| Front page | | Contents | | Previous | | Next |
Evaluation of in vitro assays for determination of estrogenic activity in the environment
5 Evaluation of the various methods
5.1 Sample preparation
5.1.1 Collection and storage
5.1.2 Filtration
5.1.3 Extraction
5.2 Laboratory facility requirements
5.3 Detection limits and EC50 values
5.3.1 Detection limits and EC50 values for the various in vitro assays
5.3.2 Detection limit and limit of quantification for the whole method
5.4 Time and cost considerations
5.5 Robustness
5.6 Utility in various matrices
5.7 Advantages and limitations compared to chemical analyses
5.8 Limitations compared to in vivo assays
5.1 Sample preparation
5.1.1 Collection and storage
The assessment of estrogenic activity in environmental water samples begins with sample collection and some sort of storage until analysis. However, detailed descriptions of the modes of water sample collection and storage for in vitro investigations are lacking in most papers. Obtaining representative samples is an important requirement and is made more problematic when working with raw sewage and other samples that are not homogenous. Sampling periods of 7 (Murk et al., 2002) or 24 h (Desbrow et al., 1998; Körner et al., 1999, 2000, 2001) have been used to collect composite, representative water samples in some studies, whereas discrete water samples have been studied in other cases. In most studies the samples have been collected in glass bottles. The sample volume varies from 1 to 25 L. The water samples were usually stored, from the time of collection until extraction, which was usually carried out within 48 h of collection, at 4°C without preservation. One study reported storage of unpreserved samples for up to 10 days (Murk et al., 2002). Other authors added methanol to the water samples to minimize bacterial activity (Desbrow et al., 1998; Kirk et al., 2002).
Kelly (2000) reported that storage of water samples for more than a week resulted in degradation of E2 to E1. Baronti et al. (2000) performed a stability study to evaluate estrogen degradation during storage of river water samples. According to their study the best sample storage scheme consists in passing the field sample through the extraction cartridge, washing the cartridge with methanol, and storing it at -18°C. Under these conditions, which facilitate the storage of many samples in extensive monitoring, no significant loss of estrogens was detected following storage for 60 days.
5.1.2 Filtration
Sample preparation usually begins with filtration. This step is especially crucial when subsequent extraction of the sample is based on the use of solid-phase extraction, since suspended solids could easily clog the adsorbent bed. The majority of the studies reviewed utilized glass fibre filters (pore size between 0.45 and 1.2 mm). To elucidate whether or not the (xeno)estrogens are retained by the filter material, Desbrow et al. (1998) extracted sequentially, with a series of solvents of increasing polarity, the material removed from wastewater treatment plant effluent by filtration through glass fibre. The estrogenic activity of the solvent extracts, determined by means of the YES assay, indicated that the estrogenic activity was not retained by the filters but was present in the dissolved phase of the effluent samples. It is questionable, however, whether merely rinsing particulates with organic solvents will quantitatively desorb the analytes from these particulates (Xiao et al., 2001). Huang and Sedlak (2001) performed recovery experiments to assess the potential for adsorption of dissolved E2 onto filters. E2 was added to wastewater effluent. After filtration, extraction and cleanup, a recovery of 99% was obtained, indication that sorption onto filters was negligible. Mol et al. (2000) reported that alkylphenols are prone to losses during filtration of water samples, but that these compounds, however, can readily be extracted from the filter again using a combined filtration/solid-phase set up. In one study (Kirk et al., 2002), as a supplement to filtration, centrifugation was employed with the same purpose of eliminating suspended materials.
5.1.3 Extraction
In general, crude water samples, with no pH adjustment or addition of modifiers, were extracted and analysed. Exceptions to this were addition of 0.5% (v/v) methanol to the sample, to facilitate solid-phase extraction (Desbrow et al., 1998; Körner et al., 1999, 2000; Kirk et al., 2002), and adjustment of the pH of the sample (to 2-3), as made by Körner et al. (1999, 2000) who found that extraction of untreated wastewater at neutral pH was incomplete. The pH adjustment step may deconjugate the steroid metabolites present in the water samples (Desbrow et al., 1998). Extraction of (xeno)estrogens from water samples is usually carried out by solid-phase extraction. Both disks and, most commonly, cartridges have been utilized for the solid-phase extraction of (xeno)estrogens from water samples, although the latter have been described as disadvantageous compared with the former. Disks are not clogged as easily as cartridges by the suspended material present in the samples, have a comparatively larger water/extractant surface area (which results in higher extraction rates), and eliminate the risk of sample contamination as a consequence of leaching of plasticizers from the cartridge support material during elution (Schülein et al., 1995; Kelly, 2000). Other considerations suggest, however, that these disadvantages of cartridges are not a great problem. Thus, filtration usually prevents clogging of cartridges. Furthermore, appropriate cleaning of the cartridge, before its conditioning and the sample loading, with the solvents which will subsequently be used for elution should eliminate, or at least minimize, leaching from the plastic holders of the cartridges (López de Alda and Barceló, 2001). Cartridges, compared with disks, have the advantage of being amenable to system automation, because