| Front page | | Contents | | Previous | | Next |
The Influence of Sorption on the Degradation of Pesticides and other Chemicals in Soil
7 Bound residues
Several decades ago "aging" was frequently mentioned in the pesticide literature whereby was understood the phenomenon that chemicals – most frequently described for pesticides – were becoming less extractable and with this less bioavailable after a prolonged residence time in the soil. In recent years mention of this phenomenon has again become relevant where it now most frequently is linked to discussions of the formation of bound residues. On examination of literature on bound residues there seems to be some confusion concerning the use of this term. Therefore, IUPAC (the International Union of Pure and Applied Chemistry) has found it relevant to define the term bound residues as "chemical species (active ingredient, metabolites and fragments) originating from pesticides, used according to good agricultural practice, that are unextracted by methods which do not significantly change the chemical nature of these residues, but which remain in the soil. These non-extractable residues are considered to exclude fragments, recycled through metabolic pathways leading to naturally occurring products" as stated by Roberts (1984) and Kearney (1982).
The ability of the chemicals to be bound (sorbed) to the soil organic or mineral particles and to be degraded by the soil microorganisms is key properties when the leaching risk of the substances is to be assessed. These characteristics are determined by the molecular structure of the substances, but the current binding and degradation taking place in a given environment are further determined by the environment, e.g. the soil structure, biological activity, and the amount of water running through. The binding is more or less reversible, which was illustrated by e.g. Barriuso (1994) (Figure 13).
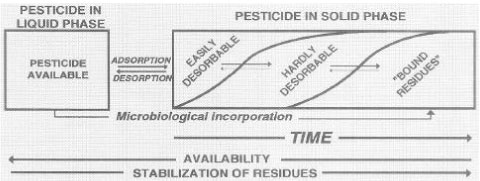
Figure 13. Distribution of pesticide over time between liquid phase and solid phase (Barriuso, 1994). The figure is reproduced with the kind permission from Elsevier.
Figur 13. Pesticidfordeling over tid mellem væskefase og jordfase (Barriuso, 1994). Figuren er gengivet med tilladelse fra Elsevier.
The distribution of pesticides between the aqueous phase and the soil phase was also illustrated by Barriuso (1994) as in Figure 14, where there is a distinction between a water-extractable part, a solvent-extractable part, and a non-extractable part of bound residues. Barriuso mentions a level of biocide pollution, which is found at the time when a lot of pesticide and/or metabolite is still present in the soil environment, which may have a measurable toxic effect on e.g. fish, plants or microorganisms; a level of chemical pollution, which is measured by chemical extraction methods where the efficiency of these methods influence on the given result; and a level of ecological pollution, which arises because he considers everything that is not measurable as active bound residues of the pesticide remaining in the environment. Barriuso (1994) was of the opinion that a chemical analysis with classic analytical techniques underestimates the chemical pollution, as not all bound residues present are extracted. At the same time Barriuso (1994) wrote that if the interesting part is the size of the amount of pesticide present in the soil that may have a toxic effect on soil organisms, then a chemical analysis will result in a overestimated result, as the chemical analysis is traditionally carried out with extraction agents that extract as large a quantity of the substance as possible whereas a toxic effect on soil organisms only occurs if the substance is present in the aqueous phase. In the same way a traditional chemical method of analysis will often overestimate the amount of chemical that may be a threat to the groundwater.
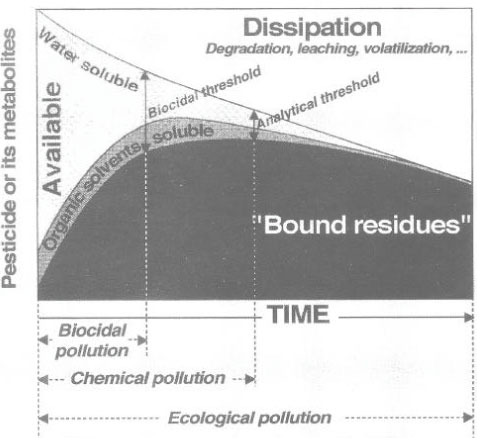
Figure 14. Soil pollution levels over time (Barriuso, 1994). The figure is reproduced with the kind permission from Elsevier.
