| Front page | | Contents | | Previous | | Next |
Spatial differentiation in LCA impact assessment
5. Terrestrial eutrophication
Background information for this chapter can be found in:
- Chapter 5 of the "Environmental assessment of products. Volume 2: Scientific background" by Hauschild and Wenzel (1998a).
- Chapter 4 of the "Background for spatial differentiation in life cycle impact assessment – EDIP2003 methodology" by Potting and Hauschild (2005).
5.1 Introduction
Nutrients are essential to ensure (re-)production and subsistence of aquatic and terrestrial systems. Enrichment of ecosystems with nutrients, the literal meaning of the term "eutrophication", is therefore not
harmful until a certain, critical level (critical load) is reached. Each ecosystem and each of its species has its own level of nutrients that relates to optimum growth. Availability of nutrients in excess of this
optimum or critical load leads to a change of the species composition and hence to an unwanted change in the character of the given ecosystem.
5.2 Classification
Normally, biological growth in terrestrial ecosystems is limited by nitrogen. In principle, most compounds containing nitrogen will thus contribute to terrestrial eutrophication, but in practice, Table 5.1 will
cover all emissions in the inventory to be classified as terrestrial eutrophying. For natural soils, atmospheric deposition provides the main man-made supply of nitrogen (and other nutrients).
Free nitrogen (N2) does not contribute to terrestrial eutrophication, even though it is available for certain bacteria and algae. This is because emission of N2 has no additional fertilising effect, inasmuch as the
greater part of the atmosphere already consists of free nitrogen.
In practice, only air-borne emissions will contribute to eutrophication of terrestrial natural ecosystems.
5.3 EDIP97 characterisation factors
Current characterisation factors for eutrophication are typically based on the Redfield ratio. The Redfield ratio refers to the typical composition of aquatic phytoplankton: C106H263O110N16P. The
presently typical eutrophication factors do in most cases not distinguish between aquatic systems and terrestrial systems and actually model both as if they were impacts on aquatic systems. This is also the
case with the EDIP97 factors as listed in Table 23.6 in Wenzel et al. (1997). Only Lindfors et al. (1995) explicitly assess the impact on terrestrial systems by summing nitrogen emissions to air separately.
Additional problems with the current methods for characterisation of terrestrial eutrophication lie in the fact that they disregard the large spatial variations in dispersion patterns and in ecosystem sensitivities
within the deposition areas. These problems are discussed in Section 4.3 under acidification.
5.4 EDIP2003 characterisation factors
Until now, no characterisation factors have been developed specifically for terrestrial eutrophication. In the current context, the RAINS model2 has been used to establish terrestrial eutrophication factors.
Site-generic factors have been established (see Table 5.1), as well as site-dependent factors for 44 European regions (see the Annex 5.1 to this chapter) which through the region of release relates a nitrogen
emission to the terrestrial eutrophication, it causes within its deposition area. The principles of the RAINS-model and its use for calculation of site-dependent characterisation factors are described in Section
4.4.
The application of the EDIP2003 site-generic factors for terrestrial eutrophication is basically similar the application of the likewise site-generic EDIP97 factors (Section 5.5).
Application of the site-dependent factors for terrestrial eutrophication is also straightforward (see Section 5.6). Typical life cycle inventories already provide the only additional information which is required
for site-dependent characterisation, namely the geographical region where the emission takes place. The use of site-dependent terrestrial eutrophication factors adds resolving power of up to a factor
thousand between highest and lowest ratings, while combined uncertainties in the RAINS model to a large extent are cancelled out in the characterisation factors due to the large area of ecosystems they
cover. It has to be mentioned, however, that the critical loads for terrestrial eutrophication are more uncertain than those for acidification.
The dependence on the background situation of the receiving environment means that the potential for terrestrial eutrophication must be expected to vary with the total emission level and hence in time. To
allow assessment of this variation, the characterisation factors are also calculated for the predicted emission levels for 2010 as shown in Annex 5.1. The factors based on the 1990 emissions are chosen as
the default EDIP2003 characterisation factors but the factors for 2010 allow temporal differentiation for those emissions of the product system that will take place in the future (e.g. from the late use stage of
long-lived products or from the disposal stage).
