| Front page | | Contents | | Previous | | Next |
Background for spatial differentiation in LCA impact assessment - The EDIP2003 methodology
4 Terrestrial eutrophication
4.1 Introduction
4.2 The RAINS model
4.2.1 Emission estimates
4.2.2 Exposure assessment
4.2.3 Effect measures
4.3 Calculation procedure
4.4 Results
4.5 Discussion
4.6 Conclusions and recommendations
4.6.1 Acknowledgement
4.7 References
Authors: José Potting [21], Wolfgang Schöpp [22] and Michael Hauschild [23]
4.1 Introduction
The eutrophying impact usually characterised in life cycle impact assessment refers to eutrophication of aquatic ecosystems. This follows from the modelling of impact that in life cycle assessment typically
takes its basis in the composition of aquatic biomass (Wenzel et al. 1997, Heijungs et al. 1992, Lindfors et al. 1995). Until recently, Finnveden et al. (1992) were the only ones explicitly addressing
eutrophication of terrestrial ecosystems. They proposed to separate between summing together waterborne and airborne nitrogen emissions [24] because terrestrial ecosystems get eutrophied mainly via
nitrogen emissions to air (waterborne nutrients rarely contribute to terrestrial eutrophication) [25].
The contribution to terrestrial eutrophication in the proposal of Finnveden et al. (1992) consists of the airborne nitrogen emission accumulated over the full life cycle of the considered product. Obviously,
such an approach does not pay attention to the site where an emission is released, nor to the fate and exposure and sensitivity of the subsequent environments where the released nitrogen is received, and
where the loading increase may lead to impact. This lack of spatial differentiation affects the relevance of the assessed impact, as was clearly demonstrated by Potting and Blok (1994, 1995).
Potting et al. (1998a,b) developed a framework for constructing factors that relate the region of emission to the impact on its deposition areas (see also Chapter 3). This framework is used to establish
characterisation factors for 44 European regions and for the impact categories of acidification, ground-level ozone formation and terrestrial eutrophication. The factors were established with the help of the
RAINS model.
This chapter reports the results for the terrestrial eutrophication factors. Section 4.2 illustrates RAINS step by step with the several outputs of the model. The procedure for calculating the terrestrial
eutrophication factors is exemplified in Section 4.3, and the results are presented and discussed in Section 4.4. Section 4.5 draws some main conclusions.
4.2 The RAINS model
RAINS is an integrated assessment model that combines information on regional emission levels with information on long-range atmospheric transport in order to estimate patterns of deposition and
concentration for comparison with critical loads and thresholds for acidification, eutrophication and tropospheric ozone formation. Relating the site of an emission to the impact on its deposition areas is one
of the key elements in the RAINS. This model (version 7.2) has therefore been used, similar as for acidification (see Chapter 3), to establish site-factors for terrestrial eutrophication.
Alcamo et al. (1990) and Amann et al. (1995) provide a detailed description of the model. Section 3.3 gives a technical summary of the parts of RAINS that are relevant for this chapter. This section
illustrates this technical summary step by step with the several outputs of the RAINS model.
4.2.1 Emission estimates
Typical distances between an emission source and its locations of deposition are easily several hundreds of kilometres. The geographical scale of analysis, accordingly, has to be large in order to cover most
of the impact from an emission source. The RAINS model focuses therefore on the regional dimension (rather than on the local dimension) of the assessed environmental impact. (Alcamo et al. 1990, Barret
and Berge 1996)
Though phosphorus is a key nutrient in eutrophication of inland waters (rivers and lakes), it has only minor relevance for terrestrial ecosystems since they are under natural conditions rarely limited in their
growth by phosphorus. The soil can provide its own phosphorus by weathering or by mineralisation of indigenous organic matter. There may also be phosphorus input by atmospheric deposition of pollen
and dust (for Sweden estimated to be 0.07 kg per hectare per year). Depositions of phosphorus of anthropogenic origin are small [26] compared to phosphorus deposition by pollen or dust. (Chardon
2000, Berdowski and Jonker 1994)
Emissions of nitrogen oxides and ammonia are the principal contributors to terrestrial eutrophication. Energy consumption is the main emission source of nitrogen oxides. Ammonia emissions stem
predominantly from livestock farming and agricultural use of fertiliser. The 7.2 version of the RAINS model incorporates sectoral databases on energy consumption and agricultural activity for 44 regions in
Europe.
The calculations in this article are based on the scenarios for nitrogen emissions only. The relevant scenarios are presented in Table 4.1 (for the year 1990) and Table 4.2 (for the year 2010). The emission
levels for 1990 reflect the actual situation, whereas those for 2010 are a forecast. Section 3.3 gives more details about the background of the numbers.
Table 4.1. Total emissions for the year 1990 are given for each region (column 1) by substance (columns 2 and 3). Columns 4 up to, and including column 9represent the total area of that region (column 4),
the total area of ecosystem in that region (column 5), the total area of unprotected ecosystem in that region (column 6) as a percent of total area (column 7), the total area of ecosystem that gets unprotected
by the total emission from that region (column 8; normalisation factors), and the terrestrial eutrophication factors per substance for that region (columns 9and 10).