specific devices are available for unattended washing, conditioning, sample loading, eventual drying, and final elution of a large number of samples (López de Alda and Barceló, 2001). Xiao et al. (2001) found extraction discs suitable for river water samples, giving good recoveries of the major estrogens E1, E2, E3 and EE2. However, the recoveries of estrogens extracted from samples of treated sewage effluent were found to be low, possibly due to overloading by the large amount of organic material present. For these samples, extraction using large volume C18 cartridges provided an alternative procedure. Octadecyl (C18)-bonded silica has been the solid-phase extraction adsorbent most commonly utilized (Desbrow et al., 1998; Körner et al., 1999, 2001; Balaguer et al., 2000; Kirk et al., 2002; Fenet et al., 2003; Kawagoshi et al., 2003; Tashiro et al., 2003). However, styrenedivinylbenzene (SDB), available as ENV+ cartridges (Körner et al., 2000) or SBD-XC discs (Witters et al., 2001; Murk et al., 2002; Huggett et al., 2003) has also been employed. A study comparing the behaviour of C18 and SDB however showed that the polymeric adsorbent (SDB) was unsuitable for quantitative extraction of E3 from water (López de Alda and Barceló, 2000). Körner et al. (1999) found that after solid-phase extraction with C18 or ENV+ cartridges, analysis of the extracts in the E-screen assay gave practically the same quantitative results. Using the C18 phase the extraction procedure generally required more time and higher vacuum, especially when the sample contained larger amounts of suspended matter (raw sewage). The ENV+ phase was therefore preferred for extraction of sewage samples. The XAD-2 resin columns utilized by Shen et al. (2001) has been shown to be inadequate for the preconcentration of estrogens from water. Kuch and Ballschmiter (2000) compared the efficiencies of XAD-2 and a mixture of LiChrolut EN and Bondesil C18 for extraction of the recovery standard cholesteryl acetate from sewage treatment plant effluent samples. The recovery obtained with these adsorbents varied between 8 and 39% (mean: 23%) and between 61 and 94% (mean: 78%), respectively. Where stated, the sample-loading flow rate in the reviewed studies varied between 7 and 100 ml/min (Desbrow et al., 1998; Körner et al., 1999, 2000; Shen et al., 2001; Witters et al., 2001; Tashiro et al., 2003). Elution of the retained compounds from C18 is typically carried out with methanol (Desbrow et al., 1998; Kirk et al., 2002; Vethaak et al., 2002; Tashiro et al., 2003), with total elution volumes varying between 5 and 45 ml. Elution from SDB adsorbents has been achieved with various solvents such as acetone, methanol, methylene chloride and hexane (Witters et al., 2001; Körner et al., 2000; Murk et al., 2002; Huggett et al., 2003). Subsequent drying of the cartridge with either nitrogen or air is a common procedure. Ultimately extracts are taken up in methanol, dimethylsulfoxide (DMSO), hexane or ethanol for exposure in the in vitro assays (Desbrow et al., 1998; Körner et al., 1999, 2000, 2001; Balaguer et al., 2000; Shen et al., 2001; Witters et al., 2001; Kirk et al., 2002; Koh et al., 2002; Murk et al., 2002; Fenet et al., 2003; Huggett et al., 2003; Kawagoshi et al., 2003). Beresford et al. (2000) found a slightly increased sensitivity of the YES assay with DMSO compared to ethanol.
To the knowledge of the author, the only published study of estrogenic activity in liquid manure using in vitro assays is from the Dutch national investigation into the occurrence and effects of estrogenic compounds in the aquatic environment (Vethaak et al., 2002). In this investigation, the sample preparation method for cattle manure samples for the ER-CALUX assay was the same as described for wastewater with a large load of suspended solids (personal communication, Gerard Rijs, RIZA, the Netherlands). This extraction procedure included soxhlet extraction of the solid material in the samples.
To the knowledge of the author, the only published study of estrogenic activity in agricultural drain water using in vitro assays is from the Central Valley of California (Johnson et al., 1998). In this study, water samples were extracted with chloroform. The solvent was extracted under nitrogen and dried samples resuspended with dioxane and diluted with DMSO. The estrogenic activity in the water samples was then assessed with an ER binding assay.
Estrogenic activity and estrogenic chemicals in landfill leachate were investigated by use of an in vitro yeast assay and chemical analysis (Kawagoshi et al., 2003). Leachate samples extracted by liquid-liquid extraction with dichloromethane showed a higher in vitro estrogenic activity than samples extracted by solid-phase extraction. In the Dutch national survey, results from solid-phase and liquid-liquid extraction were also compared (Vethaak et al., 2002). In addition to the wastewater samples prepared with standard solid-phase extraction (including filtration for some of the samples), some of the samples were extracted by liquid-liquid extraction (3 ml wastewater with 3x4 ml diethyl ether). The extracts were then tested with the ER-CALUX assay. Comparison of the results indicated a considerable difference in ER-CALUX activity measured. The EEQ values of the samples extracted with the standard solid-phase extraction procedure were on average only 17% of the EEQ values obtained via the liquid-liquid extraction procedure. This indicates that a relatively large portion of the estrogenic activity in water may be lost via filtration and solid-phase extraction. However, for surface water samples, Mol et al. (2000) found both liquid-liquid extraction (at pH 5-6) and a combined filtration/solid-phase extraction (at pH 4) to be suited for extraction of (xeno)estrogens. With the latter method, recoveries between 58% (for bisphenol A) and 106% (for EE2) were found. For the liquid-liquid extraction method, recoveries of 109-117% were found.
