| Forside | | Indhold | | Forrige |
Stimuleret in situ reduktiv deklorering. Vidensopsamling og screening af lokaliteter
Appendiks G Erfaringer fra Holland
Contractor: COWI A/S, Denmark
Project title: In-situ enhanced reductive dechlorination
Experiences in the Netherlands
Project code: 2003.2161
Document type: Report
Publication date: December 17th, 2003
Project leader: M.Sc. M.J.C. Henssen
Author(s): M.Sc. N.J.P. van Ras, M.Sc. M.J.C. Henssen
Keywords: bioremediation, reductive dechlorination, in-situ, chlorinated solvents
Bioclear b.v.
Post address:
P.O. Box 2262, 9704 CG Groningen
The Netherlands
Visit address:
Rozenburglaan 13c, Groningen
The Netherlands
Telephone: 31 (0) 50 571 8455
Telefax: 31 (0) 50 571 7920
E-mail: info@bioclear.nl
Website: www.bioclear.nl
All rights reserved. No part of this material may be reproduced or utilized in any form or by any means, electronic or mechanical, including photocopy, recording or by any informa.tion storage and
retrieval system, without written permission from Bioclear.
© Bioclear b.v.
Bioclear supports industry, authorities, engineering companies and institutes in the area of environmen.tal technology
For all orders to Bioclear the General Conditions for research orders to Bioclear, as registered at the Chamber of Commerce at Groningen, are applicable.
1. Introduction
At the request of Mr. N.E. Bordum of COWI A/S in Denmark, an overview has been given concerning the status and use of Enhanced Reductive Dechlorination (ERD) in the Netherlands
as a remediation approach for sites contaminated with chlorinated ethenes. The activities have been performed according to the proposal with reference 2003.2161/1717, dd. 29 September
2003 and confirmation with reference P-058326-A-1/NBR, dd. 3 October 2003.
The goal of the review study is to provide a broad overview of ERD, including scientific knowledge, but also practical experiences. Bioclear has extensive knowledge and practical
experience in the field of ERD. This knowledge has been developed in the Netherlands over the last 15 years. In this report, the status of ERD in the Netherlands, the most important
practical experiences and the goals that have been achieved are discussed. The emphasis is put on the use of ERD in clay soils.
The following information is provided:
1. the approach used in the planning phase of ERD, and the use and function of laboratory testing in this approach;
2. ways of delivery of substrates, and the types of substrates used;
3. how to handle regulatory permits for introduction of chemicals and microbes into the subsurface;
4. an overview of the remediations that have been performed and/or are currently performed with ERD, including data on soil type, contaminants, types of substrates, redox conditions,
project scale and method of substrate delivery.
In chapter 2, a general overview of reductive dechlorination is given, and the situation concerning the redox conditions and organic carbon content in the Netherlands is presented. In
chapter 3, the process to evaluate the possibilities of ERD is presented, together with a description of the use and function of field and laboratory testing. In chapter 4, electron donors and
techniques to deliver the electron donors that can be used are presented and practical experiences are given. In chapter 5, the legislation in the Netherlands concerning the introduction of
chemicals and micro organisms is presented. Chapter 6 provides an overview of ERD projects in the Netherlands.
2. Reductive dechlorination
2.1. Degradation of chlorinated ethenes
Over the last two decades, numerous laboratory and field studies have shown that chlorinated ethenes can be degraded to harmless end products by micro-organisms. The degradation
process can occur through three different processes:
- mineralisation, the contaminant is used as an electron donor;
- co-metabolic degradation, in which the degradation of the contaminant is fortuitous, without benefit for the micro-organism;
- reductive dechlorination, in which the contaminant is used as an electron acceptor.
Reductive dechlorination is the most important process for the degradation of chlorinated ethenes. During this process, the chlorinated ethenes are used as electron acceptor. Stepwise a
chlorine atom is removed and replaced with a hydrogen atom. In general, this process occurs by sequential dechlorination from PCE to TCE to DCE (mainly the cis-isomer) to VC and then
to ethene and/or ethane. The chlorine atoms are released as chloride ions.
2.2 Prerequisites for reductive dechlorination
When favourable conditions prevail, chlorinated ethenes can be degraded to harmless end products like ethene and ethane by specific micro-organisms. For complete degradation of
chlorinated ethenes by reductive dechlorination, the following prerequisites have to be met simultaneously:
- presence of strongly reduced conditions (methanogenic);
- sufficient electron donor (the `substrate' for dechlorinating micro organisms) of good quality;
- presence of specific micro organisms capable of degrading chlorinated ethenes to harmless end products.