Compared to the spatially determined variation between countries, the temporal variation within countries, determined in this way, is less significant.
What do the impacts express?
The site-generic, as well as the site-dependent, EDIP2003 eutrophication potentials of an emission are expressed as the area of terrestrial ecosystem within the full deposition area that is brought to exceed
the critical load of eutrophication as a consequence of the emission (area of unprotected ecosystem = m2 UES).
In comparison, the EDIP97 nutrient enrichment potential aggregates the terrestrial and the aquatic eutrophication potentials. It is expressed as a separate N-potential and P-potential simply reflecting the
content of the two nutrients in the emission. EDIP97 also facilitates aggregation into an NO3--potential reflecting the amount of NO3- that would lead to the same potential eutrophication in aquatic systems
limited by the relevant nutrient (i.e. in the aggregation, N-emissions are assumed emitted to N-limited aquatic systems and P-emissions to P-limited systems).
5.5 Site-generic characterisation
The site-generic terrestrial eutrophication factors are established as the European average over the 15 EU member countries in EU15 plus Switzerland and Norway, weighted by the national emissions in
Table 5.1.
Table 5.1. Factors for site-generic, and for site-dependent characterisation (in
0.01 m2 unprotected ecosystem/g)
|
Site-generic assessmentsg-CF(te)s |
Site-dependent assessmentSite-dependent factors for terrestrial eutrophication(sd-CF(te)s.i factors in Annex 5.1) |
Substance |
Factor |
standard deviation |
|
NO2 | 2.54 | 2.34 | sd-CF(te)|(NO2) |
NOx | 2.54 | 2.34 | sd-CF(te)|(NO2) |
NO | 3.88 | 3.58 | 1.53•sd-CF(te)|(NO2) |
HNO3 | 1.85 | 1.77 | 0.73•sd-CF(te)|(NO2) |
NH3 | 10.10 | 13.11 | sd-CF(te)|(NH3) |
The site-generic terrestrial eutrophication impact of a product can be calculated according to the following formula:

(5.1)
Where: sg-EP(te) = The site-generic terrestrial eutrophication impact, or area of ecosystem that becomes unprotected by the emissions from the product system (in 0.01 m2/f.u.)
sg-CF(te)s = The site-generic characterisation factor for terrestrial eutrophication from Table 5.1 which relates accumulated emission of substance
(s) to the impact on its deposition area in 0.01 m2/g)
Es = The emission of substance (s) (in g/f.u)
The spatially determined variation which potentially lies hidden within the site-generic terrestrial eutrophication impact can be estimated from the standard deviation given in Table 5.1 for each substance.
5.6 Site-dependent characterisation
The terrestrial eutrophying impact from a product system is often determined by one or a few processes. To avoid unnecessary work, applications where a site-dependent assessment is desired, may
therefore start with calculation of the site-generic terrestrial eutrophication impact of the product as described in the previous section. This site-generic impact can be used to select the processes with the
dominating contributions (step 1), and next to adjust their site-generic impacts with the relevant site-dependent terrestrial eutrophication factors (step 2 and 3). This procedure can be seen as a sensitivity
analysis-based reduction of those uncertainties in the site-generic impact which are posed by refraining from site-dependent characterisation.
Step 1
The site-generic terrestrial eutrophication impact from a product, as calculated in the previous section, is broken down into the contributions of the separate processes. These contributions are then ranked
from the largest to the smallest contribution, and the process with the largest contribution is selected.
Step 2
The site-generic terrestrial eutrophication impact calculated in step 1 is reduced with the contribution of the process selected in step 1. Next, the site-dependent impact from the emissions of this process is
calculated with the relevant site-dependent terrestrial eutrophication factors in Annex 5.1.

(5.2)
Where: sd-EP(te)p = The site-dependent terrestrial eutrophication impact or area of ecosystem that becomes unprotected by the emissions from the selected process (p) (in m2/f.u.)
sd-CF(te)s,i = The site-dependent characterisation factor for terrestrial eutrophication from Annex 5.1 that relates the emission of substance (s) in country or region (i) where the selected process (p) is
located to the impact on its deposition area (in m2/g). Emissions from an unknown region or from non-European regions can as a first approach be represented by the site-generic factors.