In the comparative study with an ER binding assay, the YES assay and the ER-CALUX assay for detection of estrogenic potency in wastewater and surface water, the same sample preparation was used for all three in vitro tests (Murk et al., 2002). The amounts of material needed to determine the estrogenic potency of the three in vitro assays, however, differed greatly: ER binding assay > YES > ER-CALUX. In the ER-CALUX assay 6-30 ml surface water; 0.2-2.5 ml wastewater influent; and 0.9-9 ml wastewater treatment plant effluent was needed. In the YES assay the corresponding amounts were 60-250 ml, 4-100 ml, and 10-100 ml, respectively. In the ER binding assay the needed amounts were approximately 950 ml for surface water and 400 ml for wastewater influents or effluents.
Körner et al. (1999, 2000, 2001) used the E-screen for analysis of estrogenic activity in sewage plant effluents in Germany. Following sample extraction, stock solutions of the extracts were prepared with steroid-free experimental medium. This medium consisted of phenol red-free Dulbecco‘s modification of Eagle‘s medium supplemented with charcoal dextran-treated fetal calf serum (CD-FCS), HEPES, glutamine, amino acids and a penicillin/streptomycin/amphotericin solution. CD-FCS was prepared by treatment of fetal calf serum (FSC) with charcoal-dextran (CD) followed by stirring for 24 h, centrifugation and filtration.
5.2 Laboratory facility requirements
ER binding assays include the use of radiolabels, which involves potential health hazards and the requirement for special licenses, equipment and precautions for handling and disposing radioactive material. The major equipment required is a liquid scintillation counter.
Unlike the ER-binding assays, reporter gene assays and the E-screen involve cell cultures. Compared with yeast cells, mammalian cells are more expensive and difficult to cultivate. Mammalian cells require more constant care and fresh medium; and are vulnerable to the risks of contamination. Cultured mammalian cells are particularly sensitive to variations in temperature, pH, dissolved oxygen and certain metabolites, which makes it necessary to control culture conditions carefully. Yeast cells are more resilient and highly resistant to adverse environmental conditions, making them relatively easy to maintain and to grow.
The specific needs as related to the various in vitro procedures utilizing reporter genes, whether transiently or stably transfected, are essentially the same. A standard cellular or molecular biology laboratory with cell culture capabilities is required. The major equipment required for mammalian-based studies is a cell incubator with temperature, CO2, and humidity controls; sterile laminar cabinets; and a luminometer for assays requiring luciferase detection. The YES assay with yeast cells does no require that the researchers work to the same standards of sterility as for the mammalian cell assays (personal communication, Dr. Juliette Legler, IVM, Vrije Universiteit Amsterdam, the Netherlands). A sterile laminar cabinet may not be required for the YES assay as the yeast cells are less susceptible to effects by bacterial and fungal infections. A specialized incubator is also not necessary for the yeast cells.
The E-screen has the same facility and equipment requirements as mammalian-based reporter gene assays, except that cell-counting equipment (Coulter counter) would be an additional requirement if the method of Soto et al. is followed.
5.3 Detection limits and EC50 values
5.3.1 Detection limits and EC50 values for the various in vitro assays
This section deals with the detection limits and EC50 values for E2, not including the concentration factors employed when assessing environmental samples. The EC50 is the concentration at which half-maximal activity is induced. The definition of the detection limit is often not stated in the published studies. However, Witters et al. (2001) calculated the detection limit of the YES assay as absorbance elicited by the solvent control plus three times the standard deviation. Körner et al. (1999) defined the detection limit of the E-screen as the concentration of a single compound or an environmental sample inducing a cell proliferation significantly higher than that of the hormone-free negative control. An overview of the detection limits and EC50 values for the various in vitro assays is found in Table 2.
Detection of estrogenic potency in wastewater and surface water with three in vitro assays was studied by Murk et al. (2002). The three assays applied were an ER binding assay and two reporter gene assays: ER-CALUX and YES. All assays were able to detect estrogenicity in wastewater and surface water. However, the detection limits differed greatly between the three assays: ER binding assay >> YES > ER-CALUX. The detection limit for the ER-CALUX assay was 0.1 ng/l E2; for the YES assay it was 2.7 ng/l E2; and for the ER binding assay it was 272 ng/l E2. The EC50 values for the ER-CALUX, the YES and the ER binding assay were 1.6 ng/l E2, 27 ng/l E2 and 3162 ng/l E2, respectively. For E2, nonylphenol and o,p’-DDT, a difference of approximately 6- to 20-fold was found between the EC50 values in the ER-CALUX and the YES assay (Legler et al., 2002a).
In order to assess the (anti)estrogenic potential of pure compounds and complex environmental samples Gutendorf and Westendorf (2001) compared an array of in vitro test systems, (i) two luciferase reporter gene assays: the MVLN cell assay and the HGELN cell chimeric receptor/reporter gene assay; (ii) competitive binding assay s with recombinant human ER a and b; and (iii) the E-screen. The sensitivity of the assays for E2 decreased in the order: MVLN cell assay = E-screen > HGELN cells > binding to ER-a > binding to ER-b. The EC50 for the MVLN cells and the E-screen was 1 ng/l E2. For the HGELN cells, binding to the ER-a and binding to the ER-b the EC50 values were 11 ng/l, 953 ng/l and 17,705 ng/l, respectively.
A study using the E2 Bioassay for detection of estrogenic activity in pulp and paper mill black liquor and effluent showed a detection limit of approximately 5 ng/l and an EC50 of 5.4 ng/l for E2 (Zacharewski et al., 1995). Another study using the E2 Bioassay for assessment the estrogenic activities of chemicals and complex mixtures likewise showed a detection limit of approximately 5 ng/l E2. The EC50 was 11 ng/l E2 (Balaguer et al., 1996).
