| Front page | | Contents | | Previous | | Next |
More environmentally friendly alternatives to PFOS-compounds and PFOA
6 Environment and health assessment of PFOS and PFOA and other PFAS substances
6.1 Environmental fate
6.1.1 Physical-chemical properties
Most perfluoroalkyl substances (PFAS) are very stable compounds, which have low vapour pressures, surface energies, and special surface-active properties. There are similarities to persistent organic
pollutants (POPs) in being stable and hydrophobic but differ with PFAS having both oleophobic properties in one end of the molecule and sometimes polar/hydrophilic properties in the other functional end,
where POPs are lipophilic and non-polar. Hence, PFAS will not accumulate in fatty tissues and will often occur dissociated as anions and interacts with polar sites in membranes and in sediments. For PFOA
a reported octanol-water partition coefficient (Log Pow) is 5 (3M 2000), but the special solubility profiles of PFOS and PFOA make environmental fate predictions based on octanol-water partition
coefficients irrelevant for these chemicals.
For the individual PFOS and PFOA-related substances, large differences exist between the water solubility and vapour pressure etc. Some data examples are shown in Table 5.1. It should be noted that
commercial products are not pure substances, and can contain several % of branched isomers and traces of other PFAS.
Perfluorinated carboxylic acids (PFCA) are stronger acids than their non-fluorinated counterparts and have the corresponding lower pKa. For PFOA the pKa is 2.80 (Kissa 2001). PFOA is dissociated in
water and does not evaporate from the water phase. Water is believed to be the target compartment for PFOA.
Fluorotelomers (linear, long-chain, polyfluorinated alcohols) have higher calculated vapour pressures than the parent alcohol; for example 10:2 FTOH is 1000 times more volatile than dodecanol, possibly
because of the unique molecular geometry (Stock et al. 2004). Examples are given in Table 6.1:
Table 6.1: Water solubility and vapour pressure for some PFAS (Heckster et al. 2003; Stock et al. 2004; Shoeib et al. 2004)).
Substance |
Solubility in water (g/L) |
Vapour pressure (Pa) at 25°C |
Octanol-air partition coefficient LogKoa (20°C) |
Potassium perfluorooctane sulfonate (PFOS-, K+) |
0.519 |
3.31 x 10<-4 |
|
Perfluorooctanoic acid (PFOA) |
9.5 (20?) |
70 |
|
Ammonium perfluorooctanoate (PFOA-, NH4+) |
>500 |
<1.3-9.2 x 10<-3 |
|
N-Methyl perfluorooctane sulfonamidoethanol (MeFOSE) |
|
0.002 |
7.7 |
N-Ethyl perfluorooctane sulfonamidoethanol (EtFOSE) |
1.51 x 10<-4 |
0.504; 0.009? |
7.78 |
N-Ethyl perfluorooctane sulfonamidoethyl acrylate (EtFOSEA) |
8.9 x 10<-4 |
0.002 |
7.87 |
1H,1H,2H,2H-Perfluorohexanol (4:2 FTOH) |
|
992 |
|
1H,1H,2H,2H-Perfluorooctanol (6:2 FTOH) |
1.2-1.7 x 10<-2 |
713 |
|
1H,1H,2H,2H-Perfluorodecanol (8:2 FTOH) |
1.40 x 10<-4 |
254 (2.93) |
|
1H,1H,2H,2H-Perfluorododecanol (10:2 FTOH) |
|
144 |
|
6.1.2 Abiotic degradation
The perfluorocarbon chain, with the very strong C-F bindings, is extremely resistant to heat, UV-radiation and to chemical attacks by acids and bases, and to reducing and oxidizing agents. The functional
end groups (sulfonamides, alcohol etc.) will on the other hand be more readily transformed in the environment and in organisms, and the more complex compounds will finally be degraded to the most
persistent sulfonates (e.g. PFOS) and carboxylates (e.g. PFOA).
Fluorinated organic polymers are very stable to hydrolysis resulting in half-lives from 1-5 years to 500 years (3M). However, heating of fluoropolymers such as polytetrafluorethylene (PTFE) to more than
350oC, degradation in smaller molecules occurs, including a small (0.01%) formation of PFOA (Ellis et al. 2001).
Smoke chamber experiments with fluorotelomer alcohols (4:2, 6:2 and 8:2 FTOH) exposed to chlorine atoms as a surrogate for OH-radicals indicate that these chemicals in the atmosphere can
oxidise/degrade to series of perfluorinated carboxylic acids (Ellis et al. 2004). The yields from 8:2 FTOH was mainly PFNA [10] (1.6%) and PFOA (1.5%) and to a lesser extend shorter chain acids.
Atmospheric lifetime of short chain FTOHs, as determined with its reaction with OH-radicals, was approximately 20 days making the molecule able to travel about 7000 km (Ellis et al. 2004).
6.1.3 Biodegradation
The 175 polyfluorinated substances on a list developed by Canadian authorities were studied with a computer program simulating microbial degradation. The prediction was that 109 substances might be
degraded to PFOS and 61 to PFOA (Dimitrov et al. 2004).
In a laboratory test using a microbial enrichment culture 8:2 FTOH aerobic degradation occurred. Telomer acids and PFOA were identified as metabolites. 85% of the telomer alcohol was degraded after a
week. The half-life was about one day (Schröder 2003). There was no aerobic degradation of either PFOS or PFOA derivatives. However, microbiological degradation of PFOS and PFOA in
contaminated sludge occurred under anaerobic conditions. PFOS biodegraded easier than PFOA. The former compound disappeared within 2 days and the later in 25 days. The degradation products were
not identified (Schröder 2003).
Dinglasan et al. (2004) examined the aerobic biodegradation of the 8:2 Telomer alcohol (8:2 FTOH) using a mixed microbial system. The initial measured half-life of the 8:2 FTOH was 0.2 days mg-1 of
initial biomass protein. Volatile metabolites and nonvolatile metabolites were identified and quantified. Telomer acids (CF3(CF2)7CH2COOH; CF3(CF2)6CFCHCOOH) and PFOA were identified as
metabolites during the degradation, the unsaturated telomer acid being the predominant metabolite measured.
6.1.4 Sorption
Table 6.2 provides the results summary table from the adsorption/desorption study of ammonium perfluorooctanoate (APFO) to soil according to OECD test guideline 106: Adsorption-Desorption Using a
Batch Equilibrium Method (APME, 2004). These results indicates that the Koc values obtained for APFO are in the low end of the range of Koc values used for the recommended reference substances
from the OECD test guideline 121: HPLC screening method based on soil adsorption data. This range goes from a low log Koc value of 1.25 (Koc ~ 18) for Acetanilide, to a high log Koc value of 5.63
(Koc ~ 426580) for DDT.
Table 6.2: Summary of the results from the adsorption/desorption study of ammonium perfluorooctanoate (APFO) to soil according to OECD test guideline 106.
|
|
Drummer |
Hidalgo |
Wilmington
Sludge
|
Cape Fear |
Keyport |
Adsorption |
(%) |
79.3 – 88.9 |
27.8 - 43.8 |
69.5 - 87.3 |
54.1 - 73.7 |
64.5 - 80.8 |
Kd |
(mL/g) |
4.25 – 8.86 |
0.41 - 0.83 |
12.6 - 36.8 |
1.19 - 2.84 |
1.82 - 4.26 |
Koc |
(mL/g) |
73.8 – 111 |
53.0 - 108 |
20.5 - 59.6 |
95.9 - 229 |
48.9 - 115 |
Kom |
(mL/g) |
42.8 – 89.2 |
30.8 - 62.6 |
11.9 - 34.6 |
55.6 - 133 |
28.4 - 66.5 |
KF |
(μg 1-1/n (mL)1/n g -1 ) |
5.64 |
0.59 |
3.90 |
3.23 |
1.64 |
1/n |
|
0.994 |
1.00 |
1.36 |
0.885 |
1.11 |
Soils tested at 1:1 soil:solution ratio; Wilmington sludge tested at 1:5 solids:solution ratio
24 hour equilibration time
6.2 Environmental exposure and occurrence
6.2.1 Environmental release
Emissions of PFOA, PFOS and related chemicals to the environment (air and water) may happen directly from production and processing plants, e.g. release during product use, e.g. fire-fighting foam, and
as product impurities or degradation products, or release of PFOA during the production of fluoropolymers such as PTFE . It should be realised that the use of fire fighting foams on e.g. offshore oil
platforms in the North Sea, may be a potential direct water pollution risk.
Sources of FTOH are currently unknown, although it is likely that they may be released from the decomposition of polymeric and non-polymeric materials that incorporate FTOHs or from release of residual
amounts of the FTOHs themselves that failed to be covalently linked to the polymer during production (Ellis et al. 2003)
It is expected that PFAS is present in the environment primarily in the form of the final stable degradation products PFOS and PFOA.
6.2.2 Long-range transportation
The binding to water and the low volatility make it less likely that PFOS and PFOA will be spread long-range with the air by the "grass-hopping" and cold condensation mechanisms as persistent organic
pollutants (POPs) in general can. The occurrence of PFOS and PFOA in remote areas such as the arctic and in arctic animals is therefore puzzling. However, the prevailing hypothesis is that long-range
transport to the arctic occurs via volatile precursors of both PFOS and PFOA, with subsequent degradation to these stable products. Volatile PFOS-precursors are a.o. MeFOSE, EtFOSE (OECD,
2002). It has been hypothesized that fluorotelomer alcohols (FTOH) may be long-range transported and hereby reach remote arctic areas, where they can degrade to the more stable PFOA (Ellis et al.
2004).
Alternatively or in addition, direct use of fluorosurfactants in fire-fighting foams used in remote regions may constitute a source of PFOS and PFOA. Recently a hypothesis has also been brought forward that
ocean current transport may in part explain the presence of PFOS and PFOA in the arctic (Yamaguchi et al. 2004; Caliebe et al. 2004).
6.2.3 Levels in air
Six different perfluorinated substances have been detected in the air at a highly urbanized site of Toronto, Canada. The mean concentrations ranged from 14 pg/m3 EtFOSA to 205 pg/m3 EtFOSE, and
totally 260 pg/m3. At a rural site (Long Point) in Canada the levels were 2-3 fold less (74 pg/m3) and only one substance was found (Martin et al. 2002).
Polyfluorinated sulfonamides and fluorinated telomer alcohols have been measured by Stock et al. (2004) in six North American cities – Reno (NV), Griffin (GA), Cleves (OH), Winnipeg (MB), Long Point
(ON) and Toronto (ON). Mean concentrations of total perfluorinated sulfonamides (EtFOSA, MeFOSE and EtFOSE), ranged from 22 pg/m3 (Winnipeg) to 403 pg/m3 in Griffin. Mean concentrations of
total FTOHs (6:2, 8:2 and 10:2) ranged from 11 pg/m3 (Winnipeg) to 165 pg/m3 (Toronto). Surface treatment products, previously manufactured by 3M Company for soil, stain and water protection of
home furnishings – including carpets, were primarily MeFOSE-based polymers. It was in line with the fact that the highest mean and single levels of MeFOSE in the air (359 pg/m3 and 1549 pg/m3) were
measured in Griffin, a location of carpet production.
Three perfluoroalkyl sulfonamides (MeFOSE, EtFOSE and MeFOSEA) used in surface treatment formulation for textile and paper products to impact oil and water resistance were measured in indoor and
outdoor air. Levels of MeFOSE and EtFOSE in outdoor air were 16-32 pg/m3 and 8,5-10 pg/m3, respectively. MeFOSEA was not detected. Indoor air from 4 houses and an old laboratory ranged
667-8315 pg/m3 and 289-1917 pg/m3, respectively. Thus, indoor air levels were about 100 times higher than outdoor values. Indoor levels of MeFOSEA in three of the houses were 5-283 pg/m3 (Shoeib
et al. 2004).
The high indoors air levels of perfluoroalkyl sulfonamides compared to outdoors levels were confirmed in the recent study by Shoeib et al. (2004) using passive air samplers showing 25 times higher levels
indoors of MeFOSE (geometric mean 1968 pg/m3) and 13 times higher levels of EtFOSE (geometric mean 1033 pg/m3). Results for EtFOSA (geometric mean 54 pg/m3) and MeFOSEA (geometric mean
38 pg/m3) were a magnitude lower.
The content of PFOS in airborne dust along Japanese roads was up to 427 ng/g. The air concentrations ranged 0.1-2.1 pg/m3 in a rural town and 2.3 -22 pg/m3 in an urban city (Sasaki et al 2003). Higher
concentrations were observed in the summer than in the winter.
FTOH and polyfluorinated sulfonamides detected in tropospheric concentrations typically ranging from 7 to 106 pg/m3 and from 14 to 393 pg/m3; higher concentrations were observed in urban locations
relative to rural locations (Martin et al. 2002). Tropospheric measurements in the arctic in support of the long-range transport hypothesis have not yet been published.
Table 6.3: Levels of PFAS in the air (pg/m3).
Click here to see the table
6.2.4 Levels in ground- and drinking water
Fluorotelomer sulfonates, primarily polyfluoroalkylthioamido sulfonates, are used as surfactant to lower the surface tension of fire-fighting foams used to fight hydrocarbon-fuelled fires (aqueous film forming
foams (AFFF)). These surfactants and their degradation products may occur in ground water as a result of this use. The concentrations will vary with time and distance from the use place (Moody & Field
1999; Moody et al. 2003; Schultz et al. 2004).
