| Front page | | Contents | | Previous | | Next |
Spatial differentiation in LCA impact assessment
6. Aquatic eutrophication
Background information for this chapter can be found in:
- Chapter 5 of the "Environmental assessment of products. Volume 2: Scientific background" from Hauschild and Wenzel (1998a).
- Chapter 5 of the "Background for spatial differentiation in life cycle impact assessment – EDIP2003 methodology" by Potting and Hauschild (2005)
6.1 Introduction
Eutrophication literally means "the process of becoming rich of nutrients". The eutrophying impact typically characterised in life cycle impact assessment relates implicitly to eutrophication of aquatic
ecosystems. This follows from the modelling of impact which in life cycle assessment usually takes its bases in the composition of aquatic biomass (Wenzel et al. 1997, Heijungs et al. 1992, Lindfors et al.
1995). Aquatic eutrophication is the subject of this chapter while impact assessment methodology for terrestrial eutrophication can be found in Chapter 5.
6.2 Classification
The nutrients, which normally limit biological growth in aquatic ecosystems, are nitrogen and phosphorus. For a compound to be regarded as contributing to aquatic eutrophication, it must thus contain
nitrogen or phosphorus in a form which is biologically available. In practice, Table 6.1 will cover all emissions in the inventory to be classified as aquatic eutrophying.
Free nitrogen (N2) is not regarded as a contributor to aquatic eutrophication, even if it is available for certain bacteria and algae. This is because emission of N2 has no additional eutrophying effect, inasmuch
as the greater part of the atmosphere already consists of free nitrogen.
Aquatic eutrophication can be caused by emissions to air, water and soil.
6.3 EDIP97 characterisation factors
Current characterisation factors for eutrophication allow adding contributions from nitrogen and phosphorus based on the Redfield ratio. These factors do in most cases not distinguish between aquatic
systems and terrestrial systems and model both as if they were impacts on aquatic systems. Also in EDIP97, terrestrial and aquatic eutrophication are taken together as one impact category called nutrient
enrichment. However, the Redfield ratio refers to the typical composition of aquatic phytoplankton:
C106H263O110N16P. A too large growth of phytoplankton, as a result of eutrophication, pushes aquatic ecosystems out of balance and starts a chain of ecological effects.
The EDIP97 factors from Wenzel et al. (1997) are listed in Table 6.1.
Table 6.1. Characterisation factors for eutrophication from Wenzel et al. (1997)
Substance |
Formula |
CF(N)
(g N-eq./g substance) |
CF(P)
(g P-eq./g substance) |
CF(ne)
(g NO3--eq/g substance) |
Nitrogen |
|
|
|
|
Nitrate |
NO3- |
0.23 |
0 |
1.00 |
Nitrogen dioxide |
NO2 |
0.30 |
0 |
1.35 |
Nitrite |
NO2- |
0.30 |
0 |
1.35 |
Nitrogen oxides |
NOx |
0.30 |
0 |
1.35 |
Nitrious oxide |
N2O |
0.64 |
0 |
2.82 |
Nitric oxide |
NO |
0.47 |
0 |
2.07 |
Ammonia |
NH3 |
0.82 |
0 |
3.64 |
Cyanide |
CN- |
0.54 |
0 |
2.38 |
Total Nitrogen |
N |
1.00 |
0 |
4.43 |
Phosphorus |
|
|
|
|
Phosphate |
PO43- |
0 |
0.33 |
10.45 |
Pyrophosphate |
P2O72- |
0 |
0.35 |
11.41 |
|
|
|
|
|
TotalPhosphorus |
P |
0 |
1.00 |
32.03 |
Wenzel et al. (1997) propose to aggregate impacts from nitrogen emissions (3rd column) and from phosphorus emissions (4th column) separately, but nevertheless also provide factors to add impacts from
phosphorus and nitrogen (5th column). The preferred separate aggregation of the two nutrients is due to the fact that phosphorus is typically limiting the growth of biomass in inland waters (rivers and lakes)
while nitrogen usually is the limiting nutrient in marine waters.
There are several problems with characterisation factors based on the Redfield ratio. Such factors do not take into account that:
- The hydrogeological conditions in the region of application determine the transport of nutrients from agriculture – by surface runoff/erosion (nitrogen and phosphorus), and groundwater drainage (nitrogen) –
to surface water.
- Phosphorus will usually not be removed again (at most temporarily stored in bottom sediment), but nitrogen does to some extent leave the aquatic system through denitrification (nitrate is used for respiration
in anaerobic biomass decomposition resulting in release of N2). The amount of nitrogen available for biomass growth over time is thus smaller than the amount entering the aquatic system.
- All nutrients remaining in the aquatic system will finally end up in marine waters, but some releases are directly to sea while most are first to inland waters and then through river transport reaching the sea.
- Aquatic ecosystems differ in their capacity to cope with eutrophication and subsequent phytoplankton growth, and the already operative "background" loading determines to what extent this capacity is used
and additional input of nutrient is harmful.