Other preconditions are a favourable pH (5.5 – 8.5), the presence of sufficient nutrients (nitrogen and phosphorous sources) and trace elements and the absence of toxic compounds that
limit biological activity.
2.3. Situation in the Netherlands
In the coastal provinces of the Netherlands the soil mainly consists of clay and peat. In these soils, the organic carbon content is often high (up to 40 to 50 mg/l) and sufficient to sustain
intrinsic reductive dechlorination of chlorinated ethenes. Because of the high organic carbon content, redox conditions are often favourable for reductive dechlorination. A natural
attenuation approach can be a feasible technique for contaminations with chlorinated ethenes. In the Eastern and Southern parts of the Netherlands, the soil mainly consists of sand with
low organic carbon content and unfavourable redox conditions. Natural attenuation is only feasible if another anthropogenic contamination is present which can be used as electron donor,
in sufficient quantities. As this is rarely the case, the biological degradation of chlorinated ethenes has to be stimulated by adding an electron donor (ERD) or an electron donor and
dechlorinating micro-organisms (bio-augmentation).
Based on the results of numerous field investigations, a chart of the Netherlands has been made to visualize the capacity for natural attenuation based on the organic carbon content of the
groundwater (figure 1).
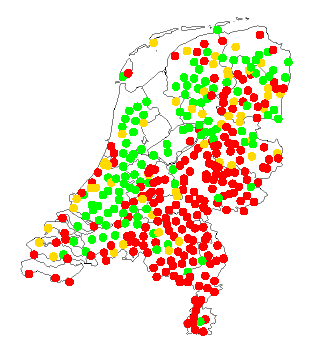
Figure 1. Capacity for natural attenuation based on organic carbon content at a depth of 10 m-bgl in the Netherlands
=sufficient organic carbon, = neutral balance = insufficient organic carbon
Such charts can be used in an early stage of the site investigation to gain insight in the possibilities for natural attenuation of a chlorinated ethenes contamination based on the area the
site is located in. If conditions appear to be favourable, specific parameters can be incorporated in first field investigations aimed at the delineation of the contamination, like analyses for
ethene and ethane, TOC and redox parameters. The decision to investigate biological processes as a (part of the) remediation strategy can then be made early, optimising the spending of
time and budget.
3. Implementing an ERD remediation
3.1. Enhanced reductive dechlorination
A contamination with chlorinated ethenes can be remediated using biological reductive dechlorination processes. As mentioned before, the prerequisites for reductive dechlorination have
to be met simultaneously. In some cases, the conditions at a contaminated site already satisfy the prerequisites for reductive dechlorination, without any technical `interference'. In these
cases, natural attenuation might be a suitable remediation approach. When the prerequisites are not met, the conditions may be optimised by adding a suitable electron donor (enhanced
reductive dechlorination). The electron donor is used to reduce electron acceptors (oxygen, nitrate, trivalent iron and sulphate) present or supplied by inflowing groundwater. This creates
highly reduced conditions that are favourable for the degradation of chlorinated ethenes. In addition, the organic material is used as electron donor for the reductive dechlorination of the
chlorinated ethenes.
In some cases, the addition of an electron donor is not sufficient to stimulate the reductive dechlorination because no micro-organisms are present that can degrade the chlorinated
ethenes to harmless end products. This is often encountered in areas with sandy aquifers where the groundwater has elevated concentrations of nitrate and/or sulphate and a low
concentration of electron donor (TOC). The conditions have not been favourable for the development of a microbial community that is capable of complete reductive dechlorination. At
these sites, the addition of an electron donor and a microbial dechlorinating consortium can be the solution to achieve biological remediation. This is called bio-augmentation. The addition
of the electron donor reduces the groundwater until favourable redox conditions prevail, the amount of electron donor for the reductive dechlorination process is increased and the addition
of dechlorinating micro-organisms leads to a rapid and complete breakdown of the contamination.
3.2. Approach
In figure 2, the approach used in initial site investigation is visualized. During initial site investigation, the contaminant situation is determined and the `urgency' and `deadline' before the
remediation must be started is defined. As mentioned before, the incorporation of specific parameters in this initial site investigation can help selecting appropriate remediation techniques
for the site.
