| Front page | | Contents | | Previous | | Next |
Background Report on Pre-validation of an OECD Springtail Test Guideline
3 Results and Discussion
3.1 Background
3.1.1 Ecology and Biology of Species
3.1.2 Ecotoxicology
3.1.3 Species inclusion in draft guideline
3.2 Sensitivity of species
3.2.1 Ecotoxicology - General sensitivity differences between the two species
3.2.2 Age- and size-toxicity relationships using dimethoate
3.2.3 Additional differences: Sex-ratio of off-spring
3.3 Test concentration ranges of possible ring-test compounds
3.3.1 Fipronil
3.3.2 3,5-dichlorophenol
3.3.3 Boric acid
3.3.4 Proposed test ranges for a ring-test
3.1 Background
3.1.1 Ecology and Biology of Species
The following summary is based on the chapter written by Wiles and Krogh (1998). The Collembola, commonly known as springtails, are the most numerous and widely occurring insects in terrestrial
ecosystems. This is one of the main reasons why they have been widely used as bioindicators for detecting the effects of environmental pollutants and different agricultural (especially pesticide) regimes on
biodiversity in soils (e.g. Paoletti et al. 1991; Crossley et al. 1992; Filser 1995; Filser et al. 1995; Frampton 1997; Paoletti and Bressan 1996, Fountain and Hopkin 2004 a, b). A wealth of literature exists
concerning the systematics, evolution, physiology, behaviour and ecology of these organisms (see Hale 1967; Wallwork 1970; Fjellberg 1985; Eisenbeis and Wichard 1987; Greenslade 1991; Christiansen
1990; Curry 1994 and Hopkin 1997).
Population densities of Collembola commonly reach 105 m-2 (e.g. Burges and Raw 1967; Peterson and Luxton 1982) in soil and leaf litter layers in many terrestrial ecosystems. Because of their small size
however, adults typically measure 0.5 - 5 mm long, their contribution to total soil animal biomass and respiration is low, estimated at being between 1 and 5% (see Petersen 1994). Their most important role
may therefore be as potential regulators of processes through microbivory and microfauna predation. Springtails are prey animals for a wide variety of endogeic and epigeic invertebrates, such as mites,
centipedes, spiders and carabid and rove beetles. The role of Collembola in humus formation is not well known, but it is commonly accepted that they may contribute significantly to the decomposition
processes on acidic stands, where earthworms and diplopods are absent.
3.1.2 Ecotoxicology
Collembola possess many attributes of the ideal experimental organism for ecotoxicological research. They are ecologically important, widely distributed, often highly abundant, easily sampled in the field, can
be cultured or maintained in the laboratory and have a relatively rapid life-cycle with a high reproductive rate (Spahr 1981). They lend themselves to studies over a wide range of spatio-temporal scales
including field monitoring of population trends (e.g. Filser 1995), field bioassays (e.g. Wiles and Frampton 1996), meso- and microcosm studies (e.g. Parmelee et al. 1993) and a wide variety of laboratory
investigations (e.g. Crommentuijn et al. 1993; Krogh 1995, Scott-Fordsmand et al 2000; van Gestel and Koolhaas 2004, da Luz et al 2004). They have been used in studies to investigate the environmental
effects of a wide range of environmental contaminants.
One of the earliest laboratory studies was undertaken by Sheals (1956) who studied effects of organochlorine compounds on microarthropod communities and used supplementary tests with filter paper to
screen for species differences in susceptibility to DDT. In a later study Scopes and Lichtenstein (1967) used 6 week old F. fimetaria in an acute test, also using exposure through filter paper. Their results
showed that the LD50 for adults was greater than twice the LD50 for juveniles, a fact that has since been used to explain interspecific differences between collembolan species of different sizes. In 1972
Thompson and Gore described an acute test on filter paper and in soil for a sexual population of F. candida. They were one of the first authors to promote the use of F. candida as a test species for
laboratory bioassays of pesticides. A large number of laboratory studies with Collembola followed in the 1970's, 80's and early 90's. In these studies a range of ten to fifteen different species of Collembola
were used, of which four species have been by far the most common; Folsomia candida, Folsomia fimetaria, Onychiurus armatus (Protaphorura armata), Orchesella cincta. Some of the first
standard acute toxicity tests with Collembola were suggested by Huang (1992) and Kiss and Bakonyi (1992) and more recently by Houx et al. (1996).
