| Forside | | Indhold | | Forrige | | Næste |
Binding af nikkel til og frigivelse fra naturlige kalksedimenter
Summary and conclusions
In this chapter the major results of the project are described. The chapter is written as an article to the Danish EPA journal “Ny Viden”.
New aspects of nickel related water quality problems in limestone aquifers: Sorption and secondary release from limestone aquifer sediments.
Elevated nickel concentrations in the groundwater of limestone aquifers as a result of primary nickel release due to oxidation of nickel containing sulfides, such as pyrite (FeS2), are well known and well
documented. New investigations show that a significant part of the nickel released by the oxidation process secondarily sorbs to the limestone sediments. Consequently, a secondary pool of nickel
accumulates in the limestone aquifer sediments. When changes in the hydrogeological and/or hydrogeochemical conditions occur, release of the secondary pool of nickel may lead to elevated nickel
concentrations in the groundwater. At well fields, secondary release of nickel can lead to elevated well water nickel concentrations when the groundwater level rises after a period where barometric pumping
through the well field borings has occurred. A conceptual model based on results from the investigation indicate that nickel transport over long distances in limestone aquifers apparently only in rare cases can
cause nickel drinking water concentrations exceeding the 20 µg/L drinking water limit.
Background and aim of project
Elevated nickel concentrations in groundwater are a serious water quality problem to many water supplies located in the eastern part of Zealand. In this part of Denmark, groundwater is extracted from
limestone aquifers for drinking water purposes. Most often, nickel problem areas are located where the limestone formation is vertically elevated and capped only by thin clayey till deposits, and in the same
time where extensive pumping has caused a change from confined to unconfined aquifer conditions.
The release of nickel in limestone aquifers due to barometric pumping through water supply wells has been reported in the Danish Environmental Protection Agency (EPA) Environmental Project: “Release of
nickel by pyrite oxidation due to barometric pumping/breathing”, published in January 2003. The main conclusion of this report is that oxidation of sulfide minerals due to barometric pumping, as well as the
concomitant release of nickel to the groundwater, is a local problem occurring at a distance of up to 100 m from the breathing well. Assessment of the potential of nickel transport over long distances in
limestone aquifers, e.g., as relevant to nickel mobilization by nitrate oxidation of pyrite, is not presented in the 2003 Danish EPA report.
In addition, the results of the 2003 Danish EPA report indicate that nickel released by pyrite oxidation is subsequently sorbed, at least to some extent, to the limestone aquifer sediments. However, it has not
been assessed whether this pool of secondarily bound nickel can be remobilized and cause further water quality problems to the water supplies, though this might be a problem. Thus, during the last ten
years, several of the water supplies in the eastern part of Zealand, e.g. the water supplies of the cities Brøndby and Tårnby, have observed a general increase in nickel and sulfate concentrations concurrently
with a general increase in the groundwater level. Based on the previous investigations of 2003, it is not possible to evaluate whether this increase is caused by a secondary release of nickel from the limestone
sediments or whether it is due to hydrogeological processes only.
Therefore, the overall objective of the present investigation is to gain knowledge about the sorption of nickel to the various types of limestone sediments that constitute the aquifers of Eastern Zealand. As part
of this, the objective is to assess the mobility of nickel in these types of aquifers.
Methodology
The present investigation comprises laboratory and field work as well as numerical model calculations.
Laboratory experiments have tested nickel sorption to and desorption from natural limestone sediments. The limestone sediments used for the laboratory experiments represent the main limestone lithologies
present in the Eastern Zealand limestone aquifers. In addition, the sorption and desorption experiments were carried out using the pure minerals calcite and glauconite, in order to represent experimental end
member lithologies. The sorption and desorption of nickel was investigated at varying aqueous nickel and calcium concentrations.
To investigate specifically the secondary release of nickel, a limestone sediment sample, naturally enriched with nickel, was selected from a sediment core collected near the city Brøndby, as part of the
investigation. As for the other experiments, the secondary release was tested at varying nickel and calcium concentrations.
The field investigations were conducted at Brøndby Water Supply, at which increased nickel concentrations are present in the groundwater. The field experiments comprise studies of the chemistry of
sediment and water as well as hydrogeological studies.
Finally, a number of simple conceptual numerical models have been set up, serving a basis for an evaluation of the mobility of nickel in fractured limestone aquifers.
Main conclusions
Based on the laboratory and field investigations, in combination with the simple conceptual models, the following conclusion can be made:
- Limestone sediment samples of the Lellinge Greensand Formation, the Danien Limestone and the Maastrichtian Chalk are capable of binding nickel to such an extent that it significantly affects the
mobility of nickel in limestone aquifers.
- The sorption and desorption properties of nickel to the limestone sediment is controlled by the aqueous calcium concentration. For operational purposes, nickel sorption and desorption can be
modelled by a simple ion exchange model, including ion exchange of nickel for calcium on the sediment surface.
