| Forside | | Indhold | | Forrige | | Næste |
Evaluering af mulige tiltag til reduktion af landbrugets metanemissioner
4 Modelling greenhouse gas emissions from manure handling
Willem A.H. Asman, Danmarks JordbrugsForskning, Afd. for Jordbrugsproduktion og Miljø
4.1 Introduction
Emissions of the greenhouse gases methane (CH4) and nitrous oxide (N2O) from agriculture is closely coupled to the turnover of carbon (C) and nitrogen (N), which also are closely coupled and where
other emissions also are involved (e.g. CO2, N2, NO, NH3, NO3-). It is thRrefore important to describe the emission of these greenhouse gases as a part of the C and N cycle, so that the consequences of
emission reduction measures for one gas can be evaluated also on other environmental emissions.
Berntsen et al. (2003) developed a process oriented whole-farm model of the C and N flows and related emissions. However, the existing version of FASSET does not include all greenhouse gas emissions,
only emissions of N2O from the fields and CH4 emissions from enteric fermentation in cattle are included. However, a less detailed model of greenhouse gas emissions from cattle farms (FarmGHG) has
been developed by Olesen et al. (2005). The work presented here uses existing knowledge to develop models for FASSET for the emissions of CH4 and N2O from slurry and solid manure.
The emissions of CH4 and N2O from manure storages are the result of physiochemical and microbiological processes and are influenced by agricultural practice, such as manure handling system, housing
design, cleaning habits etc. Physicochemical processes can be described relatively easily, whereas microbiological processes are much more complicated. The complexity of such processes makes it more
difficult to generalize experimental results, e.g. different emissions can be observed at apparently the same conditions. In that case factors that are important for the process have apparently been overlooked.
The best way to model the emissions is to include all the processes and the interaction between the emission processes for all gases (including CO2 and N2) and to include all compounds, so that a mass
balance approach can be followed. This is the approach that as far as possible is used here.
4.2 Emission models
4.2.1 Methane emissions from slurry
Based on studies of Sommer et al. (2004) and Olesen et al. (2005) the following descriptive model for methane emissions from slurry based systems is proposed:

where factor NH3 is factor by which the CH4 emission rate is reduced due to the concentration of high free NH3 molecules. This factor will be a function of the free NH3 concentration, but the precise
description of this function cannot yet be given. It cannot be excluded that this factor is different for pig and cattle slurry. factoramount is a factor by which the CH4 emission rate is increased due to the fact
that it increases with the amount present in the tank. The precise description of this function cannot yet be given. It is likely that it will be different for pig and cattle slurry. VSd is the concentration of easily
degradable volatile solids (kg kg-1). VSnd is the concentration of slowly degradable volatile solids (kg kg-1). b1 is a rate correction factor for VSd (can be different for pig and cattle slurry). b2 is a rate
correction factor for VSnd (can be different for pig and cattle slurry). A is an Arrhenius parameter (can be different for storage in-house and storage outside) (g CH4 kg-1 VS h-1). E is the heat of formation
(J mol-1) (can be different for pig and cattle slurry). R is the gas constant (8.314 J K-1 mol-1). T is the temperature (K).
Different authors give different values for the heat of formation E, depending on the type of slurry (cattle or pig), season or presence of crusts (Table 4.1). The emissions in all experiments increase with
temperature, but to different extents. There are a few possible explanations for the differences in temperature responses. It could partly result from the fact that there are different CH4 forming bacteria that
have their own temperature optimum. Another explanation is that ebullition of CH4 may have occurred in summertime, which reduces the transport time through the crust, where CH4 oxidation takes place.
According to De Mol and Hilhorst (2003) there is no CH4 emission below 4C. Amon et al. (2003) found that during the summer measurements the accumulated CH4 emission reaches a plateau, whereas
this is not observed during the winter measurements. A possible reason for this could be that there is not enough VSd left at the end of the summer experiments.
Table 4.1. Heat of formation for CH4 emission used by different authors.