Region |
Emissions
NOx NH3
(kton) (kton)
|
Total area of:
Region Ecosystem Unprotected ecosystem
in region by region
(1000 ha) (1000 ha) (1000 ha) (%) (1000 ha)
|
Terrestrial
eutroph-
ication
NOx NH3 (ha/ton) (ha/ton)
|
Albania |
|
29.99 |
30.00 |
2881 |
1062 |
113 |
11 |
368 |
1.58 |
6.91 |
Austria |
|
221.97 |
91.00 |
8373 |
4872 |
4531 |
93 |
1988 |
1.03 |
3.38 |
Belarus |
|
284.95 |
257.00 |
20706 |
1901 |
1757 |
92 |
1834 |
1.67 |
2.81 |
Belgium |
|
352.00 |
95.00 |
3054 |
621 |
621 |
100 |
945 |
1.44 |
1.10 |
Bosnia Herzegovina |
|
79.76 |
36.00 |
5151 |
1449 |
967 |
67 |
898 |
2.97 |
13.33 |
Bulgaria |
|
375.97 |
140.63 |
11102 |
3782 |
3393 |
90 |
3945 |
1.02 |
9.06 |
Croatia |
|
82.95 |
37.00 |
5640 |
1638 |
976 |
60 |
678 |
1.52 |
6.21 |
Czech Republic |
|
741.98 |
105.00 |
7904 |
2656 |
2627 |
99 |
1632 |
1.68 |
2.52 |
Denmark |
|
268.98 |
140.00 |
4217 |
974 |
593 |
61 |
2947 |
5.33 |
9.80 |
Estonia |
|
71.98 |
29.00 |
4549 |
1891 |
654 |
35 |
907 |
6.63 |
42.02 |
Finland |
|
299.92 |
41.00 |
33449 |
32208 |
4465 |
14 |
4470 |
11.29 |
91.69 |
France |
|
1585.00 |
700.00 |
54783 |
14483 |
10000 |
69 |
13210 |
2.93 |
9.15 |
Germany new
Germany old
|
|
693.97
2376.93
|
205.00
554.00
|
} 35642 |
} 8693 |
} 8596 |
} 99 |
2688
13553
|
2.15
2.04
|
3.64
4.86
|
Greece |
|
305.91 |
78.00 |
12582 |
2455 |
204 |
8 |
790 |
0.56 |
15.67 |
Hungary |
|
237.98 |
176.00 |
9297 |
1620 |
1601 |
99 |
2978 |
1.70 |
5.67 |
Ireland |
|
114.97 |
126.00 |
6900 |
489 |
0 |
0 |
116 |
0.37 |
0.51 |
Italy |
|
2047.00 |
416.00 |
30174 |
6627 |
1992 |
30 |
6404 |
1.12 |
13.26 |
Latvia |
|
92.98 |
38.00 |
6441 |
2716 |
1486 |
55 |
1252 |
3.92 |
7.69 |
Lithuania |
|
157.98 |
84.00 |
6498 |
1896 |
1863 |
98 |
1979 |
3.23 |
5.72 |
Luxembourg |
|
23.00 |
7.00 |
260 |
88 |
88 |
100 |
6 |
0.10 |
0.16 |
Netherlands |
|
574.98 |
236.00 |
3610 |
320 |
312 |
98 |
2225 |
1.91 |
2.30 |
Norway |
|
229.90 |
39.00 |
31752 |
32065 |
659 |
12 |
1755 |
6.29 |
10.11 |
Poland |
|
1279.00 |
508.00 |
31119 |
6372 |
6345 |
99 |
9089 |
2.15 |
4.39 |
Portugal |
|
215.00 |
93.00 |
8884 |
2829 |
570 |
20 |
1136 |
3.11 |
30.74 |
Moldova |
|
34.98 |
50.00 |
2917 |
8 |
3 |
36 |
168 |
0.16 |
1.18 |
Romania |
|
546.00 |
300.00 |
23713 |
6234 |
1667 |
27 |
3252 |
1.29 |
5.18 |
Kalingrad region
Kola, Karelia
Remaining Russia
St. Petersburg reg.
|
|
16.00
47.94
2500.80
109.96
|
11.23
5.64
1129.16
44.97
|
} 373489 |
} 345607 |
} 1162 |
} 0 |
14
100
2012
659
|
0.21
0.72
0.55
3.37
|
0.92
5.07
0.57
5.93
|
Slovakia |
|
226.98 |
62.00 |
4836 |
1992 |
1957 |
98 |
1622 |
1.34 |
6.27 |
Slovenian |
|
57.00 |
27.00 |
2029 |
906 |
624 |
69 |
781 |
1.09 |
10.22 |
Spain |
|
1178.00 |
353.00 |
49525 |
8523 |
1949 |
23 |
4699 |
2.44 |
13.40 |
Sweden |
|
410.91 |
61.00 |
44469 |
43650 |
3837 |
19 |
5073 |
11.97 |
70.06 |
Switzerland |
|
165.00 |
62.00 |
4126 |
1189 |
1707 |
81 |
1445 |
0.90 |
5.76 |
Macedonia |
|
38.84 |
16.78 |
2537 |
1066 |
376 |
35 |
314 |
0.25 |
13.66 |
Ukraine |
|
1096.83 |
926.00 |
57977 |
8253 |
6968 |
84 |
5814 |
0.62 |
3.42 |
United Kingdom |
|
2701.92 |
320.00 |
23103 |
7890 |
530 |
7 |
6040 |
1.77 |
3.14 |
Yugoslavia |
|
210.84 |
99.00 |
10215 |
3413 |
1771 |
52 |
2402 |
5.55 |
35.96 |
Atlantic ocean |
|
348.57 |
0.00 |
|
|
|
|
186 |
0.96 |
|
Baltic sea |
|
81.00 |
0.00 |
|
|
|
|
507 |
6.20 |
|
Mediterranean sea |
|
13.00 |
0.00 |
|
|
|
|
7 |
0.08 |
|
North sea |
|
191.91 |
0.00 |
|
|
|
|
362 |
1.86 |
|
Table 4.2. Total emissions for the year 2010 are given for each region (column 1) by substance (columns 2 and 3). Columns 4 up to, and including column 9represent the total area of that region (column 4),
the total area of ecosystem in that region (column 5), the total area of unprotected ecosystem in that region (column 6) as a percent of total area (column 7), the total area of ecosystem that gets unprotected
by the total emission from that region (column 8; normalisation factors), and the terrestrial eutrophication factors per substance for that region (columns 9and 10).
