens
than steroid estrogens. This expectation corresponds well with the findings in the Netherlands reported by Vethaak et al. (2002).
Effluents from two fish farms (Cat. K) only displayed little estrogenicity but the sampling carried out in the fall indicated that fish farms that rear adult fish for production of eggs or fry may have higher levels in
the fall where a larger number of the fish are sexually mature. An alternative explanation of the higher estrogenicity in the fall samples could be that hormones have been used in the production (this hypothesis
has not been verified).
8.1.4 Geographical variation
There were no signs of systematic geographic variation in the results from neither the categories A-F (WWTPs) nor the categories G-K (open land sources).
Such differences are not likely to occur with regard to the categories A-F (and G, which actually is a kind of WWTP) because Denmark is a small and demographically very uniform country, i.e. the cultural
and technological regional differences are likely to be correspondingly small.
With regard to geographical variation in categories H-K, and in particular in the categories H and I (the field drains), some geographical variation may exist but has not been possible to elucidate within the
framework of this study.
8.1.5 Seasonal variation
The data set from WWTPs is quite comprehensive (winter, spring, summer and fall samples at 12 WWTPs), and it is clear that the estrogenic activity in the winter samples are lower than in the summer
samples, but otherwise there is not a clear pattern.
However, the four samples taken at each of the two low tech treatment facilities (C and D) sampled for seasonal change all show a clear increase in estrogenic activity in the spring samples compared to the
winter samples. No clear seasonal variation is observed in the A, B or F categories while there is a slight tendency in the E category for the estrogenicity to increase from the winter/spring samples to the
summer/fall samples. There has been found no evident explanation for these observations.
With the limited number of samples taken in the G-K categories it was not possible to reveal seasonal variations, if any. As mentioned before, effluents from fish farms with sexually mature fish should,
theoretically at least, display more estrogenicity in the fall compared to the spring.
8.2 State of the aquatic environment
8.2.1 General state of the aquatic environment
About 130 samples (categories M-R plus upstream samples from streams receiving WWTP effluents (category L)) have been sampled and tested by the YES bioassay to determine the state of the Danish
aquatic environment with regard to total estrogenic activity. A limited number of samples (47) were also analysed chemically.
In one third of the samples there was no detectable estrogenic activity while in about half of the samples the estrogenicity was low; from the LOD up to 1 ng/L. In all sub-categories except "generally exposed
lakes" (category R), the median estrogenic activity of the samples with detectable estrogenic activity (i.e. > LOD = 0.05 ng/L) was 0.6 ng/L or lower. In the "generally exposed lakes" category, the median
level of estrogenicity was 1 ng/L for the 4 out of 9 samples with detectable estrogenic activity.
There is a slight tendency that in the samples taken in streams and lakes situated in areas with known high density of pigs and cattle (categories N and O), and thereby significant need for disposal of manure
onto fields, a higher frequency of detection than in the other categories was observed. This weak trend was, however, not accompanied by a correspondingly higher median level of estrogenic activity.
17α-estradiol was detected in too few samples to allow any correlations with husbandry intensity to be drawn. This corresponds with the findings of Vethaak et al. (2002) in the Netherlands.
If discarding one inexplicable result of 80 ng/L (presumably an artefact since the result could not be repeated), only 25 of the 130 samples showed a total estrogenic activity of more than 1 ng/L, hereof four
samples above 5 ng/L with a maximum of 8.8 ng/L. In 18 of the 25 samples containing >1 ng/L, the estrogenic activity was below 2 ng/L.
These results compare quite well with literature data from investigations or surveys undertaken in other European countries. Thus, Fawell et al. (2001) analysed water from 8 sites in the Severn and Trent
rivers in the UK and found E2-equivalent (EEQ) levels from <0.01 ng/L to 2.3 ng/L. Murk et al. (2002) studied the estrogenic activity in Dutch surface waters (4 sites) by means of (among others) the YES
assay and found activities of free estrogens from about 0.4 to 3.0 pmol/l corresponding to 0.1-0.8 ng EEQ/l.