A study at a former Air Force base in Michigan showed that ground water from wells around a fire-training area contained perfluorinated degradation products (PFOS, PFHxS, PFOA and PFHxA) five or
more years after its last known use ranging in concentrations from 3-120 μg/L (Moody et al. 2003).
In a follow-up study, including two another former military facilities in Nevada and Florida, where such fire foams were used, these four degradation products were found in ground water from all three
facilities. In addition, even fluorotelomer sulfonates were measured at the Michigan (<0.6-182 μg/L) and Florida facilities (1,100-14,600 μg/L). In the Nevada and Florida facilities some ground water samples
also contained PFBS, PFPS and PFHpA (Schultz et al. 2004).
In tap water delivered from 8 waterworks in Japan the PFOS levels were 0.1-4 ng/L. In tap water delivered by another waterwork, which obtained the raw water from a polluted river, levels of PFOS were
about 50 ng/L (Harada et al. 2003).
For guidance purposes, 3M scientists, taking into account the US Environmental Protection Agency (USEPA) risk assessment approach, have developed a lifetime drinking water health advisory for PFOS.
This advisory of 1000 ng/L (1 ppb) represents the concentration of PFOS in drinking water that is not expected to cause any adverse (non-cancer) effect over a lifetime of exposure, with a substantial margin
of safety. It was assumed that 2L of water is consumed daily, and that drinking water represents 20% of the daily exposure to PFOS (Hansen et al. 2002).
A team of scientific experts and governmental regulatory agencies – including US EPA – developed screening levels for PFOA protective of human health. Their recommended safe level for PFOA content
in water was 150 ug/L (150 ppb) (CATT, 2002). The US Ohio EPA independently reviewed and confirmed the CATT findings.
6.2.5 Levels in surface water
Hansen and co-workers (2002) have measured PFOS and PFOA in the Tennessee River in Alabama. Upstream Baker's Creek, which receives influents from several manufacturing facilities including a
fluorochemicals manufacturing plant, PFOS levels in the river water averaged 32±11 ng/L and ranged from 17 to 54 ng/L. Upstream PFOA levels were below the detection limit of 25 ng/L. Downstream
concentrations averaged 114±19 ng PFOS/L and 394±128 ng PFOA/L and maximum values reached 144 ng PFOS/L and 598 ng PFOA/L.
In June 2000 at the international airport at Toronto, Canada, an accidental spill happened, and 22,000 litre of fire-fighting foam containing perfluorinated surfactants including about 300 kg PFOS entered
into Etobicoke Creek. During a 153 days period 54 samples of creek water were obtained and analysed for perfluorohexane sulfonate (PFHxS), perfluorooctane sulfonate (PFOS), perfluorooctanoic acid
(PFOA), and total perfluorinated surfactant concentrations. Upstream levels of PFHxS and PFOS were below the detection limit (<0.01 μg/L), and PFOA levels were also very low (0.008-0.033 μg/L).
Downstream levels were <0.02 –134 μg/L for PFHxS, <0.016-2210 μg/L for PFOS, and <0.02-10.6 μg/L for PFOA. Total perfluorinated surfactant concentrations upstream ranged between 0.011 and
0.028 μg/L, and downstream the concentrations ranged from 2 to 17,000 μg/L. The highest levels were measured on day one and day two after the accident and until 15 km downstream (Moody et al. 2002).
On September 26, 2003, magnitude 8.3 earthquakes strucked Hokkaido Island in Japan and initiated a. o. major fires at an oil storage facility at a refinery (Yamashita et al. 2004). At least 40,000 litre of
fire-fighting foam containing perfluorinated compounds were used to control the fires. The runoff waters contained 3,669 ng PFOS/L, 149 ng PFHxS/L, 200 ng PFOSA/L, 300 ng PFNA/L, 162 ng
PFOA/L and smaller levels of PFBS, PFUnA, PFDeA, PFHpA and PFHxA. Since October 2003 the environmental levels of these chemicals were monitored in waters and snow around. Mean
concentrations of PFOS decreased from 42 ng/L to 4 ng/L from October to December. High levels in soil and snow in December indicate some persistent airborne sources.
In Japan PFOS concentrations in 126 samples of surface waters, mainly from rivers, ranged 0.2-157 ng/L with a geometric mean of 2.37. In effluents from a wastewater plant concentrations were between
300 and 440 ng/L (Harada et al. 2003). Another Japanese study found PFOS in nine out of 25 surface water samples and reported levels of PFOS in the Tokyo Bay surface water ranging from 8 to 59
ng/L with a mean of 26 ng/L (Taniyasu et al. 2003). In other bay water samples levels were lower and below the detection limits. In Osaka Bay and Ariake Bay mean levels of PFOS were respectively 8.7
(range: <4-21) and 4.8 ng/L (range <9-11). In Lake Biwa PFOS levels ranged <4-7.4 ng/L with a mean of 3.8 ng/L. Levels of PFHxS and PFBS were below detection limits. In seawater from South China
Sea and Sulu Sea PFOS levels ranged 0.01-0.11 ng/L. Concentrations of PFOA were 4-7 times higher than those of PFOS. Overall concentration of PFOS in open ocean waters was 60 to 600 times
lower than coastal waters of Japan (Yamashita et al. 2003).
Coastal waters of Hong Kong, the Pearl River Delta, including South China Sea, and Korea have been analysed for PFOS and PFOA (So et al. 2004). The ranges of PFOS concentrations were 0.09-3.1,
0.02-12, and 0.04-730 ng/L, respectively, and the ranges of PFOA concentrations were 0.73-5.5, 0.24-16, and 0.24-320 ng/L, respectively. Thus highest concentrations analysed in Asia were in coastal
waters around Korea.
In a newer study with a lower limit of quantification highest levels were found in seawater samples from Tokyo Bay with up to 192 ng PFOA/L, 71 ng PFNA/L, 58 ng PFOS/L and 5.6 ng PFHxS/L
(Taniyasu et al. 2004). Coastal area of Hong Kong, China and Korea had about 10 times lower maximum levels, though PFNA levels were 100 times lower. Even lower levels of PFOA (less than 0.4 ng
PFOA/L), PFOS and PFHxS but not PFNA were detected in open Pacific Ocean waters. Similar low levels of PFOA, PFOS and PFHxS were also determined in the deep (>1000 m) ocean waters
indicating widespread contamination. The "background" surface water levels of perfluorinated compounds in the Pacific Ocean were in general 5-10 timeslower than in the Atlantic Ocean.
The first data for perfluorooctane surfactants in Great Lakes water have recently been published (Boulanger et al. 2004). Sixteen samples from Lake Erie and Lake Ontario were analysed for eight
perfluorinated chemicals. Concentrations of PFOS in the two lakes ranged from 21-70 and 27-50 ng/L, respectively. Analysis also showed the presence of PFOS precursors, NEtFOSAA [11] (range of
4.2-11 ng/L) and FOSA [12] (range of 0.6-1.3 ng/L). Perfluorooctane sulfinate, another precursor, was identified at six of eight locations in concentrations up to 17 ng/L. Other precursors such as EtFOSE,
PFOSAA and EtFOSA were not detected.
In Michigan surface water samples background concentrations were between 2 and 5 ng/L for PFOS and <8-16 ng/L for PFOA with maxima of 29 and 36 ng/L, respectively (Sinclair et al. 2004).
Seven perfluorinated compounds have in 2003 been measured in water sampled in the depth of 5 m at 15 locations in the North Sea (Caliebe et al. 2004). The highest levels, about 20 ng/L, were found for
PFOS and PFOA in samples from the mouth of River Elbe while the other detectable compounds (PFHxA, PFHxS, PFHpA, PFNA, PFDeA and PFOSA) range from 1-3 ng/L. Relatively high levels of
PFOA and PFOS were also found in samples from the Dutch and German Waddensea (5-10 ng/L). One sampling station at the northern end of the island of Sild will be representative for the Danish
Waddensea. In open waters levels were below 1 ng/L, and only PFOA could be quantified. In River Elbe waters the concentration of PFOS was higher than of PFOA. In seawaters the relation was
opposite.
In a recent Nordic screening project a few samples of water from Norway, Denmark, Sweden, Finland, Iceland and Faroe Islands were investigated for perfluorinated compounds (Berger et al. 2004;
Kallenborn et al. 2004). The levels of PFOA were highest with median levels of 5.2 ng/L in seawater, 7.8 ng/L in lake water, 13.1 ng/L in rainwater, 20.5 ng/L in sewage effluent and 297 ng/L in landfill
effluent.
6.2.6 Levels in sediment and sludge
The binding of some PFAS to sediments and sludge is strong and stable, which means a high potential for accumulation herein. In a 3M study of six urban locations in the USA, where some PFAS was
detected in various media the highest levels occurred in sewage sludge from cities with production or industrial use of the PFAS (3M 2001).
In a recent Nordic screening project a few samples of sewage sludge from Norway, Denmark, Sweden, Finland, Iceland and Faroe Islands were investigated for perfluorinated compounds (Berger et al.
2004; Kallenborn et al. 2004). PFOS was the dominating substance in Denmark, Norway and Sweden and PFOA was highest in Iceland and Faroe Islands. Highest maximum levels of PFOS were found in
Sweden (2644 pg/g ww), Denmark (1041 pg/g ww) and Norway (1023 pg/g ww). Somewhat lower levels in Finland (925 pg/g ww), and in Island and Faroe Islands the levels were much lower at
220-241 pg/g ww. In Finland PFHxA was the second most abundant, and PFNA was found in Norwegian, Danish and Finnish sludge. Sediment samples had in general lower levels than sludge. The highest
level of PFAS was 1150 pg/g ww in a Finnish sample. Contamination was also high in Norway but not in Sweden and other countries.
6.2.7 Levels in biota and wildlife
John P. Giesy and co-workers at Michigan State University did report for the first time the global contamination and widely distribution of PFOS in wildlife (Giesy & Kannan 2001). Tissues of various
species of aquatic animals (seals, otter, sea lion, dolphin, polar bear, mink), birds, fish and amphibians collected in the 1990's for other purposes were scanned and analysed for PFOS, PFOSA, PFHxS and
PFOA. However, in most samples only PFOS could be quantified in concentrations > 1 ng/g (ppb) and reported. There was no difference between levels in male and female animals as for the lipophilic
POPs. PFOS is also found in bird and fish eggs (up to 250 ng/g) suggesting possible maternal transfer during yolk formation (Giesy & Kannan 2002).
Other studies were initiated and in Europe PFOS levels in the biota were especially high at 36 ng/g to 1700 ng/g in the rivers and coast near Antwerp in Belgium, where a fluorochemical factory is located
(Van de Vijver at al. 2002; Hoff et al. 2003). Where the PFOS level in seals is higher in Europe than the US, it is opposite with the levels in dolphins and seabirds.
Kannan and Giesy (2002) have studied the frequency of detection (detection limit: 1-35 ng/g ww) and the maximum concentrations of PFOS, PFOA, FOSA and PFHxS in animal tissues (see Table 6.4):
Table 6.4: max. conc. (ng/g ww or ppb) and frequency of detection (%) in animal tissues
Species |
PFOS |
PFOA |
PFOSA |
PFHxS |
Marine mammals |
1520 (77%) |
41 (4%) |
880 (15%) |
86 (10%) |
Mink and Otter |
4900 (100%) |
110 (11%) |
680 (11%) |
85 (10%) |
Birds |
2570 (60%) |
440 (4%) |
530 (10%) |
185 (11%) |
Fishes |
1000 (38%) |
46 (2%) |
120 (9%) |
20 (3%) |
6.2.7.1 Levels in zooplankton and shrimps
Composites of zooplankton from Frobisher Bay in the Eastern Canadian arctic contained 1.1-2.1 ng PFOS/g ww and 1.7-3.4 ng PFOA/g ww. Levels in shrimps from the same area were about 5 times
lower (Tomy et al. 2003).
6.2.7.2 Bioaccumulation in fish
Analysis of existing wildlife data shows that organisms consuming fish contain greater concentrations than their food sources, and a PFOS liver accumulation ratio was measured at 22 for mink feeding on fish
meals (Giesy & Kannan 2001).
In two separate laboratory experiments juvenile rainbow trout (Oncorhynchus mykiss) were exposed either via water or diet to a mixture of a homologous series of perfluoroalkyl carboxylates and
sulfonates during 32 days followed by a 41 days depuration period (Martin et al. 2003ab). The carcass bioconcentration factors increased with increasing chain length and ranged from 4.0 to 23,000 (Martin
et al. 2003a). The substances were efficient absorbed from the feed but only carboxylates with more than 6 perfluoroalkyl carbons and sulfonates with more than 4 perfluoroalkyl carbons were detectable in
fish tissues at all given sampling times. The perfluorinated acids accumulated to the greatest extend in blood > kidney > liver > gall bladder. Under the circumstances of the study no biomagnification was
observed because the half-lives did not exceed 46 days, thus uptake via water (bioconcentration) is probably more important (Martin et al 2003b). Data from the two papers of Martin and co-workers are
shown in Table 6.5
The depuration carcass half-lives for rainbow trout ranged from 3 days for PFOA to 13 days for PFOS and to 32 days for PFTA. The food chain bioaccumulation factor (BAF) ranged from 0.038 (PFOA)
to 1.0 (PFTA). Thus both half-lives and bioaccumulation factors did increase with the length of the perfluoroalkyl chain.