Region |
Emissions
NOx NH3
(kton) (kton)
|
Total area of:
Region Ecosystem Unprotected ecosystem
in region by region
(1000 ha) (1000 ha) (1000 ha) (%) (1000 ha)
|
Terrestrial eutroph-
ication
NOx NH3
(ha/ton) (ha/ton)
|
Albania |
|
30.00 |
33.81 |
2881 |
1062 |
69 |
6 |
173 |
0.80 |
3.12 |
Austria |
|
116.01 |
94.54 |
8373 |
4872 |
3012 |
62 |
3292 |
2.86 |
28.62 |
Belarus |
|
184.00 |
162.98 |
20706 |
1901 |
1570 |
83 |
2113 |
0.98 |
2.45 |
Belgium |
|
196.17 |
105.86 |
3054 |
621 |
599 |
96 |
1834 |
1.78 |
2.45 |
Bosnia Herzegovina |
|
47.95 |
22.98 |
5151 |
1449 |
328 |
23 |
578 |
6.61 |
30.29 |
Bulgaria |
|
290.00 |
125.65 |
11102 |
3782 |
2685 |
71 |
3039 |
1.18 |
17.50 |
Croatia |
|
63.87 |
37.85 |
5640 |
1638 |
453 |
28 |
878 |
5.99 |
25.36 |
Czech Republic |
|
226.12 |
124.59 |
7904 |
2656 |
2314 |
87 |
3390 |
2.62 |
9.70 |
Denmark |
|
119.00 |
103.00 |
4217 |
974 |
357 |
37 |
1045 |
2.13 |
6.04 |
Estonia |
|
72.00 |
28.71 |
4549 |
1891 |
508 |
27 |
990 |
2.89 |
9.29 |
Finland |
|
162.51 |
29.80 |
33449 |
32208 |
756 |
2 |
793 |
3.40 |
79.00 |
France |
|
895.21 |
668.61 |
54783 |
14483 |
6091 |
42 |
10389 |
9.10 |
20.03 |
Germany new
Germany old
|
|
296.64
982.36
|
139.66
398.98
|
} 35642 |
} 8693 |
} 7083 |
} 81 |
3813
11577
|
2.36
3.01
|
8.00
12.66
|
Greece |
|
282.07 |
75.96 |
12582 |
2455 |
91 |
4 |
757 |
0.42 |
2.04 |
Hungary |
|
196.00 |
136.82 |
9297 |
1620 |
623 |
38 |
2512 |
7.33 |
20.73 |
Ireland |
|
73.20 |
126.00 |
6900 |
489 |
0 |
0 |
82 |
0.15 |
0.19 |
Italy |
|
1164.80 |
390.93 |
30174 |
6627 |
1193 |
18 |
4513 |
2.16 |
14.28 |
Latvia |
|
93.00 |
29.32 |
6441 |
2716 |
507 |
19 |
760 |
2.31 |
13.05 |
Lithuania |
|
136.57 |
80.56 |
6498 |
1896 |
1655 |
87 |
2365 |
2.11 |
14.98 |
Luxembourg |
|
10.37 |
6.40 |
260 |
88 |
85 |
97 |
37 |
1.30 |
3.61 |
Netherlands |
|
138.00 |
82.00 |
3610 |
320 |
271 |
85 |
1246 |
1.69 |
3.01 |
Norway |
|
161.00 |
39.17 |
31752 |
32065 |
276 |
5 |
537 |
1.09 |
0.75 |
Poland |
|
821.48 |
545.93 |
31119 |
6372 |
5657 |
88 |
8752 |
2.41 |
9.97 |
Portugal |
|
205.98 |
84.10 |
8884 |
2829 |
277 |
10 |
701 |
9.40 |
27.66 |
Moldova |
|
65.69 |
47.65 |
2917 |
8 |
2 |
20 |
69 |
0.23 |
1.05 |
Romania |
|
452.67 |
301.22 |
23713 |
6234 |
1096 |
18 |
3138 |
2.09 |
7.02 |
Kalingrad region
Kola, Karelia
Remaining Russia
St. Petersburg reg.
|
|
23.60
81.87
2392.30
160.22
|
11.41
4.21
845.16
33.73
|
} 373489 |
} 345607 |
} 166 |
} 0 |
119
10
648
322
|
0.62
0.21
0.13
1.47
|
2.80
1.73
0.22
7.82
|
Slovakia |
|
110.29 |
53.42 |
4836 |
1992 |
1137 |
57 |
1604 |
2.69 |
30.27 |
Slovenian |
|
31.00 |
20.41 |
2029 |
906 |
220 |
24 |
748 |
2.38 |
21.83 |
Spain |
|
851.48 |
373.43 |
49525 |
8523 |
1179 |
14 |
2547 |
3.71 |
16.02 |
Sweden |
|
207.30 |
53.00 |
44469 |
43650 |
91 |
0 |
792 |
2.75 |
6.24 |
Switzerland |
|
78.35 |
58.00 |
4126 |
1189 |
1243 |
59 |
1628 |
2.65 |
24.78 |
Macedonia |
|
22.20 |
15.62 |
2537 |
1066 |
243 |
23 |
323 |
0.26 |
10.82 |
Ukraine |
|
1094.00 |
648.96 |
57977 |
8253 |
5426 |
66 |
6247 |
0.47 |
3.40 |
United Kingdom |
|
1224.46 |
270.26 |
23103 |
7890 |
42 |
1 |
2654 |
0.84 |
0.89 |
Yugoslavia |
|
118.41 |
82.88 |
10215 |
3413 |
705 |
21 |
1493 |
3.74 |
15.16 |
Atlantic ocean |
|
349.79 |
|
|
|
|
|
985 |
0.39 |
0.22 |
Baltic sea |
|
81.13 |
|
|
|
|
|
291 |
2.72 |
0.48 |
Mediterranean sea |
|
13.30 |
|
|
|
|
|
0 |
0.02 |
0.00 |
North sea |
|
191.52 |
|
|
|
|
|
921 |
1.15 |
0.88 |
Table 4.3. Total nitrogen dioxide emission for Albania, Belgium and Finland in 1990 (Amann et al. 1996) and the breakdown into main economic sectors (abstracted from the RAINS model; version 7.2).
