| Front page | | Contents | | Previous | | Next |
Background for spatial differentiation in LCA impact assessment - The EDIP2003 methodology
2 General issues
2.1 Introduction
2.2 The basis for spatial differentiation
2.3 Levels of sophistication and uncertainties in impact modelling
2.4 The mathematical framework for spatial differentiation
2.5 Application of site-dependent characterisation factors
2.6 LCA in relation to RA and EIA
2.7 Threshold exceedance in LCA
2.8 Site-dependent normalisation
2.9 Temporal differentiation in LCA
2.10 References
Authors: José Potting [6] and Michael Hauschild [7]
The text in this Chapter has been modified from Chapter 7 in Potting (2000).
2.1 Introduction
This Technical Report explores possibilities and limitations, as well as the relevance of spatial differentiation for characterisation of emission related impact categories in present life cycle impact assessment.
Only conceptual thoughts were available at the start of the research. These thoughts have in the course of the research evolved into a sound framework. This chapter discusses a number of issues of general
relevance in relation to this framework and the following chapters on individual impact categories.
2.2 The basis for spatial differentiation
The effective implementation of control measures in the industrialised countries has by now led to a situation where risk from a single source to its local environment is prevented in most cases. As elaborated
in Potting and Hauschild (1997), many environmental problems nowadays are characterised by the fact that the exposure of any receptor is the result of the - long distance transport of - emissions from very
many sources. The background exposure of a single receptor thus usually results from a multiplicity of sources. The other way around, a single source often contributes to the exposure of many receptors
because of long distance transport. The consequence of the multiple source, multiple receptor character of present environmental problems is that a single source contributes only very little, in many cases
marginally to the exposure of its receptors (except for exposures within the first kilometres from the source).
The small or marginal contribution from a single source to exposure of its receptors means that the time behaviour of the emission from that source (i.e. whether it is a flux or a pulse) becomes less important.
The temporal variation of the contributions from a single source emission will usually namely to a large extent be cancelled out against the high background exposure from all sources together. Exposure of
receptors thus shows a relative invariability in time for the contributions of single sources (this does not imply the opposite reasoning, that the temporal variations in the total exposure of receptors are
unimportant).
If the exposure of receptors shows a relative invariability in time for the contributions of a single but full source, the same of course inherently holds true for the emission per functional unit. The temporal
variation of emissions is thus of minor importance in impact assessment in LCA, except for local impacts or extreme situations (like the very slow emissions of landfill). This is an important learning, because
the lack of information about the temporal variation of emissions has long been an issue of intensive debate in LCA.
The multiple source, multiple receptor character of present environmental problems also provides the possibility to establish site-dependent characterisation factors. The long distance transport of emissions
means that one has to look over several hundreds to thousands kilometres to catch most of the impact from a source. The large impact area of an emission makes the precise location of a source of less
importance because the dispersion patterns and impact area of neighbouring sources overlap (i.e. show largely the same gradients). Dispersion patterns and impact area will only start to deviate considerably
when sources are located at larger distances from each other. This makes it possible to establish site-dependent characterisation factors that with reasonable to good accuracy estimate the impact from a
source located in a given region (as distinct from the characterisation factors for a source in another region). The geographical region of emissions is often already provided by inventory analysis in present
LCAs.
The lack of temporal and spatial information from inventory analysis has always been put forward as the main reason for LCA not being able to take into account spatial differentiation. As already mentioned
before, temporal information is only of limited relevance, while the relevant level of spatial information is usually already available from inventory analysis in the present LCA. Spatial differentiation is thus not
seriously limited by a lack of spatial and temporal information from the inventory phase in LCA.
The multiple source, multiple receptor character holds for most of the present "non-local" environmental problems that are the result of atmospheric emissions of mainly a limited number of substances with
relatively long lifetime (stratospheric ozone depletion, global warming, acidification, tropospheric ozone formation, and terrestrial eutrophication). It is less evident for the problem of toxicity with its large and
still growing number of potential toxic substances that have relatively short as well as rather long lifetimes and which are emitted sometimes by a limited number of sources only. It is obvious that in the points
presented above about marginal contributions, the temporal aspects and the overlapping impact areas are more "valid" for toxic substances emitted by multiple sources as well as for substances with a long
lifetime [8] (than for short-lived substances emitted by few sources only).
The multiple source, multiple receptor reasoning may not be valid for all impact categories or for all the substances within an impact category. However, it represents a line of thinking that is considered to
roughly describe the state of the present environmental situation. This line of thinking has been one of the main pillars of the methodology development and consensus project.
The multiple source, multiple receptor reasoning is most obvious for environmental problems related to air emissions. However, problems related to waterborne emissions (like aquatic eutrophication) tend to
show similar characteristics (EEA 1998). The full water system through which a waterborne emission disperses, and the background concentrations from a multiplicity of other sources have to be considered
to characterise the full impact from a single source.
2.3 Levels of sophistication and uncertainties in impact modelling
Potting and Hauschild (1997) argues that more sophistication should only be added to characterisation for the purpose of increasing the resolving power of the impact modelling. This requires the
uncertainties introduced by this additional sophistication to be in balance with the gain of information it provides.
The levels of sophistication in impact assessment can roughly be seen in two directions:
The extent to which the relevant parameters (or environmental mechanisms) in the causality chain are taken into account in the characterisation factors. That is, whether these factors are based on no, some or
full modelling of fate, exposure [9] and effect.
The extent to which spatial (and temporal) variation is allowed in each parameter of the modelling underlying the characterisation factors.