Table 6.5: Accumulation of PFAS in fish carcass (Martin et al. 2003ab).
Compound |
Half-life (days) |
BAF |
BCF |
PFOA |
3.0±0.4 |
0.038±0.006 |
4.0±0.6 |
PFDA |
9.9±1.3 |
0.23±0.04 |
450±62 |
PFUnA |
11±1.4 |
0.28±0.04 |
2,700±400 |
PFDoA |
15±1.9 |
0.43±0.06 |
18,000±2,700 |
PFTA |
35±8.3 |
1.00±0.25 |
23,000±5,300 |
PFHxS |
9.1±1.1 |
0.14±0.02 |
9.6±1.0 |
PFOS |
13±1.8 |
0.32±0.05 |
1,100±150 |
Clearance of PFOA from fathead minnows took more than 15 days (3M 1995).
In a recent study Martin et al. (2004) analyzed for PFOS, the homologous series of PFCAs ranging from 8 to 15 carbons in chain length, and the PFOS-precursor heptadecafluorooctane sulfonamide
(FOSA) in various organisms from a food web of Lake Ontario. PFOS was the dominant acid in all samples, but long chain PFCAs, ranging in length from 8 to 15 carbons, were also detected in most
samples between <0.5 and 90 ng/g. By accounting for the known diet composition of lake trout, it was shown that bioaccumulation was indeed occurring at the top of the food web for all perfluoroalkyl
compounds except PFOA.
It is clear that perfluoroalkyl sulfonates have greater BCF, BAF, BMFs, half-lives and uptake rates than corresponding acids. The extent of bioconcentration of PFAS appeared to be highly structure
dependent, and PFOA with less than seven and PFOS with less than six fluoroalkylcarbons did not accumulate in rainbow trout.
6.2.7.3 Accidental release of PFOS
In June 2000 at the international airport at Toronto, Canada, an accidental spill happened, and 22,000 litre of fire retardant foam containing perfluorinated surfactants including about 300 kg PFOS entered
into Etobicoke Creek. Three weeks after the accident 6 fishes (common shiner, Notropus cornutus) were catched in the river, and the livers analysed for perfluorinated compounds (Moody et al. 2002).
Total perfluoroalkane sulfonate concentrations in the fish livers ranged 2-73 μg/g and it was mainly PFOS. Two samples contained small levels of PFBS (<0.009 μg/g) and four samples of PFHxS (<0.062
μg/g). Total levels of seven determined perfluorocarboxylates in fish livers ranged 0.07-0.40 μg/g thus 100 times lower. The most abundant of these was PFDA with 0.032-0.14 μg/g, followed by PFUnA with
0.013-0.083 μg/g, PFHpA with 0.005- 0.048 PFOA with 0.006-0.04 μg/g, PFHxA with <0.002-0.040 μg/g, PFTA 0.009-0.034 μg/g and PFPeA with <0.002-0.013 μg/g. A half year later 3 additional fishes
were catched and analysed. Total perfluoroalkane sulfonate concentrations in the fish livers then ranged 9.2-40 μg/g, thus no considerable decrease. However, total levels of perfluorocarboxylates ranged
0.82-1.02 μg/g, thus a considerable increase.
Based on the data in this study the bioaccumulation factor (BAF) for PFOS in fish livers was calculated to range between 6,300-125,000 (Moody et al. 2002; 2003). This range is much larger than the result
of the previously mentioned experimental study of rainbow trout.
6.2.7.4 Levels in fish
In their original paper Giesy & Kannan (2001) reported significant levels (up to 380 ng/g ww) of PFOS in fish (eggs, liver and plasma) from Michigan waters (see Table 6.6). PFOS levels in tuna fish from
the Northern Pacific were lower than the limit of quantification, while tuna liver from the Mediterranean contained 21-87 ng PFOS/g ww. In livers from tuna fish taken in the North Pacific the levels were
below the detection limit of 7 ng/g (Giesy & Kannan 2002). The levels were clearly higher in populated and industrialised area than in remote locations. In a later paper (Kannan et al. 2002c) PFOS levels in
blood from tuna and swordfish from the Mediterranean Sea were reported. Levels ranged 27-52 (mean 40) ng/mL and 4-14 (mean: 7.2) ng/mL, respectively. Levels of PFOSA ranged 13-19 ng/mL (mean:
15) and 1.1-28 ng/mL (mean: 15), respectively. However, levels of PFOA and PFHxS were below the quantification limit. The same was the case for all the perfluorochemicals in Atlantic salmon.
In Frobisher Bay in the Eastern Canadian arctic the highest levels of PFOA (2.9-57 ng/g ww) were in deepwater redfish (Tomy et al. 2003). This fish also contained an extremely high level of EtFOSA.
Various fish species from Japan did contain from 3 to7,900 ng/g ww PFOS in the livers and 1 to 834 ng/mL in the blood. Some of them also contained PFHxS in levels up to 18 ng/g liver and 121 ng/mL
blood (Taniyasu et al. 2003). In all samples PFBS levels were below the detection limit.
A recent study identified both PFOS and PFOA in biota from the Canadian arctic (Martin et al. 2004). Fish were collected at various locations. PFOS was the major contaminant and levels of FOSA were
alsorelatively high but all samples contained also perfluorinated carboxylic acids (PFCA) ranging in length from 9 to 15 carbons. In general, odd-length PFCAs exceeded the concentration of even-length
PFCAs and concentration decreased with increasing chain length. Levels were generally lower than levels for similar animals in the USA.
Sinclair et al. (2004) found PFOS (ranges: <7 – 381 ng/g ww; means: 43-263 ng/g ww) in livers, muscle and eggs of Chinook salmon, lake whitefish, brown trout and other Michigan fish. No other
perfluorinated compound was present.
PFOS ranged from 27 to 52 ng/mL (mean: 40) and 4 to 21 ng/mL (mean: 10), respectively, in blood from bluefin tuna and swordfish catched in the Italian part of the Mediterranean (Corsolini & Kannan
2004). In livers PFOS ranged 21-87 ng/g ww (mean: 47) and <1-13 ng/ ww (mean: 7), respectively. The levels of FOSA in bluefin tuna were 2-4 fold less than for PFOS. It was opposite in swordfish
blood, where FOSA concentrations were 1.5-fold greater.
In a recent Nordic screening project a few samples of fish were investigated for perfluorinated compounds (Berger et al. 2004; Kallenborn et al. 2004). The distribution was characterized by a high
variability. PFOS followed by PFOSA dominated over PFOA in freshwater fish. The highest levels of 551 ng PFOS/g ww and 141 ng PFOSA/g ww were measured in a Finnish pike sample. Marine fish
samples from Faroe Island had the lowest contamination, and PFOSA was the most abundant substance. Otherwise PFOS dominated in samples from other areas. In all the Icelandic samples PFDS and
PFHxA were present. In the Danish samples PFHxA, PFHpA and PFOA were present. Levels of PFOS in Danish flounders and herrings were around 20 ng/g ww.
Table 6.6: Perfluorinated substances in fish.
Click here to see the table
6.2.7.5 Shellfish
PFOS was measured in oysters (Crassostrea virginica) collected in 1996-1998 from 77 locations in the Gulf of Mexico and Chesapeake Bay. In 51 of these locations PFOS was detected in
concentrations ranging from <42 to 1225 ng/g dry weight with a median of 387 ng/g dw (Kannan et al. 2002b). Only one of the twelve samples from Chesapeake Bay had measurable levels and that was as
high as 1106 ng/g. In Puerto Rica PFOS levels averaged 437 ng/g dry weights.
6.2.7.6 Amphibians
A few frogs and turtles have been studied by Giesy & Kannan and the levels were relatively high (see Table 6.7).
Table 6.7: PFOA and PFOS in Amphibians.
Amphibian |
Location |
Species |
Tissue |
Number of samples |
PFOA concentration (ng/g ww) |
PFOS concentration (ng/g ww) |
Reference |
Range |
Mean |
Range |
Mean |
Yellow-blotched map turtle |
Mississippi |
|
Liver |
6 |
|
|
39-700 |
190 |
Giesy & Kannan 2001 |
Green frogs |
SW Michigan |
|
Liver |
4 |
|
|
<35-290 |
|
|
Snapping turtles |
Lake St. Clair, Michigan |
|
Plasma (ng/ml) |
5 |
|
|
1-170 |
72 |
|
6.2.7.7 Birds
In their original paper Giesy & Kannan (2001) reported significant levels of PFOS in birds (egg yolk, plasma and liver) from various places (see Table 6.8). The highest levels were found in blood plasma
from bald eagles collected in Midwestern USA (1-2570 ng PFOS/g ww). The levels were clearly higher in populated and industrialised areas than in remote locations. Levels in birds from Antarctica are
around or below the quantification limit.
PFOS was determined in 161 samples of liver, kidney, blood, or egg yolk from 21 species of fish-eating water birds from the USA and the remote Midway Atoll in the northern Pacific Ocean (Kannan et al.
2001b). Highest PFOS concentrations in blood plasma were from bald eagles collected in the Midwestern USA ranging from 13 to 2220 ng/mL (mean: 330 ng/mL). Concentrations were higher in blood
plasma than in whole blood. The highest liver-PFOS concentration (1780 ng/g ww) was found in a cormorant from San Diego. PFOS in blood serum from albatrosses collected at Midway ranged 3 to 34
ng/mL. In livers and kidneys from the albatrosses levels were below the quantification limit.
Livers from 83 birds collected from Japan and Korea were analysed. PFOS was found in 95% of the samples in levels up to 650 ng/g ww and concentrations were within the ranges reported from the USA
and Europe. Black-tailed gulls from Hokkaido, Japan, contained 2-12 ng PFOS/mL. PFOA and PFHxS were found in 5-10% of the samples in concentrations up to 21 and 34 ng/g ww, respectively.
Perfluorooctane sulfonamide (FOSA) was detected only in all common cormorants from the Sagami River in Japan in levels up to 215 ng/g ww. Highest levels of PFOS were also at that location. There was
no correlation between levels of PFOS and FOSA; the latter was only distributed locally. Levels of PFOS were lower in Korea compared to Japan (Kannan et al. 2002b).
In the Sardinian Sea in Italy cormorant livers contained 32-150 ng PFOS/g (mean: 61) and 29-450 ng PFOA/g (mean: 95); thus surprisingly PFOA was more abundant than PFOS. Levels of PFOSA and
PFHxS were lower than the quantification limit (Kannan et al. 2002c). In 44 white-tailed sea eagles collected near the Baltic coast of Poland and East Germany in the years 1979 to 2000, PFOS levels in
livers averaged 36 ng/g ww. There was an upward trend in levels during the years. Levels of PFOA, FOSA or PFHxS were below the quantitation limit.
PFOS levels in various birds living in Tokyo Bay were 68-1200 ng/g liver and 0.3-167 ng/mL blood (Taniyasu et al. 2003). In a few samples low levels of PFHxS were also found.
A recent study identified both PFOS and PFOA in biota from the Canadian arctic. Common loons, northern fulmars and black guillemots were collected at various locations. PFOS was the major
contaminant but common loon also contained significant levels of FOSA. In contrast to mammals and fish, perfluorinated carboxylic acids were not detected in birds. Levels were generally lower than levels
for similar birds in the USA (Martin et al. 2004).
In a recent Nordic screening project two pooled samples of fulmar eggs from Faroe Islands were investigated for perfluorinated compounds (Berger et al. 2004; Kallenborn et al. 2004). Levels were 33 and
40 ng/g ww. PFOS stood for 95% of the PFAS levels followed by PFNA and PFOSA.
Table 6.8: PFOA and PFOS in birds.