The first initiative to develop a standard sub-lethal test was taken at the Institute for Chemical Examination of the German Federal Biological Research Centre for Agriculture and Forestry. A description of
the background for the development of the resulting draft test method of sub-lethal testing with F. candida has been given by Riepert and Kula (1996). The development of a test with F. fimetaria was
started in Denmark in 1990 in a project on side-effects of pesticides funded by the Danish Ministry of the Environment (Løkke 1995). The choice of F. fimetaria was based on its wide distribution in
Danish agricultural soil and the ease with which it can be cultured in the laboratory (Petersen and Gjelstrup 1995). The procedures for culturing and conducting tests with F. fimetaria were described in
Krogh and Pedersen (1995) and Krogh (1995). Based on the above consideration these two species, F. fimetaria and F. candida, were chosen for the draft OECD guideline.
3.1.3 Species inclusion in draft guideline
The draft OECD guideline proposal includes a choice between two species (see Annex A). The most prominent reason for choosing a particular species for the toxicity test is the reason of relevance to the
compartment or life-characteristic which this toxicity study is supposed to represent, e.g. use a species present in agricultural soils when the test is used for agricultural risk assessment.
F. fimetaria is widely distributed and common in several soil types ranging from sandy to loamy soils and from mull to mor soils. It has been recorded in agricultural soils in Denmark (Christensen et al.
1987; Krogh 1994; Petersen and Gjelstrup 1995), Sweden (Andrén and Lagerlöf 1983), Finland (Huldén 1984), and Germany (Heimann-Detlefsen et al. 1994). It has an omnivorous feeding habit,
including fungal hyphae, bacteria, protozoa and detritus in its food. It interacts through grazing with infections of plant pathogenic fungi (Ulber 1983) and may influence mychorrhiza, as is known to be the
case for F. candida.
Folsomia fimetaria has a sexual mode of reproduction, whereas F. candida consists of females only with a parthenogenetic (asexual) reproductive mode. It is expected that when utilising a sexually
reproducing species the results will have a broader application, since the majority of species are assumed to have sexual reproduction.
F. candida is widely distributed throughout Europe. Although it is not common in most natural soils, it often occurs in very high numbers in humus rich sites. It is a blind, unpigmented collembolan which
reproduces parthenogenetically. It has a well developed furca (spring organ) and an active running movement and springs readily if disturbed.
3.2 Sensitivity of species
3.2.1 Ecotoxicology - General sensitivity differences between the two species
When deciding which of the two species have to be tested a relevant question is whether one of the two species proposed in the guideline can be assumed generally more sensitive than the other species.
There has hitherto been few studies dealing with differences in sensitivity between F. fimetaria and F. candida, and it is unknown whether this difference is important for their general sensitivity toward
chemicals. To study this a literature was performed and an array of experiments performed (see also 3.2.2).
With regard to the literature study two compounds were selected, i.e. the specific acting dimethoate (acetylcholinesterase inhibitor) and the toxicologically more broad acting metal Copper. Based on the
literature data for the two compounds no differences in sensitivity between the two species could be detected (see Table 4).
For dimethoate the mean EC50 for F. fimetaria was 1.8 [±1.1] dimethoate kg-1 and for F. candida it was 4.0 [±2.6] dimethoate kg-1, but a t-test showed no significant difference (P>0.05). As observed the means were more than 2-fold different, although not significant, which indicate a possible trend in F. fimetaria being more sensitive. This is in line with the observation by Krogh (1995) who exposed the two species under identical conditions and observed significant (P<0.05) differences in the EC10 values with regard to reproduction. They observed that for F. fimetaria the EC50 (reproduction) was 0.3 [0.14-0.47, 95% C.I.] mg kg-1 soil and for F. candida the EC50 (reproduction) was 0.5 [0.43-0.57, 95% C.I.] mg kg-1 soil. Significant differences were also observed on the mortality level, with F. fimetaria being the most sensitive. However, curiously the current laboratory data (see Fig 1) indicate that F. candida is more sensitive than F. fimetaria on a mortality level.
Studying the pooled literature data (Table 3) in detail reveals that much of the variation between the different reporting can probably be explained by differences in experimental conditions, such as
differences in the soil organic matter content. Differences in the exposure conditions, such as variations in soil type, may render the "bioavailable" fraction different under such conditions. To eliminate
differences due to exposure condition (especially soil type) the soil-water concentration could be calculated for KOW (Martikainen and Krogh 1999). Calculating the soil-water concentration by the
equation reported by Martikainen and Krogh (1995) it is observed that the ECx values are relatively closer for the two species, when expressed on mg dimethoate L-1 soil water (see Table 4) than when
based on soil concentrations.