Reference |
ΔE (J mol-1) |
Comments |
Husted (1994) |
-85x103 (4-18°C) |
Cattle slurry, uncertainty of same order as E. Storage is outside. Not clear if data are for slurry with or without a crust |
Husted (1994) |
-140x103 (4-18°C) |
Pig slurry, uncertainty of same order as E. Storage is outside. Not clear if data are for slurry with or without a crust |
Sommer et al. (2000) |
-230x103 (summer) -80x103 (winter) |
Cattle slurry with crust or other cover. Storage outside |
Amon et al. (2003) |
-122x103 (summer + winter) -53 to -85 (winter) – 135 to –245 (summer) |
Cattle slurry (with crust or cover), storage outside |
Béline (2003) |
-44x103 (8-18°C) -38x103 (8-18°C) |
Cattle slurry, Farm1, storage outside Cattle slurry, Farm2, storage outside, crust |
Hüther (1999) |
-91x103 (10-25°C) -432x103 (5-10°C) |
Pig slurry, storage in the laboratory, no crust Pig slurry, outdoor conditions, no crust |
The CH4 emission rate is a function of the pH. At pH 7 the emission shows is maximum and at a pH of 6.5 or 8.3 it is about half this value (De Mol and Hilhorst, 2004). It should be noted that changes in
TAN (total ammoniacal nitrogen = NH3 + NH4+) in general also will affect the pH, i.e. the TAN concentration and the pH are not independent variables.
Hüther (1999) investigated the influence of the NH3/NH4+ concentration on methane and nitrous oxide emissions. Investigations with just one microorganism species have shown that the undissociated
forms NH3 and HNO2 are much more inhibiting than the ionic forms. NH3 in high concentrations slows down the activity of methane bacteria and both nitrosomonas and nitrobacter that are involved in the
nitrification. The NH4-N concentrations in cattle and pig slurry depend on age, water content, animal species and feeding and lies between 1.5 and 5.5 g kg-1. As the NH3 concentration in aqueous solution
is highly dependent on the pH, it could in principle occur that the NH3 concentration becomes so high that CH4 and N2O emissions are influenced. The experiments have also shown that NH4+
concentrations that occur in practise can slow down the activity of methane bacteria. So higher NH4+ concentrations will lead to lower CH4 emission during normal storage periods of 3-6 months.
NH4-N concentrations in pig and cattle slurry in Denmark vary between 3 and 4 g kg-1 (Poulsen et al., 2001), i.e. in the range where an effect on the CH4 emission can be expected, but it will also depend
on the pH. There is only limited data available on slurry pH that have been made simultaneously with the NH4-N concentration measurements in Denmark. However, the observed ranges indicate that the
CH4 emission in Denmark can be reduced by NH3.
Angelidaki and Ahring (1993a) investigated the effect of inhibition of CH4 emission by anaerobic digestion of livestock waste by ammonia. They state also that free NH3 (i.e. not in the form of NH4+)
inhibits the CH4 emission, but that a the inoculum can adapt to higher NH3 concentrations if the concentration is raised gradually. Angelidaki et al. (1993b) made a mathematical model for anaerobic
digestion of manure. Their equations show that the formation of VFA (volatile fatty acids) is not influenced by free NH3, only the last step: formation of CH4 from acetic acid seems to be influenced by the
free NH3 concentration. Inhibition by free NH3 leads to higher VFA concentrations.
CH4 emission of slurry covered by a natural crust is usually lower than the emission without a crust (e.g. Husted, 1994). The reason for this could be oxidation of CH4 in the crust. Amon et al. (2003) found
that the emission of cattle slurry with a cover was about 5% less than the emission without a cover (but with a crust). Hüther (1999) found that there was not much difference between CH4 emission from
untreated cattle slurry with and without a Leca cover.
Hüther (1999) mentions that the CH4 emission per tonne slurry decreases if the ratio surface to volume becomes larger.
4.2.2 Methane emissions from solid manure
Solid manure is essentially slurry to which straw is added. Degradation of straw can also lead to CH4 emission. Farmyard manure has a varying straw content. Information from Germany shows that the dry
matter content of farmyard manure varies from 12 to 30% with an average of 22% (Hüther, 1999). The dry matter content of deep litter is larger than 22%. The difference between farmyard manure and
composting farmyard manure is that a farmyard manure heap is anaerobic, while the composting farmyard manure is aerobic because it is turned regularly. Due to processes in the manure or composting
manure, heat is generated. During composting higher temperatures are reached than without composting. The temperature of the heap does, however, also depend on the outdoor temperature. This means
that the temperature is higher during in the summer than in the winter. The temperature is not constant, but varies with time, which means that the influence of the ambient temperature on the processes also
will vary with time.