The characterisation factors developed in this technical report are in general sophisticated in both senses. They cover a large range of the relevant parameters in the causality chain, and they allow a high
degree of spatial variation.
The term "causality chain" is avoided in ISO terminology (ISO 14042 2000), because complex networks are involved rather than linear chains of environmental mechanisms (Udo de Haes et al. 1999).
Though often complex and non-linear, there is nevertheless some sequence in the environmental mechanisms that make an emission to have its final effect. Therefore the term "causality chain" was preferred
in this report. The causality chain for global warming could for example be (Udo de Haes et al. 1999): emission of CO2 increased radiative forcing climate change (i.e., temperature rise) rise of sea level
flooding of land damage to flora, fauna, and human beings (e.g., year of human life lost).
The impact to assess can basically be defined anywhere in the causality chain. There is a tendency in LCA to define the impact (or category indicator in ISO-terminology) as far as possible in the causality
chain for the given impact category (Braunschweig et al. 1998, Udo de Haes et al. 1999, Goedkoop et al. 1998). The emissions related to global warming are then no longer aggregated on the basis of their
increased radiative forcing (as now typical), for example, but modelled up to the years of human life lost as a consequence of (the complex network of changes caused by) a temperature change that is
expected to result from increased radiative forcing.
Definition of the impacts further on in the causality chain makes them easier to interpret in the subsequent weighting if this facilitates aggregation across impact categories. Goedkoop et al. (1998) and
Hofstetter (1998) therefore express all impact categories in similar damages to humans and ecosystems and resource depletion for example. Such aggregation is largely based on natural sciences and this
limits the "uncertainty" (or avoids the lack of credibility) from a value-based aggregation. On the other hand, uncertainties can rapidly become larger if the impact is defined that far in the causality chain where
little is known about environmental mechanisms.
The site dependent factors in this technical report are defined at different levels in the causality chains. Factors for acidification, terrestrial eutrophication, photochemical ozone and noise are modelled up to
and including evaluation of threshold exceedance. The factors for aquatic eutrophication, human and ecotoxicity do not cover an evaluation of threshold exceedance and basically reflect a weighed exposure
assessment. The chosen level in each impact category reflect the state-of-the-art modelling for the given impact category (see also next section), and a fair balance is aimed for between gain of information
and accuracy in modelling further up in the causality chain.
An interesting learning from this technical report is, that the spatial differences become more pronounced, up to a factor thousand between lowest and highest ranking, when indicators are defined further in
the causality chain (see also Potting 2000).
This technical report shows that spatial differentiation is feasible and provides reasonable to considerable resolving power in LCA. The credibility of LCA results has been and still is discussed intensively
among practitioners and users of LCA. A main reason for debate was the poor accordance between the expected occurrence of actual impact and the impact predicted by present life cycle impact
assessment (see also Chapter 1). The increased resolving power obtained by spatial differentiation reflects the expected variation in occurrence of actual impact. Spatial differentiation therefore improves the
credibility and therewith relevance of the modelling in present life cycle impact assessment.
2.4 The mathematical framework for spatial differentiation
The mathematical framework used in Chapter 3, 4 and 5, 6 and 7 differs from the one initially described by Potting and Hauschild (1997a,b) and used in Chapter 8 in that it is more sophisticated. An
emission does usually not disperse equally, but will show a spatially differentiated pattern of distribution to its receptors as a result of the processes of environmental fate and transport. The contribution from
an emission to exposure increase must preferably be calculated individually for each receptor. Starting from the receptor side, the total exposure of each receptor usually results from the emissions of very
many sources. There is also a spatial variation in background exposures among receptors. The background exposure of receptors should preferably also be quantified for each receptor [10]. To assess the
impact from a source on all its receptors, ideally, first the impact has to be calculated for each receptor individually, and next these contributions have to be aggregated over all receptors (the procedure is
exemplified in Chapter 4 about terrestrial eutrophication):
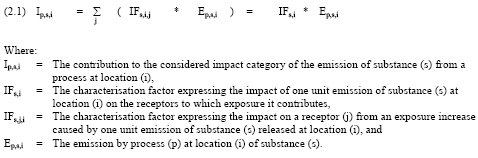
Formula 2.1 represents a sophisticated approach by considering one source to expose multiple receptors.
Such sophisticated approach was until recently regarded by many practitioners impossible in LCA. It would put an infeasible additional data demand on life cycle inventory with regard to the spatial
characteristics of the source, and on the impact assessment phase with regard to the background concentrations and sensitivities of receptors. Therefore, initially a simpler approach was proposed by Potting
and Hauschild (1997a,b).
This approach was based on the assumption that a few standard sources and a few standard target systems or receiving environments can be distinguished. The impact of each source type would sufficiently
be described by considering it to have one standard receiving environment (represented by an average receptor instead of by a multiplicity of receptors as in Formula 2.1).
Spatial differentiation could be obtained by dividing the world into a limited number of standard source types and standard receiving environments. The impact of a source type on the relevant standard
receiving environment could then be characterised by a site factor, possibly distinguished in a fate factor and a factor for the receiving environment (the target factor), which modifies the impact resulting from
the conventional site-generic framework:
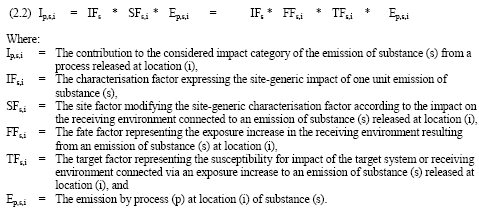
The fundamental difference between Formula 2.1 and 2.2 is the type of modelling underlying each. Formula 2.2 assumes one source to expose one standard environment existing of one average receptor,
whereas Formula 2.1 has a more sophisticated approach by considering one source to expose multiple receptors. It is an important learning of this technical report that the more sophisticated modelling can
be used quite well to establish characterisation factors.