Table 4. The EC50 values (reproduction) for Copper and dimethoate exposure of Folsomia fimetaria and Folsomia candida based on studies reported in the literature and recalculated to soil
water concentrations after Martikainen and Krogh 1999).
REFERENCE |
SPECIES |
COMPOUND |
EC50-soil (mg kg-1) |
Average (stdev) |
EC50-soil-water (mg L-1) |
Average (stdev) |
Sandifer and Hopkin (1996) |
Folsomia candida |
Copper |
700 |
|
|
|
Sandifer and Hopkin (1996) |
Folsomia candida |
Copper |
710 |
|
|
|
Sandifer and Hopkin (1996) |
Folsomia candida |
Copper |
1480 |
|
|
|
Sandifer and Hopkin (1997) |
Folsomia candida |
Copper |
700 |
|
|
|
Sandifer and Hopkin (1997) |
Folsomia candida |
Copper |
640 |
|
|
|
Pedersen et al (2000) |
Folsomia candida |
Copper |
657 |
|
|
|
Herbert et al (2004) |
Folsomia candida |
Copper |
813 |
|
|
|
Scott-Fordsmand et al (1997) |
Folsomia fimetaria |
Copper |
129 |
|
|
|
Pedersen and van Gestel (2001) |
Folsomia fimetaria |
Copper |
1244 |
|
|
|
Pedersen et al (2000) |
Folsomia fimetaria |
Copper |
519 |
|
|
|
Scott-Fordsmand et al (2000) |
Folsomia fimetaria |
Copper |
994 |
|
|
|
Martikainen (1996) |
Folsomia candida |
Dimethoate |
6.3 |
|
7,5 |
|
Martikainen (1996) |
Folsomia candida |
Dimethoate |
5.5 |
|
8,6 |
|
Martikainen (1996) |
Folsomia candida |
Dimethoate |
3.8 |
|
4,4 |
|
Krogh (1995) |
Folsomia candida |
Dimethoate |
0.5 |
4.0(±2.6) |
2,4 |
5.7(±2.8) |
Martikainen and Krogh (1999) |
Folsomia fimetaria |
Dimethoate |
0.8 |
|
3,7 |
|
Martikainen and Krogh (1999) |
Folsomia fimetaria |
Dimethoate |
1.7 |
|
4,8 |
|
Martikainen and Krogh (1999) |
Folsomia fimetaria |
Dimethoate |
3.2 |
|
5,2 |
|
Martikainen and Krogh (1999) |
Folsomia fimetaria |
Dimethoate |
2.5 |
|
4,6 |
|
Martikainen and Krogh (1999) |
Folsomia fimetaria |
Dimethoate |
2 |
|
6,0 |
|
Krogh (1995) |
Folsomia fimetaria |
Dimethoate |
0.3 |
1.8(±1.1) |
1,4 |
4.2(±1.5) |
For Cu the t-test comparison between the two species also exhibited no difference between the means with regard to reproduction (P>0.05). The mean for F. fimetaria was 722 [±496] Cu kg-1 and F.
candida was 814 [±299] Cu kg-1. This is in line with observations by Pedersen et al (1999) who observed no differences in the copper EC50 values for the two species when exposed under identical
conditions. As for dimethoate soil type models could be considered resolving the differences between the soil types, for example the biological ligand-model (BLM). The latter conversion was not performed
as the models are presently under development and analysis of the experiments showed that for example temperature and pH may be as important for toxicity as soil type (Sandifer and Hopkin 1996, 1997).
In conclusion no difference in toxicity between the two species could be identified, but a possible difference in toxicity may be blurred by confounding factors.
3.2.2 Age- and size-toxicity relationships using dimethoate
Folsomia fimetaria and F. candida can appear quite alike from a visual point-of-view, but they differ in size with F. candida being the larger. From a pure theoretical point-of-view it could be argued that
the two species are likely to differ in sensitivity as the surface-to-volume ratio is related to the size. Such sensitivity differences could be very relevant when choosing which species to test.