Other European investigators report results of chemical analyses of steroid estrogens in surface waters (e.g. Vethaak et al. 2002; Williams et al. 2003), and generally find levels of E1 and E2 in the sub-ng/L
or low ng/L range. Most often E1 is observed but also E2 is detected regularly. EE2 is uncommon to find in most surface waters. These findings are in line with the results of this study,
An American survey of exposed rivers and streams (Kolpin 2002a) operates with too high detection limits (typically 5 ng/L) to enable direct comparison with our results, but positive detections are also here
only found in 5-21% of the samples.
8.2.2 Streams receiving WWTP effluents
The streams receiving WWTP effluents appear to be typical of Danish streams/rivers when looking at the upstream levels of estrogenic activity and comparing to the data in the other freshwater environment
categories (M-R). A total of 46 data sets were available for an evaluation of whether the effluent discharges result in a measurable impact on downstream water quality.
In 11 of the data sets no estrogenic activity could be found neither up- nor downstream but among the remaining 35 data sets 24 showed an increase downstream (10x stream width) the discharge point
while only 6 samples showed the opposite. In 5 samples the up- and downstream levels were identical. A possible impact on water quality further downstream (100x stream width) has not been possible to
deduct from the data obtained in this study.
However, the impact of wastewater effluent discharges on downstream water quality in the receiving rivers or streams was generally rather limited; only in 13 of the 24 cases where downstream levels were
elevated did the increase exceed 0.5 ng/L. But in extreme cases (high estrogenicity level in discharge, low dilution in stream) significant increases will be possible. One such example exists among the data
where the discharge of a category D plant effluent at 47 ng/L into a very small stream resulted in an increase in the estrogenic activity in the stream from 1.1 ng/L upstream to 31 ng/L downstream the
discharge point.
8.2.3 Reference streams and lakes
The estrogenic activity in the reference (background) streams was generally very low i.e. from <LOD to 0.3 ng/L in all cases except one, where a total level of 7.3 ng/L was found (but no free estrogens,
therefore maybe an artefact). In more than 50% of the stream reference samples (6 out of 11), the estrogenic activity was below the LOD.
In the reference lakes it was possible to detect esrogenic activity in 9 out of 11 samples and the median level among these was higher than in the streams (0.4 ng/L against 0.1 ng/L). In 4 of the 12 samples
the levels were above 1 ng/L and one sample contained 6.0 ng/L (and the second sample at that site 1.5 ng/L) although no anthropogenic activities including husbandry were apparent at the site in question
(forest lake).
In summary, the results from the reference sampling stations indicate that estrogenic activity at a low level is often present even at locations where the anthropogenic influence is not apparent. The explanation
could be that other sources and types of estrogens (e.g. natural estrogens such as phytoestrogens) exist that give a response, albeit small, in the YES assay.
8.2.4 Geographical variation
It has not been possible to find any clear regional differences or correlations between soil types or land use and estrogenicity in the aquatic environment. To establish such possible relations, if possible at all, a
much more focused investigation with many more samples per site than was possible in this study is probably required.
8.2.5 Seasonal variation
As is the case for WWTP effluents, also the samples from the freshwater environment appear to exhibit slightly higher estrogenic activity in the summer than in the winter. No difference between spring and
fall has been observed.
Probably it is necessary to sample selected locations more intensively to be able to detect any differences, if such indeed exist.
8.3 Effect levels of estrogens in freshwater environments
The concentrations of estrogens and estrogenic activity as determined by in vitro bioassays being necessary to induce the feminising effects observed in male fish in the field are unknown. Therefore,
predictions of the potential effects of the concentrations of estrogen found in the present investigation will rely mainly on results obtained in short to medium time experiments in the laboratory and under
semi-field conditions. In such experiments production of yolk protein in males, development of intersex or other disturbances in the male testis have been used as markers for estrogenic effects.
It is important to note that sensitivity towards estrogens among different fish species may vary. Likewise, the sensitivity to estrogens varies considerably during the different life stages of a specific fish species.
Most of the controlled laboratory or semi-field experiments have been carried out with rainbow trout Oncorhynchus mykiss, roach Rutilis rutilis, carp Cyprinus carpio, japanese medaka Oryzias latipes,
fathead minnow Pimephales promelas or zebrafish Danio rerio.