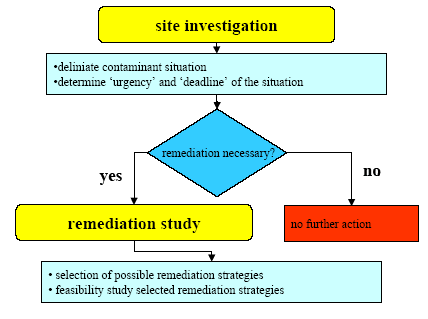
Figure 2. Approach initial site investigation
To evaluate the possibilities for reductive dechlorination to remediate a site and to decide what remediation strategy is necessary to achieve the remediation targets, a feasibility study
has to be performed. This study typically consists of the following successive activities:
- a groundwater characterisation to determine the conditions that are present at the site;
- anaerobic degradation tests to evaluate the effect of the addition of an electron donor and, if necessary, a dechlorinating microbial consortium, and to determine degradation rates;
- three-dimensional modelling to predict the evolution of the contaminant plume when the selected remediation approach is implemented;
- evaluation of the feasibility by comparison of the predicted results with the remediation targets.
The approach for this feasibility study is visualized in figure 3.
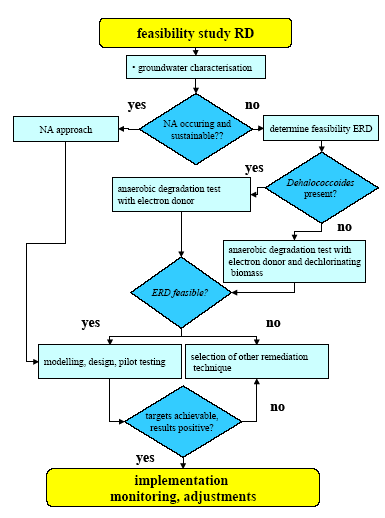
Figure 3. Approach feasibility study ERD
3.2.1. Groundwater characterisation
To determine the conditions at a contaminated site, a groundwater characterisation is performed. This activity consists of sampling, anaerobic field measurements and laboratory analyses
on groundwater samples from the contaminated site.
Samples should be taken from monitoring wells upstream from the site (references), in the source, in the plume parallel to the flow line and at right angles to the flow line (figure 4).
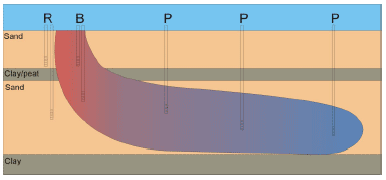
Figure 4. Diagram of a plume and the sampling strategy (left cross section, right top view), with monitoring wells in the source (B), in the plume parallel to the flow line (P), in the plume at right angles to the
flow line (L) and upstream from the source (R).
The number of monitoring wells required depends on the location and is determined by the complexity of the site. Aspects such as soil structure (presence of more water discharge layers),
the number of sources of contamination (chlorinated ethenes and secondary organic contamination) and any decontamination work that is underway play an important role here.
To determine the conditions that are present in the groundwater, on-line field measurements and laboratory analyses have to be performed (table 1).
Table 1. Monitoring parameters groundwater characterisation
Field measurements |
Laboratory analyses |
|
redox parameters |
electron donor, N en P |
contaminants, degradation products |
micro organisms |
Redox potential |
nitrate |
TOC |
PCE, TCE, c/t-DCE, |
Dehalococcoides |
Oxygen |
dissolved iron |
ammonium |
VC |
ethenogenes |
Conductivity |
sulphate, sulphide |
phosphate |
ethene and ethane |
|
pH |
methane |
|
chloride |
|
Temperature |
|
|
|
|
Because several parameters are susceptible for volatilisation or chemical oxidation, it is advised to contract specialised companies to perform the groundwater characterisation. For the
detection of Dehalococcoides ethenogenes PCR-techniques (Polymerase Chain Reaction) based on the detection of DNA specific for this organism have been developed, among others by
Bioclear. To date, this is the only isolated dechlorinating micro-organism that is able to degrade chlorinated ethenes completely.
Using existing protocols (for instance the S-NA protocol developed in the Netherlands, available at the website www.skbodem.nl under code SV-513, or the protocol of Wiedemeier et al.,
1998), the results of the groundwater characterisation are interpreted to determine if the preconditions for reductive dechlorination are met:
- determine redox conditions, based on the results of analyses for oxygen, nitrate, iron (total dissolved), sulphate, sulphide and methane;
- determine the quantity of electron donor and nutrients present, based on the results of analyses for TOC, ammonium and phosphate;
- Determine whether dechlorinating micro-organisms are present, indirect by analyses for chlorinated compounds, ethene and ethane and direct by screening for the presence of specific
dechlorinating micro organisms. The issue here is the presence of Dehalococcoides ethenogenes.