Figur 14. Jordforureningsniveauer over tid (Barriuso, 1994). Figuren er gengivet med tilladelse fra Elsevier.
When an extraction method for chemicals in soil is being developed experiments with "spiked samples" are always carried out, that is experiments where known amounts of the chemical is added to the soil to which the extraction method is then applied. It is normal practice to leave the spiked sample for 24 hours as it is a well-known phenomenon that an extraction that is carried out immediately after adding the substance will overestimate the recovery percentage, which then does not cover the recovery percentage that exists when a "genuine" soil sample is analysed as the chemical in such a sample has been present for far more than 24 hours. Here, one is in reality also a long way into the problem concerning the lag period, which influences the binding (which again influences the degradation). Figures 15 and 16 show selected examples of the change in the desorption over time of pesticides to soil (Walker et al., 1995; Johnson et al., 2000).
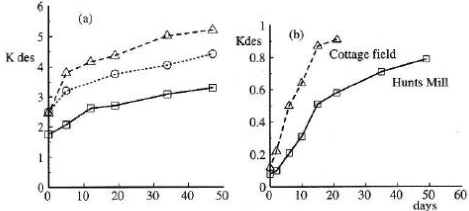
Figure 15. Changes in desorption over time for a) linuron, isoxaben, propyzamide and b) metsulfuron-methyl (Walker et al., 1995). The figure is reproduced with the kind permission from British Crop Protection Council.
Figur 15. Ændring af desorption over tid for a) linuron, isoxaben, propyzamid og b) metsulfuron-methyl (Walker et al., 1995). Figuren er gengivet med tilladelse fra British Crop Protection Council.
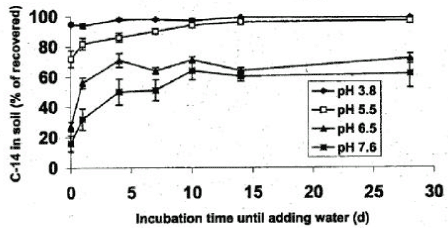
Figure 16. Change of sorption over time of imazmethabenz-methyl in soil at varying pH (Johnson et al., 2000). The figure is reproduced with the kind permission from Weed Science Society of America.
Figur 16. Ændring af sorption over tid af imazmethabenz methyl i jord ved varierende pH (Johnson et al., 2000). Figuren er gengivet med tilladelse fra Weed Science Society of America.
A number of previous studies have described how 14C-labelled bound residues have been activated. The addition of fresh soil and addition of glucose or manure increased the decomposition of 14C-parathion bound residue (Racke & Lichtenstein, 1985), and the addition of glucose increased the decomposition of 14C-atrazine bound residue (Khan & Bheki, 1990). Both studies explained the activation by an increased microbial activity. However, a chemical analysis of the mentioned 14C-labelled bound residues was not carried out. Therefore, it is important to be aware whether studies describing the activation of 14C bound residues may describe a naturally increased degradation of the organic matter where 14C from the pesticide in a molecular form that differs from the original has been built in.
Fomsgaard and Kristensen (2002) and Christensen (2002) showed that there is a significant difference between water-extractable and solvent-extractable isoproturon. Figure 17 shows selected results from Fomsgaard and Kristensen (2002) where the difference between the water-extractable and the solvent-extractable parts of isoproturon in a degradation experiment is significant.
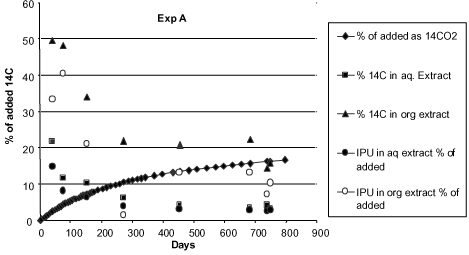
Figure 17. Degradation of 14C-isoproturon in soil 0.1 g/g. Water extractable and solvent extractable amounts of IPU and metabolites, calculated in % of added amount of 14C-isoproturon (Fomsgaard & Kristensen, 2002).