Solid manure with a low straw content is wet and is not porous. Farmyard manure with a high straw content is humid or dry and is very porous. In the manure and in its aggregates oxygen gradients will exist
that are a function of position and time. Aerobic processes occur at the surface, whereas anaerobic processes occur deeper in the manure and in its aggregates. For these reasons it can be expected that the
CH4 emission from farmyard manure is a function of the straw content. For composting manure the CH4 emission decreases with the dry matter content (Hüther, 1999). Heaps with manure are usually
stored outside the farm building. As a result they are also exposed to rain and snow, which may reduce oxygen penetration into the heap.
There is only a set of two experiments with cattle farmyard manure of which data can be used for a parameterization (Amon, 1998; Amon et al., 2001). The dry matter content of this farmyard manure was
20-21%.
One experiment was conducted in summertime during about 80 days (average ambient temperature 15.4 C). It takes a few days before the emission rate is at a higher level. This is apparently due to the fact
that the microbial activity needs some time to start. A statistical analysis shows that the CH4 emission rate was more related to the temperature in the heap than to the time after the start of the experiment.
After the initial maximum in the emission that is reached after a few days the CH4 emission rate decreases about exponentially with time. At 80 days the emission rate is still about 15% of the maximum
emission rate. This means that the duration of the experiment was too short to measure the maximum accumulated emission over time.
One experiment was conducted in wintertime during about 80 days (average ambient temperature 7.7 °C). This experiment was apparently influenced by water originating from melting snow. The shape of the
emission rate as a function of time looks similar to the shape for the summertime experiment. In this case it took a longer time to reach the maximum emission rate. At the end of the experiment the ambient
temperature increased and as a consequence the emission rate increased too. The duration of this experiment was apparently also too short to measure the maximum accumulated emission over time.
The function for the CH4 emission rate was derived from the experiments performed by Amon (1998). Due to the fact that the winter experiment is influenced by precipitation it is more difficult to reach a
conclusion.
The CH4 emission rate as a function of time is somewhat arbitrarily given by:
E = E0*exp(-a*d) (g CH4/(t.d))
where E0= facttemp*Eref with Eref = 13 g CH4/(t.d) (where t = tonne manure, d = day); a= 0.03466 d-1.
The temperature factor facttemp was estimated from the Arrhenius equation:

where E is the actual emission rate (g CH4 /(t.d)), Rref is the emission rate at reference temperature (same units as E), E is the enthalpy of formation (J mol-1); in this case –9.0104 J mol-1 is taken, and R is
the gas constant 8.314 J mol-1 K-1, T is the actual temperature (K), and Tref is the reference temperature (K, 5C = 278.15 K).
It should be noted here that the above function is just a function that approximately fits the few measurements of Amon (1998), but that its use in fact is limited to those measurements as some of the following
conditions will be different in the Danish situation:
- Dry matter content/content of easily degradable VS.
- Temperature.
- Air flow (influences the transport of oxygen into the manure).
4.2.3 Nitrous oxide emissions from slurry tanks
N2O can be formed as a by-product during the nitrification (from NH3 to NO3-) if not enough oxygen is present. N2O can also be formed as a step in the denitrification (from NO3- to N2). N2O is
generated at the boundaries between aerobic and anaerobic environments and is a result of a competition between various processes. This makes it much more difficult to model N2O emission than CH4
emission.