Economical sector |
Albania |
Belgium |
Finland |
Fuel conversion
Industrial combustion
Industrial processes
Centralised power plants & district heating
Domestic
Transportation
Otherwise
|
4
21
6
12
5
51
0
|
%
%
%
%
%
%
%
|
2
8
10
17
5
57
<1
|
%
%
%
%
%
%
%
|
<1
14
4
17
3
62
<1
|
%
%
%
%
%
%
%
|
Total |
30 |
kton |
352 |
kton |
300 |
kton |
Table 4.3 gives, as an example, the breakdown into the main economic sectors of nitrogen oxide emissions for Albania, Belgium and Finland. Every economic sector can be further broken down into finally
the separate sources. The breakdown shows that basically all economic sectors, except transport, have a relative small share in the total nitrogen oxide emission per country. Each of these sectors aggregates
the contributions of a large number of underlying individual sources. Obviously, the shares of the individual sources in the total are even smaller if not marginal compared to the sector aggregates. This applies
also to the transport sector, which is responsible for more than 50% of all nitrogen oxide emissions.
4.2.2 Exposure assessment
The RAINS model estimates dispersion and deposition of nitrogen compounds with help of "region to grid" matrices on grid elements (150km resolution) for each of the 44 regions in Europe. The grid
consists of 612 elements covering all 44 European regions, including the European part of the former Soviet Union. Figure 4.1 shows the dispersion and deposition resulting from total nitrogen emission in
Finland (Section 3.3.2 gives more details about the model)
Total deposition within one grid-element is computed by adding together the contributions from each of the 44 regions in Europe (their dispersion and deposition pattern being calculated similarly as done for
Finland in Figure 4.1). Figure 4.2 shows the dispersion and deposition from the emissions of all European regions together.
The RAINS model offers the possibility for each grid-element to quantify the contributions from each region in Europe. Table 4.4 gives such breakdown for the Finnish grid-element indicated by the arrow in
Figure 4.2. The contribution from Finland's own emissions to deposition on this grid-element, as can be seen from Table 4.4, is only a little more than 20% of total deposition (almost 80% thus being
imported from abroad). Hence the Finnish contribution to this grid-element stems from total Finnish emissions which sources are distributed over the full Finnish area (although this grid-element, being the
economic centre of Finland, may have a relatively high source-density).
Table 4.4. The shares of European regions in the total deposition of nitrogen on the southern part of Finland (grid-element x=20, y=25 indicated by the arrow in Figure 4.2) resulting from nitrogen oxide
emissions.
Click here to see the Figure.
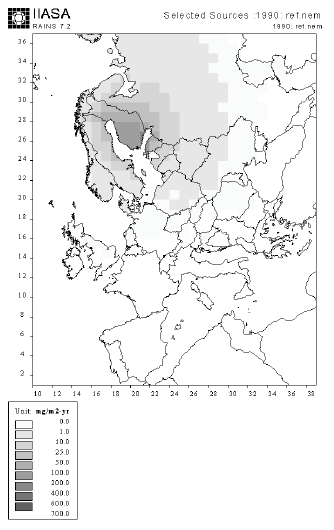
Figure 4.1. Dispersion and deposition pattern of the total emission of nitrogen from Finland
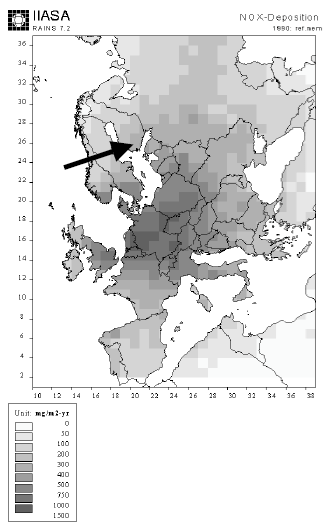
Figure 4.2. Total dispersion and deposition from emission of nitrogen from all regions in Europe. The arrow indicates a region in Finland (see text)
The region-to-grid matrices in the RAINS model are based on the Lagrangian or trajectory model developed by EMEP (co-operative Program for Monitoring and Evaluation of the long-range transmission
of air pollutants in Europe (Barrett and Berge 1996)). Section 3.3.1 gives more details about the background of the model.
4.2.3 Effect measures
Nitrogen is an essential nutrient for all species and an increase of nitrogen level is therefore not harmful until a certain level has been reached. Each ecosystem, and as a matter of fact each species being part
of that, has an optimum curve for the growth related to increasing nitrogen supplies. The optimum levels differ between the various types of ecosystems as illustrated in Figure 4.3.
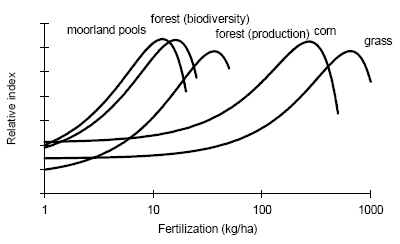
Figure 4.3. Optimum curves for different systems in relation to nitrogen fertilisation (fictive curves) (taken from Erisman 2000)
If nitrogen levels exceed the optimum for the typical species in a given ecosystem, the growth of those species start to decline whereas growth is stimulated for other species better able to benefit from the
increased availability of nitrogen. Downing et al. (1993) suggest critical levels of nitrogen in soil at which changes of vegetation composition are induced. These critical levels are used in RAINS to model
critical nitrogen depositions or loads above which these changes of vegetation are expected.