Figur 17. Nedbrydning af 14C-isoproturon i jord 0,1 g/g. Vandekstraherbare og solventekstraherbare mængder af IPU og metabolitter, beregnet i % af tilført mængde 14C-isoproturon (Fomsgaard & Kristensen, 2002).
When the amount of extractable substance in Fomsgaard and Kristensen (2002) decreases in the course of time, it is first and foremost due to the microbial degradation, which can also be seen from Figure 7 showing the formation of 14CO2 from the pesticide. Nevertheless, after about 750 days only about 18% of the added amount of 14C (calculated in equivalent amounts of 14C-isoproturon) is transformed to 14CO2. And 20% of the added amount can be extracted (4% with water and 16% with an organic solvent). The remaining amount of 14C (62%) will be built into the soil microorganisms and into the soil organic matter or will exist as bound residues so strongly bound that it cannot be extracted with a solvent. The fact that a formation of 14CO2 still takes place after 800 days is natural as there will still be a microbiological activity, which can transform the organic matter into/to which 14C now is built/strongly bound. A large pool of organic matter, which is decomposed in the course of one growing season, is constantly found in the upper soil layers. Bureaul et al. (1998) estimated that close on 8 t of organic carbon per hectare is decomposed in the course of one growing season.
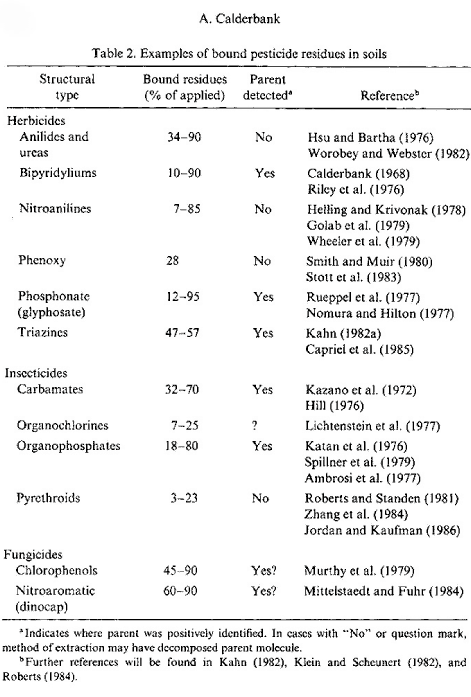
Figure 18. Outline of pesticides forming bound residues (Calderbank, 1989). The figure is reproduced with the kind permission from Springer Verlag.
Figur 18. Oversigt over pesticider, der danner bundne rester (Calderbank, 1989). Figuren er gengivet med tilladelse fra Springer Verlag.
Bound residues are formed to a greater or lesser extent from ALL types of substances. There have been many reviews on bound residues (Klein & Scheunert, 1982; Khan, 1982; Roberts, 1984; Führ, 1987), and in 1996 a major workshop was held on this subject (Führ, 1996). Calderbank (1989) summed up the lists of Klein and Scheunert (1982), Khan (1982), and Roberts (1984) of pesticides that form bound residues (Figure 18). The size of the amounts of bound residues stated in Calderbank's (1989) list that has been identified as starting substance or metabolite varies. Bound residues of glyphosate have e.g. been quantitatively extracted from a volcanic ash soil with a strong alkaline solution (Nomura & Hilton, 1977). The bipyridilium herbicides diquat and paraquat are unstable in alkaline solutions but can be extracted from soil if the soil is opened with a strong sulphuric acid (Tucker et al., 1967). Such a procedure destroys the soil colloids whereby the herbicides can be quantitatively extracted from the soil. This procedure can only be applied to a very few pesticides as most of them will be destroyed at the same time as the soil colloids are destroyed.