A number of impact categories is described in models that can be used to establish characterisation factors according to the sophisticated approach. Such sophisticated modelling does not exist for
characterisation categories as toxicity or eutrophication via water. However, this expresses the limitations in the relevant field of science rather than limits posed by LCA itself. Spatially differentiated
characterisation factors for these characterisation categories can be established according to the more simple approach. Inventory analysis usually provides the needed spatial information, and models for the
relevant characterisation categories are often available that facilitates spatial differentiation in LCA.
The use of both the sophisticated and simpler approach can be exemplified with the characterisation factors in this technical report. The factors for acidification, terrestrial and aquatic eutrophication and
photochemical ozone are established according to Formula 2.1, the factors for noise and ecotoxicity are established according to Formula 2.2, whereas the factors for human exposure increase are a mixture
of both frameworks. The hybrid approach for human exposure is chosen to adequately account for exposure increase local to the source and to respect the requirements of feasibility in LCA at the same time
(see also Chapter 7).
2.5 Application of site-dependent characterisation factors
Application of the site-dependent characterisation factors requires data additional to current life cycle impact assessment. This is for the majority of impact categories restricted to the geographical region
where an emission takes place.
The requirement of additional data is often put forward as an objection against spatial differentiation in impact assessment in LCA. However, the geographical region where an emission takes place is in
general already provided by current life cycle inventory analysis or available from the scope definition of the system, since this data is needed to calculate the interventions from transport. More difficult to
obtain in LCA is source height and population density near the source as needed for human toxicity (see Chapter 7). Chapter 7 therefore suggests following a default approach unless there are good reasons
to deviate.
A practical way to avoid needless data gathering is to first perform a site-generic assessment (i.e. without spatial differentiation), and then to retrospectively apply the site-dependent characterisation factors
to the processes dominating the considered impact category. In this way, it will probably become clear soon what the dominating processes are for which additional data is required. Such procedure is
described in more detail in the guidance document from Hauschild and Potting (2003). These references also provide site-generic characterisation factors (plus their standard deviation). The site-generic
factor is based on the spatially differentiated ones. The site-generic factors can be based on the European average, or follow a worst-case approach by taking the highest characterisation factor for the
European area. Potting and Hauschild (2003) choose to work with the European average.
The level of sophistication in spatial variation is not fixed in LCA. As discussed in By Potting et al. (1999), LCA studies differ in the extent to which data for spatial differentiation are available. Some studies
provide a lot of information, whereas other studies cover mainly processes at unknown locations and unknown calendar performance time. Site-generic factors can be used where spatial differentiation is not
possible or desired. If studies provide enough data on the other hand, the use of site-dependent characterisation factors can be replaced by a site-specific assessment with the same models as underlying the
site-dependent factor. Such site-specific modelling in a life cycle approach is for instance applied in ExternE (Krewit et al. 1998, Rabl et al. 1996).
As discussed in Section 2.2, the large impact area of an emission leads to the effect that the dispersion patterns and impact area will only start to deviate considerably when sources are located at larger
distances from each other. In a typical LCA, the precise location of a source is therefore of less importance to quantify total impact from a process. However, some applications might need the detailed
information from a site-specific impact assessment (see also Section 2.6).
A "site-dependent" assessment thus has a level of detail somewhere in between "site-specific" and "site-generic" assessment (the two extremes stretching up a continuum):

All levels of detail may be applied in a particular LCA, though the results at one level should be consistent with the results at another. Each level should preferably take its basis in models similar to the ones
underlying the other levels, and the results at each level should preferably point in similar direction as the results at the other levels. This allows a flexible shifting from one to another level of detail, and
warrants the credibility of doing so. The site-dependent characterisation factors in this technical report can be used to arrive at site-generic factors, or be replaced by site-specific impact assessment based on
the same underlying models. This improves the relevance of the established characterisation factors.
2.6 LCA in relation to RA and EIA
In the discussion about spatial differentiation, that is indirectly relevant for this Technical Report, LCA is typically positioned as distinct from risk assessment (RA) and environmental impact assessment (EIA)
due to the apparent misconception that these tools are always local in their perspective. RA and EIA cover a multiplicity of analytical tools that can be quite different in their object and designs. Both EIA and
RA can refer to a study of consequences for the environment at varying spatial and temporal scales. Some of the concerns addressed may be local and others regional, possibly all within the same study.
Though integrated assessments were incidentally already performed earlier, RA and EIA increasingly tend to involve studies that cover multiple sources.
Analytical tools used in EIA and RA can be generic in the way they consider source and receptor characteristics (as for examples EUSES and CalTOX), but typically they operate with a high degree of
spatial and temporal differentiation in source and receptor characteristics. The detail in source characteristics is necessary because concentration increases and probabilities of threshold exceedance,
particularly close to the source, are highly influenced by the height of release and the temporal variation in the emission. Those characteristics become less dominant at longer distances (see also Section 2.2).
The common element in all types of EIA and RA is that they assess risks, often by employing a threshold or quantification of distance from the threshold.
As discussed by Potting et al. (1997), LCA theoretically has no limitations to take into account spatial or temporal information and neither to perform threshold evaluation. The other way round, generic EIA
and RA also do not take into account spatial and temporal differentiation. EIA and RA can also refrain from threshold evaluation. The differences between LCA, and RA and EIA are thus more given by
current practice than based on fundamental incompatibilities. Basically, spatially differentiated RA and EIA approaches, including quantification of threshold exceedance, are used to establish the
site-dependent characterisation factors in this technical report.