17ß-estradiol (E2)
E2 has induced the production of yolk protein in rainbow trout at a concentration of 5 ng/L. Adult, male zebrafish generally respond to E2 concentrations from approximately 20 ng/L and higher (Rose et al.
2002, Brion et al. 2004). E2 has induced intersex in japanese medaka Oryzias latipes at 10 ng/L. A range of other testicular effects have been seen at concentrations between 10 – 50 ng/L and also at
concentrations above 100 ng/L. Examples of other effects are inhibition of the normal development of male germ cells, which can be seen as a lower relative weight of the testis and/or a presence of a larger
proportion of early stages of germ cells; the presence of degenerated germ cells has also been seen.
Estrone (E1)
A similar or slightly lower estrogenic activity has been detected for E1 in comparison with E2. Induction of yolk protein in male rainbow trout and fathead minnow has been seen at concentrations of
approximately 30 ng/L and 10 ng/L (Metcalfe et al 2001). Estrone alters the gonadosomatic index in fathead minnow with a NOEC of approx. 100 ng/L (Panter et al 1998).
Ethynylestradiol (EE2)
EE2 is more potent than the natural estrogens with regard to induction of disruptions in the male reproductive system. Induction of yolk protein have been seen at 0.1 ng/L in trout (42). The EC-50 value for
vitellogenin induction in adult, male zebrafish is 2.5 ng/L (Rose et al. 2002) and 1 ng/L induced vitellogenin production in fathead minnow (Pawlowski et al. 2004). EE2 changed sex ratio in zebrafish at
concentrations below 0.6 ng/L and egg production was affected in fathead minnow at exposure to 0.1 ng/L (Pawlowski et al. 2004). A range of other reproductive effects such as inhibition of normal sperm
cell development has been seen at concentrations below 10 ng/L.
The concentrations of estrogens present in the effluents from some of the WWTPs investigated in the present survey, in particular in the C and D categories, are obviously so high that they are likely to induce
feminisation in male fish, whereas effluents from well-functioning WWTPs have contents of estrogens that probably are below an effect level. The estrogenicity levels in the surface water samples are generally
lower than in the effluents, but with the existing scientific knowledge it cannot be excluded that feminisation can result from long term exposure to the levels observed in a number of the investigated streams
and lakes.
The prediction, however, whether or nor estrogenic effects are likely to occur in a specific effluent is - with our present knowledge - not straightforward. This is illustrated in a recent publication (Aerni et al.
2004) in which five WWTP effluents were investigated for estrogenic activity (YES), estrogenic chemicals (E1, E2, EE2, estriol and nonylphenol by chemical analysis) and the ability of the effluents to induce
vitellogenin production in rainbow trout. Vitellogenin production was induced in two out of five effluents, but not in a way that was totally predictable from the YES-assay results. An effluent with 2.1-5.3 E2
equivalents/l did not induce vitellogenin production, whereas an effluent with 2.4-5.5 E2 equivalents/l did; most of the estrogenic activity in the effluents was accounted for by E1 and E2 while EE2 was below
the LOD.
8.4 Occurrence of the synthetic hormone Ethynylestradiol
It was somewhat unexpected that only very few samples contained the synthetic hormone ethynylestradiol, which originate from the use of anti-contraceptive pills.
In more than 100 effluent samples from a broad range of WWTPs we only found EE2 a concentration exceeding 1 ng/L in six samples. We do find EE2 in nine of 15 influent samples and a high removal
efficiency in the more advanced WWTPs comprising the vast majority of the total effluent volume in Denmark. In the less advanced plants the elimination efficiency is low, but fortunately so are the influent
concentrations in this study (though probably only incidentally). Nevertheless, it is downstream of these plants EE2 is found in the water courses, and in the open land EE2 is detected in a drain from fields
treated with manure and in an effluent from isolated houses. Thus, although not common EE2 does occur and when it does it contributes with 25-75% of the total estrogenic activity.
The German WWTP survey published in 2001 (Körner et al., 2001; Spengler et al., 2001) demonstrated that advanced technology would remove nearly 100% of parent steroid estrogens, while the effluent
from a WWTP with a more simple technology (trickling filter) contained about 20 ng/L of E1 and 5-10 ng/L of E2 and EE2. The latter type of WWTP is roughly comparable to the categories C and D in this
study, where three of four detections of EE2 occur.