In an experiment with pirimicarb Hill (1976) showed that up to 70% of the added amount/quantity of pesticide was found as bound residues in the soil after 2 years. When extracted with 0.1M NaOH, 18% of this could be quantified as original pesticide. Khan (1982) showed that more than half the 14C-promethryn bound residues was present as the original molecule, and Capriel et al. (1985) showed that after 9 years 10% of the bound residues found in the soil from atrazine could be characterised as the original pesticide. As to substance groups such as ureas, anilides, phenoxy acids, nitroanilines, and pyrethroids, it has emerged that quantifiable amounts of the original substances cannot be extracted from the bound residues (Hsu & Bartha, 1976; Stott et al., 1983; Wheeler et al., 1979).
Burauel et al. (1998) quantified bound residues from measurements of 14C in soil from 20 different outdoor lysimeter experiments where the experiments were carried out with 14C-labelled atrazine, terbuthylazine, chloridazon, dichlorprop-P, methabenzthiazuron, pyridate and anilazine. After two years' experiments, more than 80% of the remaining 14C was found in the upper 30 cm of the soil column of which the total length was 110 cm. Burauel et al. (1998) stated that 50-90% of the remaining radioactivity in the upper layer in such experiments normally can be characterised as "bound residues", that is non-extractable substance, metabolites or fragments of substance. Burauel et al. (1998) pointed out, however, that in many cases the structure of these bound residues has not been explained. In his lysimeter experiment with methabenzthiazuron, he found that 28.8% of the added radioactivity could be extracted from the soil in the form of the original substance after 127 days whereas < 1% of the added radioactivity could be extracted from the soil in the form of the original substance after 6.5 years. The bioavailability for the following crops was minimal with the highest amount of built-in radioactivity of 2% found in winter wheat – and it cannot be ruled out that the wheat has assimilated 14CO2 as a part of the incorporated 14C. In the lysimeter experiment with anilazine only about 10% of the radioactivity in the soil was found to be extractable after just 6 weeks. As a supplement Burauel et al. (1998) carried out 13C-NMR studies of humic and fulvic acids, which had been extracted from soil incubated with 13C-anilazine. The typical dialkoxy binding, which occurs when anilazine is built into humic and fulvic acids, was present whereas the free original substance was not.
Fomsgaard et al. (2003a; 2003b) carried out lysimeter experiments with isoproturon and glyphosate. Experiments with 14C-labelled isoproturon were carried out as repeat determination in soil that had been treated conventionally whereas experiments with 14C-labelled glyphosate was carried out as repeat determination in soil that had been tilled with a reduced treatment. 48% and 54%, respectively, of the added radioactivity in the isoproturon experiments were found in the upper 10 cm of the soil while 17% and 14%, respectively, were found in soil from a depth of 10-20 cm. Extractions were carried out in the 0-10 cm soil, at first with an aqueous CaCl2 solution where 0.49% and 0.2%, respectively, of the added activity was extracted. By a subsequent extraction with acetonitril 1.19% and 0.56%, respectively, were found. Nothing extractable was found in the deeper layers. Because of the low extractable amounts no subsequent chemical analysis was carried out. In these lysimeter experiments, it must be noted that almost the whole amount of radioactivity left in the soil was bound residues. Further, it must be noted that some of the extractable amount (which may be assumed to be original matter or metabolites without it being known for certain) is more easily mobilisable than the rest, as it can be mobilised (extracted) with an aqueous solution while the rest needs acetonitrile. The possibility that the extractable substance may be mobilised under natural circumstances may be thought to be illustrated with the water extractable part. Whether the non-extractable part may be mobilised as an active pesticide or metabolite will need further investigation.