Sommer et al. (2000) measured the emission of CH4 and N2O (August-October 1996; June-September 1997) of stored cattle slurry and fermented slurry in buried containers outside. The experiments
lasted between 9 and 12 weeks. The cattle slurry was between 2 and 4 months old. The fermented slurry contained 2-4 weeks old slurry from dairy farms and 20% organic waste, mainly from food
processing industries. The experiment included different covers (the containers were open to rainfall):
- Uncovered (no crust developed on the fermented slurry; the crust on the cattle slurry was removed)
- Surface crust (crust from another cattle slurry store was added to the fermented slurry; 7-10 cm crust on cattle slurry developed naturally)
- Leca pebbles (10 cm thick)
- Straw (15 cm thick chopped wheat straw)
Sommer et al. (2000) found that no N2O was emitted from any treatment (fermented or normal cattle slurry; covers) during the fall experiments of 1996. The covers visually appeared saturated with water
during this period, which was characterized by a positive water balance.
Sommer et al. (2000) found that in the summer experiments significant N2O emissions of up to 25 mg N m-2 h-1 were encountered, but only in slurry stores with surface covers. This suggests that N2O
production took place at the interface between the anaerobic slurry and the air-filled surface covers. N2O emission from cattle slurry was highest when covered by a natural crust and appeared earlier than
with covers of Leca or straw. The high emission rate is associated with the drying out of the surface layers in periods with more evaporation of water than precipitation. Drying will create oxic zones in the
surface cover and will enhance convection of liquid upward through the cover, where dissolved NH4+ can be oxidized by nitrifying bacteria in the oxic zones, while in anoxic zones the products of
nitrification can be denitrified. As the N2O is formed in the surface crust, it is likely to be function of the surface area, rather than the volume (emission m-2 crust).
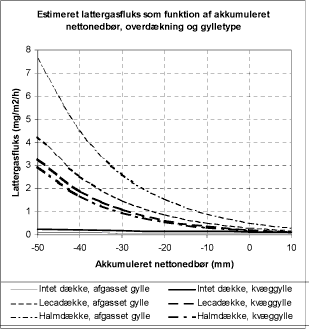
Figure 4.1. Modelled N2O flux as a function of the net precipitation amount and the type of cover as derived by Sommer et al. (2000) from their measurements.
Sommer et al. (2000) performed a statistical analysis and derived average N2O fluxes from their measurements (Figure 4.1). The emissions show variations in time. Almost no emission occurred for the
situation without a crust. For the situation with a surface crust the emission rate was also often almost zero, but a maximum up to 22 mg N m-2 h-1 was observed.
The following equation describes the statistical relation:

where E is the emission rate, i is an index for cover: 1 = no cover, 2 = floating Leca pebbles, 3 = straw, j is an index for manure type: 1 = fermented slurry, 2 = cattle slurry, is mean ln (natural logarithm)
emission, Ci is additional ln emission due to cover, Sj is additional ln emission due to slurry type, CSi,j is additional ln emission due to the interaction between cover i and slurry type j, k is mean slope of the
linear relationship between the ln emission and the water balance, w is water balance (precipitation – evaporation), ci is additional contribution to the slope of the linear relationship between the ln emission
and the water balance for cover i, sj is additional contribution to the slope of the linear relationship between the ln emission and the water balance for slurry type j, i,j,h is the residual for cover i, slurry type j
and time of measurement h. The residual is here set to 0.
It should be noted that due to an error, the term sjw in this equation was left out in the article (Henning T. Søgaard, personal communication). The values of the parameters are also not published in the
paper, but were also obtained from Henning T. Søgaard: µ = -8.6071286, C1= -0.8225919, C2 = 0.31584853, C3 = 0.50674336, S1 = 0.1140391, S2 = -0.1140391, k = -0.0404785, CS1,1 =
-0.54147, CS1,2 = 0.54147, CS2,1 = 0.0971813, CS2,2 = -0.0971813, CS3,1= 0.4442888, CS3,2 = -0.4442888, c1 = 0.02803831, C2 = -0.0135708, C3 = -0.0144675, s1 = 0.00170533, s2 =
-0.00170533.
It was not possible to find information on measured N2O emissions from slurry, where all factors that potentially could influence the emission were investigated, but the approach of Sommer et al. (2000)
could be used as a start. It should be noted, however, that this parameterization is presumably rather specific for this experiment.
4.2.4 Nitrous oxide emissions from solid manure
The following parameterisation of the emission rate E was found for solid manure stored in a heap outside (Amon, 1998):
E = 1.97*exp(-0.3466e-1*(day-8)) (g N2O/(t.d)
The emission before day 8 has in this way be set to 0.