Based on an experimental study (see 2.1.2.2) growth of F. candida was faster than F. fimetaria as would be expected from previous knowledge of this species (data not shown). The toxicity decreased
with age with juveniles being most sensitive and it decreased faster for juvenile F. fimetaria than for F. candida (Fig 1). Furthermore, there is a clear indication of increased sensitivity of the reproductive
instars about age 15 and 22 days (every second instar is reproductive and occurs approx. weekly). Thus, juvenile F. fimetaria was the least sensitive to dimethoate, which is different from a previous
studying showing adult F. fimetaria to be the most sensitivity (Krogh 1995). Hence, it seems that the sensitivity is life-stage dependent as confirmed on a refined level in this study (Fig 1). In fact it is seen
that both species have very contrasting sensitivities in the days around the age for which they are selected in the draft OECD test. For example, in the F. candida test the start age should be 10-12 days, but
if the organisms tend to rather be 9-11 days than 12-14 days the may change the sensitivity two fold (see Fig 1). It is important to realise such small differences may not only be due to a selection based on
number of days (age) for the organisms, but a slight increase or drop in temperature may also cause the organisms to be in a different life-stage at the start of the test. In fact it is seen that both species have
very contrasting sensitivities around the age for with they are selected for the OECD test. For example, in the F. candida test the start age should be 10-12 days, but if the organisms tend to be 9-11 days
rather than 12-14 days this may increase the sensitivity two fold (see Fig 1). It is important to realise such small differences may not only be due to a selection based on number of days (age) for the
organisms, but a slight increase or drop in temperature may also cause the organisms to be in a different life-stage.
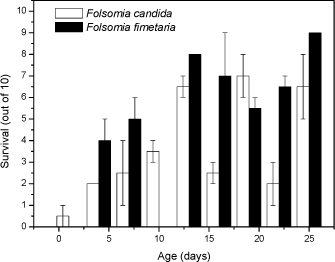
Fig 1. Survival (out of 10, mean ± standard error) of nine age-/size-classes for Folsomia candida and Folsomia fimetaria exposed for 7 days to dimethoate, 2 mg kg-1 in 5% OECD soil.
In conclusion, difference in toxicity between the species could be detected, and a pronounce life-stage toxicity dependency was present for both species.
3.2.3 Additional differences: Sex-ratio of off-spring
Given that F. fimetaria is a sexually reproducing species it is possible that compounds which introduces changes in the sex-ratio of the offspring can be identified when using this species. Measurements of
the offspring sex-ratio, if successful, would be a simple addition to, and involve a minor change of, the overall test design of the general guideline for long-term Collembola toxicity tests. Hence, based on
these considerations it was decided to attempt the sex-ratio approach.
The simplest way, and the approach taken, to identify a bias in the offspring sex-ratio was to extend the exposure period ensuring that the offspring would be of sufficient age (19-23 days) for sex
identification. Given the excessive man-power that would be required if each individual should be sexed under the microscope it was decided to use the size characteristics to class animals into sexes, this
latter could be done by employing the data produced by the digital image processing counting (see Fig 2). In performing this it is necessary to have an synchronous culture partly enabling the addition for
females and males at the beginning of the experiment, partly to ensure synchronised clutches and thus avoiding overlap of size classes. An overlap in size-classes will make sex-determination extremely
difficult by the termination of the experiment. It was decided to extend the exposure period to 42 days, which would allow for the first clutch to become adult, hence allowing for sex-ratio determination of
this.
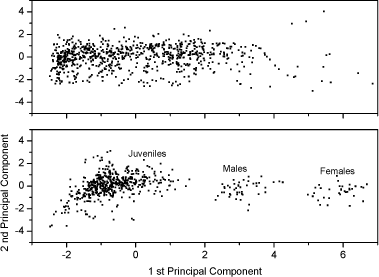
Fig 2. PCA plot of the first and second principal component scores of the original 5 dimension data: area, length, width, slimness and grey density. Lower Fig: three Folsomia fimetaria clusters can be identified: females, males and juveniles following exposure to copper (21, days of exposure, Scott-Fordsmand et al 1997). Upper Fig: No clusters can be identified following exposure to testosterone (42 days of exposure).
Two chemicals, methyl-testosterone and ethynyl-estradiol, are suggested as reference chemicals for evaluating potential endocrine-disrupting compounds in invertebrates (Ingersoll et al 1999). There is at
present no clear evidence for whether these compounds are involved in the sex-determination of invertebrates. Testosterone has been reported to have some androgen-like activity in some crustaceans
LeBlanc (1999) and endogenous concentrations of testosterone and other vertebrate-type androgens have been reported in Daphnia magna and Neomysis integer (LeBlanc and MacLachlan 2000,
Verslycke et al 2002, 2004). Estradiol has been recorded in the silk moth Bombyx mori (Keshan and Ray 2001). However, neither estradiol nor testosterone affected the sex-ratio in exposed Daphnia
magna (Kashian and Dodson 2004). No studies have previously been reported for Collembola.