Bird species |
Location |
Tissue |
Number of samples |
PFOS concentration (ng/g ww) or for plasma /serum/blood
(ng/mL) |
Reference |
|
Range |
Mean |
Double-crested cormorant |
Lake Winnepeg |
Egg yolk |
4 |
130-320 |
210 |
Giesy & Kannan 2001 |
Double-crested cormorant |
Lake Huron |
Plasma |
6
3
|
1-270
110<-430
|
170
230
|
Herring gull |
2
2
|
66-79
280-450
|
73
370
|
Ring-billed gull |
Egg yolk |
3 |
<35-150 |
|
Bald eagle |
Midwestern USA |
Plasma |
26 |
1-2570 |
360 |
Polar skua |
Antarctica |
2 |
<1-1.4 |
|
Common Loon |
North Carolina |
Liver |
8 |
35-690 |
290 |
Brown pelican |
Mississippi |
2 |
290-620 |
460 |
Laysan and blackfooted albatrosses |
Midway Atoll |
Liver
Plasma |
9
3
10 |
<35
9-26
3-39 |
18
9
|
Common cormorant |
Italy |
Liver |
12 |
33-470 |
96 |
Black-tailed gull |
Korea |
15 |
70-500 |
170 |
|
Hokkaido Japan |
Plasma |
24 |
2-12 |
6 |
Double crested Cormorant |
Michigan |
Blood |
7 |
34-243 |
143 |
Kannan et al. 2001b |
|
Plasma |
4 |
63-372 |
185 |
Herring gull |
Plasma
Blood |
2 |
239-391
57-68
|
315
63
|
Ring-billed gull |
Egg yolk |
3 |
30-126 |
67 |
Double crested Cormorant |
Manitoba |
|
4 |
21-220 |
157 |
Bald eagle |
Midwestern USA |
Plasma |
33 |
<1-2220 |
320 |
Bald eagle |
West/Midwest USA |
Liver |
4 |
24-467 |
192 |
Black-crowned night heron |
San Diego, USA |
5 |
32-648 |
393 |
Brandt's cormorant |
2 |
46-1780 |
907 |
Brown pelican |
USA |
3 |
118-533 |
302 |
Common loon |
19 |
8.7-595 |
40-280 |
Double-crested cormorant |
Louisiana, USA |
2 |
51-288 |
169 |
Franklin's gull |
Montana, USA |
4 |
<12-61 |
40 |
Great black-backed gull |
North Carolina |
2 |
187-841 |
608 |
Great blue heron |
Louisiana |
2 |
162-916 |
539 |
Great egret |
USA |
7 |
27-1030 |
404 |
Herring gull |
5 |
16-353 |
186 |
Northern gannet |
North Carolina |
1 |
85 |
Osprey |
USA |
2 |
42-60 |
51 |
Red-throated loon |
3 |
34-1120 |
584 |
Snowy egret |
Florida |
2 |
99-413 |
256 |
White pelican |
California |
6 |
30-1120 |
270 |
White-faced ibis |
1 |
17 |
Wood stork |
South Carolina |
1 |
158 |
Black-footed albatross |
Midway Atoll |
Liver
Kidney
Serum |
8
5
8 |
<30 <30
3.0-17 |
6.2 |
Laysan albatross |
Kidney
Serum
|
3
7
|
<30
5.7-34
|
14 |
Cormorant |
Sardinia, Italy |
Liver |
12 |
32-150
29-450 PFOA
|
61
95
|
Kannan et al. 2002c |
White-tailed sea eagle |
East Germany and Poland |
Liver |
44 |
<3.9-127 |
36 |
Carrion crow (Corvos corone) |
Tokyo Bay area |
Liver
Blood
|
6
5
|
68-1200
11-150
|
464
56
|
Taniyasu et al. 2003 |
Mallard (Anas platyrhynchos |
Liver
Blood
|
1
1
|
493
130
9 PFHxS |
Pintail duck (Anas acuta) |
Liver
Blood
|
2
2
|
239-497
84-167
6-20 PFHxS |
368
126
13 PFHxS |
Sea gull (Larus crassirostris) |
Liver |
1 |
230 |
Black-eared kite (Milvus lineatus) |
1 |
180 |
Common cormorant (Phalacrocorax carbo) |
10 |
170-650 |
390 |
Domestic duck (Anas platyrhynchos) |
Blood
Serum
|
2
2
|
0.3-1
6-9
|
0.65
7.5
|
Common loon (Gavia immer) |
Canadian Arctic |
Liver |
5 |
11-26
2-13
FOSA |
20
5.9
FOSA |
Martin et al. 2004 |
Northern fulmar (Fulmarus glacialis) |
5 |
1.0-1.5 |
1.3 |
Black guillemot (Cepphus grille) |
5 |
Nd |
6.2.7.8 Aquatic mammals
In their original paper Giesy & Kannan (2001) reported significant levels of PFOS in aquatic mammals from various places (see Table 6.9). The highest levels were found in mink (970-3680 ng PFOS/g
ww) and river otters (34-990 ng/g ww) from the western USA. High levels were also found in Alaskan polar bear (180-680 ng/g ww) and seals (16-230 ng/g ww) from the Baltic Sea and in dolphins
(65-430 ng/g ww) from the Mediterranean Sea. The levels were clearly higher in populated and industrialised aresa than in remote locations. Levels in animals from Antarctica are around or below the
quantification limit.
Kannan et al. (2001a), investigated 247 tissue samples (liver and blood) from 15 species of marine animals collected from Florida, California and Alaskan coastal waters, the northern Baltic Sea, the Arctic
(Spitzbergen) and Sable Island in Canada. In seals from the Canadian and Norwegian arctic the blood levels were 3-50 ng PFOS/mL. The highest PFOS concentration in livers was 1520 ng/g ww in a
stranded bottlenose dolphin from Sarasota Bay in Florida, and the highest concentration in blood was 475 ng/mL in a ringed seal from the Bothnian Bay. Ringed seals were more contaminated than grey
seals. (The same is occurring for PCB and dioxin in the Baltic, and it is explained by the fact that ringed seals eat more bottom living organisms). No age-dependent increase was observed. A few brain and
kidney samples contain non-quantifiable levels of PFOS.
In dolphins from the Mediterranean Sea blood levels of FOSA (mean 223 ng/g ww) were surprisingly greater than levels of PFOS (mean: 143 ng/g ww), which again were much higher than levels of PFOA
(mean: 3.1 ng/g ww) and PFHxS (mean: 4.5 ng/g ww). Pilot whales had also high levels of both PFOS and FOSA (Kannan et al. 2002c). For seals from the northern Baltic Sea levels of PFOS in the livers
were very high and up to 1100 ng/g ww. Levels in ringed seals were about the double of levels in grey seals.
Another study of Mediterranean dolphins has recently been published by Corsolini & Kannan (2004). The samples were from 1991-1998 and from around Italy. The blood from captive bottlenose dolphins
contained 42-210 ng PFOS /mL and livers from similar dolphins but wild-living animals contained PFOS on the same scale. Levels in striped dolphin livers were about the half, while muscle and liver tissues
from a common dolphin had 77 and 940 ng PFOS/g ww, respectively. Most of the highly contaminated dolphins contained also considerable levels of FOSA.
Van de Vijver et al. (2003) studied perfluorinated chemicals in livers and kidneys from mammals stranded along the southern North Sea coast. PFOS was predominant in terms of concentration, and the
highest concentrations were found in mammals and displayed the highest trophic position. Levels were mostly higher in liver compared to kidney. Harbour seal levels were higher compared to white beaked
dolphin > harbour porpoise > grey seal > sperm whale > white-sided dolphin > striped dolphin > fin whale/hooded seal. In 10% of the samples levels were below the detection limit of 10 ng PFOS/g wet
weight. Juvenile porpoises contained more PFOS than adult animals, and there were no significant sex differences. Thus PFOS is not depleted during lactation as lipophilic POP chemicals.
Perfluoroundecanoic acid (PFUA) was determined in the liver from a single harbour porpoise (110 ng/g ww), a sperm whale (50 ng/g ww), a white-sided dolphin (60 ng/g ww) and in three white-beaked
dolphins (50-150 ng/g ww). Perfluorononanoic acid (PFNA) was found in a sperm whale (240 ng/g ww) and a white-beaked dolphin (480 ng/g ww) only. Perfluorodecanoic acid (PFDeA) was found in
three white-beaked dolphins (90-120 ng/g ww). PFOA and perfluorododecanoic acid (PFDoA) were not detected.
In Frobisher Bay in the Eastern Canadian arctic the highest levels of PFOS (8.7-14.3 ng/g ww) were found in beluga whales. Narwhales had also rather high PFOS levels, while FFOA levels in whales were
one-tenth of PFOS levels (Tomy et al. 2003).
Contamination with perfluorinated chemicals has been measured in livers from wild mink and river otters in the USA (Kannan et al 2002d). PFOS was detected in all mink samples, while PFOA, FOSA and
PFHxS were detected only in a few samples. Mink from Midwestern USA (Illinois) contained greater PFOS concentrations in their livers (up to 5140 ng/g ww) than mink from South Carolina (up to 3110
ng/g ww), Louisiana (up to 320 ng/g ww) and Massachusetts (up to 1100 ng/g ww)). A laboratory study with mink, where carps from a polluted river were substituted for marine fishes at levels of 10, 20
and 40% in the fed, showed a dose-related sharp increase in PFOS concentrations of the livers. FOSA, which was not detectable in the carps, was measurable in the mink livers. PFOS was also measured
in all 20 liver samples (up to 994 ng/g ww) from river otters living in the states of Washington and Oregon, USA. FOSA was measured in 90% of the samples (up to 72 ng/g ww). PFOA (up to 19 ng/g ww)
and PFHxS (up to 76 ng/g ww) were only detected in a few samples.
A recent study identified both PFOS and PFOA in biota from the Canadian arctic. Polar bears, ringed seals, arctic fox, and mink were collected at various locations. PFOS was the major contaminant but all
samples contained also perfluorinated carboxylic acids (PFCA) ranging in length from 9 to 15 carbons. In all samples except for minks PFOS dominated. In minks perfluorononanoate (PFNA) was highest.
Mammals feeding at higher trophic levels had greater contamination. Highest mean levels were 3100 ng PFOS/g, 180 ng PFNA/g and 8.6 ng PFOA/g in polar bears. In general, odd-length PFCAs
exceeded the concentration of even-length PFCAs and concentration decreased with increasing chain length. Levels were generally lower than levels for similar animals in the USA (Martin et al. 2004).
In a recent Nordic screening project a few samples of marine mammals from Denmark, Sweden, Iceland and Faroe Islands were investigated for perfluorinated compounds (Berger et al. 2004; Kallenborn
et al. 2004). Grey seals from Sweden and harbour seals from Denmark were most contaminated with PFOS as dominating substances (up to 1 μg/g ww). Minke whales from Iceland were lower
contaminated than pilot whales from Faroe Island. In pilot whales PFOSA levels were sometimes exceeding PFOS levels.
Table 6.9: PFOA and PFOS in marine mammals.
Mammal |
Location |
Species |
Tissue |
Number of samples |
PFOS concentration (ng/g
ww) |
Reference |
Range |
Mean |
Ringed seal |
Canadian arctic |
|
Plasma
(ng/ml) |
24 |
<3-12 |
|
Giesy & Kannan 2001 |
Baltic Sea |
|
18 |
16-230 |
110 |
Norwegian arctic |
|
18 |
5-14 |
9 |
Gray seal |
Baltic Sea |
|
26 |
14-76 |
37 |
Canadian arctic |
|
12 |
11-49 |
28 |
Sea lion |
California |
|
Liver |
6 |
<35-49 |
|
Elephant seal |
|
5 |
<35 |
|
Harbor seal |
|
3 |
<35-57 |
|
Northern furseal |
Alaska |
|
14 |
<35-120 |
|
Weddel seal |
Antarctica |
|
1 |
<35 |
|
River Otter |
NW USA |
|
5 |
34-990 |
330 |
Sea otter |
California |
|
8 |
<35 |
|
Bottlenose dolphin |
Mediterranean Sea |
|
5 |
170-430 |
270 |
Striped dolphin |
|
4 |
65-160 |
100 |
Ganges River dolphin |
India |
|
2 |
<35-81 |
|
Mink |
Midwestern USA |
|
18 |
970-3680 |
2630 |
Polar bear |
Alaska |
|
17 |
180-680 |
350 |
Pygmy sperm whale |
Florida coastal waters (stranded) |
|
Liver |
2 |
6.6-23 |
14.8 |
Kannan et al. 2001a |
Short-snouted spinner dolphin |
|
3 |
79-168 |
123 |
Striped dolphin |
|
2 |
36-388 |
212 |
Rough-toothed dolphin |
|
2 |
42-66 |
54 |
Bottlenose dolphin |
|
20 |
48-1520 |
489 |
Sea Lion |
West Coast USA |
|
Liver |
6 |
4.6-49 |
27 |
Elephant seal |
|
5 |
<5-9.8 |
9.3 |
Harbor seal |
|
3 |
10-57 |
27 |
River Otter |
|
5 |
33-994 |
329 |
Sea Otter |
|
Liver
Brain
Kidney |
8
2
3 |
<5-9.8 <35 <35 |
8.9 |
Northern fur seal |
Alaska |
|
Liver |
13 |
<10-122 |
38 |
Polar bear |
|
17 |
175-678 |
350 |
Northern fur seal |
Alaskan Coastal waters |
|
Blood (ng/mL) |
25 |
<6 |
|
Polar bear |
|
14 |
26-52 |
34 |
Steller sea lion |
|
12 |
<6 |
|
Ringed seal |
Bothnian Bay |
|
29 |
|
92-242 |
Spitsbergen |
|
18 |
|
8-10 |
Grey seal |
Bothian Bay |
|
26 |
|
25-44 |
Sable Island |
|
12 |
|
28 |
Bottlenose dolphin |
Italy coast |
|
Blood
ng/mL |
4 |
42-210 <2.5-3.8 PFOA
190-270 FOSA <1-6.1 PFHxS |
143
3.1 PFOA
223 FOSA
4.5 PFHxS |
Kannan et al. 2002c |
|
Liver |
6 |
<1.4-110 <72 PFOA
30-139 FOSA <19 PFHxS |
64
63 FOSA |
Striped dolphin |
|
|
4 |
16-40 <72 PFOA <38 FOSA <7 PFHxS |
26 |
Common dolphin |
|
Liver
Muscle |
1
1 |
940 <38 PFOA
878 FOSA <19 PFHxS
77 <38 PFOA
142FOSA <19 PFHxS |
|
Fin whale |
|
Muscle |
1 |
<19 |
|
Long-finned pilot whale |
|
Liver
Muscle |
1
1 |
270 <38 PFOA
50 FOSA <19 PFHxS
52 <38 PFOA
48 FOSA <19 PFHxS |
|
Grey seal |
Bothnian Bay |
|
Liver |
27 |
140-360 <19 PFOA <19-47 FOSA <7.5 PFHxS |
214
42 FOSA |
Ringed seal |
|
|
|
25 |
130-1100 <19 PFOA <19 FOSA <7.5 PFHxS |
454 |
Mink |
Illinois |
|
Liver |
65 |
47-5140 <37-590 FOSA |
1177
65 |
Kannan et al. 2002d |
Massachusetts |
|
|
31 |
20-1100 |
142 |
South Carolina |
|
|
9 |
650-3110 |
1065 |
Louisiana |
|
|
7 |
40-320 |
140 |
River Otter |
Washington |
|
|
10 |
25-422 |
183 |
Oregon |
|
|
10 |
34-994 |
421 |
Harbour porpoise |
Belgian/Dutch Coast |
Phocoena phocoena |
Liver
Kidney |
48
43 (37) |
12-395 <10-821 |
93 |
Van de Vijver et al. 2003 |
Harbour seal |
Phoca vitulina |
Liver
Kidney |
24 (22)
22(21) |
<10-532 <10<-489 |
|
Grey seal |
Halichoerus grypus |
Liver
Kidney |
6
6 |
11-233
23-167 |
88
81 |
Sperm whale |
Physeter macrocephalus |
Liver
Kidney |
6
5 (1) |
19-52
12 |
36 |
White-beaked dolphin |
Lagenorhynchus albirostris |
Liver
Kidney |
7
7 |
14-443
13-290 |
132
87 |
White-sided dolphin |
Lagenorhynchus acutus |
Liver
Kidney |
2 (1)
1 |
26
18 |
|
Striped dolphin |
Stenella coerulealba |
Liver
Kidney |
2
2 (0) |
11 <10 |
|
Beluga whale |
Frobisher Bay, Canadian Eastern arctic |
|
Liver |
5 |
8.7-14.3 |
11
2.6 PFOA
2.5 FOSA
0.05 EtFOSA |
Tomy et al. 2003 |
Narwhale |
|
3 |
3.9-16.2 |
9
1 PFOA
0.05 EtFOSA |
Walrus |
|
5 |
|
2.4
0.5 PFOA |
Polar bear |
Arctic Canada |
Ursus maritimus |
Liver |
7 |
1700->4000
2.9-13 PFOA
108-230 PFNA |
3100
8.6 PFOA
180 PFNA
56 PFDA
63 PFUnA
6.2 PFDoA
11 PFTrA
12 FOSA |
Martin et al. 2004 |
Arctic Fox |
Alopex lagopus |
10 |
6-1400
2-86 PFNA
6-1400 FOSA |
250
22 PFNA
19 FOSA |
Ringed seal |
Phoca hispida |
19 |
8-37
2.4-8.8 PFNA |
18
5.4 PFNA |
Mink |
Mustela vision |
10 |
1.3-20
2-35 PFNA |
8.7
16 PFNA |
6.2.8 Levels in terrestrial animals
Wild wood mice (Apodemus sylvatica) captured in a nature reserve close by a fluorochemicals factory in Belgium had extremely high PFOS levels in the livers ranging from 470-17,855 ng/g ww with a
median of 506 ng/g ww and mean of 2618 ng/g ww (Hoff et al. 2004). Wood mice living and captured 3 km away had hundred times lower average levels (mean: 280 ng/g ww). The result suggested sex
independence of PFOS levels, increased levels in older mice, and maternal PFOS transfer to the young.