When the results of the evaluation of the data show that natural attenuation is not feasible, an enhanced reductive dechlorination remediation should be considered. In the next steps of
the study, the feasibility of enhanced reductive dechlorination is determined.
Typical costs for a groundwater characterisation at a contaminated site range from 6,000 to 8,000 euro. This includes anaerobic sampling of 6 to 8 (existing) monitoring wells, analyses
(see table 1), interpretation and report.
3.2.2. Anaerobic degradation tests
When one ore more limitations for reductive dechlorination are present, these limitations can be solved by adding an electron donor (ERD) or an electron donor and a dechlorinating
microbial consortium (bio-augmentation). The effect of these measures can be evaluated by performing anaerobic degradation tests.
Anaerobic degradation tests are performed using soil and groundwater samples for the contaminated site. These samples have to be collected anaerobically, to prevent the introduction of
oxygen. Also, the preparation of the batch experiments is performed under anaerobic conditions.
The set-up of the experiments is depending on site characteristics (among which are the results of the groundwater characterisation) and the questions that have to be addressed. In order
to evaluate the effect of the addition of an electron donor, tests are performed with and without addition of an electron donor. By regular analyses for chlorinated ethenes, degradation
products, electron acceptors, organic carbon and dechlorinating micro organisms, the dechlorination process is followed over time.
Based on the results it can be determined whether reductive dechlorination is stimulated by the addition of an electron donor and degradation rates can be estimated. To obtain reliable
results, it is advised to use specialized laboratories with experience in performing these degradability studies. A guideline that can be used when performing these tests is the guideline
developed by Bioclear and Wageningen University (NOBIS 94-4-04, available at the website www.skbodem.nl).
If the groundwater characterisation shows that no dechlorinating micro organisms are present at the site, a degradation test can be performed in which an electron donor and
dechlorinating micro organisms are added. Dechlorinating micro organisms can also be added during the incubation, when the preliminary results of the tests with only an electron donor
show that the required conditions are induced but a dechlorinating consortium is not developing fast enough or not at all.
A typical set up for an anaerobic degradation test is given in table 2.
Table 2. Typical set up anaerobic degradation test for a contaminated site
test |
conditions |
analyses |
time scale |
intrinsic |
natural |
contamination, degradation products, redox parameters, TOC, Dehalococcoides
ethenogenes |
once a month, total duration of the tests 6 months |
stimulated |
electron donor |
bio-augmented |
electron donor and dechlorinating micro organisms |
The monitoring program can be optimised based on site characteristics. For instance, if no nitrate is present in the contaminated groundwater, nitrate analyses are not necessary.
The electron donor used in the tests should be the same as is considered for use during full-scale remediation. When desirable, different electron donors can be tested to determine the
optimal electron donor. More information about possible electron donors that can be used to stimulate reductive dechlorination is presented in chapter 4.
Typical costs for an anaerobic degradation test with an incubation time of 6 months and 5 periodic gas chromatography analyses for PCE, TCE, DCE, VC, ethene, ethane and methane are
2,500 to 3,000 euro. The costs for a microcosm study which includes an intrinsic and stimulated anaerobic degradation test range from 5,000 to 6,000 euro. This does not include sampling
for soil and groundwater, necessary to perform the tests.
3.2.3. Modelling and design
Using a geohydrological model, build using site specific data, groundwater flow and velocity can be predicted. Modules that describe solute transport (incorporating retardation, dispersion
etc.) and degradation of the contaminant can then be added to the model to predict the fate of the contamination. Degradation rates for the contaminants can be obtained from anaerobic
degradation tests, in combination with data from literature.
Modelling can be used to show the development of the plume over time under different circumstances. A situation without remedial actions is calculated as a reference, and the influence
of stimulation of the reductive dechlorination process is determined by comparing the results of these model calculations with the reference output. Also, the effect of additional activities
can be determined, for instance core zone removal.
To determine the sensitivity of the model output for input parameters, it is advised to perform model calculations with a certain range of degradation rates and groundwater flow velocities.