6.4 EDIP2003 characterisation factors
Until now, typical characterisation factors for aquatic eutrophication are based on the theoretical maximum amount of biomass that can be produced from a substance. As elaborated in the previous section,
the maximum potential for biomass growth is a poor measure of the eutrophying impact of emissions to aquatic ecosystems. In the current context, the CARMEN model3 is used to establish exposure factors
for aquatic eutrophication that overcome some of the identified problems (those relating to the fate of nutrients). The factors calculated by means of the Carmen model express the fraction of a nutrient
emission from agricultural soil or wastewater treatment plant that will reach and expose inland waters or marine waters, i.e. they relate emissions of nutrients to the resulting nutrient enrichment of
natural waters.
The CARMEN model (version 1.0) calculates the change in nutrient loads in ground water, inland waters (river catchment) and coastal seas from changes in input of nutrients. The nutrient inputs modelled by
CARMEN are atmospheric deposition of nitrogen on soil and coastal seas, phosphorus and nitrogen supply to agricultural soils, and phosphorus, and nitrogen discharged with municipal wastewater (see
Figure 6.1).
CARMEN models the transport of nutrients to surface water from agricultural supply, through groundwater drainage and surface runoff and through atmospheric deposition with a high spatial resolution
based on 124320 grid-elements of 10x10 minutes (roughly 100-250km2, depending on the longitude and latitude location of the grid-element). The nitrogen and phosphorus sources have been allocated to
each grid-element on the basis of the distribution of land uses in the given grid-element (arable land, grassland, permanent crops, forest, urban area, inland waters, others). The transport of nutrient by rivers
to sea is modelled rather straightforward in CARMEN assuming fixed removal rates of N and P in freshwater systems. The water flow is the main transport mechanism that brings nutrients from soil to
surface water. The routes modelled are deep groundwater drainage (nitrogen), runoff (nitrogen) or topsoil erosion (phosphorus) followed by river transport to coastal waters.
Figure 6.1 Main sources for nitrogen (continuous arrow) and phosphorus (dashed arrow) to soil, groundwater, surface waters and coastal seas addressed in the CARMEN model (Beusen not published).
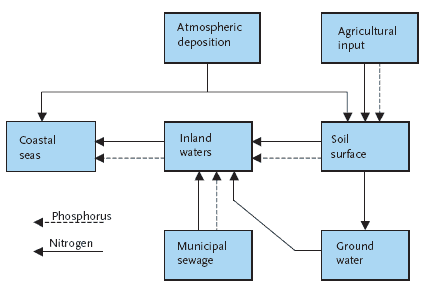
The eutrophication factors per country are calculated by changing the total amount of either nitrogen or phosphorus from a given source category in one country (other emissions for all countries and other
source categories remaining the same). Next, the increases in loading caused by this change of one country are accumulated over all river catchments and seas to obtain the factors which express what share
of the emission contributes to eutrophication of respectively inland waters and seas (in kg per kg released). For each source category, the change in eutrophying loads is calculated with a spatial resolution
over 101 river catchments and 32 coastal seas. In addition, the calculations for coastal seas also address atmospheric deposition as a nitrogen source. For atmospheric deposition, the CARMEN model
does not consider the relationships between country of origin and depositions to coastal seas. The model is therefore supplemented by data on the nitrogen deposited on European seas as a ratio of the
emission from their country of release.
A more detailed description of the CARMEN model and its use for calculation of site-dependent characterisation factors can be found in Potting et al., 2004a.
Site-generic exposure factors for Europe are given in Table 6.2, and Appendix 6.1 provides site-dependent exposure factors for 32 European regions relating nitrogen and phosphorus emissions by their
country of release to their eutrophication of inland waters and marine waters. The inland waters include all freshwater systems: lakes, streams, rivers and catchments while marine waters include the coastal
areas, brackish waters and open sea.
The site-dependent eutrophication factors express which share of an amount of nutrient released in a given country will contribute to eutrophication of
European inland waters and coastal seas. The CARMEN model does not include an assessment of the effects, i.e. whether this nutrient loading actually results in increased biomass growth and which effect
this has on the ecological quality of the water. The calculated factors thus represent the highest potential contribution to biomass growth (realistic worst case in the sense that removal of nutrients before the
reach the water has been taken into account). Compared to the EDIP2003 factors developed for terrestrial eutrophication and acidification, they thus cover a shorter part of the cause-impact chain and
should be seen as fate or exposure factors rather than as expressing an ecological effect in terms of eutrophication and biomass growth. The present state-of-the-art in integrated assessment modelling of
aquatic eutrophication does not allow such an effect assessment.
Being exposure factors, the EDIP2003 factors do not replace the EDIP97 factors which represent the relative content of nutrients in different compounds. Instead, they are to be used in combination with the
EDIP97 factors for separate characterisation of nitrogen compounds and phosphorus compounds. In the temperate and sub tropic regions of Europe, inland freshwaters are typically phosphorus limited while
marine waters typically are nitrogen limited (in the tropics, it may be the opposite). Respecting this distinction, inland waters and marine waters are treated as two sub-categories under the new impact
category aquatic eutrophication.
Application of both the EDIP2003 site-generic and the EDIP2003 site-dependent exposure factors in combination with the EDIP97 factors is straightforward (see Sections 6.5 and 6.6). The typical life
cycle inventories already provide the only additional information required for site-dependent assessment, namely the geographical region where the emission takes place.
