| Front page | | Contents | | Previous | | Next |
Dry deposition and spray drift of pesticides to nearby water bodies
5 Discussion and conclusions
5.1 General comparison dry deposition and spray drift
5.2 Comparison for pesticides that are used in Denmark
In this section the (potential) contribution from dry deposition to water bodies will be compared with the contribution from spray drift.
5.1 General comparison dry deposition and spray drift
Although spray drift to some extent depends on the properties of the additives the 95% percentile from Ganzelmeier et al. (1995) is here used for all pesticides in the comparison with the modelled dry deposition (see Table 6). The dry deposition of pesticides depends on many factors: the physico-chemical properties of the pesticides, the meteorological conditions and the properties of the water body. The following situation was chosen for all dry deposition calculations presented here:
- The wind blows over the field onto which pesticide is applied in the direction of the water body.
- The field with emission is 100 m long in the upwind direction. It should be noted here, that as spray drift is transported over short distances (30 m), only the application of pesticide in a small upwind zone contributes to the pesticide load of a water body. For transport of vapour leading to dry deposition the situation is different. It is transported over long distances and for that reason the contribution of dry deposition will to a great extent depend on the length of the field in the upwind direction. The relative contribution from the nearby part of the field to the dry deposition will, however, be larger than the contribution of an area of the same size that is further upwind. The upwind length of the field influences also how fast the dry deposition to the water body decreases as a function of distance to the field.
- In this case there is no non-spray zone.
- The surface roughness length z0m is 0.1 m (crops).
- The friction velocity u* is 0.386 m s-1 which is representative of Danish conditions.
- The calculations are made for neutral atmospheric conditions.
- The concentration of the pesticide in the water body is assumed to be 0 (i.e. or so low that the concentration will not have any influence on the flux.
- It is assumed that the mass accommodation coefficient of the pesticide is so large that it does not influence the uptake by water bodies (see Appendix C).
- It is assumed that the pesticide does not react in water.
The calculations have been made for 3 different types of water bodies:
- A stream, which has a typical minimum value of rc for Danish conditions (K2d 100 day-1 and a depth of 1.37 m).
- A stream, which has a maximum value of rc for Danish conditions (K2d 1 day1 and a depth of 1.37 m).
- A lake (wind speed is 5 m s-1).
For all these types of water bodies calculations have been made for 6 different values of Henry’s law coefficients KH and a molecular mass of 300 g mol-1, which is representative for pesticides.
The calculations have been made for a situation where 100% of the amount of pesticide volatilises. The results are presented in Figs. 32-34. The scales in all figures are the same so that a comparison is easily possible.
If, however, only a fraction f of the pesticide volatilises the values for the dry deposition in the figures have to be multiplied by this fraction. This means that the dry deposition in that case will be reduced. This is done to make a generalisation possible. It should be noted that the spray drift is not affected by the volatilisation.
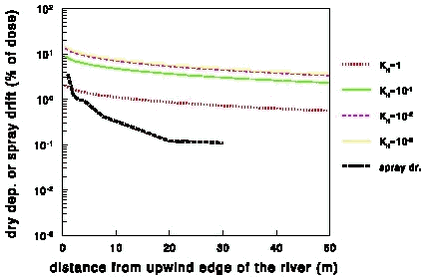
Figure 32. Dry deposition of pesticides to a stream with a low rc for different Henry’s law coefficients and spray drift as a function of the distance to the upwind edge of the stream. Only results for KH values of 100 to 10-3 are shown. In the calculations it is assumed that 100% of the pesticide volatilises. If only a fraction f of the applied pesticide volatilises the dry deposition in the figure has to be multiplied by a factor f. Figur 32. Tørdeposition af pesticider til et vandløb med en lav rc-værdi for forskellige Henry’s lov konstanter samt afdrift som funktion af afstanden til opstrøms kanten af vandløbet. Kun resultater for KH værdier fra 100 til 10-3 er vist. I beregningerne er det antaget, at 100% af pesticidet fordamper. Hvis kun en fraktion f af pesticidet fordamper skal den i figuren viste tørdeposition multipliceres med en faktor f.
Figs. 32-34 show that dry deposition to water bodies potentially can be more important than spray drift, especially for streams. Whether it is more important depends e.g. on the fraction of the pesticides that volatilises and the Henry’s law coefficient. It can be seen that the results are the same for compounds with a Henry’s law coefficient of 10-4 and lower. In that case the deposition flux is limited by the laminar boundary layer resistance only and does not any longer depend on the properties of the water body. Figs. 32-34 show clearly that the relative decrease in the spray drift as a function of distance from the emission field is much larger than for dry deposition.
Although the dry deposition model has been based on the description of many processes that have been measured, most of these processes have only been studied for larger water bodies and not for small streams or ponds. For that reason there is a need for experimental studies to determine the dry deposition of pesticides to small water bodies.
