| Front page | | Contents | | Previous | | Next |
Appendices 1-18 to: Report on the Health Effects of Selected Pesticide Coformulants
81 Toxicokinetics
81.1 Absorption, distribution, excretion
81.1.1 Inhalation
81.1.2 Oral intake
81.1.3 Dermal contact
81.2 Elimination
81.3 Mode of action
81.1 Absorption, distribution, excretion
81.1.1 Inhalation
When seven male volunteers were exposed to EGBE (20 ppm (98 mg/m3)) for 2 hours during light physical exercise (bicycle ergometer), the respiratory uptake of EGBE averaged 1.2 mg/minute (57% of the inspired amount). The concentration of EGBE in blood reached a plateau level of 0.9 mg/l within 1-2 hours and was no longer detectable 2-4 hours after the end of exposure. The apparent values of elimination half-time, mean residence time, total blood clearance, and steady-state volume of distribution were 40 minutes, 42 minutes, 1.2 l/minute, and 54 l, respectively, for EGBE. The half-life for EGBE in urine was 1.36 hours. The amount of EGBE excreted in urine was less than 0.03% of the total uptake, while that of 2-butoxyacetic acid (2-BAA) ranged from 15-55%. Relative to the uptake of EGBE, the excretion averaged 41% on an equimolar basis. (Johanson et al. 1986 – quoted from ATSDR 1998, ECETOC 1995b).
In a similar study in five healthy male volunteers, the minimum and maximum observed concentrations of 2-BAA in blood ranged from 2.4 to 6.7 mg/l; the blood level peaked after 2-4 hours. (Johanson & Johanson 1991 – quoted from ATSDR 1998).
Healthy male volunteers (four individuals) were exposed to 50 ppm (245 mg/m3) EGBE vapour for 2 hours by mouth (through a respiratory valve). The concentration of EGBE increased during the first hour and appeared to approach steady state at about 0.35 mg/l at the second hour. The respiratory uptake rate was 1.3 mg/minute. (Johanson & Boman 1991 – quoted from ATSDR 1998).
When rats (three to five animals per group) were exposed (nose-only) to 4.3, 49, or 438 ppm (21, 240, or 2150 mg/m3) [14C]EGBE for 6 hours, the respiratory uptake was 531, 541, and 423 mikrogram/ppm at 4.3, 49, and 439 ppm, respectively. A less-than-proportional amount of EGBE was, according to ATSDR, inhaled at the high dose because of decreased minute volume. 2-BAA was the major metabolite of EGBE in plasma. Ratios of ethylene glycol to 2-BAA in plasma were higher than those in urine. The majority of the inhaled [14C]EGBE was eliminated in the urine, with free 2-BAA being the major urinary metabolite, accompanied by lesser amounts of ethylene glycol and EGBE glucuronide. A small proportion (5-8%) of the retained EGBE was exhales as 14C-carbon dioxide. Faeces accounted for 1-2% of the absorbed dose. At 4.3 ppm, about 60% of the urinary radioactivity was excreted during the 6-hour exposure, whereas at 438 ppm, only 10% was excreted during the exposure period. The production of carbon dioxide most closely followed the production of ethylene glycol and may represent the metabolism of this metabolite. (Sabourin et al. 1992 – quoted from ATSDR 1998).
In another study in rats, eight male Sprague-Dawley rats were exposed to 20 or 100 ppm (98 or 490 mg/m3) EGBE 24 hours per day for 1, 2, 3, 4, 6, 8, 10, or 12 days. The concentrations of EGBE and 2-BAA in blood, muscle, liver, and testis increased rapidly during the first 1-3 days of exposure and continued to increase, but more slowly, during the remaining days. The average peak concentration of EGBE in the blood was 1.8 mg/l in the 20-ppm group and 8.5 mg/l in the 100-ppm group. Respiratory uptake averaged 37 mg/day at 20 ppm and 186 mg/day at 100 ppm. The urinary excretion rate for 2-BAA in the 20-ppm group averaged 24 mg/day, whereas the 100-ppm group excreted 122 mg/day; the observed urinary excretion rate corresponded to 64% of the calculated respiratory uptake and renal clearance of 0.53 l/hour for both groups. (Johanson 1994 – quoted from ATSDR 1998).