In the US survey of streams in risk of receiving effluents from WWTPs, it was shown that 5.7% of all samples contained EE2. It should be noted that the original publication (Kolpin et al. 2002a) states that
15.7% of samples with EE2, but a subsequent correction mentions 5.7% as the correct result (Kolpin et al.(2002b) in a response to a comment by Ericson et al., 2002). In the comparable categories of our
study (L-R), EE2 is found in approx. 2 % of the samples.
8.4.1 Samples with non-steroid estrogenic activity
The majority of data presented above should be interpreted in the light of the statistical uncertainty of the two methods, and as described earlier a ratio of 2.6 between YES and chemical data is suggested as
a criterion (Table 8.2). The uncertainties include standard analytical issues such as dilution effects, determination of recoveries etc, but also possible matrix effects and synergism.
In 82% of the effluent samples from WWTPs the chemical estimation of estrogenic activity based on analysis of three estrogens matches the biological determination. This proportion is generally similar in the
waters receiving effluents (86%) and the general aquatic environment (74%). It is in agreement with the findings reported in Kjølholt (2003), showing that steroid estrogens accounted for 80-95% of the
activity in treated waste water. This study did not include the simple WWTPs of categories C and D.
Table 8.2 Comparison of samples with detection both biologically and chemically. YES-to-Chemical ratio of 2.6 is significance criteria for matching values.
Category |
Total number of samples |
Samples with chemical and biological activity matches |
Samples with additional non-steroid estrogenic activity* |
Percentage of samples where YES and chemical data are matching |
WWTPs A-F |
60 |
49 |
11 |
82 |
Other sources
G-K |
11 |
5 |
6 |
46 |
Recipients L |
14 |
12 |
2 |
86 |
Surface waters M-R |
19 |
14 |
5 |
74 |
* Activity caused by other estrogens than E1, E2, áE2 and EE2 (possibly non-steroid).
One important prerequisite for interpretation is that the data are corrected for known losses in analytical procedures by internal recovery standards, but the data set is uncorrected for recovery as measured
against standards in quality control samples. This is a choice made to present the data as little manipulated as possible. Such a correction would increase the match between biological and chemical data. The
response factors are another parameter that affects the comparison of biological and chemically determined estrogenic activity. The response factors determined here are slightly lower than those reported by
Aerni et al (2004), who found 0.38 and 1.12 for E1 and EE2 respectively.
8.4.1.1 WWTPs
Of the 11 samples from A-F categories where ratios exceed 2.6, four are from C and D categories. These two categories also have high concentrations of steroid estrogens and the data demonstrates that
the efficiency in removing these substances and presumably also xenoestrogens is much smaller than in A-B and E-F. Thus, there is a suggestion in the data for C and D that non-steroid estrogen compounds
contribute to the observed YES activity. In their recent study of five WWTPs Aerni et al. (2004) do not attribute more than 1% estrogenic activity from e.g. nonylphenol although the contribution may be
higher from xenoestrogens in special cases.
8.4.1.2 Open land sources and surface waters
The unexpected high proportion of samples with additional estrogenic activity among the open land sources and to some extent the aquatic environment categories may be partly explained by the fact that in
many samples the E2-activity is less than 1 ng/L and the statistical error increases as the lower bound of the test's dynamic range is approached.
The activities in samples from G-K exceeding a ratio of 2.6 may have several causes: Two come from K-samples (fish farms) with a possible content of hormone disrupting agents from fodder. Samples from
the field drains may contain higher amounts of phytoestrogens, but are also much more prone to local effects from isolated houses and non-steroid estrogenic components in manure and sludge. In the aquatic
environment three such results come from “background” sites, which is surprising, but with the concentrations approaching the detection limits the data is less reliable.
Based on the available data it is therefore our preliminary conclusion that there is no indication of widespread occurrence of non-steroid estrogen related activity in the aquatic environments. In effluents from
particular sources (in particular categories C, D and possibly K) the activity from steroid estrogens is rivalled by non-steroid estrogen compounds.
| Front page | | Contents | | Previous | | Next | | Top |
Version 1.0 Januar 2005, © Danish Environmental Protection Agency
|