The parameterisations of the results of Amon (1998) are too specific for that particular situation and cannot be used in general. Information is also lacking on important factors that influence the emission rate.
4.3 Scenario analyses
The CH4 emission depends on the amount of slurry stored and is also a function of the temperature. To get an impression how the CH4 emission changes with agricultural practise two simple scenarios were
adopted, where e.g. no slurry was applied in the summer.
- Scenario “May 1” it is adopted that there is no slurry in the tank on May 1 and all the slurry present on April 1 is applied in April (Table 4.2).
- Scenario “April 1” it is adopted that there is no slurry in the tank on April 1 and all the slurry present on March 1 is applied in March (Table 4.2).
In both cases it is assumed that the amount produced in September and October is applied in those months. These scenarios are about in agreement with the results of an inquiry that addresses the amount of
slurry applied in different seasons (Dansk Landbrug, 2004).
Table 4.2. Average amount of slurry stored for each month for different scenarios, expressed in the amount produced in one month.
Month |
Amount stored Scenario May 1 |
Amount stored Scenario April 1 |
Amount stored Scenario 1990 |
Amount stored Scenario 2003 |
January |
6.50 |
7.50 |
3.00 |
6.60 |
February |
7.50 |
8.50 |
4.20 |
7.68 |
March |
8.50 |
4.50 |
4.08 |
7.68 |
April |
4.50 |
0.50 |
1.44 |
4.56 |
May |
0.50 |
1.50 |
0.84 |
0.96 |
June |
1.50 |
2.50 |
1.44 |
1.68 |
July |
2.50 |
3.50 |
1.92 |
2.16 |
August |
3.50 |
4.50 |
2.16 |
2.52 |
September |
4.00 |
5.00 |
1.44 |
2.52 |
October |
4.00 |
5.00 |
0.60 |
3.36 |
November |
4.50 |
5.50 |
0.72 |
4.44 |
December |
5.50 |
5.50 |
1.92 |
5.52 |
From Sommer et al. (2004) it can be seen that almost only the easily degradable VS content plays a role in the emission of CH4. To simplify the calculations in this sensitivity study it was thRrefore assumed
that the increase and decrease of the CH4 emission rate as a function of the temperature can be described by only the taking the emission from the easily degradable VS into account. Average monthly
temperatures for Foulum were used in the calculations. It was implicitly assumed that fraction of VSd that was converted to CH4 was the same at the same time of the year for different values for the heat of
formation. The effect of this is discussed wherever it is relevant.
To simplify the calculations further the ratio emission rate at the actual temperature/emission rate at the annually averaged temperature at Foulum (facttemp) was calculated for each month. The (relative)
emission rate as compared to the annually averaged temperature at Foulum was described by:

where E is actual emission rate at temperature T (g CH4-C g-1 VS d-1), Rref is the emission rate at reference temperature (same units as E), ΔE is the enthalpy of formation (J mol-1), R is the gas constant
8.314 J mol-1 K-1, T is the actual temperature (K), and Tref is the annually averaged temperature at Foulum (7.3°C = 280.4 K).
The relative emission was multiplied by the amount of slurry stored for each month. At last these results were summed up. In that way a relative annual emission was obtained for one scenario that can be
compared with the relative annual emission of another scenario.
As there is uncertainty on the enthalpy of formation, the calculations were made with the value that Sommer et al. (2004) took (-1.127x105 J mol-1) to obtain the same result as the IPCC methodology for
the present storage practise. The calculations were also made with values that represent more extreme cases found in the literature: -0.5x105 J mol-1 and 2x105 J mol-1.
Table 4.3 illustrates that the uncertainty in the CH4 emission from slurry due to only the uncertainty in the amount stored will be of the order of 10-30%. The emission in the “April 1” scenario is larger than
that of the “May 1” scenario because in general more slurry is stored.
Table 4.3. Ratio annual emission of scenario “April 1”/annual emission of scenario “May 1” for different enthalpies of formation for CH4.
Enthaply of formation (J mol-1) |
Emission ratio “April 1”/”May 1” |
-1.127x105 |
1.23 |
-0.5x105 |
1.11 |
-2.0x105 |
1.32 |
Notes: The same enthalpy is taken in both scenario's.