Similar to what has been done for acidification, critical loads for eutrophication of terrestrial ecosystems have been estimated for the whole of Europe. Ecosystems covered are mainly forests, but for some
countries also heathland and grassland. The critical loads are per grid-element summarised in a cumulative distribution curve for eutrophication (see Figure 4.4). While only few grid-elements contain less than
10 ecosystems, there are several with more than 10,000 ecosystems per grid-element (the highest number being 53,000 in a grid-element shared by the Netherlands, Belgium and Germany). (Posch et al.
1997) The full critical load database in the RAINS 7.2 version was based on almost 700,000 ecosystems (the most recent version relates to over 1.4 million ecosystems; Posch et al. 2002) [27]. Section
3.3.3 gives more details about the background of critical loads for acidification. See Downing et al. (1993) and Posch et al. (1995, 1997, 1999 and 2001) for more details about critical loads for
eutrophication.
4.3 Calculation procedure
The total deposition per grid-element (as represented in Figure 4.2) can be compared with the cumulative critical load distribution curve for that grid-element (as in Figure 4.4) to establish the area of
ecosystems for which critical loads are exceeded (unprotected ecosystems or UES in RAINS terminology). Proceeding in this manner grid-element by grid-element, the total area of unprotected ecosystem
over the whole European domain can be calculated. Figure 4.5 represents the results of this exercise spatially resolved over Europe for the emission situation in 1990.
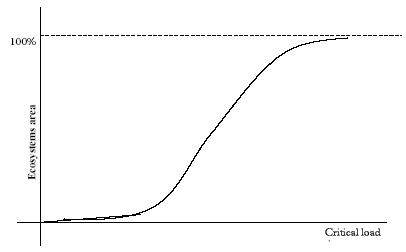
Figure 4.4. Typical cumulative distribution curve of critical load for eutrophication for a grid element
The total area of unprotected ecosystems can be calculated basically for every desired emission situation. This has been used to establish factors that express the area of ecosystems that becomes
unprotected against eutrophication as result of small changes in the reference emission situation for 1990. The mathematical derivation of those eutrophication factors is given in Section 4.4, but the calculation
procedure will be exemplified here.
Figure 4.5 shows the total area of unprotected ecosystem in the whole European domain caused by the European emission level of 1990. This area covered to 76.96 mln ha. We can calculate the area of
unprotected ecosystem for exactly the same emission situation as in 1990, except that the total nitrogen oxide emission of 300 kton by Finland is reduced by 10% to 270 kton. The area of unprotected
ecosystem for this new situation amounts to 76.62 million ha. The difference between the old reference and the new emission situations is 340,000 ha. This means that as a result of the 10% reduction in
emission, the deposition of N will no longer exceed the critical load for 340,000 ha of ecosystem. Figure 4.6 shows where in Europe the reduction in Finnish nitrogen oxide emission leads to a reduction in
the area of unprotected ecosystem. The change in total area of unprotected ecosystem in Europe divided by the change in Finnish emissions gives the characterisation factor representing the change in
unprotected ecosystem area per unit change of nitrogen dioxide emission in Finland (340,000 ha/30 kton = 11,000 ha/kton = 0.11 m2/g).
Click here to see the Figure.
Figure 4.5. Percentage of ecosystem unprotected Figure 4.6. Percentage of ecosystem eutrophication by emissions in becoming protected by reduction 1990 from all European countries of the total nitrogen dioxide emission from Finland by 10%
In the same way, eutrophication factors have been established for nitrogen oxide emissions as well as for ammonia emissions for all European regions. These factors have been calculated for the emission
scenarios for 1990 and 2010. The emission scenarios and the calculated factors for terrestrial eutrophication are shown in Table 4.1 and 4.2.
The factors for terrestrial eutrophication are thus calculated in exactly the same way as the acidification factors in Chapter 3. Section 3.4 describes in more detail the followed calculation procedure including
the mathematical terms.
4.4 Results
The established factors for terrestrial eutrophication are given in Table 4.1 (for 1990) and in Table 4.2 (for 2010), and aggregated to provide "site-generic" factors in Table 4.5. There are many similarities
but also some notable differences to the acidification factors (see Section 3.4 and 3.5).
Similar to the acidification factors, both the 1990 and 2010 factors for terrestrial eutrophication show large differences among regions. The Scandinavian regions and Baltic regions also here have rather high
factors due to their high sensitivity, whereas factors for the western and mid-European regions are usually moderate to low. In contrast to the acidification factors, however, the terrestrial eutrophication
factors are moderate and even high in several cases for many eastern and southern European regions. Whereas the calcareous soils in southern Europe adequately buffer acidification, it has little influence on
the sensitivity of these soils to terrestrial eutrophication.
Table 4.5. The mean, standard deviation, minimum and maximum values of the terrestrial eutrophication factors for nitrogen oxide and ammonia, for different selections from the 44 European regions
(calculated as simple means and standard deviations and weighted for the national emissions). Total Europe covers all 44 European regions. EU+2 consist of Austria, Belgium, Denmark, Finland, France,
Germany (old and new), Greece, Ireland, Italy, Luxembourg, Netherlands, Portugal, Spain, Sweden, Switzerland and United Kingdom. EU consists of the same population minus Norway and Switzerland.
East Europe covers Albania, Belarus, Bosnia-Herzegovina, Bulgaria, Croatia, Czech Republic, Estonia, Hungary, Latvia, Lithuania, Macedonia, Moldavia, Poland, Romania, Russia (four regions), Slovakia,
Slovenia, Ukraine and Yugoslavia.
Click here to see the Figure.
The nitrogen oxide and ammonia factors for terrestrial eutrophication are for most regions considerably higher than the respective factors for acidification. Since both impacts are expressed in the same unit,
this suggests soils to be more sensitive for eutrophication than for acidification from nitrogen deposition. This makes sense, since depositions do not contribute to acidification as long as the nitrogen is
assimilated by the ecosystem. However, this assimilation of nitrogen is precisely the ecosystem growth discussed in Section 4.3.3 that can lead to a shift in species composition if the supply of nitrogen
exceeds the critical load for eutrophication.