The use of site-dependent exposure factors, however, adds only a moderate resolving power of at most a factor 7 difference between highest and lowest ratings. The moderate range found between the
highest and lowest site-dependent exposure factors justifies a certain reluctance in site-dependent assessment, considering that the modelling in itself also brings uncertainty into the site factors.
The main use of the site-dependent exposure factors for aquatic eutrophication is for sensitivity analysis.
What do the impacts express?
The EDIP2003 aquatic eutrophication potentials of a nutrient emission express the maximum exposure of aquatic systems that it can cause. In this respect they are similar to the EDIP97 nutrient enrichment
potential and they are also expressed in the same units, namely as N- or P-equivalents. However, compared to the EDIP97 impacts, a larger part of the fate of the substances is modelled, and the EDIP2003
impact potential thus represents
the fraction of the emission which can actually be expected to reach different aquatic systems. Where EDIP97 impacts represented a worst case eutrophication potential, the EDIP2003 eutrophication
potential can thus be seen as realistic worst case.
6.5 Proper inventory data
It is common practice in life cycle assessment to consider the topsoil of agricultural fields as part of the technosphere. The data in life cycle inventory for nutrient supply in agriculture therefore usually refer to
the amount of nutrient available for leaving the topsoil after plant uptake and binding. In case this figure is not known, Annex 6.3 provides factors which can be used to estimate the combined leaching and
run-off of nutrients from the agricultural soil if the applied quantity of fertiliser is known (i.e. before plant uptake and binding in the soil).
Similarly, the exposure factors of Table 6.2 and Annex 6.1 refer to the emission of nutrients with waste water, i.e. after the relevant waste water treatment. In case, the waste water treatment has not been
modelled as part of the inventory analysis, Potting et al., 2005a provides typical removal efficiencies for nutrients with different types of waste water treatment in Europe.
6.6 Site-generic characterisation
The moderate range between highest and lowest site-dependent exposure factors found in Annex 6.1 means that there is only little motivation for performing a full site-dependent exposure assessment for this
impact category since the additional resolution that is obtained is small.
There is, however, still good reason to perform site-generic characterisation using the new site-generic exposure factors from Table 6.2 in combination with the EDIP97 factors as described below. The
procedure is the same for both of the subcategories:
Inland waters and marine waters.
The site-generic aquatic eutrophication impact on inland waters and marine waters from a product system can be calculated according to the following formula:

(6.1)
Where: sg-EP(ae) = The site-generic aquatic eutrophying impact from an emission to inland waters or marine waters (in either N-equivalents or P-equivalents)
sg-AEEFs = The site-generic exposure factor from Table 6.2 that relates the emission of substance (s) by the given source category to the eutrophying impact on either inland waters or marine waters
CF(ne)s = The characterisation factor from Table 6.1 that allows aggregating substance (s) with other substances belonging to the same group (nitrogen or phosphorus compounds)
Es = The emission of substance (s) (in g/f.u)
Table 6.2. Factors for site-generic characterisation, and for site-dependent characterisation of eutrophication of inland waters and marine waters
Emission to inland
waters
|
Site-generic assessment
Site-
generic exposure
=
sg-AEEF(s)
|
Site-dependent assessment
Site-dependent exposure factors(factor
to be found in Annex 6.1) |
Substance/source category |
Factor |
(standarddeviation) |
factor = sd-AEEFi,s |
P-agricultural (*) |
0.06 |
(0.03) |
sd-AEEF|(P-agricultural) |
P-wastewater (**) |
0.88 |
(0.15) |
sd-AEEF |(P-wastewater) |
N-agricultural (*) |
0.53 |
(0.08) |
sd-AEEF |(N-agricultural) |
N-wastewater (**) |
0.59 |
(0.15) |
sd-AEEF |N-wastewater) |
Emission to marine waters |
Site-generic assessment |
Site-dependent assessment |
|
Site-generic exposure factors |
Site-dependent exposure factors |
Substance/source category |
= sg-AEEF(s) |
|
(factor to be found in Annex 6.1) |
|
Factor |
(standarddeviation) |
factor = sd-AEEF |(s) |
P-agricultural (*) |
0.06 |
(0.03) |
sd-AEEF |(P-agricultural) |
P-wastewater (**) |
1.00 |
|
sd-AEEF |(P-wastewater) |
N-agricultural (*) |
0.54 |
(0.08) |
sd-AEEF |(N-agricultural) |
N-wastewater (**) |
0.70 |
|
sd-AEEF |(N-wastewater) |
*These factors relate to nutrient emissions after plant uptake
**The factors for wastewater basically express what share is released directly to marine waters or indirectly through rivers in a European average
situation
The standard deviations given for each substance in Table 6.2 represent the spatial variation underlying the site-generic exposure factors.
6.7 Site-dependent characterisation
Given the moderate range between the highest and the lowest site-dependent exposure factors in Annex 6.1, there is, as stated earlier, only little motivation for performing a full site-dependent exposure
assessment for aquatic eutrophication. Instead, the site-dependent factors should be seen as an information for a sensitivity analysis and possibly also for reduction of the potential spatial variation in the
site-generic impact.
If site-dependent characterisation is performed, a three-step procedure has been developed. The aquatic eutrophying impact from a given product is in many cases determined by one or a few processes.