81.1.2 Oral intake
Case reports of intentional poisonings with EGBE indicate that EGBE is absorbed in humans following oral exposure.
When male F-344 rats were given a single oral dose of [14C]EGBE at 125 or 500 mg/kg, detectable exhalation of radioactivity began 1-2 hours after treatment indicating rapid absorption and distribution. Approximately 18 or 10% of the respective doses was exhaled as 14C-carbon dioxide in 48 hours. Cumulative urinary excretion of radioactivity amounted to approximately 32 or 18% of the respective doses at 8 hours, to approximately 64 or 30% at 24 hours, and to approximately 70 or 40% at 48 hours. Faecal excretion of radioactivity amounted to 2-3% of the doses in 48 hours. Thus, by adding the 48-hour excretion of radioactivity in the expired air and urine, at least 88% of the 125 mg/kg dose and at least 50% of the 500 mg/kg dose was absorbed. According to ATSDR, the fact that the percentage of radioactivity excreted was less at the higher dose than at the lower dose may indicate saturation of a metabolic pathway rather than lower absorption of the high dose.
EGBE was distributed to all tissues, with the highest levels (determined 48 hours after dosing) detected in the forestomach. The increase in the tissue concentration was not proportional to the increase in dose. While the ratio of the administered doses was 1:4, the ration of the tissue concentration in most tissues was greater than 1:10. According to ATSDR, this may be related to saturation of EGBE metabolism at the high dose.
The major route of elimination was the urine (40-70%), followed by exhalation (11-18%). A small portion was excreted in the bile (8% at the 500-mg/kg dose) 8 hours after dosing, and a small amount was detected in the faeces (2-3%).
(Ghanayem et al. 1987 – quoted from ATSDR 1998).
In male F-344 rats given single gavage doses of 8.6 or 126 mg/kg [14C]EGBE, 59% of the low dose of radioactivity and 38-70% of the high dose of radioactivity was excreted in the urine during the first 24 hours. Excretion of 14C-carbon dioxide in the expired air amounted to 7.2 or 8%, respectively, in 24 hours. Thus, at least 66% of the low dose and 46-78% of the high dose was absorbed. (Corley et al. 1994 – quoted from ATSDR 1998).
Male F-344 rats were administered [14C]EGBE in the drinking water at concentrations of 290, 860, or 2590 ppm (corresponding to 28, 47, or 141 mg/kg b.w./day according to ATSDR) for 24 hours. The majority of the radioactivity was excreted in urine (50-60% as 2-BAA, 10% as ethylene glycol) or exhaled as 14C-carbon dioxide (8-10% of the dose). Less than 5% of the dose was exhaled as unmetabolised EGBE. Ethylene glycol was excreted in urine, representing approximately 10% of the dose of EGBE. Elimination in the faeces was a minor pathway (1.6-2.8% of the dose). Unmetabolised EGBE and its glucuronide conjugate were also identified in the urine. The maximum excretion occurred during the first 12-24 hours after the start of exposure. (Medinsky et al. 1990 – quoted from ATSDR 1998).
No quantitative or qualitative alterations of metabolism or disposition were observed in rats by repeated exposure to EGBE as compared to treatment with a single dose. Elimination of 14C-carbon dioxide and EGBE-derived radioactivity in the urine of rats receiving multiple doses of EGBE was essentially the same as for rats receiving a single dose. The ratios of free 2-BAA, the EGBE-glucuronide and the EGBE-sulphate conjugates, and parent EGBE excreted in the urine of rates treated for 4 or 8 days were relatively similar to those from rats treated with a single dose. (Ghanayem et al. 1992 – quoted from ATSDR 1998).
81.1.3 Dermal contact
Dermal absorption of EGBE was evaluated in workers using window-cleaning agents containing EGBE in concentrations ranging from 0.9 to 21.2% in volume (Vincent et al. 1993 – quoted in ATSDR 1998). Positive correlations between exposure levels of EGBE and 2-BAA in the urine indicate dermal (and probably some pulmonary) absorption of EGBE by these workers.