Whereas EIA and RA can be both local and regional in their perspective, LCA is poor in accounting for the contribution of a source to local impact in those situations where concentration increases and the
subsequent probability of threshold exceedance are largely determined by that source alone. Concentration increases and probabilities of threshold exceedance are particularly within the first hundreds to
thousands meters highly influenced by source characteristics as height of release and temporal variation in the emission. In a typical LCA, this information is only limited available and it will often complicate
the analysis to gather additional data.
Local impact has often a relatively small share in the full impact of a source. Where the local share is not that small, it is often not just one source in itself that creates ambient concentrations above threshold
(see also Section 2.2). The effective implementation of measures to regulate the main sources in many industrialised countries has led to a situation in which risks from those sources on their local environment
are usually under control. This will of course not always be the case, and particularly not in developing countries where environmental policy is still limited. However, EIA or RA rather than LCA will be the
obvious tool to support decision-making to bring under control the impact from sources on their local environment.
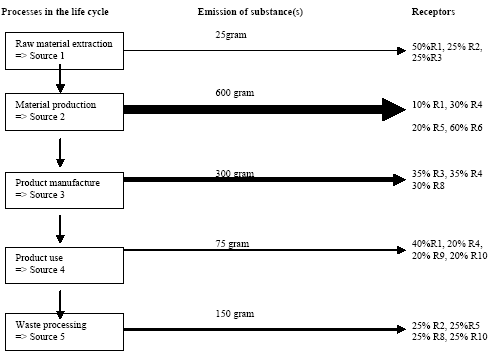
Figure 2.1. The amount of substance(s) emitted from a hypothetical product system and the share received by different environments after emission dispersion.
Typical LCA is optimised in another direction than EIA and RA. LCA is an obvious tool to support policy-making directed towards prevention and control of environmental impact resulting from a
multiplicity of sources, and where each source impacts on a multiplicity of receptors. A product system consists of a collection of processes (or sources) that are functionally interconnected by the output of
one process being the input of another one (see Figure 2.1). A product system functions within a larger economical system of also interconnected processes to which the product system only contributes
marginally (in the same way as it only contributes marginally to environmental impact). LCA provides the possibility to prioritise in the sub-system of a product, those processes that make the largest
contribution to environmental impact taking into account feedback to other processes and other environmental problems within that product system. It is a tool for source comparison in the context of specific
products.
The ability to identify the consequences from the changes in one process for other processes, or from the changes in one impact category for other categories is the strength of LCA above EIA and RA. EIA
and RA are each optimised in their own directions. They supplement each other and may be used in combination to make solid and informed decision-making. White et al. (1995) provide an interesting
overview and discussion of the possibilities and limitations of the different tools in overall environmental management.
2.7 Threshold exceedance in LCA
Where possible, the characterisation factors in this Technical Report take into account the exceedance of thresholds. The discussion whether to perform evaluation of threshold exceedance in LCA is topical
for already quite some years now. Unfortunately, the discussion seems to get stuck in a controversy about "less is better" versus "only above threshold" (see also Potting et al. 1999).
The impact assessment phase in LCA is relatively young and emerged from the wish to simplify the large amount of data from inventory analysis by aggregation into a manageable amount of impact data.
Characterisation factors for most impact categories initially allowed rather simple equivalency assessment on the basis of intrinsic substance characteristics like the potential to release hydrogen ions
(acidification assessment) or toxic effect-levels (toxicity assessment) [11]. Assessment of threshold exceedance (i.e. PEC/PNEC 1) was not performed since the available data did not allow such evaluation.
Threshold information, usually in the form of a no-effect-level, was used in toxicity assessment only to express the emission of a given substance as a dilution volume of the receiving environment. The basis of
equivalency was thus taken in the toxicity potential of each substance. The impact from an emission quantity equal to the no-effect-level was given an impact of one [12], and the impact from any deviating
quantity as the ratio of the emission quantity divided by the no-effect-level.
Present toxicity factors now also cover fate and exposure modelling, but aggregation of the calculated exposure increases from different substances is still often based on no-effect-levels (PEC/PNEC).
Evaluation of threshold exceedance is typically not covered, however, and neither possible with the modelling underlying those toxicity factors.
Though evaluation of threshold exceedance was initially not performed due to lack of data, it has meanwhile turned for many practitioners into a principle in itself that is justified by the reasoning that "less
pollution is better". An important understanding from the present technical report work is that limiting the threshold discussion into "less pollution is better" versus "only above threshold" is too simple and
does not respect the analytical potential of LCA. An example from acidification may clarify this.
Let's assume that we have three ecosystems receiving similar quantities of acidifying substance from our functional unit (the contribution of the functional unit is marginal compared to the background load on
each ecosystem). Let's further assume that these ecosystems have a priori equal tolerance for acidifying loading (i.e. similar critical loads), but that they are at different levels of background loading:
- A Scottish ecosystem with a background load at its critical load,
- A Scandinavian ecosystem with a background load far below its critical load, and
- A German ecosystem with a background load far above its critical load.
We want to prioritise for which ecosystem we like to reduce the full acidifying load (background load + marginal increase from our functional unit).