It is very difficult to predict how large an amount of pesticide remains that the soil can bind and deactivate, but it must be assumed that the amount of pesticide remains is very small compared with the large amount of natural organic matter, which forms part of the soil cycle every year and that therefore no saturation of the sites where the pesticide remains can be bound will ever occur, especially when it is taken into consideration that a degradation also takes place. It is normally a matter of less than 1 kg of carbon, which comes from pesticides, out of a total amount of carbon, which constitutes between 20,000 and 40,000 kg/ha in an average plough layer depth in agricultural soil.
Sequestration or entrapment in micropores is a phenomenon that has been studied and discussed very much in recent years. Waters and Oades (1991) and Bergström and Stenström (1998) described the differences in size between the soil pores, the chemical molecules, and the degrading organisms (Figure 19), and in their experiment with degradation of 2,4-D, Bergstöm and Stenström (1998) showed that after 7.7 days most of the chemical substance was found in micropores in the soil where there is no considerable exchange of water and where the bacteria cannot penetrate (Figure 20). Thus the availability of these parts of the substance is only possible if the substance diffuses into the major pores or if the degradation of the substances can take place by means of secreted enzymes from the microorganisms. Gevao et al. (2000) wrote that entrapment of chemicals in the soil could take place after a prolonged time or aging but did not define the prolonged time more closely. Dec and Bollag (1997) defined sequestration as a situation in which the substances could still be extracted from the soil. Sequestration differs from adsorption in that adsorption takes place relatively quickly whereas sequestration is a slower process; both processes can, however, take place at the same time (Huang et al., 1996; Pignatello & Xing, 1996). According to Alexander (1995), diffusion phenomena combined with sorption on available microsites, control the sequestration.
What the chemical structure is like when bound residues are concerned is another matter. Barriuso (1994) mentioned chemical binding of the pesticides and their metabolites to the other soil constituents, incorporation in the phenolic polymers, incorporation in the soil microorganisms after these have been in control of a metabolic activity or binding in microcavities in the polycondensates of the soil. However, according to IUPAC's definition of
< /p>
Figure 19. Size of soil particles compared with the size of pores and the size of chemical molecules and biota (from Bergström & Stenström, 1998). The figure is reproduced with the kind permission from The Royal Swedish Academy of Sciences.
Figur 19. Jordpartiklers størrelse sammenholdt med porestørrelser og størrelsen af kemiske molekyler og biota (from Bergström & Stenström, 1998). Figuren er gengivet med tilladelse fra The Royal Swedish Academy of Sciences.
bound residues, chemical binding or incorporation in the soil microorganisms can only be included as examples of the formation of bound residues if the foreign substance in its original form or identifiable parts of it can be released again from the new complex molecule via a microbial or enzymatic decomposition. If one looks at degradation process for bentazone proposed by Huber and Otto (1994), which is illustrated in Figure 21, the degradation products from bentazone are covalently bound in the complicated molecular structure, which is a part of the soil organic matter. Whether the parts of the foreign organic molecule that originates from bentazone become identifiable and measurable in the process where the organic matter is decomposed by the microorganisms in the soil is doubtful, however. It is more likely that parts of
< /p>
Figure 20. The influence of the soil structure on the availability of chemicals to degradation (Bergström & Stenström, 1998). The figure is reproduced with the kind permission from The Royal Swedish Academy of Sciences.
Figur 20. Jordstrukturens indflydelse på kemiske stoffers tilgængelighed for nedbrydning (Bergström og Stenström, 1998). Figuren er gengivet med tilladelse fra The Royal Swedish Academy of Sciences.
that which originates from the bentazone molecule are degraded in the big pool in which the degradation of the whole amount of organic matter in the soil takes place. Only if e.g. the A or B structure is released again in the soil – and cannot be extracted with extraction agents that change the chemical structure – will it be bound residues. Measuring bound residues is often stated as best being carried out with 14C techniques (Barriuso, 1994; Calderbank, 1989). However, when using simple 14C techniques, it is not possible to distinguish whether parts of the original substance have formed part of new chemical structures or whether the chemical structure of the pesticide or of a well-known metabolite has been preserved and the substance is just bound to the soil particles.