Though the nitrogen content of one kg ammonia is 2.7 times higher than for one kg nitrogen oxide, the ratio between the factor for ammonia and for nitrogen oxide is considerably higher for all regions. Due
to the less widespread dispersion of ammonia, reductions in emission obviously results in larger deposition reduction locally, which then leads to a stronger increase in the area of ecosystems that becomes
protected by the emission reduction.
The application of the site-dependent factors will not be discussed here. Instead, the reader is referred to a detailed description and discussion in the Guidance Document (Hauschild and Potting 2003) based
on this Technical Report.
4.5 Discussion
The comments and reflections on the acidification factors in Section 3.4 and 3.5 in Chapter 3 also apply to the terrestrial eutrophication factors discussed here. Chapter 3 is with minor modifications
reproduced from the article of Potting et al. (1998). This article and other work by Potting and colleagues on spatial issues in LCA inspired similar research activity elsewhere, and some of the developments
since 1998 are discussed here.
Krewitt et al. ( 2001) used a different model, the EcoSense model, to calculate the same type of characterisation factors as Potting et al. (1998a,b). However, the effect data for acidification and
eutrophication in EcoSense are of tentative quality and the characterisation factors in Krewitt et al. (2001) for these impact categories were later withdrawn (Krewitt 2003). They are therefore not further
discussed here.
Huijbregts et al. (2000) used the RAINS-model to calculate acidification and terrestrial eutrophication factors, but their definition of the category indicator differs slightly from the one used here and in
Chapter 3 in that they do not cover modelling of threshold or critical load exceedance in terms of the area of unprotected ecosystems. Instead, they quantify deposition – that is, increase of exposure –
divided by the critical load in grid-elements. Two sets of these so-called Hazard Indices are calculated. The first set covers all ecosystems in the model domain and is independent of the emission levels in the
reference situation. The second set covers only those ecosystems that are unprotected or exposed above their critical loads and does depend on the – deposition levels determined by the – emission levels in
the reference situation. The reference emission levels and subsequent deposition levels are used to select the ecosystems to be taken into account (in the "Only Above" indices), but they do not play a role as
such in the calculation of the hazard indices from Huijbregts et al. (2000). The "Only Above" indices are calculated for 1995 emission levels and the estimated 2000 emission levels
The acidification and eutrophication indices or factors of Huijbregts et al. (2000) show considerable, but smaller variation than in the acidification and eutrophication factors in this Technical Report. In a
comparison of the 2010 acidification factors for sulphur dioxide, nitrogen dioxide and ammonia with those presented in Chapter 3, Huijbregts et al. (2000) found a poor correlation for the "Above and
Below" indices (r2 = 0.01 - 0.33), but a moderate correlation for the "Only Above" ones (r2 = 0.41 - 0.67). Potting (2004) pointed out that the variation in factors seems to become larger as the category
indicator is defined closer to the endpoint. This might explain the bad correlation between the factors of Huijbregts et al. and those in this Technical Report. Another reason may be that the estimated emission
levels in Potting et al. differ slightly from those in Huijbregts et al., whereas the latter also used a newer set of Critical Load data. It would be useful to compare both indicators on the basis of factors that are
calculated with the same input data and the same model version.
Goedkoop and Spriensma (1999) in their Eco-indicator 1999 defined their category indicator closer to the endpoint by following a damage approach. They quantified the potentially disappeared fraction of
targeted vascular plant species as a function of the deposition of acidifying and eutrophying emissions. The resulting from-midpoint-to-endpoint-factor combines two impact categories. It is based on the
Nature Planner from the National Institute of Public Health and the Environment (RIVM) which only covers the Dutch territory. The model of Goedkoop and Spriensma therefore does not allow the
calculation of spatially differentiated acidification-eutrophication factors. Since their work, a European database with damage functions has become available (Bakkenes et al. 2002). It would be interesting to
connect this database to the RAINS-model. This would facilitate damage factors that are both site-dependent and are based on a full emission-fate-exposure-effect(-damage) modelling.
Site-dependent characterisation factors for acidification and terrestrial eutrophication have also been calculated for North America with federal states as the source regions (Bare et al. 2003). These factors
represent site-dependent fate factors that express what share of an emission deposits on land.
The calculation of the site-generic factors presented in Table 4.5 need some clarification and discussion,. A site-generic terrestrial eutrophication factor becomes necessary if the geographical location of a
process is unknown, or known only at an aggregated level (for instance located in West or East Europe). In such a case, one would like to assess the eutrophying impact of the process by means of a factor
based on the average of the factors for the regions where the process may be located. However, regions can vary considerably in economic activity. It would therefore be fair to weigh the average for the
total regional activities or total regional emissions related to the process. Also this information may be unknown and the best choice is then to use one of the European averages from Table 4.5.
For different selections of the 44 European regions, the average, standard deviation, minimum and maximum factor for terrestrial eutrophication has been determined. The site-generic factors in Table 4.5
have been calculated as simple means of the national (regional) factors of Table 4.1 and Table 4.2, and as means weighed by the country or region's total emission. The European averages or site-generic
factors in Table 4.5 show, compared to the regional or site-dependent factors on which they are based, a moderate variation in the average factors for clearly different selections of regions. Considering that
the standard deviations have the same size as the means, there is not significant difference between the site-generic factors but it is clear that aggregating at this level means that a large spatially determined
uncertainty is hidden in the factors.