Upon counting and sizing the offspring from the conducted soil-tests it was realised that with the employed approach it was not possible to identify the sex-ratio of the offspring. The problem seemed to be
that the juvenile growth was not sufficient synchronous under the present conditions and the individual clutches overlapped, hence a "small" male from the first clutch had the same size as a "large" female from
the second clutch (see Fig 2). It could then have been resolved to identifying the sex of the individual animals, but as the tests resulted in more than 18,000 animals per test this would have been an almost
impossible task. The present result indicates that the sex-ratio identification is not applicable with the present approach (see Fig 2). Other approaches may be considered in the future.
In fact another approach was partly followed using short-term exposure to an aquatic solution. Based on aquatic solutions adult Collembola were exposed for 24 hours to 12 mg testosterone L-1 or 2,0 mg
estradiol L-1 after which the adults were allowed to produce eggs over a period of 7 days. The hatched juveniles were allowed to growth for 23 days prior to size measuring. No bias in the sex-ratio could
be identified (Fig 3). No comparable literature data was available.
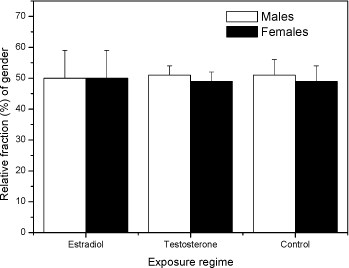
Fig 3 Relative fraction of gender Folsomia fimetaria following juvenile exposed to water, estradiol (12 mg L-1) and testosterone (2 mg L-1) following exposure of parent generation for 48 hours in an aqueous solution. Columns represent mean ± standard deviation.
3.3 Test concentration ranges of possible ring-test compounds
In connection to the test-guideline and a ring-test programme "standardized" test compounds should be available. Such compounds can be selected on a wide range of criteria, such as how non-toxic it is to humans, how easy it is to store etc. In the present project three compounds were tested, fipronil, 3,5-Dichlorophenol and boric acid. The three compounds were selected on basis of the inclusion in similar test programmes. Two of these compounds, fipronil and 3,5-DCP are currently used in the Copepod ring-test and included here upon the request from the Danish Environmental Protection Agency. Boric acid is suggested as positive control in soil test in North America. Previously, dimethoate has been suggested as a positive control in Collembola tests, but considering that this compound may be taken off the marked it is suggested to avoid using this.
As the above sensitivity studies indicated that the difference between the two species would be less than what is important for determining future test ranges, it was decided to test two of the compounds on
F. fimetaria only. In other words, the F. fimetaria test ranges is supposed also to cover the F. candida test ranges. For boric acid both species were tested, confirming that the test range covering F.
fimetaria also covered F. candida.
3.3.1 Fipronil
Fipronil has been suggested as a positive control in the Copepod ring-test programme. It is an insecticide, mainly used on pets for flea elimination (for a detailed review on this see Tingle et al 2003). As such
it should be highly toxic to arthropods, as also confirmed with the present results. For F. fimetaria exposed through the soil fipronil had EC10 and EC50 (based on nominal concentrations) values of 0.58
mg fipronil kg-1 and 1.89 mg fipronil kg-1, respectively. Based on these tests, the ring-test test ranges should be 0-10 mg fipronil kg-1 (see Fig 4).
Many data are available with regard to effects of fipronil on invertebrates, but few with soil exposed organisms. Soil toxicity data were only available for earthworms showing LC50 values above 8550 mg
kg-1 (Mostert et al 2002). As fipronil is an insecticide it is of little surprise that it is relatively non-toxic to earthworms. No soil toxicity data were found for Collembolans.
In conclusion: Based on these tests, the ring-test test ranges should be 0-15 mg kg-1 for fipronil.
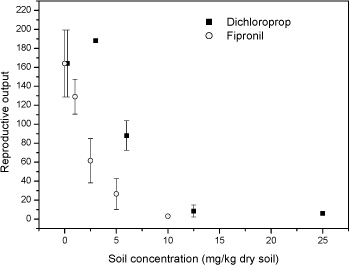
Fig 4. Number of juveniles (mean ± standard deviation) Folsomia fimetaria, following exposure of adult Collembola to fipronil or 3,5-chlorophenol for 21 days.