- The predominant part of the nickel which is released by pyrite oxidation and subsequently sorbed to limestone sediments may later be flushed from the aquifer, if the primary release due to pyrite
oxidation stops/is blocked. However possibly, a very small part of the nickel remains irreversibly bound to the sediment.
- Desorption of nickel can in most cases explain the often observed pattern of increasing nickel concentrations in response to reestablishment of the water table to confined conditions after a period of
unconfined conditions where barometric breathing has taken place. Local hydrogeological variations such as the number and magnitude of in-flow zones to the well and hydraulic barriers such as flint
layers can influence the significance of the secondary release of nickel in a well field.
- Based on simple conceptual model calculations it is not likely except in rare cases (high fracture aperture and flow velocity, and high nickel concentrations at the source) that nickel mobilization over
large distances (>500 m) in limestone aquifers cause elevated nickel concentrations above 20 µg/L within a short period of time (<50 years). In contrast, nickel mobilization over short distances (<500
m) is a likely reason for elevated nickel contents in pumped groundwater.
Project results
Limestone sediments are capable of binding nickel
The sorption of nickel to the natural limestone sediments was investigated at varying aqueous nickel concentrations between 4 and 800 µg/L. In the sorption experiments, the calcium concentration was kept
constant at approximately 190 mg/L, and the pH was approximately 6.7. The results from these experiments show that all sediment samples used are capable of binding nickel.
Pure glauconite is the strongest sorbent per mass unit and pure calcite is the least efficient sorbent per mass unit among the investigated sediments (figure A1). In correspondence with this finding, the
laboratory experiments show that the higher the percentage of clay minerals in the sediment, the stronger a sorbent per mass unit. Thus, per mass unit, the sorption of nickel to sediment from the Lellinge
Greensand Formation is stronger than sorption to the sediment samples comprising Danien Limestone or Maastrichtian Chalk. Hence, the results indicate that the clay content of the limestone sediment
samples may partly control the nickel sorption. However, for Danien Limestone and Maastrichtian Chalk it is assessed that sorption takes place to both clay and carbonate in the sediments.
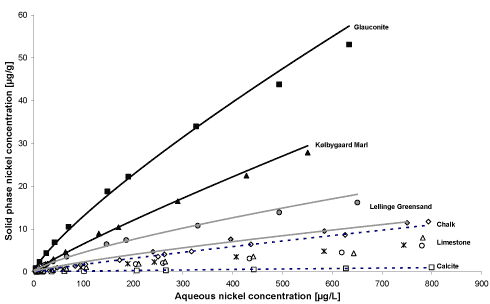
Figure A1: Sorption isotherms showing the sorption of nickel to the various sediments studied in the project.
Secondary release of nickel – implications for management of well fields
As a consequence of the sorption of nickel to the limestone sediments, it can be established that in areas where barometric breathing has caused primary release of nickel by pyrite oxidation, a significant pool
of secondarily sorbed nickel is present in the zone, where pyrite oxidation has taken place previously. When changes in the hydrogeological or hydrogeochemical conditions take place, the secondary pool of
nickel can be released to the groundwater by desorption. Thereby, the secondary pool of nickel may cause elevated nickel concentrations in the abstracted groundwater at water supplies where barometric
breathing has taken place. This is the case at the field locality at Brøndby, where a previously unsaturated zone of the aquifer recently has been submerged due to a general increase of the groundwater level.
In this zone of the aquifer, where pyrite oxidation previously took place, the present nickel content of the sediment is up to 4 µg/g and the corresponding aqueous nickel concentration is up to 400 µg/L.
As mentioned, the secondary release of nickel at Brøndby takes place due to the general increase of the groundwater level which has taken place since the early 1990’s. Thus, the actual reason to the nickel
concentrations in the pumped groundwater exceeding the drinking water limit of 20 µg/L is the secondary release of nickel. This is the case even though the nickel problem was primarily created because of
barometric breathing at the abstraction wells. Thus, the nickel concentration of the abstracted groundwater increases each time the groundwater level increases (figure A2).
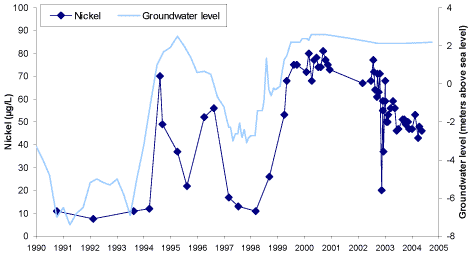
Figure A2: Groundwater level and nickel concentration in the groundwater at well no. 207.1335 of the Brøndby Water Supply.
At Brøndby, several fractured zones are present in the limestone aquifer. In-flow to the abstraction wells takes place from all of these zones, which hydraulically are separated due to the presence of flint
layers in the aquifer. The deep water-bearing zones of the aquifer contain acceptable concentrations of nickel, which is because the deep zones has not been affected by pyrite oxidation to the same extend
as the more shallow water bearing zones. Hence, the solution to the nickel problem at Brøndby is to minimize the in-flow to the abstraction wells from the shallow water-bearing zones. At Brøndby, this is
done by changing the construction – e.g. the filter interval – of the abstraction wells.