A comparison of the estrogenic potencies of E2, EE2, diethylstilbestrol, nonylphenol and methoxychlor in vivo and in vivo showed that the EC50 values for all five chemicals were approximately one order of magnitude higher in the YES assay than in the E-screen assay. In the YES assay the EC50 for E2 was 57 ng/l whereas in the E-screen the EC50 for E2 was 8.7 ng/l (Folmar et al., 2002).
A recent study compared the potencies of estrogenic compounds in the YES assay (Segner et al., 2003). The EC50 values for EE2, E2, 4-tert-octylphenol and bisphenol A were 220 ng/l, 212 ng/l, 436 mg/l and 2615 mg/l, respectively.
In a comparative study of in vitro and in vivo assays for estrogenicity in effluent from North American municipal wastewater facilities Hugget et al. (2003) reported the detection limit of the YES assay as £ 1 ng/l E2. A study using the YES assay for detection of estrogenic activity in Flemish surface waters showed a detection limit of ~2.75 ng/l E2 and EC50 values of ~100 ng/l E2 (Witters et al., 2001). Similar detection limits and EC50 values were reported in other studies (Beresford et al., 2000; Kirk et al., 2002). Comparison of short-term estrogenicity tests for identification of hormone-disrupting chemicals revealed EC50 values of 54 ng/l or 218 ng/l E2 for the YES assay (Andersen et al., 1999). Arnold et al. (1996), Tyler et al. (2000) and Miller et al. (2001) likewise reported EC50 values around 54 ng/l for the YES assay.
In a number of papers dealing with the E-screen and estrogenic active compounds in sewage treatment plants in Germany, EC50 values between 0.3-2.7 ng/l E2 were recorded (Körner et al., 1998, 1999, 2000, 2001). The detection limit of the E-screen method was 0.27 ng/l E2.
A comparison of short-term estrogenicity tests for identification of hormone-disrupting chemicals revealed EC50 values of 0.03-1.9 ng/l E2 for the E-screen (Andersen et al., 1999). A study of the estrogenic potency in each step of a controlled landfill leachate treatment plant in Japan showed an EC50 of 1.7 ng/l E2 for the E-screen (Behnisch et al., 2001).
The above studies show that the yeast-based YES assay is less sensitive than the mammalian-based MVLN cell assay, ER-CALUX and E-screen. There may be a number of explanations for this difference in sensitivity between the assays. One explanation could be differences in uptake of compounds through the yeast cell wall relative to mammalian cell membranes (Zysk et al., 1995) and the ability of yeast cells to actively transport specific compounds out of the cell (Kralli et al., 1995). Other mechanisms that may be involved in the difference in sensitivity between the yeast- and mammalian-based assays include differences in cellular transcription factors (Halamachi et al., 1994), multiple drug resistance (Dexter et al., 1994), and endogenous yeast estrogen binding proteins (Feldman et al., 1982). For the reporter gene assays, the type of reporter protein used may also have a major impact on the sensitivity of the assay, because it determines the type of instruments or analytical methods that can be used to detect it (Villeneuve et al., 1998). Because of the availability of sensitive detectors for light and the high quantum efficiency of the luciferase reaction, the light-producing endpoint for luciferase-based reporter gene assays can be very sensitively detected using a luminometer. Colorimetric endpoints, such as the b-galactosidase endpoint used in the YES assay tend to be less sensitive.
The YES assay can be made more sensitive by using longer incubation periods (UK Environment Agency, 1997; Beresford et al., 2000).
5.3.2 Detection limit and limit of quantification for the whole method
This section deals with the detection limit and limit of quantification for the whole method, i.e. including the concentration factors employed when assessing environmental samples. In calculating a detection limit for the whole method for analysing a liquid sample the maximally achievable concentration factor has to be taken into account. However, few studies report this concentration factor, the detection limit or the limit of quantification for the whole method. Körner et al. (1999) used a maximal concentration factor of 20, resulting in a detection limit of 0.014 ng/l EEQ for the whole method. As the highest concentration of the effluent samples showed some cytotoxic effects in the E-screen but not anymore in the 5-10-fold dilution, the limit of quantification was 0.07-0.14 ng EEQ/l. Witters et al. (2001) used a maximal concentration factor of 100 for the YES assay, which would result in a detection limit for the whole method of 0.028 ng EEQ/l. Although they observed cytotoxic effects at the highest test concentrations they did not report at which dilution cytotoxic effects were no longer observed. Thus, the limit of quantification is not known but is >0.028 ng EEQ/l. Arijs et al. (2002) reported a detection limit of 0.027 ng EEQ/l for the YES assay. The detection limit was calculated as EC50[E2] divided by the max. extract concentration that was not toxic to the yeast (personal communication, Katrien Arijs, Ghent University, Belgium ).
5.4 Time and cost considerations
Other considerations are the time consumption and cost of the various in vitro methods.
Table 2 provides information on the time needed to perform a study with the various in vitro assays. Measuring relative binding affinities is a fast (1-2 d) way to screen compounds, whereas the E-screen is more time-consuming (~6 d) than the other in vitro assays.