The geohydrological model is also an important instrument to design a biological remediation system. Optimal extraction and infiltration well configuration, groundwater flows and system
operation (continuous, intermittent) or, in the case of direct injection, grid design can be determined.
3.2.4. Evaluation feasibility
Based on the results of the modelling study, it is determined if the proposed remedial actions are sufficient to comply with remediation targets and if the proposed remediation approach is
feasible. The output of the modelling can be an important aspect in the procedure to obtain approval of regulatory authorities.
4. Electron donors and techniques
4.1. Types of electron donor
To stimulate reductive dechlorination several electron donors can be used. The most important electron donors that have been used in laboratory studies, pilot testing and full-scale
remediation are given in table 3. A distinction has been made between well-defined, single component electron donors and undefined complex mixtures of electron donors.
Table 3. Possible electron donors to stimulate reductive dechlorination
substance |
source |
single component |
methanol |
product chemical industry |
ethanol |
product chemical industry |
lactic acid |
product chemical industry |
acetic acid |
product chemical industry |
HRC1 |
product chemical industry |
|
|
undefined complex mixtures |
compost percolate |
by product composting facilities |
molasses |
by product beet sugar industry |
protamylasse |
by product potato industry |
vegetable oil |
raw material food industry |
1: HRC (hydrogen release compound) is a trade name of Regenesis, USA and consists of a polylactate ester
In general, single component electron donors are more expensive than complex electron donors, which are usually by products from industrial processes. Because these single component
electron donors do not contain nutrients (nitrogen, phosphorous, trace elements) these components have to be added separately. Also, some single component electron donors like
methanol can be toxic at high concentrations.
Disadvantages of complex electron donors are the presence of suspended solids which may cause infiltration problems. Also, because these complex mixtures contain nutrients and trace
elements, the risk of biofouling of infiltration wells is higher. A good design of the system can prevent such problems.
Eventually, the choice for the electron donor which is used in the field is mainly directed by its suitability to stimulate reductive dechlorination, the practical feasibility (availability,
possibilities to infiltrate or inject, storage life etc.) and acceptance by the regulatory authorities. If an electron donor also has its use as, e.g., a food additive, it will be much easier to get
approval for injection of this component for in-situ trea™ent.
In the Netherlands, there is a tendency to use complex mixtures as electron donor when applying full-scale remediation projects. Important motives are the costs, availability,
biodegradability, the fact that these mixtures contain nutrients and trace elements and their harmlessness for the environment. Last but not least, very good results have been obtained
using molasses or protamylasse in laboratory studies and in full-scale applications.
Before using an electron donor full-scale, it is advised to verify its feasibility as an electron donor for reductive dechlorination at a particular site by anaerobic degradation tests. The
amount of electron donor that is needed to induce the required redox conditions and to support reductive dechlorination has to be calculated based on concentrations of nitrate, sulphate,
chlorinated ethenes and the amount of electrons a given amount of electron donor can generate during biological degradation. An extensive suite of analyses should be performed to
evaluate if the concentrations of potential contaminants like heavy metals are below standards.
4.2. Techniques to deliver the substrates
In the past years, several techniques have been developed and used in the Netherlands to deliver electron donor to contaminated groundwater. In general, three different techniques can
be distinguished:
- extraction of groundwater, dosage of the electron donor and infiltration;
- injection of a gaseous electron donor using nitrogen as carrier gas;
- direct injection of a concentrated electron donor mixture.
4.2.1. Extraction and infiltration
Groundwater is extracted, supplied with the necessary electron donor and infiltrated again. Based on the results of several projects in the Netherlands, specific design criteria have been
formulated to obtain a proper design and to prevent clogging of the wells, with special emphasis on measures to remove suspended solids, to prevent or control degassing and to prevent
the introduction of oxygen.
Infiltration of the groundwater has been performed using horizontal or vertical infiltration wells. The most suitable configuration is depending on soil characteristics (hydraulic conductivity,
groundwater flow) and volume of groundwater that has to be treated. Using a three-dimensional model, different configurations can be evaluated and the optimal configuration can be
determined.
Extraction and infiltration is especially suitable to treat large volumes of contaminated groundwater in aquifers with sufficient conductivity. Possible disadvantages are clogging and the
technical complexity of the system. Also, the system is less suitable for use in low permeability soils like peat and clay.