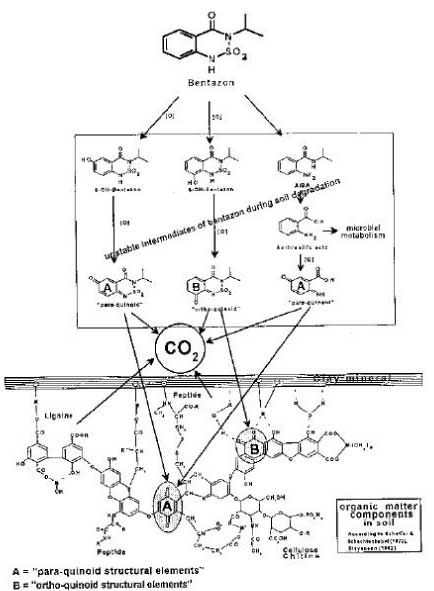
Figure 21. Proposed structure of bentazone degradation products built into the soil macromolecular organic matter (Huber & Otto, 1994). The figure is reproduced with the kind permission from Springer Verlag.
Figur 21. Foreslået struktur af bentazons nedbrydningsprodukter indbygget i jordens makromolekylære organiske materiale (Huber & Otto, 1994). Figuren er gengivet med tilladelse fra Springer Verlag.
Barriuso and Koskinen (1996) discussed which methods might be the most careful for identification of where the bound residues are found in the soil. They referred to studies by Andreux et al. (1991), Khan (1982), Capriel et al. (1985), and Bertin and Scaviona (1989), who had chemically fractioned the soil organic matter and found that the soil content of fulvic acid played an important part in the initial quick immobilisation of pesticides while a higher extent of bound residues formed by association of pesticides with more polymeric humine links was observed over time. Barriuso and Koskinen (1996) questioned whether the chemical fractioning with alkaline extraction agents had modified the soil and the pesticides to an extent where it was no longer a matter of bound residues. Barriuso and Koskinen (1996) were therefore advocates of a physical fractioning of the soil particles. Barriuso and Koskinen (1996) added 14C-labelled atrazine to the soil in a number of lysimeters and studied how the binding of the substance over time was distributed on different fractions of the soil, which were grouped according to particle size (Figure 22). The formation of bound residues was increased over the first 4 months. Non-humus parts of the soil organic matter with particles sizes of more than 50 m showed the largest capacity for the formation of bound residues, and the ability of the organic matter to form bound residues with atrazine decreased with smaller particle size. This was explained by the fact that the access of the microorganisms to the substance was expected to decrease in the most difficultly accessible finest fractions of aggregates. The most stable bound residues, however, were formed in the fraction where the particle size was like that of clay. Depending on where bound residues are found, their subsequent fate varies. Barriuso and Koskinen (1996) were of the opinion that if they are found in the coarsest fractions and are bound to not fully transformed plant material then they may partly be released again. But if they are bound to the finest particles, they may be leached to the groundwater by particle-bound transport.
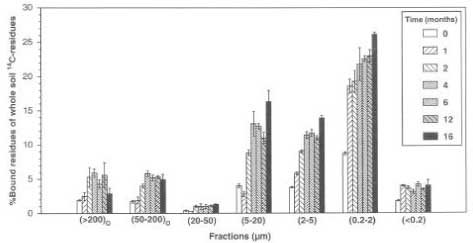
Figure 22. The distribution of bound residues from 14C-atrazine on different soil size fractions (Barriuso & Koskinen, 1996). The figure is reproduced with the kind permission from Soil Science Society of America.
Figur 22. Fordelingen af bundne rester fra 14C-atrazin på forskellige størrelsesfraktioner af jorden (Barriuso og Koskinen, 1996). Figuren er gengivet med tilladelse fra Soil Science Society of America.