PFOS levels in blood serum from two pet rabbits from Tokyo ranged 6-9 ng/mL (Taniyasu et al. 2003).
The accumulation of 13 different perfluorinated chemicals (PBFS, PFHxS, PFOS, PFPeA, PFHxA, PFHpA, PFOA, PFNA, PFDeA, PFUnA, PFDoA, PFOSA, THPFOS) was studied in cattle from
Japan by analysis of blood plasma (Guruge et al. 2004). There was no clear age-dependency, and the levels were on that scale lower than in fish.
6.3 Human exposure
The pathways leading to human exposure to PFOS and PFOA are not well known. Protections of clothes and carpets are among the most important uses of these chemicals and precursors. Thus exposure
to dust indoor may be important. Vacuum cleaner dust from Japanese homes also contained between 11 and 2,500 ng PFOS/g dust (mean: 200 ng/g; median: 24.5 ng/g) and between 69 and 3,700 ng
PFOA/g dust (mean: 380 ng/g; median: 165 ng/g), respectively. PFOA levels were in general higher than PFOS levels. The highest concentrations of both were in the same sample, and there was an
association between PFOS and PFOA in all samples; an indication of a similar origin (Moriwaki et al. 2003).
The daily intake of PFOS from air exposure in Japan was calculated to 10-100 pg/day, which would result in 1.2-12 ng/L excess of plasma PFOS levels (Sasaki et al. 2003).
Food intake may be another exposure route. N-Ethylperfluorooctane sulfonamide (N-EtFOSA) is present (or at least has been present [13]) in some oil- and water repellent coatings for paper and
paperboard used in food packaging. A Canadian study of fast food composites collected before 2000 revealed that more than 55% of the composites contained this chemical (detection limit: 10 pg/g). The
highest level measured (23,500 pg/g) was in a pizza. PFOS was also detected in three samples. Samples after 2000 were free of these contaminants (Titlemeier et al. 2003).
A Japanese study has shown that consumption of drinking water obtained from a polluted river may lead to a significantly increased daily intake of 0.2-1 mg PFOS/day and may contribute 8-16 μg PFOS/L
to blood serum levels and result in a 25-50% rise in normal levels (Harada et al. 2003).
6.4 Levels in humans
Perfluorochemicals in human blood have for decades been detected in occupational exposed individuals. In a worker handling the ammonium salt of PFOA the blood contained up to 71 g organic fluorine/ml
blood (Ubel et al. 1980). Serum PFOS concentrations among production workers in PFOS-related processes averaged 0.5-2 ppm (mg/L) and ranged <0.1-12 ppm (Olsen et al. 2003). Normally,
exposure to the stable fluoropolymers will not give rise to increased free fluoride concentration in the blood.
Human samples from 1998-2000 showed average PFOS levels of 17-53 ng/mL for PFOS and 3-17 ng/mL for PFOA (OECD 2002). Mean concentration in 645 sera samples from six different states in
the USA was 34.9 ng/mL (range: <4.3-1660 ng/mL) (Olsen et al. 2003b). Thus occupational levels may be 100-1000 times higher than levels in the general population.
In 65 commercial available human serum samples the average level of PFOS was 28.4 ng/mL with a range of 6.7-81.5 ng/ml. PFOA and PFHxS were quantified in half of the samples and PFOSA only in
one sample and at much lower levels (Hansen et al. 2001).
Blood serum and livers in 31 American blood donors were analysed for fluorochemicals by Olsen et al. (2003a). Liver PFOS concentrations ranged between <4.5 to 57 ng/mL, and serum PFOS ranged
between <6.1 to 58.3. PFOS was determined in all but one serum sample. However, in about half of the liver samples levels were below the limit of quantification. The means of the positive samples were
28.0 ng/g ww for liver and 18.3 ng/mL for serum. Among the 23 human donors with paired mean liver and serum PFOS concentrations were 20.8 ng/g (ppb) and 18.2 ng/ml (ppb), respectively. The mean
serum to liver ratio was 1.3. Only a few sample had levels of FOSA and PFHxS above the quantification limits, and only 1 liver was found with measurable amount of PFOA. In blood serum the mean levels
for PFOA, FOSA and PFHxS were 3.1, 4.5, and 2.4 ng/mL, respectively.
A larger study characterised PFOS and six other related fluorochemicals in serum samples taken in 2000-2001 from a 645 adult American blood donors from cities in six states (Olsen et al. 2003b). The
levels ranged from the lower limit of determination of 4.1 ppb (ng/mL) to 1,656 ppb with a geometric mean of 34.9 ppb. The mean was a little higher among males than females but no substantial difference
was observed by age. The levels of the other fluorochemicals were on that scale lower. The mean of PFOA was 4.3-5.3 ppb (Olsen et al. 2003b).
In a recent study of an elderly population (ages 65-96) PFOS in the blood ranged <3.4-175 ng/mL with a geometric mean of 31 ng/mL (Olsen et al. 2004). This is inside the normal range (30-40 ng/mL) for
non-occupational exposed individuals. The most elderly had slightly lower concentrations but no differences between sexes were noted (Olsen et al. 2004). In the same study levels of PFOA, PFHxS,
PFOSAA and M570 ranged respectively <1.4-16.7 ng/mL (mean: 4.2), <1.4-40.3 ng/mL (mean: 2.2), <1.6-21.1 (mean: 1.5) and <1.0-6.6 (mean: 1.2).
A study of historic and recent serum samples from Japan revealed that the levels were influenced by several factors (Harada et al. 2004). Sex and residential area are the most influential factors, while age
and smoking status are not so important. In general males had 50-100% higher concentrations than females. The PFOS and PFOA mean levels in sera in 2003 ranged from 3.5 to 28.1 μg/L and from
2.8-12.4 μg/L, respectively. Highest levels of PFOS and PFOA were measured in Kinki, an area with known contamination of surface water. Historic data seems to indicate that levels of PFOS reach a
maximum in the mid 1990's while PFOA concentrations are still increasing.
Perfluorinated compounds were investigated in maternal and in cord blood in 15 pregnant Japanese women (Inoue et al. 2004). PFOS concentrations in maternal samples ranged from 4.9 to 17.6 μg/L,
whereas those in foetal samples ranged from 1.6 to 5.3 μg/L, or one-third of maternal levels. In contrast PFOSA was not detected in all samples while PFOA was determined in 3 out of 15 samples ranging
0.5-2.3 μg/L.
The presence of perfluorinated compounds in 23 archived pooled samples of blood plasma from females of the northern population in Canada was studied by Tittlemier et al (2004). Average levels were
36.9 ng PFOS/mL and 2.2 ng PFOA/mL. All samples also contained PFNA (0.11-1.98 ng/mL), while 70% of the samples contained detectable levels of PFHpA and only one sample contained PFHxA.
Levels in cord blood plasma were about the half of maternal blood. There was no difference between levels in Inuit and Caucasian people as there are for PCB and dioxin, thus the source of exposure may
not be food.
In a recent study from Sweden 66 whole blood samples from the general population were analysed for 12 perfluorinated compounds with chain length 4 to 14 (Kärmann et al. 2004). PFOS, PFOA,
PFOSA, PFHxS and PFNA were found in 92-100% of the samples, while traces of PFDeA and PFDoA were found in 65% of the samples. Besides this, PFDeS, PFHxA, PFDoA and PFTeA were
detected in 3-8% of the samples. Levels of PFOS dominated with a range of 1.7-37 ng/mL and a mean of 18.2 ng/mL. The mean sum of all perfluorinated compounds was 24.6 ng/ml.
Rather high levels of perfluorinated chemicals were found in blood and semen from Sri Lanka (Guruge et al. 2004). The levels in the capital Colombo were a little higher than in a rural area. Levels in semen
were ten times lower than in serum. Maximum serum concentrations were 18 ng PFOS/mL and 23 ng PFOA/mL.
PFOS and other perfluorinated chemicals were determined in blood serum from the general population of the USA, India and Italy by Kannan et al. (2003). PFOS levels (mean: 32 ng/mL) in Americans are
7-8 times higher than in Italians who again have twice the levels of Indians. In the majority of samples from the USA PFHxS, PFOA and PFOSA were also detected (means: 3.9, 5.1, and 3.4 ng/mL,
respectively). In contrary, one third of the samples from Italy and India had detectable levels of PFHxS while PFOA and PFOSA only were detectable in a few or zero samples. The concentration of PFNA
and PFDA was between 0.5 and 1 ng/mL. No variation in sex or age was seen.
In a recent study Kannan et al. (2004) reported results of perfluorinated compounds in blood from additional countries. In all countries besides Korea PFOS was far the dominating chemical with means
ranging from 66 ng/mL in Kentucky, USA, to 2.3 ng/mL in India. In Korea mean concentration of PFOA (88 ng/mL) in females was higher than that of PFOS (15 ng/mL). Curiously, PFOA was only
determined in 19-25% of the samples. This may be caused by individual exposure to a local contamination. These data from Korea were also published separately by Yang et al. (2004), who analysed 50
whole blood samples from Daegu, the fourth largest city in Korea.
Figure 6.1: Perfluorinated chemicals in human blood from various countries (Kannan et al. 2004; OECD 2002; Kärmann et al. 2004)
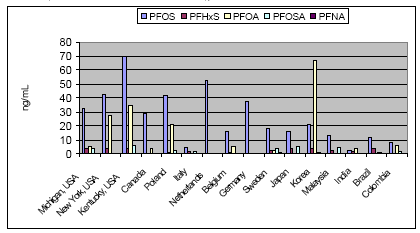
Table 6.10: Perfluorochemicals in human samples.