The sensitivity of ecosystems, as expressed by their critical loads, does not play any role in yet typical "less is better" acidification factors. However, Heijungs and Huijbregts (1998) propose to establish
acidification factors similar as the ones Guinée et al. (1996) established for human toxicity. Such factors express the contribution to the acidifying load of an ecosystem per unit of emission, divided by the
critical load for that ecosystem (ΔPAL/CL) [13]. The a priori tolerance of the ecosystems is taken into account, but the background loading does not play a role here. Since all ecosystems have the same
critical load, this would result in a similar acidification impact from our functional unit to each of these ecosystems.
The practical implication is that the Scottish ecosystem exposed around the critical load is regarded equally important as the German ecosystem exposed far above the critical load (and thus difficult to
rescue), and equally important as the Scandinavian ecosystem exposed far below the critical load (and thus hardly in danger). Whether these ecosystems are exposed far below or at or far above their critical
loads, they are all characterised as being equally vulnerable in such a "less is better" approach. That might be or might not be a justified choice, but it is at least important to realise that such choice is made by
following a "less is better" approach.
An "only above threshold" approach as proposed by Pleijel et al. (not published) and followed by Lindfors et al. (1998) typically assess impact only for those ecosystems already exposed above their critical
loads (the impact of ecosystems exposed below their critical load is assessed zero). The a priori tolerance of ecosystems and their background loading are taken into account to distinguish ecosystems
exposed below from those exposed above their critical load. After the ecosystems are classified, however, the background loading and critical loads do not longer play a role. Whether ecosystems are
exposed far above or just above their critical loads, they are all characterised as being equally vulnerable in this approach. Equal priority would be given here to the German and Scottish ecosystem (assumed
that the latter is just above its critical load).
The characterisation factors of Lindfors et al. (1998) can be adjusted by multiplying the change in exceedance with a severity factor given by the existing background load divided by the critical load for the
given ecosystem (Posch 1998). Such sophisticated way to account for background load and a priori tolerance of ecosystems is presaged by Pleijel et al. (1997).
The acidification factors in Chapter 4 can be typified as following an "only around threshold approach). This approach takes into account differences between ecosystem in background levels and their a
priori tolerance for acidifying loading by basing the characterisation factors on the slope of the curvilinear dose-effect curve. This curve is defined by the critical loads of all ecosystems to which one source
contributes. The practical implication is that ecosystems with depositions far above or below their critical loads are not expressed in the characterisation factor. In the above example, this approach would
prioritise the Scottish ecosystem (just above its critical load and therefore easy to rescue) rather than the Scandinavian ecosystem or the German ecosystem.
In the above example, the value choices underlying the different approaches were illustrated with help of single ecosystems. The emission per functional unit from a given process does usually not contribute
to only one ecosystem but to a multiplicity of ecosystems, each with their own critical loads and background loads. Assessing the impact of a process to acidification thus basically involves adding together
the dissimilar contributions from one process to a multiplicity of heterogeneous ecosystems (see Formula 2.1 in Section 2.1).
Several other possibilities are available to deal with both differences in a priori tolerance for acidifying loading and background loading. The choice for any approach remains in the end a matter of how to
value ecosystems with different critical loads as well as different background loads. Some approaches seem more obvious than others. A systematic, quantitative comparison of the actual consequences on
the final characterisation factors of each approach would be a helpful input to the discussion about how to deal with thresholds in LCA.
The example from acidification applies to all impact categories, and theoretically also to human and eco-toxicity assessment. Although now also EIA and RA have made a start in integrated assessments of
human toxicity, however, these models will in practice remain to be less sophisticated than for other impact categories due to the complexity of toxicity assessment. The complexity of toxicity assessment
arises from the large number of toxic substances, whereas impact categories as global warming and acidification are determined by a limited number of substances and specific impact mechanisms.
2.8 Site-dependent normalisation
Normalisation is an optional step in impact assessment that calculates the assessed impact relative to a selected reference value. This reference value typically refers to impact of the total emission of a
reference area (possibly on a per capita basis) (ISO 14042 2000).
Different normalisation methods exist. Lindeijer et al. (1996) provides an interesting review in which they discuss some basic issues in normalisation methodology. Thinking about a spatially differentiated
normalisation has put another fundamental issue forward. This issue has probably not been discussed before in the literature, but addresses the question whether normalisation should be based on marginal or
average impacts. This question is relevant for all normalisation methodologies (and is also not exclusively related to spatial differentiation), but will here be illustrated with the methodology proposed by
Wenzel et al. (1997) and Hauschild and Wenzel (1998).
Impact categories relate to different spatial scales. Wenzel et al. (1997) and Hauschild and Wenzel (1998) propose to base normalisation on the spatial scale typical for each impact category (that is, the
normalisation factor should reflect the level of impact experienced in that region). The global impact level is taken for global impacts as ozone depletion, while for practical reasons the impact level in
Denmark is taken for the non-global categories (though the spatial scale of these impact categories can be smaller as well as larger than Denmark). The global impacts will obviously be larger than the
regional ones, since more people and larger economic activity are involved. In order to establish a "common scale", the impact in the region defined by the spatial scale of the given impact is divided by the
population in that area. This results in the normalisation factors being the impact per inhabitant of Denmark for the non-global categories, and the impact per global citizen for global impacts.
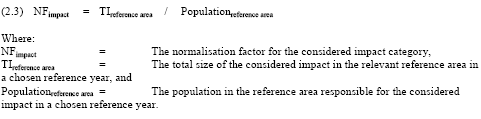
The methodology of Wenzel et al. (1997) and Hauschild and Wenzel (1998) is unique in their use of different reference areas across impact categories. Other methodologies may differ in the chosen
reference area, but each methodology usually operates with the same reference area for all impact categories. It is here that normalisation on the basis of marginal or average methodology comes most clearly
in sight.