Table 4.4 illustrates that the uncertainty in the enthalpy of formation for CH4 can lead to uncertainties in the annual CH4 emission of 15-90%. It should be noted, however, that in these calculations it is not
taken into account that the amount of easily degradable VS remaining varies with the enthalpy of formation of CH4. This effect would lead to smaller changes in emission than calculated here.
Table 4.4. Ratio annual emission calculated with different enthalpies/ratio emission for an enthalpy of –1.127105 J mol-1.
Enthaply of formation (J mol-1) |
Ratio emission/emission for ΔE = –1.127x105 J mol-1 |
-1.127x105 |
1.00 |
-0.5x105 |
0.85 |
-2.0x105 |
1.89 |
A policy to reduce NH3 emissions was adopted in the nineties, where the period during which slurry was applied was reduced and the capacity to store slurry was increased. Steen Gyldenkærne (National
Environmental Research Institute, Roskilde, Denmark) has performed a recalculation on the amounts stored for each month for the situation in 1990 when no measures were taken and for 2003 when the
policy was established (Table 4.2) based on data obtained from the Danish Agricultural Advisory Centre.
The results of the calculations for those scenario's are presented in Table 4.5. The CH4 emission increased by 30-90% during this period, which is mainly due to the increased amount of slurry stored.
Table 4.5. Ratio emission 2003/emission 1990 for the same amount of slurry produced.
Enthaply of formation (J mol-1) |
Ratio emission 2003/emission 1990 |
-1.127x105 |
1.58 |
-0.5x105 |
1.88 |
-2.0x105 |
1.33 |
The results of climate models for Denmark indicate that the average annual temperature in 2100 could be 3-5 °C higher than in 1990 (Olesen et al., 2004). The warming is largest during the night and there is
only small difference between the rise in temperature during summer and winter. To get an impression of the possible effect of the rise in temperature on the emission of CH4 from storage an increase in
temperature of 4°C was assumed for every month. For the “May 1” scenario (see previous section) this leads to an increase in the CH4 emission of about 90% (for an enthalpy formation of -1.127x105 J
mol-1), 35% (for an enthalpy formation of –0.5x105 J mol-1) or 220% (for an enthalpy formation of 2.0x105 J mol-1). It should be noted, however, that these calculations do not taken into account that the
amount of easily degradable VS is partly consumed and that a reduced amount of easily degradable VS will lead to reduced emissions and a larger amount of easily degradable VS would lead to larger
emissions. If this effect would be taken into account this would lead to smaller changes in emission than calculated here.
4.4 Conclusions
There is in general knowledge on which factors potentially influence the emission of CH4, N2O and NH3 from manure storages. However, there is almost no experimental investigation where factors that
influence the emission of greenhouse gases as a function of time have been investigated systematically. For that reason there is not yet possible to describe the emission of CH4 and N2O in a satisfactory way
with the FASSET model, although a preliminary approach is proposed for the emission of CH4 and N2O from slurry.
The emissions of CH4, N2O and NH3 are not independent, e.g. high concentrations of NH3 in the slurry may lead to smaller emissions of CH4 and N2O. Having a crust on slurry may reduce the emission
of CH4 and NH3, but could potentially lead to an increase in the N2O emission. Any research of the possibilities to reduce the emission of one agricultural gas, should also include the effects on the
emissions of the other agricultural gases. Other factors should also been taken into account, such as animal welfare, CO2 emissions (to make it possible to make a complete C balance in a model) and N2
emissions (to make it possible to make a complete N balance in a model), emission of odour and energy consumption. If such and integrated approach is not done, there is a considerable risk that a political
focus on one of these problems will lead to and increase of the other problems.
A model like FASSET that is based on C and N balances and should be able to predict emissions of CH4, N2O and NH3 can only be based on the results of experiments where factors that influence the
emission are varied systematically. However, attempts at such experiments have almost never been done, and there is thus a need for a comprehensive research in this area where experiments are linked to a
mechanistic modelling of the processes.