These are identified from a calculation of the site-generic impact as described in Section 6.6 (step 1), and, if wanted, the site-generic impact can be adjusted with the relevant site-dependent factors (step 2
and 3).
Step 1
The site-generic aquatic eutrophication impact from a product system, as calculated in the previous section, is broken down into the contributions from the separate processes. These contributions are then
ranked from the largest to the smallest contribution, and the process with the largest contribution is selected.
Step 2
The site-generic aquatic eutrophication impact from step 1 is reduced with the contribution of the process selected in step 1. Next, the site-dependent impact from the emissions of this process is calculated
with the relevant site-dependent aquatic exposure factors.

(6.2)
Where: sd-EP(ae)p = The site-dependent aquatic eutrophication impact from process (p) on inland waters or marine waters (in either N-equivalents or P-equivalents)
sd-AEEFs,i= The site-dependent exposure factor from Annex 6.1 that relates the emission of substance (s) by the relevant source category in country or region (i) where process
(p) takes place to the eutrophying impact on either inland waters or marine waters. Emissions from an unknown region or from non-European regions can as a first approach be represented by the
site-generic factors.
CF(ne)s = The characterisation factor from Table 6.1 that allows aggregating substance (s) with other substances belonging to the same group (nitrogen or phosphorus compounds)
Es,p = The emission of substance (s) from the selected process (p) (in g/f.u).
The exposure factors in Annex 6.1 for wastewater cover situations where the country of emission is known. As regards the distribution of waste water emissions between inland waters and marine waters,
the exposure factors of Annex 6.1 reflect the average situation in the given country. If an emission of nutrients is known to be released fully to inland waters, the factor should be
0.7 for nitrogen and 1.0 for phosphorus instead of the value found in Annex
6.1. If the full emission is to marine waters, the factors for inland waters should be 0 for both nitrogen and phosphorus.
The geographic region in which the emissions take place determines the relevant factors. As a first approach, emissions from a non-European or unknown region can be characterised using the site-generic
factors from Table 6.2. The standard deviations for these factors in Table 6.2 give a range for spatial variation covered by the site-generic factor within Europe. Given the size of the variation in emissions and
sensitivities within Europe, the site-dependent factor is expected to lie within this range for most regions also in the rest of the world. Expert judgement may be used in the interpretation to assess whether the
factor for emissions from processes in non-European regions should be found in the lower or upper end of the range.
Step 3
The site-dependent contributions from the process selected in step 1 are added to the adjusted site-generic contribution from step 2. Step 2 is repeated until the site-dependent contribution of the selected
processes is so large that the spatial variation can no longer influence the conclusion of the study (e.g. when the site-dependent share is larger than 95% of the total contribution).
6.8 Normalisation
The EDIP2003 person equivalents for aquatic eutrophication using the EDIP2003 exposure factors are 12 kg N-eq/person/year and 0.41 kg P-eq/person/year or in aggregated form 58 kg NO3
--eq/person/year.
Following the EDIP97 approach, the normalisation reference for aquatic eutrophication is based on the impact caused by the actual emission levels for 1995 (see Hauschild and Wenzel 1998d and
Stranddorf et al., 2005). Applying the EDIP2003 exposure factors for aquatic eutrophication together with the characterisation factors from EDIP97, the total impact from the European emissions is 4467 kt
N-eq/year and 151 kt P-eq/year or in aggregated form 21467 kt NO3--eq/year The person equivalent is calculated as an average European impact per person assuming a total European population of
3.70?108 persons. The calculation of the normalisation reference is documented in Annex 6.4.
6.9 Interpretation
Considering the moderate range found between the highest and lowest site-dependent exposure factors the main interest of the established site-dependent exposure factors lies in their use for representing
this part of spatial variation in a sensitivity analysis.
The exposure factors express the share of the emission that will contribute to eutrophication of respectively inland waters and seas (in kg per kg released). Combined with the EDIP97 or similar
characterisation factors for aquatic eutrophication, the exposure factors indicate the amount of phytoplankton that at maximum can be produced from the nutrient emission. Compared to the factors
developed for terrestrial eutrophication and acidification, the factors for aquatic eutrophication cover a shorter part of the cause-impact chain and are thus fate or exposure factors rather than factors which
express the ecological effect in terms of eutrophication and biomass growth.
Inland waters are typically phosphorus limited and marine waters mostly nitrogen limited. This should be reflected in the interpretation of the assessed eutrophying impact. The present state-of-the-art in
integrated assessment modelling of aquatic eutrophication does not allow a closer assessment of ecological effect. Annex 6.2 contains an overview of the ecological quality of rivers in a number of countries.
This overview may be used for a qualitative evaluation.
In Potting et al., 2005a the site-dependent exposure factors are also within the countries spatially resolved over a total of 101 river catchments and 42 marine waters. This information can be used, if desired,
for a qualitative effect evaluation in the interpretation.
6.10 Example
Applying the EDIP2003 factors, characterisation is performed on the inventory presented in Section 1.6.
Site-generic characterisation
As described in Section 6.6, first the site-generic impacts are calculated. The aquatic eutrophication impact shown in Table 6.3 is determined using the EDIP97 factors from Table 6.1 and the site-generic
exposure factors from Table 6.2.