Es,p = The emission of substance (s) from the selected process (p) (in g/f.u)
The geographic region in which the emissions take place determines the relevant factors. The impact of emissions from unknown but probably European regions is calculated with the site-generic factors for
terrestrial eutrophication. The information about the spatial variation in these factors (see Table 5.1) should be taken into account in the next step. As a first approach, also the emissions from a non-European
or unknown region can be calculated with the site-generic factors from Table 5.1. The standard deviations in Table 5.1 give a range of potential spatial variation for the application of the site-generic factor
within Europe. Given the size of the variation in emissions and sensitivities within Europe, the site-dependent factor is expected to lie within this range for most regions also in the rest of the world. Expert
judgement may be used in the interpretation to assess whether the factor for emissions from processes in non-European regions should be found in the lower or upper end of the uncertainty range.
Step 3
The site-dependent contributions from the process selected in step 1 are added to the adjusted site-generic contribution from step 2. Step 2 is repeated until the site-dependent contribution of the selected
processes is so large that the residual spatially determined variation in the terrestrial eutrophication score can no longer influence the conclusion of the study (e.g. when the site-dependent share is larger than
95% of the total contribution).
5.7 Normalisation
The EDIP2003 person equivalent for terrestrial eutrophication is
2.1?103 m2/pers/yr.
Following the EDIP97 approach, the normalisation reference for terrestrial eutrophication is based on the impact caused by the actual emission levels for 1990 (see Hauschild and Wenzel, 1998d and
Stranddorf et al., 2005). Applying the EDIP2003 characterisation factors for terrestrial eutrophication, the total area of unprotected ecosystem in Europe is 77•106 ha or 77•101010 m2. The person
equivalent is calculated as an average European impact per person assuming a total European population of 3.70•108 persons.
5.8 Interpretation
The EDIP2003 terrestrial eutrophication impact potentials are improved in two aspects compared to the impact potentials calculated using the EDIP97 characterisation factors; the environmental relevance is
increased and spatial variation in sensitivity of the receiving environment is now included.
Environmental relevance
The environmental relevance is increased because the exposure of the sensitive parts of the terrestrial environment as well as the variation in sensitivity of these ecosystems are included in the underlying
model, which now covers most of the causality chain towards the protection area: Ecosystem health. This is particularly important because it increases consistency with weighting factors based on the
environmental relevance. The EDIP default weighting factors for nutrient enrichment are based on political reduction targets. These targets are also aimed partly at protecting ecosystem health. In comparison,
the EDIP97 factors only cover the potential for release of nutrients and furthermore, the eutrophication of terrestrial ecosystems is treated using aquatic eutrophication factors even though there are important
differences in the two types of eutrophication.
Spatial variation
The spatial variation in natural soil sensitivity to eutrophication can be large due to differences in background exposure and natural nutrient status. The variation in sensitivity between European regions shows
a factor 103 of difference between least and the most sensitive emission countries when expressed on a national scale. This variation is hidden when the EDIP97 factors or similarsite-generic characterisation
factors are used for characterisation.
5.9 Example
Applying the EDIP2003 factors, characterisation is performed on the inventory presented in Section 1.6.
Site-generic characterisation
As described in Section 5.5, first the site-generic impacts are calculated. The terrestrial eutrophication impact shown in Table 5.2 is determined using the site-generic factors from Table 5.1.
Table 5.2. Site-generic terrestrial eutrophication impacts for one supporting block made from plastic or zinc expressed as area of unprotected ecosystem (UES) per functional unit.
Click here to see the Table
Using site-generic characterisation factors, the largest terrestrial eutrophication impacts are found to be caused by the zinc supporting block. However, the potential spatial variation is so large (as revealed by
the spatially determined standard deviation) that the conclusion is highly uncertain. Therefore, a site-dependent characterisation is performed for those processes that contribute the most to the site-generic
terrestrial eutrophication impacts in order to reduce the spatially determined uncertainty and strengthen the conclusion.
Site-dependent characterisation
Table 5.2 shows that the predominant contributions to the site-generic terrestrial eutrophication impact are caused by the emissions of NOx.A minor contribution from NH3 is negligible in the overall impact.