Experimental evidence exists that dermal absorption of EGBE vapours may contribute to the overall absorption of EGBE during vapour exposure (Johanson & Boman 1991 – quoted in ATSDR 1998, EPA 1999, ECETOC 1995b). Healthy male volunteers were exposed to 50 ppm (245 mg/m3) EGBE vapour for 2-hour, followed by 1 hour of no exposure, followed by 2 hours of body-only exposure (exposed in a chamber while breathing fresh air via respirator) to 50 ppm. Capillary blood samples (finger prick, assuming that the finger prick blood samples represented mixed arterial blood) were collected at regular intervals and analysed for EGBE. The average concentration in blood and the calculated rate of uptake of EGBE was about 3-4 times higher during dermal exposure than during inhalation exposure, suggesting that about 75% of the total uptake during normal vapour exposure could be accounted for by dermal absorption.
The results obtained in the above study have been questioned by Corley et al. (1994 – quoted in EPA 1999). They reanalysed the data assuming that the finger prick blood samples represented venous blood draining the skin prior to mixing systemically. These simulations resulted in a dermal absorption contributing no more than 22% of the total uptake of EGBE in a whole-body exposure.
Corley et al. (1997 – quoted in ATSDR 1998, EPA 1999) observed a much lower dermal absorption (<1500 times) of EGBE vapour when blood samples were taken from an unexposed arm compared to blood samples taken from the exposed arm (finger prick sample).
About two-thirds of the 2-BAA excreted in the urine was in the form of the N-butoxyacetyl glutamine conjugate. No free EGBE, free og conjugated ethylene glycol ether, or glycolic acid were detected in the urine. 2-BAA was eliminated in the urine primarily during the first 12-hour collection period.
Dermal uptake has been estimated under worst-case resting conditions (respiration rates at their lowest, no clothing worn) and under more realistic conditions (35% of the body surface area exposed). Under the worst-case conditions, 15-27% of the total uptake of EGBE vapour would be through the skin, while dermal uptake would be 4.5-8.5% of the total if only 25% of the body surface was exposed. Under simulated exercise, uptake was estimated as 4.6-8.7% and 1.2-2.3% of the total assuming 100 or 25% of the body surface area was exposed, respectively. (Corley et al. 1997 – quoted from ATSDR 1998).
Percutaneous absorption of liquid EGBE has been investigated in male volunteers keeping two or four fingers immersed in neat EGBE for 2 hours. The presence of EGBE in blood and 2-BAA in urine confirmed that EGBE enters the systemic circulation. The excretion rate of 2-BAA increased during the first hours after exposure, reached a maximum at about 5 hours, and then declined, with an average half-life of 3.1 hours. Seventeen percent of the absorbed dose was excreted as 2-BAA in 24 hours. (Johanson et al. 1988).
EGBE is also absorbed through animal skin. Percutaneous absorption in rats appears to be 20-30% of the applied dose (Bartnik et al. 1987, Sabourin et al. 1992, 1993 – both quoted in ATSDR 1998).
Only a small amount of the applied dose (0.3-2%) was still present at the application site 72 hours following dosing. The majority of the absorbed dose was excreted in the urine (82-83%); 3-5% was found as carbon dioxide, 3-6% in the faeces, and 3-13% in the carcass. Free 2-BAA was the main metabolite (66-70%), followed by the EGBE-glucuronide (13-15%9, and ethylene glycol (4-6%). (Sabourin et al. 1992, 1993 – quoted in ATSDR 1998).
81.2 Elimination
According to ATSDR, there is no available evidence to suggest that the route of administration has any substantial effect on the subsequent metabolism of EGBE in humans, but data from animals indicate that the route of exposure may make some differences in the relative amounts of metabolites produced.
The proposed metabolic pathway in rats and humans for EGBE is shown in Figure 2.2. EGBE is oxidised in the liver by alcohol dehydrogenase to butoxyacetaldehyde (BAL), which is further oxidised to 2-BAA by acetaldehyde dehydrogenase. In humans, 2-BAA can be conjugated with glycine or glutamine to form N-butoxyacetyl glycine or N-butoxyacetyl glutamine, respectively, or it can be further metabolised (humans, rats) to carbon dioxide. EGBE can also undergo O-dealkylation (by a cytochrome P450 dealkylase CYP2E1) to form ethylene glycol, and butyraldehyde and butanol. In rats, EGBE can also be conjugated with sulphate to form butoxyethanol sulphate, or with glucuronic acid to form the glucuronide. (EPA 1999, ATSDR 1998).