Table 6.3. Site-generic aquatic eutrophication impacts expressed as N- and P-equivalents for one supporting block made from plastic or zinc.
Click here to see the Table
Using site-generic characterisation factors, the zinc supporting block has the largest N-equivalent while only the plastic block has any contributions to aquatic eutrophication with P. For both components the
aquatic nutrient enrichment is predominantly caused by NOx-emissions deposited to marine areas, while the contribution from the waterborne emissions is negligible. For the site-generic impacts, the potential
spatial variation is so large (as revealed by the spatially determined standard deviation) that the conclusion might change if spatial variation were to be included.
Therefore, a site-dependent characterisation is performed for those processes that contribute the most to the site-generic aquatic eutrophication impacts with N in order to reduce the spatially determined
uncertainty and strengthen the conclusion.
Site-dependent characterisation
Table 6.3 shows that the predominant contributions to the site-generic aquatic eutrophication impact are caused by the emissions of NOx to air. The contributions from emissions of NH3 to air and
NO3—N and NH4+-N to water are negligible in the overall impact. For the zinc component, the main sources for NOx emission are identified as the production of zinc from ore which takes place in
Bulgaria, the casting of the component which takes place in Yugoslavia and that part of the transport of the component, which takes place by truck through Germany (data not shown). For the plastic
component the main sources for NOx are found to be the production of plastic polymer in Italy, the flow injection moulding of the supporting block in Denmark and the transportation of the component by
truck, mainly through Germany (idem). The emissions from these processes contribute 99% and 75% of the full site-generic impacts of Table 6.3 for the zinc component and the plastic component
respectively (data not shown).
In the calculation of the site-dependent impacts for these key processes, the relevant site-dependent factors from Annex 6.1 are applied. The results are shown in Table 6.4.
Table 6.4. Site-dependent aquatic eutrophication impacts for key processes from either product system.
Zinc part |
Emissiong/f.u. |
Characterisation
factor,
Table 6.1g N-eq./g |
Aq. eutr. factor,
Annex 6.1g/g |
Impact sd-EP(ae)g N-eq/f.u. |
NOx emissions |
|
|
|
|
Zinc production, Bulgaria |
0.97 |
0.3 |
0.31 |
0.09 |
Zinc casting, Yugoslavia |
1.65 |
0.3 |
0.19 |
0.09 |
Transport,mainly Germany |
4.56 |
0.3 |
0.23 |
0.31 |
Total. zinc part |
|
|
|
0.5 |
Plastic part |
Emission |
Characterisation factor, Table 6.1 |
Aq. eutr. factor, Annex 6.1 |
Impactsd-EP(ae) |
|
g/f.u. |
g N-eq./g |
g/g |
g N-eq/f.u. |
NOx emissions |
|
|
|
|
Plastic production, Italy |
0.63 |
0.3 |
0.40 |
0.08 |
Flow injection moulding, Denmark |
0.48 |
0.3 |
0.41 |
0.06 |
Transport,mainly Germany |
1.74 |
0.3 |
0.23 |
0.12 |
|
|
|
|
|
Total, plastic part |
|
|
|
0.3 |
The site-generic impacts from the key processes are subtracted from the original site-generic impacts in Table 6.3 and the site-dependent impacts of Table 6.4 are added. The thus corrected aquatic
eutrophication impacts are found in Table 6.5 and the difference to the original site-generic impacts of Table 6.3 is illustrated in Figure 6.2.
Site-dependent characterisation reduces the size of the aquatic eutrophication impact with N slightly and reduces the dominance of the zinc component. For the zinc-based component, around 99% of this
impact is calculated using site-dependent characterisation factors, while the site-dependent share for the plastic-based component is around 75%. Even if the site-dependent characterisation was performed
for all the remaining processes in the product system, it is not likely that the result would change significantly, given their modest share in the total and the standard deviation. The spatially conditioned potential
for variation of the impact has largely been cancelled for both components.
Table 6.5. Aquatic eutrophication impacts as N-equivalents from either product system with site-dependent characterisation of key process emissions
| Aquatic eutrophication,
sd-EP(ae)
g N-eq./f.u |
Zinc component | 0.50 |
Plastic component | 0.35 |
Figure 6.2 Site-generic and site-dependent aquatic eutrophication impacts with N from the two product systems. For the site-dependent impacts, the site-dependent exposure factors have only been
applied for the key processes as described above.