For the zinc component, the main sources for NOx emission are identified as the production of zinc from ore which takes place in Bulgaria, the casting of the component which takes place in Yugoslavia, and
that part of the transport of the component, which takes place by truck through Germany (data not shown). For the plastic component, the main sources for NOx are found to be the production of plastic polymer in Italy, the flow injection moulding of the supporting block in Denmark and the transportation
of the component by truck, mainly through Germany (idem). The emissions from these processes contribute 99% and 75% of the full site-generic impacts of Table
5.2 for the zinc component and the plastic component respectively (data not shown).
In the calculation of the site-dependent impacts for these key processes, the relevant site-dependent factors from Annex 5.1 are applied. The results are shown in Table 5.3.
The site-generic impacts from the key processes are subtracted from the original site-generic impacts in Table 5.2 and the site-dependent impacts from the key processes calculated in Table 5.3 are added.
The terrestrial eutrophication impacts thus corrected are found in Table 5.4, and the difference to the original site-generic impacts of Table 5.2 is illustrated in Figure 5.1.
Table 5.3. Site-dependent terrestrial eutrophication impacts for key processes from either product system.
Zink part |
|
|
Emissions.
g/f.u. |
Terr. Eutr. impact,
Annex 5.10.01 m2 UES/g |
Impact0.01 m2 UES/f.u |
NOx emissions |
|
|
|
|
|
Zinc production, Bulgaria |
|
|
0.97 |
1.02 |
0.99 |
Zinc casting, Yugoslavia |
|
|
1.65 |
5.55 |
9.16 |
Transport, mainly Germany |
|
|
4.56 |
2.04 |
9.30 |
Total, zinc part |
|
|
|
|
19.4 |
Plastic part |
|
|
Emissions. g/f.u. |
Terr. Eutr. impact,
Annex 5.1 |
Impact |
|
|
|
|
0.01 m2 UES/g |
0.01 m2 UES/f.u |
NOx emissions |
|
|
|
|
|
Plastic production. Italy |
|
|
0.63 |
1.12 |
0.71 |
Flow injection moulding, Denmark |
|
|
0.48 |
5.33 |
2.56 |
Transport, mainly Germany |
|
|
1.74 |
2.04 |
3.55 |
Total, plastic part |
|
|
|
|
6.8 |
Table 5.4. Terrestrial eutrophication impacts from either product system with site-dependent characterisation of key process emissions
| Terrestrialeutrophication |
|
0.01 m2 UES/f.u |
Zinc component |
19.5 |
Plastic component |
9.2 |
Site-dependent characterisation hardly influences the size of the terrestrial eutrophication impacts. The zinc component has the largest impact in both cases. Around 99% of this impact is calculated using
site-dependent characterisation factors for the zinc-based component while the site-dependent share for the plastic-based component is around 75%. Even if the site-dependent characterisation were
performed for all the remaining processes in the product system, the result would thus not change significantly for the zinc-based component, given their modest share in the total and the standard deviation.
The spatially conditioned potential for variation of the impact has largely been cancelled. For the plastic component it might be required to include one or two processes more to obtain the needed robustness
of the result but this would hardly change the dominance of the zinc component.
Figure 5.1 Site-generic and site-dependent terrestrial eutrophication impacts from the two product systems. For the site-dependent impacts, the site-dependent characterisation factors have only been
applied for the key processes as described above.