Nickel sorption is reversible and depend on the aqueous calcium concentration
Selected sediment samples where used to study the sorption of nickel at varying calcium concentration between 20 and 1400 mg/L. The results from these experiments show that the sorption of nickel to the
limestone sediments depends on the aqueous calcium concentration. Thus, the sorption is stronger, the lower the aqueous calcium concentration (Figure A3).
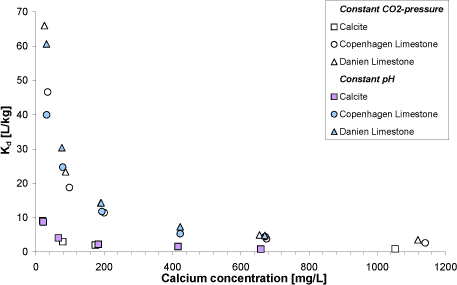
Figure A3: Nickel distribution coefficient at carbonate rich sediment samples (insoluble residue < 1.5 % (w/w)) as a function of the aqueous calcium concentration. Open/white symbols show results from
sorption experiments carried out at variable pH and constant CO2 partial pressure. Blue symbols show results from sorption experiments carried out at constant pH (6.74 ± 0.02) and variable CO2 partial
pressure.
In correspondence with the results from the sorption experiments carried out at variable calcium concentration, the nickel release experiments show that the release of nickel from nickel enriched sediments
increase with increasing calcium concentration. Regarding sediments with a high carbonate content (Danien Limestone and Maastrictian Chalk samples), the relation between nickel sorption/desorption and
aqueous calcium concentration can, for practical purposes, be described by a simple ion exchange model
Furthermore, the nickel release experiments show that the major pool of the secondary sorbed nickel is readily desorbable. Consequently, within a relatively short period of time, it is possible to achieve
acceptable nickel concentrations in the groundwater at abstraction wells where pyrite oxidation has previously been causing primary release of nickel. However, the prerequisite is that the primary release of
nickel by pyrite oxidation is stopped. The duration of the period where secondary mobilization of nickel takes place depends among others on the local hydrogeological setting, the aqueous calcium
concentration, and the quantity of nickel previously released by pyrite oxidation.
Implications for transport of nickel in limestone aquifers
As a result of the nickel sorption to the limestone sediments, the transport of nickel in limestone aquifers is retarded compared to conservative solute transport. Thus, the mobility of nickel in limestone
aquifers is lower than e.g. of chloride. Due to the sorption dependency on the aqueous calcium concentration, the Kd for nickel in the limestone aquifers may vary with a factor of 2-4. According to the
laboratory experiments, the typical Kd for nickel in aquifers of Danien Limestone or Maastrichtian Chalk is between 8 L/kg and 30 L/kg, while for Lellinge Greensand, the typical Kd is between 50 L/kg and
100 L/kg.
The limestone aquifer is a double permeable medium, where groundwater flow and solute transport takes place in both the matrix and in fractures. Exchange of solutes between fractures and matrix takes
place by diffusion, and the storage of solutes mainly occurs in the matrix.
In order to assess, in general, the mobility of nickel in limestone aquifers, the Kd values observed in the laboratory were used in simple conceptual and numerical model set ups. Generally, the numerical
models were set up based on knowledge about aperture of fractures, distance between fractures, etc., from a previous field study at Tune.
Calculations with the numerical models show that the transport of nickel in limestone aquifers depends highly on the fracture aperture, and thereby the fracture flow velocity. Thus, it is the combination of
nickel sorption and the occurrence of fractures that control the transport of nickel in limestone aquifers.
Based on the numerical models, it can be assessed that in zones of the limestone aquifers with medium-to-low flow velocities (2*10-6 m/s < Kbulk < 2*10-4 m/s), the present nickel problems in Eastern
Zealand are most likely due to transport of nickel within a short distance (<500 m) from the abstraction wells.
Often, in the upper part of the Danish limestone aquifers, a highly fractured zone is present. In this zone, the hydraulic conductivity is typically up to 10 times greater than the hydraulic conductivity used in the
numerical models. However currently, knowledge about fracture apertures and fracture distances in this zone is not well established. Thus, the transport of nickel in such highly fractured zones is difficult to
assess and estimate.
Thus, in the present investigation, hypothetical models simulating the transport of nickel in highly fractured zones were set up. Based on these hypothetical models, it is assessed that it is possible only in rare
cases to exceed the drinking water limit for nickel of 20 µg/L, because of regional transport of nickel.
Hence, it is assessed that the present nickel problems in Eastern Zealand are mainly due to hydrogeochemical reactions taking place locally at affected abstraction wells. Therefore, new abstraction wells,
which are unaffected by nickel, can presumably be established within a short distance (400-500 m) from existing abstraction wells with nickel problems.
| Forside | | Indhold | | Forrige | | Næste | | Top |
Version 1.0 Marts 2006, © Miljøstyrelsen.
|