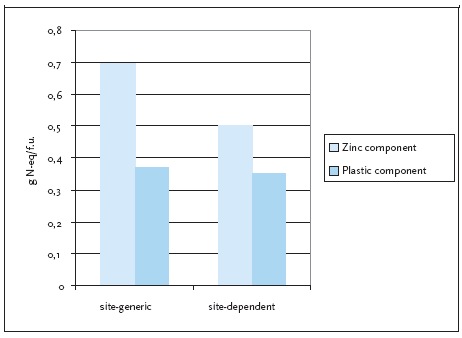
Annex 6.1: Site-dependent exposure factors for eutrophication of inland and marine waters
| Inlandwaters |
Marinewaters |
|
|
Nitrogen |
Phosphorus |
Nitrogen |
Phosphorus |
|
Agri |
WW |
Agri |
WW |
Agri |
WW |
NH3 |
NO2 |
Agri |
WW |
Albania |
0.53 |
0.57 |
0.1 |
0.81 |
0.53 |
0.7 |
0.29 |
0.32 |
0.1 |
1 |
Austria |
0.6 |
0.7 |
0.15 |
1 |
0.6 |
0.7 |
0.06 |
0.18 |
0.15 |
0.98 |
Baltic countries |
0.51 |
0.63 |
0.05 |
0.9 |
0.52 |
0.71 |
0.19 |
0.2 |
0.05 |
1 |
Belarus |
0.45 |
0.7 |
0.04 |
1 |
0.45 |
0.71 |
|
|
0.04 |
1 |
Belgium & Luxemburg |
0.56 |
0.66 |
0.05 |
0.94 |
0.58 |
0.7 |
0.19 |
0.27 |
0.06 |
1 |
Bulgaria |
0.56 |
0.7 |
0.03 |
0.99 |
0.55 |
0.7 |
0.13 |
0.31 |
0.03 |
1 |
Caucasus |
0.53 |
0.59 |
0.06 |
0.88 |
0.54 |
0.7 |
|
|
0.06 |
1 |
Czechia& Slovakia |
0.64 |
0.7 |
0.07 |
1 |
0.64 |
0.7 |
0.07 |
0.16 |
0.06 |
0.99 |
Denmark |
0.34 |
0.35 |
0.02 |
0.48 |
0.44 |
0.7 |
0.45 |
0.41 |
0.03 |
1 |
Finland |
0.57 |
0.46 |
0.04 |
0.64 |
0.64 |
0.71 |
0.29 |
0.32 |
0.04 |
1 |
France |
0.57 |
0.65 |
0.06 |
0.93 |
0.59 |
0.7 |
0.28 |
0.34 |
0.06 |
1 |
Germany, east |
0.53 |
0.66 |
0.03 |
0.94 |
0.55 |
0.7 |
0.16 |
0.23 |
0.03 |
1 |
Germany, west |
0.52 |
0.68 |
0.06 |
0.97 |
0.53 |
0.71 |
0.16 |
0.25 |
0.06 |
1 |
Greece |
0.51 |
0.42 |
0.04 |
0.63 |
0.51 |
0.7 |
0.38 |
0.55 |
0.04 |
1 |
Hungary |
0.5 |
0.7 |
0.03 |
1 |
0.51 |
0.69 |
0.07 |
0.16 |
0.02 |
0.99 |
Iceland |
0.64 |
0.59 |
0.09 |
0.88 |
0.64 |
0.7 |
|
|
0.09 |
1 |
Ireland |
0.62 |
0.64 |
0.13 |
0.91 |
0.62 |
0.7 |
0.51 |
0.69 |
0.13 |
1 |
Italy |
0.52 |
0.55 |
0.06 |
0.79 |
0.52 |
0.7 |
0.29 |
0.4 |
0.06 |
1 |
Moldavia |
0.5 |
0.7 |
0.02 |
1 |
0.51 |
0.68 |
0.1 |
0.2 |
0.02 |
0.98 |
the Netherlands |
0.26 |
0.31 |
0.03 |
0.37 |
0.36 |
0.72 |
0.27 |
0.38 |
0.03 |
1 |
Norway |
0.56 |
0.5 |
0.08 |
0.71 |
0.64 |
0.71 |
0.52 |
0.51 |
0.09 |
1 |
Poland |
0.47 |
0.69 |
0.03 |
0.98 |
0.47 |
0.7 |
0.11 |
0.18 |
0.03 |
1 |
Portugal |
0.62 |
0.52 |
0.06 |
0.75 |
0.62 |
0.7 |
0.37 |
0.44 |
0.06 |
1 |
Rumania |
0.57 |
0.7 |
0.04 |
1 |
0.57 |
0.7 |
0.08 |
0.18 |
0.04 |
1 |
Russia |
0.55 |
0.6 |
0.04 |
0.86 |
0.55 |
0.7 |
0.18 |
0.38 |
0.04 |
1 |
Spain |
0.61 |
0.6 |
0.03 |
0.86 |
0.61 |
0.7 |
0.25 |
0.41 |
0.03 |
1 |
Sweden |
0.52 |
0.56 |
0.04 |
0.83 |
0.59 |
0.71 |
0.37 |
0.38 |
0.04 |
1 |
Switzerland |
0.63 |
0.7 |
0.12 |
1 |
0.65 |
0.7 |
0.06 |
0.19 |
0.12 |
1 |
Turkey |
0.53 |
0.59 |
0.06 |
0.88 |
0.54 |
0.7 |
|
|
0.06 |
1 |
Ukraine |
0.49 |
0.68 |
0.03 |
0.97 |
0.5 |
0.7 |
0.11 |
0.17 |
0.03 |
1 |
United Kingdom |
0.53 |
0.58 |
0.08 |
0.84 |
0.6 |
0.71 |
0.48 |
0.57 |
0.09 |
1 |
Yugoslavia |
0.59 |
0.69 |
0.09 |
0.99 |
0.59 |
0.69 |
0.08 |
0.19 |
0.09 |
0.98 |
Mean |
0.53 |
0.59 |
0.06 |
0.88 |
0.54 |
0.7 |
0.23 |
0.32 |
0.06 |
1 |
Standard deviation |
0.08 |
0.15 |
0.03 |
0.15 |
0.08 |
|
0.15 |
0.14 |
0.03 |
|
Minimum |
0.26 |
0.05 |
0.02 |
0.37 |
|
|
0.06 |
0.16 |
|
|
Maximum |
0.64 |
0.07 |
0.15 |
1 |
0.65 |
|
0.52 |
0.69 |
0.15 |
|
Annex 6.2: Percentage of river reaches in various European countries classified as being of good, fair, poor or bad quality River reaches of good quality have nutrient-poor water, low levels of
organic matter, saturated with dissolved oxygen, rich invertebrate fauna, and suitable spawning ground for salmonid fish. River reaches with moderate organic pollution and nutrient content, good oxygen
conditions, rich flora and fauna, large fish population are classified as fair. Poor quality river reaches have heavy organic pollution, usually low oxygen concentrations, locally anaerobic sediment, occasional
mass occurrence of organisms insensitive to oxygen depletion, small or absent fish population, periodic fish kill. Of bad quality are those rivers with excessive organic pollution, prolonged periods of very low
oxygen concentration or total deoxygenation, anaerobic sediment, severe toxic input, devoid of fish (Kristensen and Hansen 1994). It should be noted that The inventory given in the table is based on very
different numbers of rivers and river stations in each country, and the classification is based on the specific countries own measurements, which have not been intercalibrated.