The question whether LCA should take a marginal or an average approach was first discussed in the context of inventory analysis. Though being topical for several years, the discussion seems to move to a
consensus. Average methodology is seen as connected to LCAs that describe an existing or historical situation (e.g. for environmental reporting or declaration). Marginal methodology is required for LCA's
that analyse the consequences from changes in a product system. (Weidema 1998)
A marginal normalisation methodology would consider the impact over the full European area that is affected by all emissions of a society, compared to the impact over that area without the emissions from
that society (but against the unchanged background of the emissions of other regions). Table 3.2 and 3.3 provide such normalisation factors per country for acidification (see the column "unprotected
ecosystem by region"). For acidification, this would for instance mean the impact in the total European area caused by emissions from Denmark and all other regions, compared to the impact in the total
European area without the Danish emissions (but with the emissions of all other regions). This increase of impact caused by all Danish emissions divided by the total population in Denmark gives the impact
caused per capita [14]:
Click here to see the Figure.
The marginal normalisation factor for the European area could be taken as the average of the marginal normalisation factors for all regions weighed by the emission of each region. Another possibility is to
take the average normalisation factor for Europe.
A normalisation methodology consistent with Wenzel et al. (1997) and Hauschild and Wenzel (1998) would probably follow an average approach by taking the total impact in an area divided by the
population in that same area. The impact area is "defined" by the total area over which an impact more or less clearly extends [15]. For acidification, this would mean for instance the total acidified area in
Europe divided by total European population:
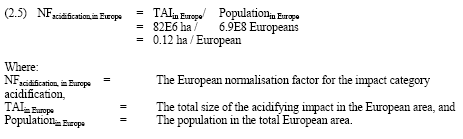
It does not make sense to calculate an average normalisation factor for an area smaller than the European area (that is for separate countries). The average normalisation factor for Denmark, for instance,
would be the area affected within Denmark divided by Danish population. However, the total impact within Denmark is caused by the emission of all regions and not by the emission from Denmark alone.
The meaning of such an average Danish normalisation factor is therefore not very transparant.
An average approach as from Wenzel et al. (1997) and Hauschild and Wenzel (1998) puts the emphasis on the impact itself, rather than on the society causing this impact. A possible consequence of such
an approach could be to apply several normalisation factors for non-global impact categories. The area where a process takes place gives the relevant normalisation factor. For example, a "European"
normalisation factor should be used for impact from acidifying emissions in Europe, whereas the impact from emissions in North America should be normalised with a "North American" factor. The number
of required normalisation factors would however become rather large for impact categories that relate to a much smaller than the regional scale, which is unpractical. Furthermore, the set of normalisation
factors required for a product system is no longer similar with the set for its alternative. This makes the results of this normalisation step relatively difficult to interpret.
Normalisation methodology based on a marginal approach could also choose to operate with several normalisation factors per impact category. However, it is more obvious to work with only one
normalisation factor, typically based on the impact from the region where the product is marketed. A relevant question is then whether such normalisation factor should be based on the economic activity of
the selected region, or on its consumption. The factors in Table 3.2 and 3.3 relate in this context to the first option, but may be seen, with some caution, as the best estimate of the second option for the time
being. Normalisation factors based on consumption should account for the net import and export of impact by the selected region. (Blonk et al. 1997, Lindeijer et al. 1996)
As Lindeijer et al. (1996) emphasised, the choice for one or another normalisation method depends amongst others on the following valuation step. It goes beyond the scope of this technical report to further
enter into the subject of site-dependent normalisation in the context of valuation, but clearly a better understanding of the aims of valuation, and normalisation in relation to spatial differentiation is needed. The
site-dependent normalisation factors for the guidance document follow the method as developed by Wenzel et al. (1998).
2.9 Temporal differentiation in LCA
Temporal differentiation is an important and intensively discussed issue in LCA. The discussion has focused for a long time predominantly on the lacking time dimension in the inventory data (i.e. whether it is
a flux or a pulse). As argued in Section 2.2, the temporal variation of the small (or even marginal) contributions from a single source emission to exposure of their multiple receptors will to a large extent be
cancelled out against the very high background exposure from all sources together. The multiple source, multiple receptor perspective of present environmental problems make the lacking time dimension in
inventory data to a less dramatic problem (see also Section 2.2).
The calendar time to which the different processes in a product system relate is a more important issue when it comes to impact assessment in LCA. The calendar time of a process determines the
(estimated) total economic activity with all its emissions being responsible for the total environmenta.l load causing an impact to which background that process adds. The background situation can be rather
different between calendar times (and thus between different processes in a product system as discussed below). This could result in rather different factors related to these different calendar times (since the
factors do account for the - differing - background situation). The photochemical ozone creation potential from an emission of a volatile organic compound, may be different in 1990 and 2010 due to
considerable differences in the background concentration levels of nitrogen oxides posed by the total economy in those years. For instance, the acidification factors from Chapter 3, which are calculated for
the reference years 1990 and 2010, show that the difference between different calendar times can be notable.
A product system can easily cover a time frame of several decades depending on time-of-use of the product and the time needed for each subsequent process in the product system. For example, a linoleum
floor covering will on average first be discarded 15 years after it has been bought (Potting and Blok, 1995). The characterisation factors used to assess the impact from a given process should relate to the
calendar time in which that process takes place (a time-dependent characterisation factor).