Northcott and Jones (2000) reviewed analytical techniques for measuring organic bound residues in soil and sediment and described the most dominating techniques as "release and identify" techniques (Dec & Bollag, 1997). Gevao et al. (2000) stated that the separation between free and bound chemicals in the soil is not always easy. Even though a substance can easily be extracted without its chemical structure being changed, it will not be completely free. Therefore, "extractability" is defined from the extraction agent and the other experimental conditions under which the extractions take place. Many methods of extraction have been used over the years for the extraction of chemicals from soil for subsequent analysis. Soxhlet extraction, shaking techniques with a solvent or ultrasound treatments are the more traditional methods of extraction. In addition to these, the following methods are now in use: supercritical fluid extraction (Khan, 1995; Khan, 1996, Barnabas et al., 1994; Weber & Young, 1997; Rochette & Koskinen, 1997), microwave extraction (Stout et al., 1996; Molins et al., 1996; Lopez-Avila et al., 1996), silylation previous to the extraction (Bolag & Stotsky, 2000), and accelerated solvent extraction. "Release and identify" techniques can only to a smaller extent tell something about the types of binding based on the solvents that extract the substances. The methods that extract different fractions of the organic matter, such as humic acid, fulvic acid, and humine, have a more elucidating effect concerning bindings. Methanolic saponification, alkaline extraction, silylation of humic compounds, and pyrolysis have been used to release chemicals that have been strongly bound to or sequestrated in the soil organic matter (Northcott & Jones, 2000; Eschenbach et al., 1994; Haider et al., 1992; Wais et al., 1995; Thorn et al., 1996; Schulten & Leinweber, 1996; Khan & Hamilton, 1980). However, with such methods, which are based on extraction, fractioning or destruction, there is a risk that the methods have also influenced the amount or the chemical form of the substance that is to be studied. In recent years a number of non-destructive spectroscopic methods have therefore been introduced, which now are preferred if one wishes to study the interaction of chemicals with the soil constituents. These spectroscopic methods can be ESR (Electron Spin Resonance spectroscopy), FTIR (Fourier Transform InfraRed Absorption spectroscopy), Fluorescence spectroscopy or NMR spectroscopy (Nuclear Magnetic Resonance spectroscopy). ESR is a good technique for studies of charge-transfer, which for example can be the mechanism for the binding of diquats and atrazine to humic acid (Sanchezpalacios et al., 1992; Senesi et al., 1987). Infrared absorption spectroscopy is very practicable for identifying the structure of organic compounds, as the infrared absorption spectrum is complex even for relatively simple compounds. FTIR is an advanced form of infrared spectroscopy with which the changes taking place in the adsorption spectrum of the organic matter because of bindings to the foreign chemicals can be detected. FTIR is particularly suitable for substances where hydrogen bondings and proton transfers occur (Aochi et al., 1992; Martin-Neto et al., 1996; Weissmahr, 1997). Fluorescence spectroscopy is most frequently used for measuring the association of hydrophobic substances such as PAH to dissolved humic matter (Backhus & Gschwend, 1990; Chin & Gschwend, 1992). NMR has been used very much for characterising the humus matter of the soil and is a well-tested non-destructive spectroscopic technique. 13C-NMR is the preferred method for studying covalent and non-covalent interactions between specific xenobiotic organic molecules and natural organic matter (Hatcher et al., 1993; Nanny et al., 1996; Kim et al., 1997; Haider et al., 1992; Haider et al., 1993; Wais et al., 1995).