Chemicals |
Country |
Sample type |
No. of samples (% pos) |
Range (g/L; ng/g ww) |
Average (g/L; ng/g ww) |
Reference |
PFOS |
USA |
Serum |
65 |
7-82 |
28.4 |
Hansen et al. 2001 |
PFOA |
65 (49%) |
<5-35 |
6.4 (estimate) |
PFHxS |
65 (46%) |
<5-21 |
6.6 (estimate) |
PFOSA |
65(0.02%) |
<1-2 |
|
PFOS |
Netherlands |
Serum |
6 |
39-61 |
53 |
OECD 2002 |
Belgium |
5 |
4.9-22 |
17 |
Germany |
6 |
32-46 |
37 |
PFOS |
Canada |
Serum |
|
|
29 |
Kubwabo et al 2004; cit.Tittlemier et al. 2004 |
PFOA |
|
|
3.4 |
PFOS |
Japan |
Blood
Serum |
10
3 |
5-14
19-41 |
11
27 |
Taniyasu et al. 2003 |
PFHxS |
Blood |
5 |
<1-3.8 |
2.0 |
PFOS |
USA |
Liver
Serum |
23
23 |
paired samples |
20.8
18.2 |
Olsen et al. 2003a |
Liver
Serum |
30 (43%)
25 (96%) |
<4.5-57.0
2.4-58.3 |
28.0 (of samples >LOQ)
18.3 |
PFOS |
Michigan USA |
Serum |
75 (93%) |
<1.3-124 |
32.7 |
Kannan et al 2003 |
PFHxS |
75 (76%) |
<1.3-13.6 |
3.9 |
PFOA |
75 (45%) |
<1.3-14.7 |
5.1 |
PFOSA |
75 (52%) |
<1.3-23.5 |
3.4 |
PFOS |
Siena, Italy |
50 (91%) |
<1-10.3 |
4.3 |
PFHxS |
50 (33%) |
<1-2.1 |
1.6 |
PFOA |
50 (0%) |
<3 |
|
PFOSA |
50 (9.5%) |
<1.3-2.3 |
1.8 |
PFOS |
Coimbatore, India |
45 (50%) |
<1-3.1 |
1.8 |
PFHxS |
45 (41%) |
<1-2.9 |
1.6 |
PFOA |
45 (3%) |
<3-3.5 |
3.5 |
PFOSA |
45 (0%) |
<3 |
|
PFOS |
USA
Six states |
Serum |
645 (99%) |
<4.3-1,656 |
34.9 |
Olsen et al. 2003b |
PFOA |
645 (92%) |
<1.9-52.3 |
4.6 |
PFHxS |
645 (52%) |
<1.4-66.3 |
1.9 |
PFOSAA |
645 (42%) |
<1.6-60.1 |
2.0 |
M570 |
645 (40%) |
<1-16.4 |
1.3 |
PFOS |
USA |
Serum |
238 |
<3.4-175 |
31.0 |
Olsen et al. 2004 |
PFOA |
<1.4-16.7 |
4.2 |
PFHxS |
<1.4-40.3 |
2.2 |
PFOSAA |
<1.6-21.1 |
1.5 |
M570 |
<1.0-6.6 |
1.2 |
PFOS |
Japan |
Serum |
205 |
3.5-28.1 (means) |
10.4 |
Harada et al. 2004 |
PFOA |
2.5-12.4 (means) |
4.1 |
PFOS |
Japan |
Maternal blood |
15 |
4.9-17.6 |
8.8 |
Inoue et al. 2004 |
Cord blood |
15 |
1.6-5.3 |
2.8 |
PFOA |
Maternal blood |
15 (20%) |
0.5-2.3 |
1.1 |
6.5 Animal toxicity
The effects of PFOA on biological systems have been extensively studied in rodents (Kennedy et al. 2004). PFOA causes peroxisome proliferation as well as induction of various enzymes involved in lipid
metabolism. Toxic effects have been reported, such as induction of fatty liver and uncoupling of the mitochondrial respiratory chain. PFOA also affects the serum levels of various hormones, i.e. reducing
testosterone, and increasing estradiol in rats.
Subchronic exposure of animals to PFOS may lead to significant weight loss accompanied by hepatotoxicity and reduction of serum cholesterol and thyroid hormones.
When evaluating animal studies it has to be taken into account that control animals can be exposed to the general PFOS contamination through fish-based feedstuff.
6.5.1 Toxicokinetics and metabolism
6.5.1.1 Absorption
PFOS is readily absorbed in the body (Lau et al 2004), as is PFOA (Kennedy et al 2004).
6.5.1.2 Transformation
PFOA and PFOS are both considered being metabolically inert (Clark et al. 1973). Other PFCAs and PFASs do have similar properties, and their functional derivatives may be transformed to PFCAs and
PFASs. For example, the fluorotelomer alcohol 8:2 FTOH is transformed to PFOA in rats (Hagen et al. 1981).
6.5.1.3 Accumulation
PFOA and PFOS may bind to proteins and accumulate in various body tissues, including blood and liver of exposed organisms. PFOS also accumulates in the testis and brain (Vanden Heuvel et al 1992;
Austin et al. 2003).
In the blood these chemicals are bound to serum proteins, mainly albumin but also sex-hormone binding globulins and corticosteroid-binding globulins, which regulate and maintain the steady state of free
hormones in the blood. The potential of perfluorinated carboxylic acids (PFBA, PFOA, PFDA) and sulfonic acids (PFBS, PFHxS, PFOS) of varying chain length to displace hormones from specific serum
binding protein (receptor) in blood serum from carps, male fowl and bald eagle was studied by Jones et al. (2003b). In carp serum a very small displacement of testosterone was seen for PFOS, PFBA,
PFOA, and PFDA but not for PFBS and PFHxS. In bird serum corticosterone was slightly displaced with increasing carbon chain length and the sulfonic acids were more potent than the carboxylic acids.
Under environmentally relevant conditions no effect will appear.
6.5.1.4 Elimination
Once absorbed in the body PFOA is eliminated as the free carboxylic acid mainly with urine and to a less extent in faeces. Thus the renal elimination is critical for detoxification (Vanden Heuvel et al. 1991).
The biological half-life of PFOA in male rats (5.6 days) is 70 times longer than that in female rats (2 hrs). The difference is mainly due to the difference in renal clearance, which was significantly reduced by
probenezid, suggesting that PFOA is excreted by organic anion transporters. Castration of male rats caused a 14-fold increase in renal clearance, comparable with female rats, which again was reduced by
treatment with testosterone. Treatment with estradiol also increased the renal clearance. In female rats ovariectomy increased the renal clearance (Kudo et al. 2002).
Perfluorocarboxylic acids (PFCA) with a shorter carbon chain length than PFOA are more rapidly eliminated in urine and PFHpA (0.10/0.05) > PFOA (5.6/0.08) >PFNA (30/2.4) >PFDA (40/59 days)
for male/female rats (Kudo et al. 2001; Ohmori et al. 2003). The sex differences were most marked for PFOA.
The half-life of PFOS in the monkey was about 200 days (Seacat et al. 2002), and the half-life of PFOA in monkeys is about 1 month (Burris et al. 2002).
6.5.2 Animal toxicity
The oral rat LD50 for PFOS is 251 mg/kg bw (US EPA 2000). For PFOA and PFDA the rat intraperitoneal LD50's are 189 and 41 mg/kg, respectively. Thus PFDA is much more toxic and do have
delayed effects (Olson and Anderson 1983). The rat oral LD50 for PFOA is between 430 and 1800 mg/kg (Kennedy et al. 2004).
Female rats were injected intraperitoneal with 0, 1 and 10 mg PFOS/kg bw for two weeks. The PFOS exposure decreased food intake and body weight in a dose-dependent manner (Austin et al. 2003).
6.5.2.1 Liver toxicity
Several toxicological studies have demonstrated that the liver is the primary target organ for PFOS and PFOA. Liver cell hypertrophy and reduction in serum cholesterol are early responses to PFOS in
experimental animals. Kudo & Kawashima (1997) found that fish oil-feeding prevents PFOA induced fatty liver in mice.
In an in vitro test various perfluorinated chemicals were tested for interference with the liver-fatty acid binding protein (L-FABP). The most potent chemical was PFOS followed by EtFOSA, EtFOSE, and
PFOA (Luebker et al. 2002). This interference may contribute to the toxicity of these chemicals.
In a sub-chronic dietary toxicity study of potassium perfluorooctane sulfonate rats were exposed to up to 20 ppm for 14 weeks (Seacat et al. 2003). Decreased serum glucose and serum cholesterol
increased liver weight and alanin transferase (ALT) activity, hepatocytic hypertrophy and cytoplasmic vacuolisation were observed. Serum and liver PFOS concentrations were proportional to dose, and
serum concentrations were higher in female than in males. The serum to liver PFOS ratios ranged from approx. 3:1 to 12:1. The no-observed-adverse effect level (NOAEL) was 5 ppm corresponding to 44
ppm (mg/L) in males and 64 ppm in females and mean liver PFOS concentration of 358 ppm (mg/kg) and 370 ppm.
PFOS concentrations associated with the no adverse effect (0.15 mg/kg/d) in a 6-month monkey study were 59-70 mg/kg in the liver and 67-83 mg/L for serum (Seacat et al. 2002a). Rats seem to tolerate
somewhat higher liver concentrations of PFOS than monkeys.
PFOA is considerably less reactive than PFOS (PFOA LOEL of 3 to 10 mg/kg/d), and the target is different (Butenhoff, 2002). The only site of change in the monkey was liver enlargement at the doses
cited.
6.5.2.2 Peroxisome proliferation
Peroxisome proliferation is a well-known toxicological mechanism in rodents but not in humans. It may cause lipid accumulation in the liver, uncoupling of the mitochondrial oxidative phosphorylation, and
reduction of thyroid hormone in circulation. Many hypolipidemic drugs, solvents and environmental chemicals can cause peroxisome proliferation, for example Clofibrate, DEHP, chloroform,
perchloroethylene, trichloroethylene, HFC-123, and MTBE.
Perfluorocarboxylates (PFCA), particularly PFOA and PFDA, are highly potent peroxisome proliferators in rodent livers and affect mitochondrial, microsomal, and cytosolic enzymes and proteins involved
in lipid metabolism (Ikeda et al. 1985; Vanden Heuvel 1996; Upham et. al. 1998). PFOA is a peroxisome proliferator in rodents, whereas PFOS is much less reactive in rodents, but EtFOSE has no effect
(Berthiaume and Wallace 2002). Peroxisome proliferation is a less relevant endpoint to humans. The PFOS derivatives FOSA and EtFOSA may also uncouple the oxidative phosphorylation (Schnellmann &
Manning, 1990)
Administration of PFOA (0.0025-0,04% ww) and PFDA (0.00125-0.01%) in the feed for a week to male rats induced various enzyme systems and significantly increased accumulation of fats in the liver,
increased cell size and proliferation of peroxisomes in a dose-dependent manner. At highest exposure concentrations PFDA was more liver toxic than PFOA (Kawashima et al. 1995).
Increase in hepatic fatty acid -oxidation activity is a biochemical measure of peroxisome proliferation. Kudo and co-workers (2001) studied PFCAs with different chain length (C7, C8, C9 and C10) in male
liver. The result was that the liver concentration and not the chain length was decisive, but the longer the chain the more of the compound was accumulated in the liver.
In vitamin A deficient mice, PFOA had a stronger effect and caused a 3-6 times increase in the β-oxidation of fatty acids (Sohlenius et al. 1995).
6.5.2.3 Cellular effects
Some of the observed effects of perfluorinated compounds may be due to alterations in cell membrane fluidity, which is a measure of the relative mobility of the phospholipid bilayer of the cell membrane. The
selectively permeable cell membrane forms the first barrier that separates the cell from exogenous exposures. Effects on the permeability status of the cell membrane could play an important role in mediating
the adverse effects of a number of environmental contaminants.
In some in vitro assay systems PFOS - but not PFBS and PFHxS – significantly increased in a dose-dependent manner membrane fluidity of fish leukocytes, and decreased mitochondrial membrane
potential determined by flow cytometry. PFOS alone did not induce cytochrom P450 1A1 activity but at co-exposure of cells to TCDD ("dioxin") and PFOS the cytochrom P450 1A1 activity induced by
TCDD was increased (Hu et al. 2003).
Gap junction intercellular communication (GJIC) is the major pathway of intracellular signal transduction, and it is thus important for normal cell growth and function. Defects in this communication may lead
to teratogenesis, neuropathy, infertility, diabetes, autoimmune disorders, cancer, and other diseases (Trosko et al. 1998).
Upham and co-workers (1998) showed that perfluorinated carboxylic acids with carbon chain length of 7-10 can rapidly and reversibly inhibit gap junction intercellular communication in a dose-dependent
manner in vitro and with PFDA inhibiting more than PFOA.
In in vitro and in vivo test systems PFOS, PFOSA, and PFHxS – but not PFBS - inhibit gap junction intercellular communication in a dose-dependent fashion, and this inhibition occurred rapidly and was
reversible (Hu et al. 2002).
Cellular effects such as cell membrane fragility and gap junction communication are but two of the hypothetical explanations for the effects of these molecules. These are not the hallmark effects, such as
interference with liver function or lipid metabolism.
6.5.3 Mutagenicity
Ammonium perfluorooctanoate was found to be non-mutagenic in an Ames test using five strains of Salmonella typhimurium and in a single strain of Saccharomyces cerevisiae (Griffith and Long 1980).
Several other mutagenicity studies of PFOA published by contract laboratories supports the inactivity of PFOA (Kennedy et al. 2004). No result of mutagenicity testing of PFOS was found in the open
literature.
6.5.4 Cancer
Leydig cells in the testis are the main sites for testosterone biosynthesis. In animal studies with CD rats, a strain that has a low spontaneous incidence of these tumours, PFOA produced a dose-dependent
increase in Leydig cell adenomas, and PFOA modified Leydig cell steroidogenesis in vitro (Biegel et al. 1995; Liu et al. 1996). The tumours may be a result of endocrine changes, because a reduced
aromatase activity and a sustained increase in serum estradiol were observed (Biegel et al. 2001). However, US Environmental Protection Agency classifies PFOA as a carcinogen in animals (US EPA
2000).