As a matter of fact, also processes themselves can cover a time frame of several years to several decades (and indeed for landfill processes centuries of millennia). A specific type of linoleum will be
produced over a certain time interval before the type is taken from the market. This determines the time or calendar interval over which that type is marketed, used, and disposed. Basically, the
characterisation factor used to assess the impact from given processes should thus not relate to the calendar time, but to the calendar interval in which a given process takes place.
Similar to spatial differentiation, the level of temporal differentiation can be seen as placed on a continuum stretched up by the two extremes "time-specific" and "time-generic". Time-dependent" assessment
thus has a level of detail somewhere between those extremes:

An interesting question and subject in need of further research is the level of temporal differentiation needed in LCA. Without going into details, trend analyses show emission projections to be relatively
stable over a couple of years (Jol and Kielland 1997). This suggests a time-dependent assessment based on time-intervals of several years to be adequate for LCA. However, the issue was not further
elaborated in the work for this Technical Report.
2.10 References
Blonk, H., M. Lafleur, R. Spriensma, S. Stevens, M. Goedkoop, A. Agterberg, B. van Engelenburg, and K. Blok. Three reference levels for normalisation in LCA: Dutch territory 1993/1994, Dutch final
consumption 1993/1994, West European territory in the beginning nineties (in Dutch). The Hague (the Netherlands), Ministry of Traffic and Water affairs and Ministry of Housing, Spatial Planning and
Environ.ment (VROM), 1997.
Braunschweig, A., R. Förster, P. Hofstetter and R. Müller-Wenk. Developments in LCA valuation (ISBN 3-906502-31-7). St. Gallen (Switzerland), Institut für Wirtschaft und Ökologie, 1996.
EEA. Europe's environment: The second assessment. Copenhagen (Denmark), European Environmental Agency (EEA), 1998.
Goedkoop, M., P. Hofstetter, R. Müller-Wenk, R. Spriensma. The Eco-Indicator 98 explained. Int. Journal of Life Cycle Assessment, Volume 3 (1998), Issue 6, pp352-360.
Guinée, J., R. Heijungs, L. Van Oers, D. Van de Meent, T. Vermeire, M. Rikken. LCA impact assessment of toxic releases. Generic modelling of fate, exposure and effect for ecosystems and human beings
with data for 100 chemicals. Leiden (the Netherlands), Centre of Environmental Science of Leiden University, 1996.
Hauschild, M. and J. Potting. Spatial differentiation in life cycle impact assessment – the EDIP2003 methodology. Guideline from the Danish Environmental Protection Agency, Copenhagen, 2003.
Hauschild, M.Z. and Wenzel, H.: Environmental assessment of products. Vol. 2 - Scientific background, 565 pp. Chapman & Hall, United Kingdom, 1998, Kluwer Academic Publishers, Hingham, MA.
USA. ISBN 0412 80810 2
Heijungs, R. and M. Huijbregts. Threshold-based life cycle impact assessment and marginal change: incompatible? (CML-SSP Working paper 99.002). Leiden (the Netherlands), Centre of Environmental
Science of Leiden University, 1999.
Heijungs and Wegener Sleeswijk. The structure of impact assessment: Mutually independent dimensions as a function of modifiers. Int. Journal of Life Cycle Assessment, Vol. 4 (1999), Issue 1, pp2-3.
Hofstetter, P. Perspectives in life cycle impact assessment. A structured approach to combine models of the technosphere, ecosphere and valuesphere. Boston (United States of America), Kluwer Academic
Publishers, 1998.
ISO 14042. Environmental management - life cycle assessment – life cycle impact assessment. International Organisation for Standardisation (ISO), 2000.
Jol, A. and G. Kielland. Air pollution in Europe 1997. Copenhagen (Denmark), European Environmental Agency (EEA), 1996.
Krewit, W., P. Mayerhofer, A. Friedrich, A. Trukenmüller, and R. Friedrich. Application of the impact pathway analysis in the context of LCA. Int. Journal of Life Cycle Assessment, Vol. 3 (1998), Issue 2,
pp86-94.
Lindeijer, E., J. Assies, M. Bovy, A. Braunschweig, G. Finnveden, R. Förster, R. Heijungs, P. Hofstetter, A-M Jørgensen, R. Müller-Wenk, B. Stahl,, H.A. Udo de Haes, R. Walz, B.P. Weidema, B.
Wilson and N. Wrisberg. Normalisation and valuation. In: Udo de Haes, H.A. (ed.). Towards a methodology for life cycle impact assessment. Brussels (Belgium), Society of Environmental Toxicology and
Chemistry – Europe, 1996.
Lindfors, LG., M. Alkemark, C. Oscarsson and C. Spännar. A manual for the calculation of ecoprofiles intended for third party certified environmental product performance declarations. Stockholm
(Sweden), Swedish Environmental Institute (IVL), 1998.
Pleijel, K., J. Altenstedt, H. Pleijel, G. Lövblad, P. Grennfelt, L. Zetterberg, J. Fejes and L-G. Lindfors. A tentative methodology for the calculation of global and regional impact indicators in type-III
ecolabels used in Swedish case studies. Stockholm (Sweden), Swedish Environmental Institute (IVL), not published.
Potting, J. and K. Blok. Life cycle assessment of four types of floor covering. Journal of Cleaner Production, Vol.3 (1995), Issue 4, pp201-213.
Potting, J. and M. Hauschild. Predicted environmental impact and expected occurrence of actual impact. Part 1: The linear nature of environmental impact from emissions in life-cycle impact assessment. Int.
Journal of Life Cycle Assessment, Vol. 2 (1997a), Issue 3, pp171-177.