Roberts (1984) defined bound residues as "chemical species originating from pesticides, used according to good agricultural practice, that are unextracted by methods which do not significantly change the chemical nature of these residues". As mentioned above, this definition was used by IUPAC. Calderbank (1989) suggested a modification of this definition that took the bioavailability into consideration: "It may be that bound residues in soil could be defined as residues of the intact pesticide or degradation products derived from it that are no longer able to exert their original biological activity to any significant extent and/or cannot be extracted from the soil by extraction methods which do not degrade the compound unless such methods are able to destroy the soil structure without affecting the compound. Clearly the important matter is not so much how the residue is defined but the question of its biological availability". At a workshop held by the Deutsche Forschungsgemeinschaft, "Pesticide Bound Residues in Soil", a new definition of bound residues was suggested (Führ et al., 1996): "Bound residues represent compounds in soil, plant or animal which persist in the matrix in the form of the parent substance or its metabolite(s) after extractions. The extraction method must not substantially change the compounds themselves or the structure of the matrix. The nature of the bond can be clarified in part by matrix-altering extraction methods and sophisticated analytical techniques. To date, for example, covalent, ionic and sorptive bonds, as well as entrapments, have been identified in this way. In general the formation of bound residues reduces the bioaccessibility and the bioavailability significantly". The difference between the suggestion for a definition by Führ et al. (1996) and the existing IUPAC definition is important as Führ et al. (1996) incorporate a new condition, that the extraction agent must not change the chemical structure of either the pesticide or the soil matrix in order that it is possible to define the non-extractable part as bound residues, while IUPAC's definition only mentions that the extraction agent must not change the chemical structure of the pesticide in order that it is possible to define the non-extractable part as bound residues. In order to apply Führ's definition it would be necessary to define what it is meant by the soil matrix. If the soil structure is meant, that is the association of primary particles in secondary particles, then these can be destroyed by many substances, which would have as a result that, on the whole, practicable extraction agents for carrying out this separation could not be found. It must be assumed that by "matrix" is meant the soil colloids. The soil organic matter is to a most predominant extent bound to clay minerals and in that way forms an integrated part of the colloids. The clay minerals of the soil are affected by acids and bases, as they are more or less dissolved in these depending on the contact time (Borggaard, pers. comm.). It would therefore be obvious to regard organic solvents as extraction agents that can be used for distinguishing extractable residues from bound residues according to Führ's definition while to the extent that they do not destroy the foreign chemical, it will also be possible to use acids and bases as extraction agents according to IUPAC's definition. Whether IUPAC's or Führ's definition is used, it will nevertheless be necessary always to define the specific extraction procedure, but this limit will always solely be related to the chosen procedure and not to any naturally occurring clear-cut limit in the soil. The effect of the extraction agent on the soil colloids differs according to the given type of soil and perhaps even according to the different contents of iron compounds and organic matter in different soils (Christensen, pers. comm.).
Alkaline and acid extractions as carried out by Nomura and Hilton (1977), Tucker et al. (1967), and Hill (1976) would thus yield different results in different types of soil when one is to assess whether the extracted substance has been bound residues. This discussion will be relevant when assessing whether glyphosate that is extracted from soil with a strong alkaline solution is to be regarded as bound residues or not.
Jones et al. (2000) summed up the debate concerning bound residues: It has been shown for certain that bound residues are formed from potentially toxic/biologically active molecules in soil and that these residues are persistent. IF they can still be or again become bioavailable or biologically active although they cannot be extracted chemically – then they can still form part of the food chain or influence the fertility of the soil in the long run. Processes in the soil that result in the remobilization of bound residues may possibly also take place. If these assumptions are correct, bound residues are not to be desired. On the other hand, it is often pointed out that a reduced chemical extractability indicates that the substance has a weaker tendency to enter the soil water phase again and that lower extractability can be correlated with lower bioavailability. By this, the formation of bound residues must be seen as the first steps in a deactivation of the foreign organic molecule, which finally ends with a complete incorporation in the organic pool of the soil. Thus, increased binding and formation of bound residues can be understood as natural processes, which may even be used in the bioremediation as opposed to the manner in which bioremediation is most frequently carried out today, namely by increasing the bioavailability. "It seems likely that the significance, or otherwise, of bound residues in soils will ultimately be addressed by the bioavailability issue and this is likely to be the fOC us of ongoing research over the coming years" (Jones et al., 2000).
| Front page | | Contents | | Previous | | Next | | Top |
Version 1.0 March 2004, © Danish Environmental Protection Agency
|