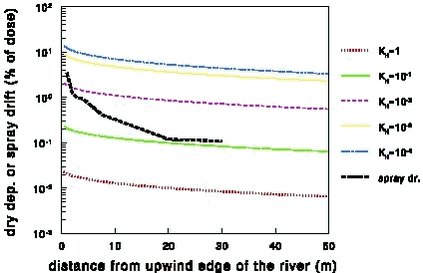
Figure 33. Dry deposition of pesticides to a stream with a high rc for different Henry’s law coefficients and spray drift as a function of the distance to the upwind edge of the stream. Only results for KH values of 100 to 10-4 are shown. In the calculations it is assumed that 100% of the pesticide volatilises. If only a fraction f of the applied pesticide volatilises the dry deposition in the figure has to be multiplied by a factor f. Figur 33. Tørdeposition af pesticider til et vandløb med en høj rc-værdi for forskellige Henry’s lov konstanter samt afdrift som funktion af afstanden til opstrøms kanten af vandløbet. Kun resultater for KH værdier fra 100 til 10-4 er vist. I beregningerne er det antaget, at 100% af pesticidet fordamper. Hvis kun en fraktion f af pesticidet fordamper skal den i figuren viste tørdeposition multipliceres med en faktor f.
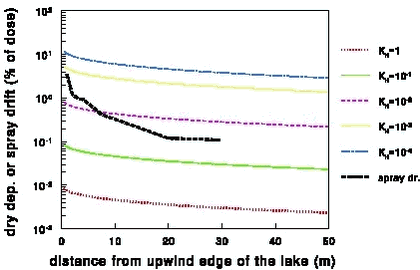
Figure 34. Dry deposition of pesticides to a lake for a wind speed of 5 m s-1 at 10 m height for different Henry’s law coefficients and spray drift as a function of the distance to the upwind edge of the lake. Only results for KH values of 100 to 10-4 are shown. In the calculations it is assumed that 100% of the pesticides volatilises. If only a fraction f volatilises the dry deposition in the figure has to be multiplied by a factor f Figur 34. Tørdeposition af pesticider til en sø ved en vindhastighed på 5 m s-1 i 10 m højde for forskellige Henry’s lov konstanter samt afdrift som funktion af afstanden til opstrøms kanten af søen. Kun resultater for KH værdier fra 100 til 10-4 er vist. I beregningerne er det antaget, at 100% af pesticidet fordamper. Hvis kun en fraktion f af pesticidet fordamper skal den i figuren viste tørdeposition multipliceres med en faktor f..
5.2 Comparison for pesticides that are used in Denmark
For pesticides for which the dry deposition is limited by the laminar boundary resistance, the accumulated dry deposition flux is a direct function of the accumulated emission flux. As it is difficult to show the dry deposition flux for all compounds that were sold in large quantities in Denmark in the year 2000 a selection of compounds was made. Compounds were chosen for which the percentage of the dose that volatilises (Table 3) decreases with a factor of about two for each compound. The calculations were made for a stream, which has a typical minimum value of rc for Danish conditions (K2d 100 day-1 and a depth of 1.37 m).
Fig. 35 shows the results for these compounds. It should be noted that these curves look exactly the same for other water bodies for all compounds apart from fenpropimorph. For fenpropimorph the dry deposition flux would maybe be 40% lower for ditches or ponds. For pendimethalin for which no results are shown this difference would be larger: it could be up to a factor of 5 lower for ditches and ponds. Fig. 35 shows that the accumulated dry deposition flux for many important compounds is larger or of the same order of magnitude as the spray drift flux. The dry deposition flux becomes relatively more important with the distance to the emission field.
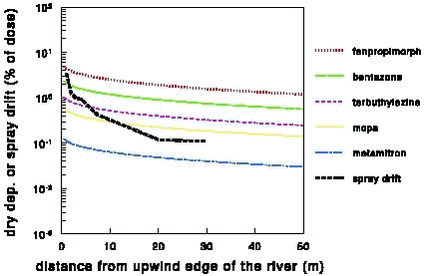
Figure 35. Dry deposition of pesticides to a stream with a low rc for different Henry’s law coefficients and spray drift as a function of the distance to the upwind edge of the stream. Compounds: fenpropimorph (36%), bentazone (17%), terbuthylazine (8%), mcpa (4%), metamitron (1%). The numbers in brackets indicate the percentage of the dose that volatilises. In the calculations it is assumed that the pesticide does not dissociate in water. Figur 35. Tørdeposition af pesticider til et vandløb med en lav rc-værdi for forskellige Henry’s lov konstanter samt afdrift som funktion af afstanden til opstrømskanten af et vandløb. Stoffer: fenpropimorph (36%), bentazon (17%), terbuthylazine (8%), mcpa (4%), metamitron (1%).Tallene i parentes angiver procentdelen af dosen, som fordamper. Ved beregningerne er det antaget, at pesticiderne ikke dissocierer i vand.
| Front page | | Contents | | Previous | | Next | | Top |
|