The two main oxidative pathway of EGBE metabolism in rats are alcohol dehydrogenase and O-dealkylation by CYP2E1. EGBE may also form conjugates with glucuronide and sulphate to some extent. Primarily because 2-BAA is excreted in the urine of both rats and humans following exposure to EGBE, it has been suggested that the former pathway would be applicable to both rats and humans. However, the other three proposed metabolic pathways of EGBE may be applicable only to rats as the metabolites of these pathways (ethylene glycol, EGBE-glucuronide, and EGBE-sulphate) have been observed only in the urine of rats and not in the urine of humans. In addition, approximately two-thirds of the 2-BAA formed by humans is conjugated with glutamine and, to a lesser extent, glycine; these 2-BAA conjugation pathways have not been detected in the rat. (Medinsky et al. 1990, Corley et al. 1997, Bartnik et al. 1987, Ghanayem et al. 1987 – quoted from EPA 1999).
81.3 Mode of action
The most characteristic toxic effect of EGBE in experimental animals is on the haematological system and includes elevated osmotic fragility of erythrocytes, haemolysis, decreased haematocrit (Hct), haemoglobinuria, haemoglobinaemia, and haemolytic anaemia. Compensatory erythropoiesis occurring in response to haemolysis results in an increase in reticulocytes, increased mean cell volume, increased or normal mean cell haemoglobin, and decreased mean cell haemoglobin concentrations. Most of the other toxic effects of EGBE observed in animal studies may be secondary to the haemolytic effects. (ATSDR 1998, NTP 2000).
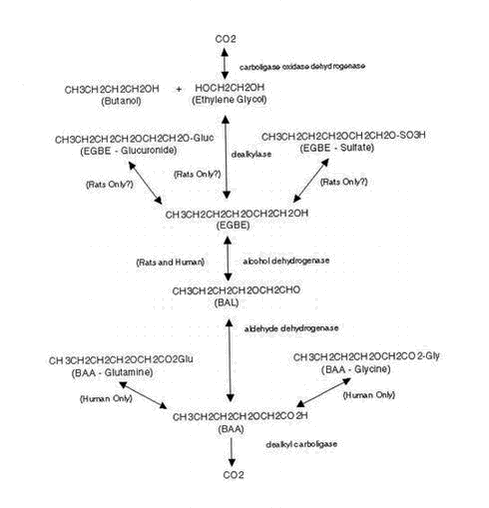
Figure 2.2. Proposed metabolic pathway for EGBE in rats and humans. From EPA (1999 – adapted from Medinsky et al. 1990 and Corley et al. 1997).
The EGBE-induced haemolysis is a direct effect on the erythrocyte membrane causing osmotic fragility and intravascular lysis. Significant species differences have been reported with rats appearing to be particularly sensitive to EGBE induced haemolysis, whereas humans appear to be more resistant to this effect. (ECETOC 1995a, ATSDR 1998, NTP 2000).
The mechanism(s) of action is not fully understood, but the metabolite of EGBE, 2-butoxyacetic acid (2-BAA), shows a significantly greater haemolytic effect in vitro than EGBE (ECETOC 1995a, EPA 1999, ATSDR 1998). Furthermore, studies in rats showed that pyrazole and cyanamide as metabolic inhibitors of alcohol and aldehyde dehydrogenases, respectively, protected or significantly reduced the EGBE-induced haematotoxicity (EPA 1999).
Spermatogenesis is adversely affected by the lower ethylene glycol ethers (EGME, EGEE) and their acetates with EGME being the most potent glycol ether inducing testicular toxicity. There is no evidence however, that higher homologues, as EGBE, are testicular toxicants. (ECETOC 1995a).
The developmental effects of ethylene glycol ethers are mediated by alkoxy acetic acid metabolites. However, ethylene glycol ethers with alkyl chains of more than 2 carbon atoms, as EGBE, do not appear to be selectively toxic to the foetus. For EGBE, foetotoxic effects have been observed only in the presence of maternal toxicity. In an in vitro study, the alkoxy acetic acids methoxyacetic acid (MAA) (from ethylene glycol monomethyl ether (EGME)) and ethoxyacetic acid (EEA) (from ethylene glycol monomethyl ether (EGEE)) showed similar developmental effects, whereas 2-BAA (from EGBE) was only slightly active. (ECETOC 1995a).
| Front page | | Contents | | Previous | | Next | | Top |
|