Whether or not to perform a site-dependent impact assessment is still an unsolved point of discussion in life cycle assessment. A considerable group of practitioners would rather avoid the – limited – data
gathering required in addition to present typical life cycle assessment. Even though the only additional required data, the geographical site of emission, is in general already provided by current inventory
analysis. However, the geographical region of a process may sometimes really be unknown. This is the case in life cycle assessments dealing with processes taking place in the – far – future or for products
consisting of materials taken from the spot market (though an average as described above may be appropriate in the latter case). There are also applications where even the effort of limited additional data
gathering might exceed the resources of the practitioner [28]. One of the averages from Table 4.5 (e.g. the one for total Europe) can then be chosen as the "site-generic" characterisation factor. Given the
large ranges and standard deviations in these European averages or site-generic characterisation factors, a sensitivity analysis with the regional or site-dependent characterisation factors is highly
recommended in order to evaluate whether suggested improvement options are indeed significant. The procedure for such a sensitivity analysis is described in detail in the Guidance Document of Hauschild
and Potting (2003).
4.6 Conclusions and recommendations
In the same way as for acidification, characterisation and normalisation factors for terrestrial eutrophication have been established for 44 regions in Europe to facilitate site-dependent LCIA of terrestrial
eutrophication. The eutrophication factors relate the region of emission to the impact on its deposition areas. The conclusions about the acidification factors established in Chapter 3 basically also apply for
the terrestrial eutrophication factors and are not repeated here.
Average factors, standard deviation, minimum and maximum factors for terrestrial eutrophication have been determined for different selections of the 44 European regions. Results show a moderate variation
in the average factors for clearly different selections of regions (West and East Europe), but the variation between rather similar selections of regions is small (EU and EU+2). The exact selection of regions
becomes thus less important as a rough indication for the unknown area where a process is expected to take place. Whereas even a rough indication of the process' location does result in clearly different
averages, the large range and even large standard deviations prevent the differences from being significant.
There are applications where even the effort of limited additional data gathering might exceed the resources of the practitioner. One of the averages from Table 4.5 (f.i. the one for total Europe) can than be
chosen as a sort of "site-generic" characterisation factor. Given the large ranges and standard deviations in these averages, a sensitivity analysis with the regional characterisation factors is highly
recommended in order to evaluate whether suggested improvement options are indeed significant. The procedure for such sensitivity analysis is described in detail in the Guidance Document of Hauschild and
Potting (2003).
4.6.1 Acknowledgement
Research for this work was also supported by a Training and Mobility grant from the European Commission, and by the Danish Agency for Industry and Trade under the EUREKA project LCAGAPS.
4.7 References
Alcamo, J., R. Shaw and L. Hordijk (eds.). The RAINS model of acidification. Science and strategies in Europe. Dordrecht (the Netherlands), Kluwer Academic Publishers, 1990.
Amann, M., I. Bertok, J. Cofala, F. Gyarfas, C. Heyes, Z. Klimont and W. Schöpp. Cost-effective control of acidification and ground-level ozone. Second interim report to European Commission, DG-X1.
Laxenburg (Austria), International Institute for Applied Systems Analysis (IIASA), 1996.
Amann, M., M. Baldi, C. Heyes, Z. Klimont and W. Schöpp. Integrated assessment of emission control scenarios, including the impact of troposheric ozone. Water, air and soil pollution, Vol. 85 (1995),
Issue 4, pp2595-2600.
Bakkenes, M., R. Alkemade, F. Ihle, R. Leemans and J.B. Latour. Assessing effects of forecasting climate change on the diversity and distribution of European higher plants for 2050. Global Change
Biology, Vol. 2002, Issue 8, pp390-407.
Bare J.C., Norris G.A., Pennington D.W., McKone T. TRACI: The US EPA's tool for the reduction and assessment of chemical and other environmental impacts. Journal of Industrial Ecology, Vol. 6
(2003), Issue 3/4, pp49-78.
Barrett, K. and E. Berge (eds.). Transboundary air pollution in Europe. Part 1: Estimated dispersion of acidifying agents and of near surface ozone. EMEP MSC-W status report (research report no.
321996). Oslo (Norway), Norwegian Meteorological Institute, 1996.
Berdowski, J.J.M. and W.J. Jonker. Emissions in the Netherlands. Industrial sectors, regions and individual substances (1992 and estimates for 1993; Publication series emission registration no. 21; in
Dutch). The Hague (the Netherlands), Ministry of Housing, Spatial Planning and Environment, 1994.
Chardon, W.J. The role of the soil in the phosphorous cycling In: Weidema, B.P. and M.J.G. Meusen (eds.) Agricultural data for life cycle assessment. Volume 1 and 2. The Hague (the Netherlands),
Agricultural Economics Research Institute (LEI), 2000,p13-24.
Downing, R.J., J.P. Hettelingh and P.A.M. de Smet (Eds). Calculation and mapping of critical thresholds in Europe. CCE status report 1993. Bilthoven (the Netherlands), Co-ordination Centre for Effects
(CCE) at the National Institute of Public Health and the Environment (RIVM), 1993.
Finnveden, G., Y. Andersson-Sköld, M.-O. Samuelsson, L. Zetterberg and L.-G Lindfors. Classification (impact analysis) in connection with life cycle assessment. In: Product life cycle assessment.
Principles and methodology (Nord 1992:2). Copenhagen (Denmark), Nordic Council of Ministers, 1992, pp172-231.
Finnveden, G. and J. Potting. Eutrophication as an impact category. Int. Journal of Life Cycle Assessment, Vol, 4 (1999), Issue 6, pp311-314.
Goedkoop, M. and R. Spriensma. The Eco-indicator 99. A damage oriented method for life cycle impact assessment. Methodology report and annex. Amersfoort (the Netherlands), Pré consultants, 1999.
Hauschild, M. and J. Potting. Spatial differentiation in life cycle impact assessment – the EDIP2003 methodology. Guideline from the Danish Environmental Protection Agency, Copenhagen, 2003.
Heijungs, R. G. Huppes, R.M. Lankreijer, H.A. Udo de Haes, A. Wegener Sleeswijk, A.M.M. Ansems, P.G. Eggels, R. van Duin and H.P. de Goede. Environmental life cycle assessment of products.