Country |
Good |
Fair |
Poor |
Bad |
Austria (1991) |
14 |
82 |
3 |
1 |
Belgian Flandern (1989-1990) |
17 |
31 |
15 |
37 |
Bulgaria (1991) |
25 |
33 |
31 |
11 |
Croatia |
15 |
60 |
15 |
10 |
Czech republic |
12 |
33 |
27 |
28 |
Denmark (1989-1991) |
4 |
49 |
35 |
12 |
England/Wales (1990) |
64 |
25 |
9 |
2 |
Finland (1989-1990) |
45 |
52 |
3 |
0 |
Former west Germany (1995) |
44 |
40 |
14 |
2 |
Iceland |
99 |
1 |
0 |
0 |
Ireland (1987-1990) |
77 |
12 |
10 |
1 |
Italy |
27 |
31 |
34 |
8 |
Latvia |
10 |
70 |
15 |
5 |
Lithuania |
2 |
97 |
1 |
0 |
Luxemburg |
53 |
19 |
17 |
11 |
The Netherlands |
5 |
50 |
40 |
5 |
Northen Ireland (1990) |
72 |
24 |
4 |
0 |
Poland |
10 |
33 |
29 |
28 |
Romania |
31 |
40 |
24 |
5 |
Russian Federation |
6 |
87 |
5 |
2 |
Scotland (1990) |
97 |
2 |
1 |
0 |
Slovenia (1990) | 12 | 60 | 27 | 1 |
Annex 6.3: Leaching and surface run off of nutrients from soil
The factors are used for estimation of combined leaching and surface run off of nutrients from agricultural soil to surface waters after plant uptake and binding (the exposure factors of Table 6.1 relate to this
kind of inventory information). To be applied in the case where inventory information regards the quantity of nutrients applied rather than the emission from the soil.
|
Nitrogen after plant uptake andbinding
(in kg/kg applied) |
Phosphorus after plant uptake
(in kg/kg applied) |
|
Grassland<100 kg Nappl./ha |
Grassland>100 kg Nappl./ha |
Arable &Natural land |
All land types |
Sand |
0.00 |
0.15 |
0.25 |
0.10 |
Loam |
0.00 |
0.10 |
0.18 |
0.10 |
Clay |
0.00 |
0.05 |
0.10 |
0.10 |
Peat |
0.00 |
0.01 |
0.05 |
0.10 |
Annex 6.4: Normalisation reference for aquatic eutrophication
Based on national emission inventories of nutrient emissions for a number of European countries provided by Larsen, 2005a, European normalisation reference is calculated for aquatic eutrophication
applying the EDIP2003 exposure factors and the EDIP97 characterisation factors according to Equation 6.2 for site-dependent aquatic eutrophication impact:

A number of assumptions have been made:
1. For the EU15 countries, the inventories of waterborne emissions of N and P report the quantity from each country which annually reaches the sea (Baltic, North Sea/Atlantic ocean or Mediterranean).
There is thus no need for application of an exposure factor since the removal, which occurs between emission from agriculture or waste water treatment plant and the sea, has already occurred.
2. A correction is performed to assess the man-made share of the total nutrient load reaching the sea. Danish results show that 85-90% of the waterborne emissions of nitrogen and phosphorus are
man-made. Assuming that these figures are valid for Europe, the total emission inventories are multiplied by a factor 0.88 to arrive at the total annual man-made emissions reaching the seas around Europe.
3. For the national emission inventories of the air-borne nutrient emissions of NOx and NH3, the relevant national AEEFs are found in Annex 6.1 and applied together with the nutrient enrichment
characterisation factors from EDIP97.
The calculation of the aquatic eutrophication normalisation reference is shown in the table below.