4.5 References
Amon, B. (1998) NH3-, N2O- und CH4-Emissionen aus der Festmistanbindehaltung für Milchvieh – Stall – Lagerung – Ausbringung. Dissertation. Forschungsbericht Agrartechnik 331. Universität für
Bodenkultur, Institut für Land-, Umwelt und Energietechnik, Wien, pp. 182.
Amon, B., Amon, T., Boxberger, J., Alt, C. (2001) Emissions of NH3, N2O and CH4 from dairy cows housed in a farmyard manure tying stall (housing, manure storage, manure spreading). Nutr. Cycl.
Agroecosyst. 60, 103-113.
Amon, B., Kryvoruchko, V., Amon, T. (2003) Quantifying the effect of different slurry storage conditions on GHG emissions. Appendix 1 in: Amon, B., Kryvoruchko, V., Amon, T., Béline, F., Petersen,
S.O. Quantitative effects of storage conditions on GHG emissions from cattle slurry, and N2O and CH4 turnover inside natural surface crusts. Deliverable No. 5.5 , MIDIAR project.
Angelikdai, I., Ahring, B.K. (1993a) Thermophilic anaerobic digestion of livestock waste: the effect of ammonia. Appl. Microbiol. Biotechnol. 38, 560-564.
Angelidaki, I., Ellegaard, L., Ahring, B.K. (1993b) A mathematical model for dynamic simulation of anaerobic digestion of complex substrates: focusing on ammonia inhibition. Biotechnol. Bioeng. 42,
159-166.
Béline, F. (2003) Monitoring GHG from manure stores on organic and conventional dairy farms. Report MIDAIR, Cemagref, Rennes, France.
Berntsen J., Petersen B.M., Jacobsen B.H., Olesen J.E. & Hutchings N.J. (2003). Evaluating nitrogen taxation scenarios using the dynamic whole farm simulation model FASSET. Agricultural Systems 76,
817-839.
Dansk Landbrug (2004) Statistisk analyse af GfK data. Dansk Landbrug, København.
De Mol, R.M. & Hilhorst, M.A. (2004) Emissiereductieopties voor methaan uit mestopslagen (Emission reductions for methane from storage facilities, in Dutch). Report 165, Agrotechnology & Food
Innovations, Wageningen, The Netherlands.
Husted, S. (1994). Seasonal variation in methane emission from stored slurry and solid manures. J. Environ. Qual. 23, 585-592.
Hüther, L. (1999). Entwicklung analytischer Methoden und Untersuchung von Einflussfaktoren auf Ammoniak-. Methan- und Distickstoffmonoxidemissionen aus Flüssog- und Festmist. Landbauforschung
Völkenrode, Sonderheft 200, FAL, Braunschweig, Germany.
Olesen, J.E., Gyldenkærne, S., Petersen, S.O., Mikkelsen, M.H., Jacobsen, B.H., Vesterdal, L., Jørgensen, A.M.K., Christensen, B.T., Abildtrup, J., Heidmann, T., Rubæk, G. (2004) Jordbrug og
klimaændringer. DJF rapport Markbrug nr. 109, Danish Institute of Agricultural Sciences, Tjele, Denmark.
Olesen, J.E., Schelde, K., Weiske, A., Weisbjerg, M.R., Asman, W.A.H. & Djurhuus, J. (2005). Modelling greenhouse gas emissions from European conventional and organic dairy farms. Agriculture,
Ecosystems and Environment (in press).
Poulsen, H.D., Børsting, C.F., Rom, H.B., Sommer, S.G. (2001) Kvælstof, forsfor og kalium i husdyrgødning – normtal 2000. Rapport 36, Husdyrbrug. Danmarks JordbrugsForskning, Foulum.
Sommer, S.G., Petersen, S.O. & Søgaard, H.T. (2000) Greenhouse gas emission from stored livestock slurry. J. Environ. Qual. 29, 744-751.
Sommer, S.G., Petersen, S.O. & Møller, H.B. (2004) Algorithms for calculating methane and nitrous oxide emissions from manure management. Nutr. Cycl. Agroecosyst. 60, 133-145.
| Forside | | Indhold | | Forrige | | Næste | | Top |
Version 1.0 Juli 2005, © Miljøstyrelsen.
|