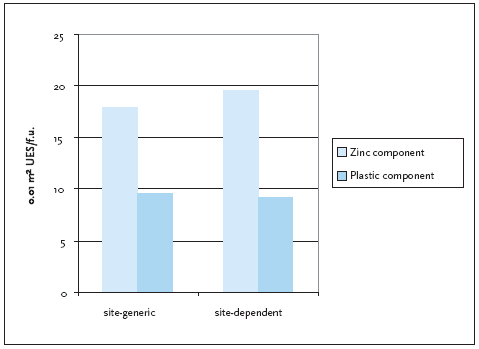
Annex 5.1: Site-dependent characterisation factors for terrestrial eutrophication
| 1990 factors |
2010 factors |
|
(in 0.01 m2 UES/gram) |
(in 0.01 m2 UES/gram) |
Region |
NOx |
NH3 |
NOx |
NH3 |
Albania |
1.58 |
6.91 |
0.80 |
3.12 |
Austria |
1.03 |
3.38 |
2.86 |
28.62 |
Belarus |
1.67 |
2.81 |
0.98 |
2.45 |
Belgium |
1.44 |
1.10 |
1.78 |
2.45 |
Bosnia/Herzegovina |
2.97 |
13.33 |
6.61 |
30.29 |
Bulgaria |
1.02 |
9.06 |
1.18 |
17.50 |
Croatia |
1.52 |
6.21 |
5.99 |
25.36 |
CRZF |
1.68 |
2.52 |
2.62 |
9.70 |
Denmark |
5.33 |
9.80 |
2.13 |
6.04 |
Estonia |
6.63 |
42.02 |
2.89 |
9.29 |
Finland |
11.29 |
91.69 |
3.40 |
79.00 |
France |
2.93 |
9.15 |
9.10 |
20.03 |
Germany new |
2.15 |
3.64 |
2.36 |
8.00 |
Germany old |
2.04 |
4.86 |
3.01 |
12.66 |
Greece |
0.56 |
15.67 |
0.42 |
2.04 |
Hungary |
1.70 |
5.67 |
7.33 |
20.73 |
Ireland |
0.37 |
0.51 |
0.15 |
0.19 |
Italy |
1.12 |
13.26 |
2.16 |
14.28 |
Latvia |
3.92 |
7.69 |
2.31 |
13.05 |
Lithuania |
3.23 |
5.72 |
2.11 |
14.98 |
Luxemburg |
0.10 |
0.16 |
1.30 |
3.61 |
Netherlands |
1.91 |
2.30 |
1.69 |
3.01 |
Norway |
6.29 |
10.11 |
1.09 |
0.75 |
Poland |
2.15 |
4.39 |
2.41 |
9.97 |
Portugal |
3.11 |
30.74 |
9.40 |
27.66 |
Moldova |
0.16 |
1.18 |
0.23 |
1.05 |
Romania |
1.29 |
5.18 |
2.09 |
7.02 |
Kaliningrad region |
0.21 |
0.92 |
0.62 |
2.80 |
Kola, Karelia |
0.72 |
5.07 |
0.21 |
1.73 |
Remaining Russia |
0.55 |
0.57 |
0.13 |
0.22 |
St.Petersborg reg. |
3.37 |
5.93 |
1.47 |
7.82 |
SKRE |
1.34 |
6.27 |
2.69 |
30.27 |
Slovenia |
1.09 |
10.22 |
2.38 |
21.83 |
Spain |
2.44 |
13.40 |
3.71 |
16.02 |
Sweden |
11.97 |
70.06 |
2.75 |
6.24 |
Switzerland |
0.90 |
5.76 |
2.65 |
24.78 |
Region |
NOx |
NH3 |
NOx |
NH3 |
Macedonia |
0.25 |
13.66 |
0.26 |
10.82 |
Ukraine |
0.62 |
3.42 |
0.47 |
3.40 |
United Kingdom |
1.77 |
3.14 |
0.84 |
0.89 |
Yugoslavia |
5.55 |
35.96 |
3.74 |
15.16 |
Atlantic ocean |
0.96 |
|
0.39 |
|
Baltic sea |
6.20 |
|
2.72 |
|
Mediterranean sea |
0.08 |
|
0.02 |
|
North sea |
1.86 |
|
1.15 |
|
(*) Mean |
2.54 |
10.10 |
3.25 |
13.51 |
(*) Standard deviation |
2.34 |
13.11 |
3.25 |
10.10 |
Minimum |
0.10 |
0.16 |
0.15 |
0.19 |
Maximum |
11.97 |
91.69 |
9.40 |
79.00 |
(*) The mean and standard deviations relate to E15+Norway+ Switzerland and are weighed with the national emissions of these countries
Footnotes
2 RAINS is an integrated assessment model that combines information on national emission levels with information on long range atmospheric transport in order to estimate patterns of deposition and
concentration for comparison with critical loads and thresholds for acidification, terrestrial eutrophication-via-air and tropospheric ozone creation.
| Front page | | Contents | | Previous | | Next | | Top |
Version 1.0 january 2006, © Danish Environmental Protection Agency
|