A finished two-years feeding study with PFOS has only been briefly reported (Seacat et al. 2002b). A modest liver tumour response was observed in the high dose group (20 ppm PFOS potassium salt).
EtFOSE, for which 20% of an oral dose is converted to PFOS, has already been studied and a dietary dose of 100 ppm caused increase of hepatocellular adenomas in females and thyroid follicular cell
adenomas in males (Thomford et al. 2002).
6.5.5 Impacts on reproduction and development
Teratological studies have been conducted in rat, rabbit and mouse with potassium and lithium salts of PFOS (Lau et al. 2003; 2004). The findings are in agreement between laboratories and across species
examined, and are generally unremarkable, when maternal effects are taken into consideration. Observed developmental effects include reduction of foetal weight, cleft palate, oedema, delayed ossification of
bones, and cardiac abnormalities. These structural abnormalities were found in the highest PFOS dose groups, where significant reductions of weight gain and food consumption were also observed in the
pregnant dams.
The no-observed-effect-level (NOEL) for PFOS in rabbits was 0.1 mg/kg/d (Case et al. 2001).
High doses of EtFOSE also caused reduced maternal body weight and foetal weight in rodents and had effects quite similar to its metabolite PFOS (Lau et al. 2004).
Both PFBS and PFHxS have been assessed for developmental and reproductive effects. Maternal exposure to PFBS potassium salt did not produce any adverse effect on embryo/foetal development, and
no significant alterations were noted in a two-generation study in rats at doses as high as 1 g/kg. PFHxS is only examined in a screening system at lower doses without any effect observed (Lau et al. 2004).
Very high doses (30 mg/kg/d) of PFOA resulted in a reduction of maternal weight and a significant increase of mortality in the pups but at lower concentrations no significant effects were noted (Lau et al
2004).
PFDA and PFOA had no teratogenic effect in mice (Lau et al. 2004).
In a 2-generation reproductive toxicity study with PFOA, no structural changes were observed, and marginal changes in survival were seen at 30 mg/kg and not at 10 mg/kg (Butenhoff, 2004).
6.5.6 Endocrine disruption
Female rats were injected intraperitoneally with 0, 1 and 10 mg PFOS/kg bw for two weeks. The estrous cyclicity was affected, serum corticosterone level was increased, and serum leptin concentration and
norepinephrine concentration in the paraventricular nucleus of the hypothalamus were decreased. It was concluded that PFOS could affect the neuroendocrine system in rats and be an endocrine disruptor
(Austin et al. 2003).
Endocrine changes were studied in adult male rats fed at 25 mg ammonium perfluorooctanoate per kg by gavage (Biegel et al. 1995). Serum testosterone levels and testicular interstitial fluid testosterone
levels were decreased, and serum estradiol levels were increased. The reason was an induction of a hepatic aromatise by PFOA, which converts testosterone to estradiol.
In a two-week gavage study ammonium perfluorooctanoate decreased serum and testicular interstitial fluid testosterone levels, decreased relative accessory sex organ weights and increased serum estradiol
levels (Cook et al. 1992).
Several other studies have shown that PFOA can cause hormonal changes in males expressed as increased oestrogen level (Biegel 1997). In contrast, several other studies show no such effects. Functional
reproduction was not altered in rats exposed through 2-generations at APFO levels upto and including 30 mg/kg/day (Butenhoff, 2004). Further, in a 6-months oral study in monkeys, daily doses upto 20
mg/kg/day did not produce any changes in hormone levels, including oestrogen (Butenhoff, 2002)
6.6 Human toxicity
Chemical plant workers exposed to fluorochemicals in the air (up to 3.9 mg/m3) for years had about 100 times higher levels of organic fluorine in their blood (1-71 mg/L serum) than normal people but no ill
effects were attributable to exposure to these fluorochemicals. The half-life of PFOA in humans was estimated to be 18-24 months (Ubel et al. 1980).
Findings in animal studies suggest that PFOA affects hormonal states and metabolism of lipids in humans. However, no significant correlation has been observed between serum levels of PFOA (mean 5-7
mg/L) and any biological parameter in ammonium perfluorooctanoate plant workers (Olsen et al. 1998; 1999; 2000).
A retrospective cohort mortality study of a perfluorooctanesulfonyl fluoride (PFOSF) production workforce reported an excess of bladder cancer based on 3 deaths (SMR 12.77) at high-exposure jobs
(Alexander et al. 2003).
Workers at a PFOA production plant in the USA whose serum levels averaged 5 ppm had no excess of cancer mortality related to PFOA (Olsen et al. 2004). Olsen et al. (1998) concluded there was
reasonable assurance of no substantial hormonal changes associated with PFOA at serum levels measured among these male production employees.
6.7 Ecotoxicity
6.7.1 Terrestrial organisms
Recent avian toxicology studies have for bobwhites revealed a reproductive LOAEL of 10 ppm in the diet or 0.77 mg PFOS/kg bw/day for male and female birds. This corresponded to 8.7 μg PFOS/mL
and 4.9 μg PFOS/g in female serum and liver, respectively, and to 141 μg PFOS/mL and 86 μg PFOS/g in male serum and liver (Giesy & Jones 2004). In mallards the LOAEL was the same (10 ppm) for
males but 50 ppm for females.
6.7.2 Aquatic organisms
From Table 6.11 it is seen that PFOS is moderately acute toxic and slightly chronically toxic to aquatic organisms. PFOA is practically non-toxic. N-EtFOSA is slightly acute toxic to daphnids.
Hoff and co-workers (2003) studied the toxicological effects of PFOS on selected biochemical endpoints in the common carp (Cyprinus carpio). PFOS was administered by a single intraperitoneal
injection. The results suggested that PFOS induces a rise in serum transaminase levels indicative for disruption of hepatocyte membrane integrity that may be related to cell necrosis.
PFOS was three orders of magnitude more toxic to the aquatic midge Chironomus tentans than to most other aquatic organisms. The NOEC was 0.49 g/L (MacDonald et al. 2004). The actual
concentrations in the environment are still two orders of magnitude lower. PFOA had no impact on these organisms. The authors hypothesized that the high sensitivity to PFOS may be explained by
PFOS-haemoglobin interactions.
Table 6.11: Aquatic toxicity of PFAS (adapted from Heckster et al. 2003 and amended)
Substance |
Trophic level |
Species |
EC50 mg/L |
LC50 mg/L |
NOEC mg/L |
Reference |
PFOS |
Algae |
Selenastrum capricornutum |
120 (72hr) 73 (14d) |
|
|
3M 2000 |
Algae (mar.) |
Skeletonema costatum |
>3.2 (96hr) |
|
|
3M 2001 |
Invertebrates zooplankton |
Daphnia magna |
632 (48hr) |
|
7 (28d) |
3M 1984;2000 |
58 (46hr) |
|
|
Panarctic Oil 1986 |
|
21dLOELsurvival
50 |
25 (survival 21d) |
Sanderson et al. 2004 |
Daphnia pulicaria |
|
21dLOELsurvival
13 |
6 (survival 21d) |
Aquatic midge |
Chironomus tentans |
0.09 (10d) |
|
0.08 |
MacDonald et al. 2004 |
Invertebrates (mar.) |
Mysid shrimp |
3.6 (96hr) |
|
0.25 (35d) |
3M 2000 |
Fish |
Oncorhynchus mykiss |
7.8 (96hr) |
|
|
Panarctic Oil 1986 |
Fish |
Pimephales promelas |
|
|
0.30 (42d) |
3M 2000k |
Fish (mar.) |
Sheepshead minnow |
|
>15 (96hr) |
|
OECD 2002 |
PFOA |
Bacteria |
Photobacterium phosphoreum |
722 (30min) |
|
|
3M 1987a |
Algae |
Selenastrum capricornutum |
>1000 (96hr) |
|
|
3M 1996 |
Fish |
Pimephales promelas |
|
300 (96hr) |
>100 (30d) |
3M 1987b;1978b |
8:2 FTOH |
Algae |
Scenedesmus subspicatus |
|
|
0.20 (72hr) |
DuPont 2002 |
Invertebrates |
Daphnia magna |
|
|
0.16 (48hr) |
Fish |
Danio rerio |
|
|
0.18 (96hr) |
N-EtFOSA |
Invertebrates |
Daphnia magna |
48hr EL50 = 14.5 |
|
|
3M 1998a |
Fish |
Pimephales promelas |
|
96hr LL50 = 206 |
|
3M 1998b |
6.8 Summary of the environmental and health effects of PFOS and PFOA and other polyfluoroalkyl substances
6.8.1 Environmental chemistry
Polyfluoroalkyl substances (PFAS) are used because of their surfactant properties. They have both hydrophobic and oleophobic properties and are chemically and thermally inert, especially the fluorocarbon
chain is extremely resistant to heat and chemical attack, e.g. by acids and bases, and reducing and oxidizing agents.
The C-F binding is very strong. Thus the perfluoro part of the molecules is a stable identity, which in practice is non-degradable by all means. On the other hand the functional end group will be more readily
transformed in the environment and in organisms, and therefore the compounds will be degraded to the persistent sulfonates (e.g. PFOS) and carboxylates (PFOA) in the end. The sulfonates and
carboxylates are polar species, which will not accumulate in fatty compartments but in blood and liver, and these substances will often interact with polar sites in sediments.
6.8.2 Environmental release
PFOS and PFOA are intermediates and by-products from various industrial processes involving PFAS. Emissions to the environment (air and water) may happen directly from production and processing
plants or released during product use, e.g. fire-fighting foam, and as product impurities or degradation products. Environmental sources of fluorinated telomers are currently unknown but these substances
may be released, when manufacturing perfluorinated compounds and at the decomposition of polymeric materials that incorporate telomers.
6.8.3 Environmental exposure
It is expected that PFAS id present in the environment primarily in the form of the final stable degradation products PFOS and PFOA. In the recent years PFOS, PFOA and other perfluorinated compounds
have been discovered as global environmental contaminants. They have been found in indoor air, outdoor air, soil, ground water, surface waters and even at 1000 m depth in the Pacific Ocean, in wildlife and
human tissues.
6.8.3.1 Long-range transportation
The occurrence of PFOS and PFOA in the wildlife in remote areas such as the Arctic is puzzling. The binding to water and the low volatility make it less likely that PFOS and PFOA will be spread
long-range with the air by the "grass-hopping" and cold condensation mechanisms as persistent organic pollutants (POPs). However, the prevailing hypothesis is that long-range transport to the arctic occurs
via volatile precursors of both PFOS and PFOA, with subsequent degradation to these stable products. Volatile PFOS-precursors are a.o. MeFOSE and Et FOSE. It has been hypothesized that
fluorotelomer alcohols (FTOH) may be long-range transported and hereby reach remote arctic areas, where they can degrade to the more stable PFOA. Atmospheric lifetime of short chain FTOHs, as
determined with its reaction with OH-radicals, is approximately 20 days making the molecule able to travel about 7000 km. Therefore, they may be long-range transported and hereby reach remote areas,
where they can degrade to PFOA.
On the other hand products containing perfluorinated substances may also be used for example in fire-fighting foam at bases in remote areas. Recently a hypothesis has also been brought forward that ocean
current transport may in part explain the presence of PFOS and PFOA in the arctic.
6.8.4 Air levels
Data on levels of perfluorinated substances in ambient air exist only from the USA, Canada and Japan.
The substances identified in pg/m3 have mainly been MeFOSE and EtFOSE with lower levels of PFOS, MeFOSEA and FTOH. In urban areas levels were often 10 times higher than in rural areas, and in
cities with carpet production the levels were even higher. Higher concentrations were seen more in the summer than in the winter. Indoor levels are especially high and may be 10-100 times higher than
outdoors, and since the modern individual spends much time indoor, this exposure will really count.
6.8.4.1 Levels in ground and drinking water
In Japan tap water contained between 0.1-4 ng PFOS/L. Use of fire-fighting foam at military facilities in the USA has resulted in several episodes with serious pollution of groundwater with perfluorinated
compounds in concentrations up to 15 mg/L. The found substances were PFOS, PFHxS, PFBS, PFPS, PFOA, PFHxA, and PFHpA.
6.8.5 Levels in surface waters
In water from the Great Lakes both PFOS and some precursors (EtFOSAA and FOSA) were detected in unpolluted waters. Concentrations of PFOS were 20-70 ng/L. River water downstream an US
fluorochemicals plant contained up to 144 ng PFOS/L and 598 ng PFOA/L. Upstream PFOS levels were below 50 ng/L and PFOA could not be detected. Similar high levels were determined in effluents
from a Japanese wastewater plant.
In waters samples taken from remote Pacific Ocean areas low levels (<0.4 ng/L) of PFOA, PFOS and PFHxS were detected. Similar levels were found in samples from 1000 m depth. The background
levels were 5-10 times higher in water samples from the Atlantic Ocean. Coastal seawater from China, Korea and Japan contained 20 to 1000 times higher levels and PFNA could also be detected. In most
cases the concentration of PFOA was higher than PFOS.
Use of fire-fighting foams has also caused serious pollution of surface waters. An accidental spill at Toronto Airport polluted a creek. Upstream levels of perfluorinated substances were <0.03 μg/L while
downstream concentrations ranged 2 to 17,000 μg/L. The substances measured were: PFOS, PFHxS, and PFOA.