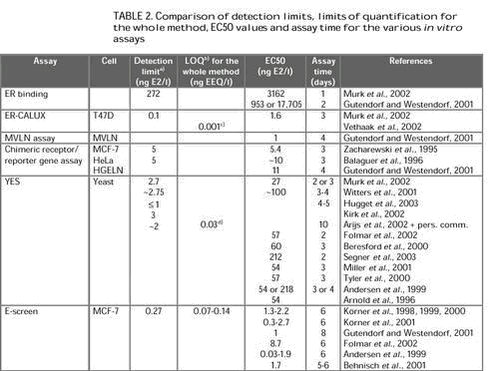 Click on the picture to see the html-version of: Table 2
a) Detection limit not including concentration factors (see section 5.3.1). b) LOQ for the whole method: Limit of quantification including concentration factors (see section 5.3.2). c) Detection/quantification limit for the whole method deduced from Vethaak et al. (2002) Table 4.6. d) Reported as a detection limit taking toxic effects into account (see section 5.3.2).
Specific cost information for the assessment of estrogenic activity of environmental samples with in vitro assays is, to the knowledge of the author, not available in the literature. However, since the classical ER binding assays are not miniturisable or easily amenable to automation (which would otherwise permit decreased reagent cost and increased throughput), they must be expected to be relatively expensive assays. The reporter gene assays and the E-screen are applicable to multiwell technology, thus reducing time consumption and cost. However, cultivation of mammalian cells is more demanding than cultivation of yeast cells in terms of growth medium reagents and time consumption. The mammalian-based reporter gene assays and the E-screen must therefore also be expected to be relatively expensive, whereas the YES assay must be expected to be relatively inexpensive.
5.5 Robustness
The success of assessments of estrogenic activity is dependent on the robustness of the in vitro assay in providing reproducible data with relatively small variations.
Despite its widespread use, considerable inter-laboratory variability has been observed in test results from the E-screen. Numerous cell lines and widely varying test procedures have been employed, which may account for much of the variability in results (Zacharewski, 1997). Since the establishment of the MCF-7 cell line 30 years ago, the cell line has undergone several changes, and studies have demonstrated that MCF-7 cell line variants exhibit fundamental divergences in characteristics such as (xeno)estrogen-dependent proliferation rate, population doubling time (Villalobos et al., 1995), and susceptibility to apoptosis (Burow et al., 1998). In addition there are differences between different MCF-7 stocks in regard to their ability to detect antagonists (Diel et al., 1999). Villalobos et al. (1995) have shown the influence of different MCF-7 cell sublines on test results. Likewise, variations in culture conditions, such as the number of cells plated and the duration of incubation in estrogen-free medium prior to treatment with test compounds, have been shown to have prominent effects on the responses to E2 (Jones et al., 1997; Rasmussen and Nielsen, 2002). In addition, only some sera support estrogen-specific growth of MCF-7 cells (Wiese et al., 1992). Furthermore, drift in responsiveness of MCF-7 cells during culture may confound their consistent use in proliferation assays (Desaulniers et al., 1998; Jones et al., 1998; Odum et al., 1998). Payne et al. (2000) have demonstrated the importance of choice of cell line and culture conditions in determining test results. In a large inter-laboratory study, Andersen et al. (1999) reported that by using a standardised cell line (MCF-7/BUS) with similar protocols, good agreement could be achieved with most test compounds. However, they found a lack of consistence with chemicals such as benzyl butyl phthalate and p,p’-DDE. It must also be noted that a range of non-estrogenic substances, including progesterone, androstenediol, insulin-like growth factors, epidermal growth factor, caffeine and ethanol have been found to influence the proliferation of human breast cancer cells (Osborne et al., 1990; van der Burg et al., 1992; Dickson and Lippman, 1995; Jones et al., 1998; Diel et al., 1999; Andò et al., 2002). Furthermore, the E-screen assay might be extremely sensitive to small changes in the physical or chemical properties of culture conditions induced by test substances, leading to non-specific increases or decreases in proliferation independent of ER-binding (Desaulniers et al., 1998). Thus, a positive response cannot be attributed strictly to estrogen receptor agonists. The use of anti-estrogens (e.g. ICI 182,780) could help to distinguish estrogenic from non-estrogenic activity. However, this increases the complexity of the assay.
In the E2 Bioassay responsiveness has been observed to be sensitive to the number of passages and the type of cells utilized (Zacharewski, 1997). MCF-7 cells recently taken from frozen stocks (i.e., within the first three passages) and those beyond 10 passages after removal from frozen stocks show lower overall E2-induced luciferase activity. Consequently the assay is most consistent when the cells utilized are between 3 and 10 passages. Differences in responsiveness have also been observed when the constructs are transfected into different cell lines. For example, maximum induction in stably transfected HeLa cells ranges from 8- to 12-fold while maximum induction in transiently transfected MCF-7 cells averages between 40- and 50-fold. Differences in the level of responsiveness within experiments and between cell types may be due to variations in ER levels and in the presence of appropriate transcription factors.
When MVLN cells are exposed to hydroxytamoxifen or tamoxifen their luciferase reporter gene can irreversibly no longer respond to estrogens (Badia et al., 1994). This raises the issue of instability of the MVLN cell assay due to exposure to inhibiting chemicals during cell culture or assay performance.