The normalisation references are calculated by dividing the total EU-15 impacts for 1994 by the total population in the EU15 countries in 1994:
Aquatic eutrophication normalisation
references |
Nutrient |
EU15 emission1994 |
EU15 populationMillion persons in 1994 |
Normalisation reference1994 |
Nitrogen |
4467 kt N-eq./year |
|
12 |
|
|
370 |
kg N-eq./person/year |
Phosphorus |
151 kt P-eq./year |
|
0.41 |
|
|
|
kg P-eq./person/year |
N and P |
21467 kt NO3- |
|
58 |
|
-eq./person/year |
|
kg NO3--eq./person/year |
Country |
Population |
Riverine discharges |
|
|
Total N |
Total P |
Anthropogenic N |
Anthropogenic P |
|
(Mio) |
(kt/year) |
(kt/year) |
(kt/year) |
(kt/year) |
Germany |
81.1 |
376.4 |
13.1 |
331.2 |
11.5 |
United Kingdom |
58.2 |
376 |
36 |
331 |
32 |
France |
57.7 |
185 |
8 |
163 |
7 |
Italy |
57 |
346 |
29 |
304 |
26 |
Spain |
39.1 |
185 |
11 |
163 |
9.7 |
Netherlands |
15.3 |
490 |
27.5 |
431 |
24.2 |
Greece |
10.4 |
117 |
7 |
103 |
6.2 |
Belgium |
10.1 |
47 |
2 |
41 |
1.8 |
Portugal |
9.9 |
15.7 |
14.2 |
13.8 |
12.5 |
Sweden |
8.7 |
137.8 |
5 |
121.3 |
4.4 |
Austria |
8 |
|
|
0 |
0 |
Denmark |
5.2 |
140.6 |
4.5 |
123.7 |
4.0 |
Finland |
5.1 |
66.1 |
3.6 |
58.2 |
3.2 |
Ireland |
3.6 |
179.1 |
10.5 |
157.6 |
9.2 |
Luxemburg |
0.4 |
|
|
0 |
0 |
EU-15 |
369.8 |
2661.7 |
171.4 |
2342.3 |
150.8 |
Country |
Population |
Airborne emissions |
|
|
NOx |
AEEF for N0x to air |
NH3 |
AEEF for NH3 to air |
|
(Mio) |
(kt/Year) |
(g/g) |
(kt/Year) |
(g/g) |
Germany |
81.1 |
2266 |
0.24 |
623 |
0.16 |
United Kingdom |
58.2 |
2387 |
0.57 |
320 |
0.48 |
France |
57.7 |
1682 |
0.35 |
667 |
0.28 |
Italy |
57 |
2157 |
0.4 |
389 |
0.29 |
Spain |
39.1 |
1223 |
|
344 |
|
Netherlands |
15.3 |
530 |
0.38 |
172 |
0.27 |
Greece |
10.4 |
357 |
0.55 |
445 |
0.38 |
Belgium |
10.1 |
374 |
0.27 |
79 |
0.19 |
Portugal |
9.9 |
249 |
0.44 |
93 |
0.37 |
Sweden |
8.7 |
444 |
0.38 |
51 |
0.37 |
Austria |
8 |
171 |
0.18 |
86 |
0.06 |
Denmark |
5.2 |
276 |
0.41 |
94 |
0.45 |
Finland |
5.1 |
288 |
0.32 |
41 |
0.29 |
Ireland |
3.6 |
117 |
0.69 |
125 |
0.51 |
Luxemburg |
0.4 |
23 |
0.27 |
7 |
0.19 |
EU-15 |
369.8 |
12544 |
|
3536 |
|
Country |
Population |
Site-dependent aquatic eutrophication potential |
|
(Mio) |
kt N-eq./year |
kt P-eq./year |
kt NO3--eq. /year |
Germany |
81.1 |
576.1 |
11.5 |
2684.84 |
United Kingdom |
58.2 |
865 |
32 |
4192.75 |
France |
57.7 |
492.6 |
7. |
2269.32 |
Italy |
57 |
655.8 |
26 |
3190.94 |
Spain |
39.1 |
162.8 |
9.7 |
822.36 |
Netherlands |
15.3 |
530 |
24.2 |
2604 |
Greece |
10.4 |
301 |
6.2 |
1401 |
Belgium |
10.1 |
84 |
1.8 |
393 |
Portugal |
9.9 |
75 |
12.5 |
465 |
Sweden |
8.7 |
187 |
4.4 |
880 |
Austria |
8 |
13 |
0 |
60 |
Denmark |
5.2 |
192 |
4.0 |
896 |
Finland |
5.1 |
96 |
3.2 |
458 |
Ireland |
3.6 |
234 |
9.2 |
1136 |
Luxemburg |
0.4 |
3 |
0 |
13 |
EU-15 |
369.8 |
4467 |
151 |
21467 |
Footnotes
3 CARMEN is an acronym for CAuse effect Relation Model to support Environmental Negotiations. It is an integrated assessment model to analyse and evaluate strategies to reduce nutrient loading of
inland waters and coastal seas in Europe. The model does not contain an assessment of ecological effects, but calculates the change in nutrient loads in ground water, inland waters (river catchments) and
coastal seas from changes in nutrient emissions and supplies (i.e. the causes).
| Front page | | Contents | | Previous | | Next | | Top |
Version 1.0 january 2006, © Danish Environmental Protection Agency
|