3.3.2 3,5-dichlorophenol
3,5-Dichlorophenol (3,5-DCP) has been suggested as a positive control in the Copepod ring-test programme. For F. fimetaria exposed through the soil 3,5-DCP had EC10 and EC50 (based on nominal
concentrations) values of 3.07 mg 3,5-DCP kg-1 and 5.53 mg 3,5-DCP kg-1, respectively (see Fig 5). Many data are available with regard to chlorophenol effects on invertebrates, but no 3,5-DCP
toxicity data for soil organisms were available. For F. candida Weigmann et al (1985) reported an LC50 value (24 hr exposure) for PCP at 32 ppm. Being weak acids, chlorophenol dissociate at a given
pH in accordance with their pKa values. Hence, it can be inferred that the solubility alters much with environmental changes in pH if the pKa is close to the environmental pH. The pKa of 3,5-DCP is 8.54
indicating that at a soil pH around 6 (as in the present test) more than 99% is on the non-ionized state.
In conclusion: Based on these tests, the ring-test test ranges should be 0-15 mg 3,5-DCP kg-1 using the reproduction test.
3.3.3 Boric acid
Boric acid is suggested as positive control in North America and has been used in many plant toxicity studies. The toxicity of boric acid was studied for both species, first in a short-term test (F. fimetaria)
and second in a reproduction study (F. fimetaria and F. candida). According to the guideline the two test should last 21 and 28 days respectively. It was, however, chosen to use a 21-day exposure for
both species in order to be able to compare the sensitivity between the two species.
The present studies revealed LC10 and LC50 values of 394 mg boric acid kg-1 and 648 mg boric acid kg-1 for F. fimetaria in a 14-day experiment (see table 5). Based on this it was decided to run the
reproduction test with concentrations ranging for 0 to 1000 mg boric acid kg-1 for both species. In the reproduction test, which lasted 7 days more than the preliminary test, the mortality proved higher
resulting in lower LCx values. The LC10 was below 200 mg boric acid kg-1 for both species. Applying dose-response modelling the EC10 and EC50 values were 24 and 117 mg boric acid kg-1 for F.
fimetaria and 22 and 111 mg boric acid kg-1 for F. candida. The latter ECx values should be considered with great care since no test concentration below 200 mg boric acid kg-1 was employed. Time
did not allow for a repetition of the experiment, besides the experiment is valid for a test-range identification. Becker-van Slooten (2004) reports ECx values corresponded with results obtained in the
present study (see Table 5).
Table 5. LCx and ECx concentrations (mg kg-1) for Folsomia fimetaria (14-days and 21-day) and Folsomia candida (21-day) exposed to boric acid in soil.
Reference |
Species |
LC10 |
LC50 |
EC10* |
EC50* |
14-day test |
|
|
|
|
|
This report |
F. fimetaria |
394
[225-563] |
648
[477-819] |
|
|
Becker van Slooten (2004) |
F. candida |
|
800
[633-1012] |
|
|
21-day test |
|
|
|
|
|
This report |
F. fimetaria |
< 200 |
>200 |
24±1
[21-26] |
117±3
[107-1289] |
This report |
F. candida |
<200 |
<200 |
22±1
[21-229] |
111±3
[103-121] |
28-day test |
|
|
|
|
|
Becker van Slooten (2004) |
F. candida |
|
|
|
147
[137-156] |
* Effect concentrations, EC10 and EC50, and bootstrapping intervals (equivalent to 95 % confidence intervals, ICP Nordberg-King, 1993). The studies by Becker van Slooten et al. (2004) is based on a
10% OECD soil
In conclusion: Based on the given data the following test concentration ranges are suggested for a ring-test: 0 to 300 mg boric acid kg-1 dry soil. The EC50 (reproduction) should be in the range of 100-150
mg boric acid kg-1 and the LC50 needs to be better defined in the ring test.
3.3.4 Proposed test ranges for a ring-test
Based on the three above tests, the ring-test test ranges should be 0-10 mg kg-1 for fipronil, 0-15 mg kg-1 for 3,5-dichlorophenol and 0-300 mg kg-1 for boric acid using reproductive output as a test
endpoint.
| Front page | | Contents | | Previous | | Next | | Top |
Version 1.0 March 2005, © Danish Environmental Protection Agency
|