After a fire at an oil storage facility at a refinery in Japan the runoff waters contained 3,669 ng PFOS/L, 149 ng PFHxS/L, 200 ng PFOSA/L, 300 ng PFNA/L, 162 ng PFOA/L and smaller levels of PFBS,
PFUnA, PFDeA, PFHpA and PFHxA. The levels in the waters decreased by time (dilution?) but it is likely that the use of fire-fighting foams is an important source of soil and water pollution.
The highest levels of PFOA and PFOS found in samples from the North Sea were about 20 ng/L in samples from the mouth of River Elbe while the other detectable compounds (PFHxA, PFHxS, PFHpA,
PFNA, PFDeA and PFOSA) ranged from 1-3 ng/L. Relatively high levels of PFOA and PFOS (5-10 ng/L) were also found in samples from the Dutch and German Waddensea, representative for the
Danish Waddensea. In open waters levels were below 1 ng/L, and only PFOA could be quantified. In River Elbe waters the concentration of PFOS was higher than of PFOA. In seawaters the relation was
opposite. In a few samples of water from Norway, Denmark, Sweden, Finland, Iceland and Faroe Islands investigated for perfluorinated compounds, levels of PFOA were highest with median levels of 5.2
ng/L in seawater, 7.8 ng/L in lake water, 13.1 ng/L in rainwater, 20.5 ng/L in sewage effluent and 297 ng/L in landfill effluent.
Background levels of perfluorinated compounds in surface water from diffuse polluted areas seem to be about 20 ng/L in comparison to 0.2 ng/L in unpolluted areas.
6.8.6 Levels in sediment and sewage sludge
The binding of PFAS to sediment sewage sludge is in general strong and stable, which means a high potential for accumulation herein. In a few samples of sewage sludge from the Nordic countries PFOS
was the dominating substance in samples from Denmark, Norway and Sweden, and PFOA was highest in Iceland and Faroe Islands. Highest maximum levels of PFOS were found in Sweden (2644 pg/g
ww), Denmark (1041 pg/g ww) and Norway (1023 pg/g ww). Somewhat lower levels in Finland (925 pg/g ww), and in Island and Faroe Islands the levels were much lower at 220-241 pg/g ww. In
Finland PFHxA was the second most abundant, and PFNA was found in Norwegian, Danish and Finnish sludge.
In general sediment samples had lower levels than sludge. The highest level of PFAS was 1150 pg/g ww in a Finnish sample. Contamination was also high in Norway but not in Sweden and other countries.
6.8.7 Levels in biota
Many perfluorinated compounds are global contaminants, which bioaccumulate and are widely distributed in wildlife. PFOS has been detected in concentrations > 1 ng/g (ppb) in most samples analysed
from various species of aquatic animals (seals, otter, sea lion, dolphin, polar bear, mink), birds, fish and amphibians. Some samples also contained PFOSA, PFHxS and PFOA.
There was no difference between levels in male and female animals as for the lipophilic POPs. PFOS is also found in bird and fish eggs suggesting possible maternal transfer during yolk formation. Where the
PFOS level in seals is higher in Europe than the US, it is opposite with the levels in dolphins and seabirds. The extent of bioconcentration of PFAS appears to be highly structure dependent. Whereas PFOS
and PFOA and perfluorinated compunds with longer alkyl chain are considered to be bioaccumulative in wildlife and sometimes biomagnifying, perfluorinated acids with fully fluorinated chain lengths of C5
and below seem not to bioaccumulate.
6.8.8 Levels in fish
Some perfluorinated compounds accumulate in fish liver with a bioaccumulation factor of more than 6,000. At the previous mentioned Toronto Airport spill fishes in the creek were highly contaminated. Total
perfluoroalkane sulfonate concentrations in the fish livers ranged 2-73 μg/g and it was mainly PFOS. A few samples also contained small levels of PFBS, PFHxS, PFOA, PFPeA, PFHpA, PFDA, PFUnA
and PFTA. Normal levels in fish are much lower at 20-100 ng/g ww. In a recent Nordic project levels of PFOS followed by PFOSA dominated over PFOA in freshwater fishes. The highest levels of 551
ng PFOS/g ww and 141 ng PFOSA/g ww were measured in a Finnish pike sample. Marine fish samples from Faroe Island had the lowest contamination, and PFOSA was the most abundant. Otherwise
PFOS dominated. In all the Icelandic samples PFDS and PFHxA were present. In the Danish samples PFHxA, PFHpA and PFOA were also present. Levels of PFOS in Danish flounders and herrings
were around 20 ng/g ww.
PFOS was the major contaminant in fish from the Canadian Arctic, and levels of FOSA were also rather abundant but all samples contained also perfluorinated carboxylic acids (PFCA) ranging in length
from 9 to 15 carbons. In general, odd-length PFCAs exceeded the concentration of even-length PFCAs, and the concentration decreased with increasing chain length. Levels were generally lower than
levels for similar animals sampled in the USA.
6.8.9 Levels in birds
Significant levels of PFOS in birds (egg yolk, plasma and liver) have been reported from various places. The levels were clearly higher in populated and industrialised areas than in remote locations. Levels in
birds from Antarctica are around or below the quantification limit. The highest levels were found in blood from bald eagles collected in Midwestern USA (2570 ng PFOS/g ww).
PFOS was the major contaminant in birds from the Canadian Arctic but common loon also contained significant levels of FOSA. In contradiction to mammals and fish perfluorinated carboxylic acids were
not detected in birds. Levels were generally lower than levels for similar birds in the USA. In fulmar eggs from Faroe Islands PFAS levels were about 40 ng/g ww and 95% of that was PFOS followed by
PFNA and PFOSA.
6.8.10 Levels in mammals
Significant levels of PFOS have been reported in aquatic mammals from various places. The highest levels were found in mink (3680 ng PFOS/g ww) and river otters (990 ng/g ww) from the western USA.
High levels were also found in Alaskan polar bear (680 ng/g ww) and seals (230 ng/g ww) from the Baltic Sea and in dolphins (430 ng/g ww) from the Mediterranean Sea. The levels were clearly higher in
populated and industrialised areas than in remote locations. Both PFOS and PFOA have been identified in polar bears, ringed seals, arctic fox, and mink from the Canadian Arctic. PFOS was the major
contaminant but all samples contained also perfluorinated carboxylic acids (PFCA) ranging in length from 9 to 15 carbons. In all samples except for minks PFOS dominated. In minks Perfluorononanoic acid
(PFNA) was highest. Mammals feeding at higher trophic levels had greater contamination. Highest mean levels were 3100 ng PFOS/g, 180 ng PFNA/g and 8.6 ng PFOA/g in polar bears. In general,
odd-length PFCAs exceeded the concentration of even-length PFCAs and concentration decreased with increasing chain length.
In the Nordic area grey seals from Sweden and harbour seals from Denmark were mostly contaminated with PFOS as the dominating substances (up to 1 g/g ww). Minke whales from Iceland were lower
contaminated than pilot whales from Faroe Island. In pilot whales PFOSA levels were sometimes exceeding PFOS levels.
6.8.11 Human exposure
The pathways leading to human exposure to PFOS and PFOA are not well known. Protections of clothes and carpets are among the most important uses of these chemicals and precursors. Thus exposure
to indoor air and dust may be important. High concentrations of perfluorinated compounds have been measured in indoor air (see earlier). Dust collected from vacuum cleaners in Japanese homes also
contained very high levels of PFOS and PFOA. PFOA levels were in general higher than PFOS levels. The daily intake of PFOS from air exposure in Japan was calculated to 10-100 pg/day, which would
result in a 1.2-12 ng/L excess of plasma PFOS levels.
Food intake may be another exposure route. Some perfluorinated compounds e.g. EtFOSA may be present in some oil- and water repellent coatings for paper and paperboard used in food packaging. A
Canadian study of fast food composites collected before 2000 revealed that more than 55% of the composites contained this chemical (detection limit: 10 pg/g). The highest level measured (23,500 pg/g)
was in a pizza. PFOS was also detected in three samples. Samples after 2000 were free of these contaminants.
A Japanese study has shown that consumption of drinking water obtained from a polluted river may lead to a significantly increased daily intake of up to 1 mg PFOS/day and may contribute 8-16 μg PFOS/L
to blood serum levels and result in a 25-50% rise in normal levels.
6.8.12 Human levels
For decades perfluorochemicals have been detected in human blood from occupational exposed individuals. Serum PFOS concentrations among production workers in PFOS-related processes averaged
0.5-2 mg/L (ppm) and ranged <0.1-12 mg/L (ppm). These occupational levels are 100-1000 times higher than present serum and liver levels of 15-50 μg PFOS/L (ppb) in the general population. Levels of
PFOA, PFHxS, PFOSAA and PFOSA are a magnitude lower (<5 μg/L).
A study of historic and recent serum samples from Japan revealed that sex and residential area are the most important factors influencing the levels while age and smoking status had no influence. It seems
that levels of PFOS reached a maximum in the mid 1990's, while PFOA concentrations are still increasing. PFOS concentrations in foetal samples were only one-third to a half of maternal levels.
Levels of PFOS and PFOA in blood samples from the Canadian Arctic were similar to other parts of Canada and seemed not to be related to food habits as it is for PCB and dioxins. All samples also
contained PFNA and a few samples also PFHpA and PFHxA.
In Sweden blood samples from the general population were analysed for 12 perfluorinated compounds with chain length from 4 to 14. PFOS, PFOA, PFOSA, PFHxS and PFNA were found in almost all
samples, while traces of PFDeA and PFDoA were found in most samples. Besides this, PFDS, PFHxA, PFDoA and PFTeA were detected in a few samples. The mean sum of all perfluorinated compounds
was 25μg/L, and levels of PFOS dominated with a mean of 18 μg/L.
6.8.13 Animal toxicity
PFOA and PFOS may bind to proteins and accumulate in various body tissues of exposed organisms, including in blood and liver, and for PFOS also in testes and brain. Although the perfluoroalkane
sulfonic acids and carboxylic acids are closely related structurally, these chemicals elicit different biological responses in vitro and in vivo.
The acute lethal toxicity is moderate corresponding to a classification as harmful. PFOS is more toxic than PFOA, and the toxicity increases with the length of the alkyl chain.
The liver is the primary target organ for perfluorinated compounds. PFCAs cause peroxisome proliferation in the rodent liver as well as induction of various enzymes involved in lipid metabolism. PFDA with
a longer alkyl chain seems even more active than PFOA. Toxic effects have been reported, such as induction of fatty liver and uncoupling of the mitochondrial respiratory chain.
PFOA also affects the serum levels of various hormones, i.e. reducing testosterone, and increasing estradiol in rats. Thus it acts as an endocrine disruptor.
In in vitro and in vivo test systems PFOS, PFOSA, and PFHxS – but not PFBS - inhibit gap junction intercellular communication in a dose-dependent fashion, and this inhibition occurred rapidly and was
reversible. Perfluorinated carboxylic acids with carbon chain length of 7-10 can also rapidly and reversibly inhibit gap junction intercellular communication in a dose-dependent manner in vitro, and with
PFDA inhibiting more than PFOA.
Gap junction intercellular communication (GJIC) is the major pathway of intracellular signal transduction, and it is thus important for normal cell growth and function. Defects in this communication may lead
to teratogenesis, neuropathy, infertility, diabetes, autoimmune disorders, cancer, and other diseases.
Although the fluorinated chemicals do not seem to be mutagenic, PFOA induces testis cancer and PFOS and EtFOSE induce liver cancer in experimental animals. USEPA classifies PFOA as a carcinogen in
animals.
PFOS causes developmental effects including reduction of foetal weight, cleft palate, oedema, delayed ossification of bones, and cardiac abnormalities. However, the structural abnormalities were found in
the highest PFOS dose groups, where significant reductions of weight gain and food consumption were also observed in the pregnant dams. Thus the relevance may be questioned. PFOA causes reduction
of foetal weight. Other PFAS (PFBS and PFHxS) have neither a significant effect on reproduction or development even at high doses.
6.8.14 Human toxicity
In general the knowledge about the toxicology of the perfluorinated compounds is rather sparse, and it will take some time and efforts, before we will have sufficient information for evaluation of the full
impact of the present levels in humans. The experience from the work environment has not indicated any important adverse health effects among exposed workers, besides a retrospective cohort mortality
study of a perfluorooctanesulfonyl fluoride (PFOSF) production workforce, which reported an excess of bladder cancer at high-exposure jobs.
6.8.15 Ecotoxicity
Only a few studies and mainly concerning acute toxicity to aquatic organism exist in open literature. PFOS is moderately acute toxic and slightly chronically toxic to aquatic organisms. PFOA is practically
non-toxic. N-EtFOSA is slightly acute toxic to daphnids. There seems to be large species difference in the biological response, because PFOS was three orders of magnitude more toxic to the aquatic midge
Chironomus tentans than to most other aquatic organisms. The scarce database indicates a need for further studies.
Footnotes
[10] Perfluorononanoic acid
[11] 2-(N-Ethylperfluorooctane sulfonamido) acetic acid
[12] Perfluorooctane sulfonamide
[13] Surveys indicate that the use of PFAS compounds for impregnation of paper and cardboard is more or less historical.
| Front page | | Contents | | Previous | | Next | | Top |
Version 1.0 June 2005, © Danish Environmental Protection Agency
|