Guide and backgrounds (ISBN 90-5191-064-9). Leiden (the Netherlands), Centre of Environmental Science of Leiden University, 1992.
Huijbregts, M., W. Schöpp, E. Verkuijlen, R. Heijungs and L. Reijnders. Spatially explicit characterisation of acidifying and eutrophying air pollution in life-cycle assessment. Journal of Industrial Ecology,
Vol. 4 (2000), Issue 3, pp125-142.
Krewitt, W. A. Trukenmüller, T. M. Bachmann and T. Heck. Country specific damage factors for air pollution; A step towards site-dependent life cycle assessment. Int. Journal of Life Cycle Assessment,
Vol. 6 (2001), Issue 4, pp199-210.
Krewitt, W. Personal email communication in September 2003 to the SETAC Work Group of Impact Assessment concerning the effect assessment of eutrophication and acidification in EcoSense in relation
to the characterisation factors in Krewitt et al. 2001.
Lindfors, LG., M. Alkemark, C. Oscarsson and C. Spännar. A manual for the calculation of ecoprofiles intended for third party certified environmental product performance declarations. Stockholm,
Swedish Environmental Institute (IVL), 1998.
Lindfors, L-G., K. Christiansen, L. Hoffman, Y. Virtanen, V. Juntilla, O-J. Hanssen, A. Rønning, T. Ekvall and G. Finnveden. Nordic guidelines on life cycle assessment (Nord 1995-20). Copenhagen
(Denmark), Nordic Council of Ministers, 1995
Posch, M, P.A.M. de Smet, J.P. Hettelingh, R.J. Downing (eds.). Calculation and mapping of critical thresholds in Europe. CCE status report 1995. Bilthoven (the Netherlands), Co-ordination Centre for
Effects (CCE) at the National Institute of Public Health and the Environment (RIVM), 1995.
Posch, M., J.P. Hettelingh, R.J. Downing, and P.A.M. de Smet (Eds). Calculation and mapping of critical thresholds in Europe. CCE status report 1997. Bilthoven (the Netherlands), Co-ordination Centre
for Effects (CCE) at the National Institute of Public Health and the Environment (RIVM), 1997.
Posch, M, P.A.M. de Smet, J.P. Hettelingh, R.J. Downing (eds.). Calculation and mapping of critical thresholds in Europe. CCE status report 1999. Bilthoven (the Netherlands), Co-ordination Centre for
Effects (CCE) at the National Institute of Public Health and the Environment (RIVM), 1999.
Posch, M, P.A.M. de Smet, J.P. Hettelingh, R.J. Downing (eds.). Modelling and mapping of critical thresholds in Europe. CCE status report 2001. Bilthoven (the Netherlands), Co-ordination Centre for
Effects (CCE) at the National Institute of Public Health and the Environment (RIVM), 2001.
Potting J. Acidification and terrestrial eutrophication. Comparison of different levels of sophistication. Int J. LCA. Accepted, 2004.
Potting, J. and K. Blok. 1995. Life cycle assessment of four types of floor covering. Journal of Cleaner Production, Vol. 3 (1995), Issue 4, pp201-213.
Potting, J. and K. Blok. Spatial aspects of life cycle impact assessment. In: Udo de Haes, H.A., A.A. Jensen, W. Klöpffer and L-G. Lindfors (eds.). Integrating impact assessment into LCA. Proceedings of
the LCA symposium held at the fourth SETAC-Europe Congress, 11-14 April 1994 at the Free University of Brussels in Belgium. Brussels (Belgium), Society of Environmental Toxicology and Chemistry –
Europe, 1994.
Potting, J., W. Schöpp, K. Blok and M. Hauschild. Comparison of the acidifying impact from emissions with different regional origin in life-cycle assessment. Journal of Hazardous materials, Vol. 1998a,
Issue 61, pp155-162.
Potting, J., W. Schöpp, K. Blok and M. Hauschild. Site-dependent life impact cycle assessment of acidifciation. Journal of Industrial Ecology, Vol. 2 (1998b), Issue 2, pp.63-87.
Wenzel, H., Hauschild M.Z. and Alting, L.: Environmental assessment of products. Vol. 1 - Methodology, tools, techniques and case studies, 544 pp. Chapman & Hall, United Kingdom, ISBN 0 412
80800 5, Kluwer Academic Publishers, Hingham, MA. USA., 1997.
Fodnoter
[21] Institute of Product Development (IPU) in Denmark until 2000, presently at the Center for Energy and Environmental Studies IVEM, University of Groningen
[22] International institute for Applied Systems Analysis (IIASA) in Austria
[23] Institute of Product Development (IPU) in Denmark
[24] Finnveden et al. (1992) do not mention airborne emissions of phosphor.
[25] All together, Finnveden et al. (1992) distinguishes five subcategories: Aggregation of airborne nitrogen emissions (terrestrial eutrophication), aggregation of waterborne phosphor emissions (and organic
material), aggregation of waterborne nitrogen emissions (and organic material), aggregation of nitrogen emissions (and organic material) to water and airborne nitrogen emissions, aggregation of all phosphor
and nitrogen emissions (and organic material).
[26] For a comparison: Emissions of phosphor to air are approximately 130 kton per year, while phosphor emissions to water exceed 5,000 kton per year in the Netherlands (Berdowski and Jonker 1994).
The atmospheric emission is thus less than 3 percent of the waterborne emission, whereas only a minor part of that airborne phosphor will reach surface water through first deposition and next topsoil
erosion.
[27] Each European country determines self which species have to be protected. Some countries might be more protective than others, and seem to have set stricter criteria in the critical load database more
recent than the one used here. The influence by this new set of critical loads on the calculated factors is not clear.
[28] For instance, a product designer should have knowledge about so many things that it may go too far to ask such additional data gathering from him.
| Front page | | Contents | | Previous | | Next | | Top |
Version 1.0 April 2005, © Danish Environmental Protection Agency
|