Beresford et al. (2000) investigated the effect of alterations in assay methodology for the YES assay on the response to certain xenoestrogens. None of the four parameters examined (incubation time, whether the solvent was allowed to evaporate or not, the type of solvent, and initial yeast cell number) had any appreciable effect on the relative potencies of nonylphenol or bisphenol A. However altering these criteria did affect both the dose-response curves produced by butyl benzyl phthalate and o,p’-DDT. In addition other factors, such as incubation temperature and growth stage of the yeast, may also alter the response in the YES assay. The YES assay does not consistently differentiate between estrogen agonists and antagonists. Tamoxifen and hydroxytamoxifen have shown both agonistic and antagonistic activity in the YES assay (Routledge and Sumpter, 1997; Sohoni and Sumpter, 1998; Legler et al., 2002a). The anti-estrogen ICI 182,780 produces a purely agonistic response in the YES assay (Beresford et al., 2000, Legler et al., 2002a).
Legler et al. have used both the ER-CALUX and the YES assay for determination of estrogenic activity in environmental matrices. They do not culture the ER-CALUX T47D cells higher than 30 passages (personal communication, Dr. Juliette Legler, IVM, Vrije Universiteit Amsterdam, the Netherlands), as some studies indicate that the responsiveness of the cells decreases, as they get older. Despite the “fussiness” of the cells, they find them to give more reproducible results (ER-CALUX %CV: 5-10) than the YES assay (%CV: 10-25) and would say that the ER-CALUX is more robust.
5.6 Utility in various matrices
In vitro assays have been employed to assess estrogenic activity in a range of environmental matrices. Various aquatic samples, such as wastewater treatment plant influents and effluents (Körner et al., 1999, 2001; Kirk et al., 2002; Murk et al., 2002; Onda et al., 2002; Tilton et al., 2002; Vethaak et al., 2002; Huggett et al., 2003), surface water (Oh et al., 2000; Khim et al., 2001; Thomas et al., 2001; Witters et al., 2001; Murk et al., 2002; Vethaak et al., 2002; Fenet et al., 2003) or agricultural drain water (Johnson et al., 1998) have been analysed by in vitro assays. For wastewater and surface water both ER binding assays, ER-CALUX, MVLN cell assays, YES and E-screen have been utilized (Körner et al., 1999, 2000, 2001; Oh et al., 2000; Behnish et al., 2001 Shen et al., 2001; Snyder et al., 2001; Witters et al., 2001; Murk et al., 2002; Onda et al., 2002; Vethaak et al., 2002; Huggett et al., 2003). To the knowledge of the author, the only published study utilizing an in vitro assay for assessment of estrogenic activity in agricultural drain water was the ER binding study performed by Johnson et al. (1998). Likewise, to the knowledge of the author, only a single study has been published on the employment of in vitro assays on manure samples (Vethaak et al., 2002). In this study the ER-CALUX assay was used to assess estrogenic activity in two liquid cattle manure samples.
In vitro assays may be subject to interferences owing to the complexity of environmental samples. Toxicity of the samples to the yeast or mammalian cells is a potential problem in assessing estrogenic activity in an environmental sample. Cytotoxic effects at high sample concentrations have been observed in both the ER-CALUX assay (Legler et al., 2002a), the MVLN cell assay (Khim et al., 1999, 2001), the E-screen (Körner et al., 2001) and the YES assay (Andersen et al., 1999; Payne et al., 2000; Witters et al., 2001). Toxic effects cause irregularities in the concentration-response curves for estrogenic activity. Consequently, toxic effects should be carefully distinguished from estrogenic responses, and the assays should not be run with sample concentrations that affect cell viability. Dilution of the samples may reduce toxicity to the cells but also reduces the possibility of detecting estrogenic activity. Yeast assays may perform better for monitoring of environmental samples, as these samples are frequently contaminated with substances other than (xeno)estrogens interfering with the growth and viability of animal cells, but not with yeast cells (Graumann et al., 1999). Yeast is more resistant to environmental contaminants such as heavy metals and bacterial endotoxins compared to mammalian cells (Breihofer et al., 1998). In a survey of water samples from Flemish freshwater systems the MVLN cell assay and the YES assay were compared (Witters et al., 2001). Significant cytotoxicity for a number of samples was found in the MVLN cell assay compared to the YES assay.
Körner et al. (1999, 2000) used the E-screen for the quantitative determination of total estrogenic activity in extracts from municipal sewage plants. As no cytotoxic effects occurred in the range of dilutions where a dose-response relationship of the estrogenic activity was observable, it was possible to analyse the samples quantitatively in the E-screen assay without any cleanup step. To exclude the theoretical possibility that a sample may stimulate proliferation of MCF-7 cells by mechanisms that are separate from an interaction with the ER an active concentration of each sample was analysed together with the ER antagonist ICI 182,780. Cell proliferation induced by the sewage samples was completely inhibited by coincubation with ICI 182,780. This verified that the cell proliferation caused by the samples was ER-mediated.
5.7 Advantages and limitations compared to chemical analyses
Significant advantages of in vitro assays over chemical analyses are that no unknown components with estrogenic activity are overlooked and that any combination effects are taken into account in the analysis. Although chemical analyses are important for the identification and quantification of compounds, they give no information about the estrogenic potency of those compounds. In vitro assays provide an integrated measure of the combined potency of all compounds in a sample. The wide structural diversity of (xeno)estrogens sets a limit to single compound chemical analysis of environmental matrices regarding time and labour. Therefore, in vitro assays are essential for monitoring environmental samples for their content of substances with estrogenic activity regardless of their chemical nature. Moreover, in vitro assays can detect compounds for which there are no analytical methods available.