Potting, J. and M. Hauschild. Predicted environmental impact and expected occurrence of actual impact. Part 2: Spatial differentiation in life cycle assessment via de site-dependent characterisation of
environmental impact from emissions. Int. Journal of Life Cycle Assessment, Vol. 2 (1997b), Issue 4, pp209-216.
Potting, J. and M. Hauschild. Levels of sophistication in life cycle impact assessment of acidification. Points of discussion additional to the presentation. In: Bare, J.C., H.A. Udo de Haes and D.W.
Pennington (eds.). International workshop on life cycle impact assessment sophistication. Bayreuth (Germany), Eco-Informa Press, in press.
Rabl, A. and J.V. Spadaro. Estimates of real damage from air pollution: Site dependency and simple impact indices for LCA. Contribution to the 8th annual meeting of SETAC-Europe, 14-18 April 1998 in
Bourdeaux, France. Paris (France), Ecole de Mines de Paris, 1996.
Udo de Haes, H.A. (ed.). Towards a methodology for life cycle impact assessment. Brussels (Belgium), Society of Environmental Toxicology and Chemistry – Europe, 1996.
Udo de Haes, H.A. and G. Huppes. Positioning of LCA in relation to other environmental decision support tools. In: Udo de Haes, H.A., A.A. Jensen, W. Klöpffer and L-G. Lindfors (eds). Integrating
impact assessment into LCA.Proceedings of the LCA symposium held at the fourth SETAC-Europe Congress, 11-14 April 1994 at the Free University of Brussels in Belgium. Brussels (Belgium), Society
of Environmental Toxicology and Chemistry – Europe, 1994.
Udo de Haes, H.A., O. Jolliet, G. Finnveden, M. Hauschild, W. Krewit and R. Mueller-Wenk (eds.). Best available practice regarding impact categories and category indicators in life cycle impact
assessment. Background document for the second working group on life cycle impact assessment of SETAC-Europe. Part 1: Int. Journal of Life Cycle Assessment, Vol. 4 (1999), Issue 2, pp66-74. Part 2:
Int. Journal of Life Cycle Assessment, Vol. 4 (1999), Issue 3, pp167-174.
Wegener Sleeswijk, A. Regionalisation and fate analysis in LCA. Their relationship and implications (abstract). In: Interfaces in Environmental chemistry and toxicology from the global to the molecular level.
Abstract book for the 8th annual meeting of SETAC-Europe, 14-18 April 1998 in Bordeaux (France). Brussels (Belgium), Society of Environmental Toxicology and Chemistry, 1998.
Weidema, B. Application typologies for life cycle assessment. Int. Journal of Life Cycle Assessment, Volume 3 (1998), Issue 4, pp237-240.
Wenzel, H., Hauschild M.Z. and Alting, L.: Environmental assessment of products. Vol. 1 - Methodology, tools, techniques and case studies, 544 pp. Chapman & Hall, United Kingdom, 1997, Kluwer
Academic Publishers, Hingham, MA. USA. ISBN 0 412 80800 5.
White, P., B. De Smet, J.W. Owens, P Hindle. Environmental management in an international consumer goods company. Resources, conservation and recycling, Vol. 1995, Issue 14, p171-184.
Fodnoter
[6] Institute of Product Development (IPU) in Denmark until 2000, presently at the Center for Energy and Environmental Studies IVEM, University of Groningen
[7] Institute of Product Development (IPU) in Denmark
[8] This can also be deduced from Chapter 5. While benzene has less than 10% of its impact within the first 10km from the source, a relatively short-lived substance as hydrogen chloride has about 40%
within the first 10km and almost 80% within the first 75km. However, at a moderate release height of 25m this short-lived substance will still have less than 10% of its impact within the first hundred metres
from the source where the peak concentration occurs. Also a short-lived substance thus has most of its impact at longer than very local distance. This makes a site-dependent approach achievable also for
these substances, though a higher spatial resolution is needed and the uncertainties will be larger (as also appears from Chapter 5).
[9] The term "exposure" covers the same as the term "target" in Chapter 4, but has been preferred here to draw the connection with publications of Heijungs and Wegener Sleeswijk (1999) and Udo de
Haes et al. (1999) that follow a similar line of reasoning.
[10] The exposure increase in any receptor resulting from an emission is usually small or marginal compared to the background exposure in that receptor. The background exposure therefore determines
whether a threshold value will be exceeded by an exposure increase. It is an ongoing discussion whether threshold exceedance should be accounted for in LCA.
[11] No-effect-levels are based on experiments on test-species under laboratory conditions and therefore say something about the intrinsic substance characteristic to cause toxic effect (rather than
something about the sensitivity of a species in real-life for this toxic substance).
[12] The underlying assumption is that the toxicity impact from a quantity at the no-effect-level of one substance has the same importance as the toxicity impact from a quantity at the no-effect-level of
another substance. To put it more clearly: If the quantities of both substances are at their no-effect-level, the impacts from a neuro-toxic substance and a skin irritating substance are regarded as equally
important. The adding together of very different effect types is one of the more serious problems in LCA, but has not been further addressed in this dissertation.
[13] "PAL" stands for predicted acidifying load (similar as to predicted environmental concentration in toxicity assessment).
[14] Hence the impact area and the territory of the selected population are not the same. This is the logic consequence of Danish emissions having transboundary impact.
[15] Actually, the definition of such impact area is not without problems. It is not really possible to isolate an area over which an impact extends, from an area in which this impact is not at stake. This creates
arbitrariness in the normalisation factor.
| Front page | | Contents | | Previous | | Next | | Top |
Version 1.0 April 2005, © Danish Environmental Protection Agency
|