If both chemical analyses and in vitro assays are performed on an environmental sample, and if the in vitro estrogenic activity of the chemicals measured is known, it is possible to determine which proportion of the total estrogenic activity can be ascribed to the (xeno)estrogenic chemicals analysed. In order to compare the in vitro estrogenic activity measured in various environmental samples with the chemically detected concentrations of known (xeno)estrogenic compounds, results of the chemical analyses of a sample can be expressed as theoretical EEQ values. By summing all the EEQ values for single compounds in a sample, the total calculated EEQ in that sample can be determined assuming concentration additivity. If the EEQ levels calculated are lower than the measured in vitro EEQ levels, other compounds, that were not chemically analysed in the study may be present in the extracts and also contribute to estrogenic activity. If the calculated EEQ values are higher than in vitro EEQ values, there may be several explanations: (i) calculation of EEQ values based on detection limits causes an overestimation of the EEQ (Murk et al., 2002; Vethaak et al., 2002); (ii) differences in extraction methods; and (iii) unidentified compounds may antagonise estrogenic compounds in the in vitro assay.
In the published studies using this comparative approach, the in vitro estrogenic activity is often found to be lower than the chemically measured estrogenic activity. The EEQ values of effluents of sewage treatment plants in Southwestern Germany as determined by the E-screen were compared to those calculated from the results of chemical analysis of 13 natural and synthetic estrogenic substances (Körner et al., 2001; Spengler et al., 2001). For the majority of the samples, the EEQ calculated from the GC-MS results was higher than that determined in the E-screen assay by a factor 2 to 4, whereas on the other hand, no sample had an EEQ in the cell culture test higher than that derived from chemical analysis. Murk et al. (2002) demonstrated that the calculated EEQ values in the surface waters in the Netherlands were 5 to 10 times higher than EEQ values measured in the ER-CALUX assay. Similar results were found by utilizing the YES assay on effluents from North American municipal wastewater (Huggett et al., 2003). Likewise, lower in vitro activities in the MVLN cell assay versus measured concentrations were observed in Nevada wastewater evaluations (Snyder et al., 2001) and in Taihu Lake, China (Shen et al., 2001). The Dutch national survey (Vethaak et al., 2002) and pilot study (Belfroid et al., 1999) compared the calculated estrogenic activity and in vitro estrogenic activity measured in the ER-CALUX assay . In the pilot study, the compounds studied accounted for 70% of the estrogenic activity of untreated wastewater. The corresponding value for wastewater treatment plant effluents was only 20%. For surface water, on the other hand, the measured activities were much lower than the calculated activities. In the national survey, some of the sewage water plant effluents and surface water samples had calculated EEQ levels below the measured in vitro EEQ levels. However, calculated EEQ values were higher than in vitro EEQ values by one order of magnitude for most of the extracts.
In vitro assays facilitate identification of environmental samples that warrant additional chemical analysis. If no significant response is detected in vitro, there may be no need to conduct expensive and time consuming chemical analyses. Since the method detection limit is known for the in vitro assay, an upper limit of EEQ in the sample can be defined. However, if a sample produces a significant response in an in vitro assay, chemical analysis can be employed to uncover the cause. Fractionation of sample extracts showing significant activities, and subsequent reanalysis with the in vitro assays can identify important classes of compounds that are responsible for the observed activity. Chemical analysis is utilized to determine the compounds likely responsible for the activity observed in each fraction. Once candidate compounds have been identified, a theoretical estrogenic activity can be estimated as the summation of the potency of the single compounds based on identification and quantification by chemical analysis. This theoretical estrogenic activity may be compared to the actual estrogenic activity detected in the in vitro assay to find out if all of the estrogenic activity has been identified.
A combination of in vitro assays and chemical techniques has been utilized in studies on the effluents from sewage treatment works to both confirm that they exhibit estrogenic activity and to identify the compounds that were responsible. Such a study was carried out in the United Kingdom, following the observation that sewage effluent was estrogenic to fish, in an attempt to identify the causative compounds (Desbrow et al., 1998). Samples were fractioned and the YES assay was employed to identify the fractions that contained estrogenic activity. The active fractions were then analyzed using GC-MS to enable elucidation of the compounds present. A similar approach has been used to identify estrogenic compounds in effluents from a sewage treatment works in Michigan (Snyder et al., 2001).
5.8 Limitations compared to in vivo assays
Although in vitro assays are attractive for monitoring studies, one of their main disadvantages is their simplification of the in vivo situation. In vitro assays are mechanism specific, whereas in vivo assays permit the detection of effects resulting from multiple mechanisms. In vitro assays do not completely account for complex in vivo events, such as bioavailability, toxicokinetics, metabolism and cross talk between biological pathways (Zacharewski, 1997). For example, in vitro assays are limited in their ability to mimic whole animal metabolism. As a result, extrapolation from in vitro to in vivo assays can lead to false negatives for compounds that are bioactivated, and overestimates of potency for compounds readily inactivated in vivo. Though some metabolic capacity has been observed in some in vitro assays (Soto et al., 1995; Sohoni and Sumpter, 1998; Andersen et al., 1999; Legler et al., 1999; Tyler et al., 2000; Layton et al., 2002), the complex processes of uptake, binding to carrier proteins, transport, targeting and disposition of compounds in whole animals are not taken into account in the in vitro systems (Zacharewski, 1997). In addition, bioaccumulation and homeostatic controls generally are not simulated by in vitro testing systems. In vitro assays would not reveal compounds that exert (anti-)estrogenic responses in vivo by modifying endogenous E2 levels via effects on the hypothalamus or pituitary. In vivo assays can integrate estrogenic effects that may occur by different mechanisms in multiple tissues simultaneously. Fish can serve as integrators of responses to mixtures of toxicants that occur in the aquatic environment and are thus useful in vivo models for studying effects of estrogenic compounds in water. In addition, effects related to growth and reproduction in fish are more easily related to population level and ecological effects than are effects in in vitro systems. Thus, in vivo studies in both the field and laboratory are essential for linking exposure to biologically relevant effects. They are, however, impractical for routine, high throughput monitoring of environmental samples. In vivo studies are in general more laborious, time consuming and costly compared to in vitro assays, and may involve large amounts of animals. Furthermore, considerable inter-individual variations often exist in responses. Utilization of in vitro assays circumvents much of the inter-individual, seasonal, and temporal variability, which can complicate interpretation of in vivo responses. Additionally, in vitro assays avoid many of the ethical issues associated with whole animal studies. However, due to the clear drawbacks of in vitro assays that might result in unreliable predictions, a combination of test methods, including in vivo assays, which assess both the ER- and non-ER-mediated mechanisms of action is suggested as most appropriate to determine the estrogenic activity of environmental matrices.
It is not uncommon for in vitro estrogenic activity to differ from in vivo activity. An investigation was made to determine how accurately two in vitro assays (YES and E-screen) predicted responses of estrogenic compounds in inducing vitellogenin synthesis in sheepshead minnow (Folmar et al., 2002). The results showed major discrepancies between the relative estrogenic activities of the xenoestrogens methoxychlor and nonylphenol in the in vitro assays relative to the in vivo assay. The xenoestrogens were clearly more potent in vivo than in the in vitro assays. Likewise, another study showed that the relative potency of (xeno)estrogens in the YES assay did not reflect potency in a medaka assay (Metcalfe et al., 2000). Segner et al. (2003) compared potencies of estrogenic compounds in in vitro assays (YES, ER-receptor binding and vitellogenin induction in cultured fish hepatocytes) and in life cycle tests with zebrafish in vivo. The in vivo activity of the test compounds was not accurately predicted by the in vitro assays, with respect to neither sensitivity nor ranking. The in vitro assays tended to overestimate the relative potency of the xenoestrogens; i.e. the ratio between the EC50 of the reference compound, EE2, and that of the test compound. The best prediction of the in vivo fish test results was obtained from the YES assay. Although still being clearly less sensitive than the in vivo test, the YES assay showed the highest absolute sensitivity of the in vitro test systems. The sensitivity difference between the YES and zebrafish assay was most pronounced for EE2 (about two orders of magnitude) while it was smaller for the xenoestrogens (about one order of magnitude). To compare in vitro and in vivo assays, wastewaters from North American municipal wastewater facilities were evaluated for estrogenic activity using the YES assay and an in vivo fish vitellogenin assay (Huggett et al., 2003). In vivo estrogenic activity was nearly 10-fold greater than YES activity. In addition to the already mentioned risks of cytotoxic effects in in vitro assays, other potential explanations for enhanced in vivo activity are enhanced bioavailability/absorption of compounds by the fish relative to in vitro cells, bioaccumulation in vivo, and/or the occurrence of non-ER ligands, which elicit estrogenic effects in fish through indirect mechanisms (Folmar et al., 2002; Huggett et al., 2003).
Compounds with similar estrogenic activities in vitro sometimes possess very different activities in vivo. Several in vitro assays (ER binding assays, ER-CALUX, MVLN cell assay, HGELN cell chimeric receptor/reporter gene assay, YES and E-screen) have displayed approximately the same estrogenic activity of E2 and EE2 (Gutendorf and Westendorf, 2001; Shen et al., 2001; Murk et al., 2002) while EE2 in vivo has been found to have approximately 10-100 times higher estrogenic activity than E2. For example, EE2 has been reported to be 16-fold more potent than E2 in inducing vitellogenin synthesis in male zebrafish (Rose et al., 2002), 11- to 27-fold fold more potent than E2 in inducing vitellogenin synthesis in juvenile rainbow trout (Thorpe et al., 2003) and 100 times more potent than E2 in inducing testis-ova in Japanese medaka following exposure during early life-stages (Metcalfe et al., 2001). Legler et al. (2002b) likewise found considerable differences in the relative potencies of (xeno)estrogens in vitro and in vivo. EE2 was the most potent of the (xeno)estrogens tested and was 100 times more potent than E2 in an in vivo transgenic zebrafish assay whereas in the ER-CALUX assay, EE2 was only slightly (1.2 times) more potent than E2. For wastewater treatment plant effluent extracts the average measured EEQ value in the ER-CALUX assay was 3 ng/l whereas for the transgenic fish assay the corresponding value was 51 ng/l (Legler, 2001). Thus it was demonstrated that the effluent samples were more estrogenically potent in vivo than would be expected based on their in vitro estrogenic activity. Similarly, Huggett et al. (2003) found that in vivo estrogenic activity of effluent from North American municipal wastewater facilities was nearly 10-fold greater than in vitro estrogenic activity assessed by the YES assay . The results from the above studies suggest that the sole use of in vitro assays to monitor for estrogenic activity may severely underestimate in vivo estrogenic potential of environmental samples and thus could potentially result in false negative evaluations.
| Front page | | Contents | | Previous | | Next | | Top |
|