4.2.2. Injection of gaseous electron donor
A second technique for the delivery of an electron donor to the contaminated groundwater is LINER, a technique developed within the SKB-program. LINER (Liquid Nitrogen Enhanced
Remediation) uses nitrogen gas as a carrier to deliver a gaseous electron donor and nutrients in the subsurface. The system is comparable to an air sparging system; the electron donor is
atomised in a nitrogen gas flow, which is injected under pressure into the groundwater. The electron donor dissolves into the groundwater and can be used by dechlorinating micro
organisms to degrade the chlorinate ethenes. By using nitrogen gas, the groundwater is kept anaerobic.
Advantages are a good distribution of the electron donor, prevention of clogging problems and an increased bioavailability of the contaminants. Disadvantages are the possibility of
volatilisation of the contaminants which might escape to the surface and the costs for nitrogen and volatile electron donor (ethyllactate). Complex electron donors, which have several
advantages above single component electron donors, cannot be used when implementing LINER. Also, the technique is not feasible in low-permeability soils like peat or clay.
4.2.3. Direct injection of an electron donor
An alternative for the use of water (extraction and infiltration) or nitrogen gas (LINER) as a carrier for the electron donor is the direct injection of a (solution of) the electron donor. This
technique, with which extensive experience has been gained in civil engineering and bioremediation, uses a injection conus that is pushed into the soil. When the required depth has been
reached, a high-pressure pump is used to inject an amount of the electron donor (figure 5).
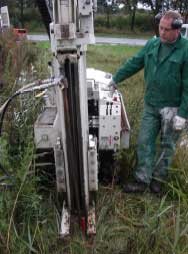
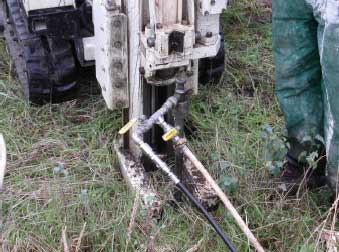
Figure 5. Direct injection of an electron donor. Left: the injection conus is pushed into the soil. Right: the electron donor is injected using a high pressure pump.
When it is expected that the injection has to be repeated, fixed injection points can be installed. Depths up to 40 meters can be reached, depending on the system used. Typical radius of
influence range from 3 to 5 meters in low-permeability soils. Advantages of this technique are the relatively low costs, simplicity (no tubing, wells etc.) and its applicability in
low-permeable soil types.
4.2.4. Status, experience and selection criteria
In the Netherlands, mainly extraction and infiltration and direct injection are used as techniques to deliver an electron donor to contaminated groundwater. The LINER concept has been
developed but reference projects are not yet available, the first full-scale applications are in preparation. Advantages and disadvantages of the techniques are presented in table 4.
Table 4. Characteristics of the techniques to deliver electron donor
technique |
permeability |
clogging risks |
distribution of electron donor |
types of electron donor |
costs |
experience |
|
high |
low |
|
|
|
|
|
extraction and infiltration |
+ |
- |
+/- |
+ |
all |
+/- |
+ |
direct injection |
+/- |
+ |
+ |
+/- |
all |
+ |
+ |
LINER |
+ |
- |
+ |
+ |
limited |
+/- |
+/- |
The choice between these techniques is mainly dependent on soil conductivity, the volume of groundwater that has to be treated, the depth of the contamination, costs and accessibility of
the site. In general, extraction and infiltration or LINER are most suitable for trea™ent of large volumes of contaminated groundwater in soil with high hydraulic conductivities and
groundwater flows. Direct injection techniques are preferred when low-permeable soil types have to be treated.
5. Legislation, introduction of chemicals and micro organisms
In the Netherlands, the use of chemicals and micro organisms to remediate soil and groundwater contamination is not explicitly regulated by law. In general, it is not allowed to inject or
infiltrate `anthropogenic' substances; components that are normally not present in soil or groundwater. However, in specific cases, for instance in soil remediation, specific components
can be used to degrade or remove the contamination. This includes chemical oxidation fluids, surfactants or solvents for surfactant/solvent flushing or electron donors to enhance
reductive dechlorination.
The decision to approve the use of electron donors is made using `common sense', in which the composition of the electron donor (possible contaminants, biodegradability) and its fate in
the subsurface play an important role. By demonstrating the biodegradability, non-toxicity and the positive overall effect of injecting an electron donor on soil quality, approval of the
regulatory authorities can be obtained. Normally, this does not pose a problem because the electron donor is used to stimulate biological processes; an important prerequisite for an
electron donor is that it is biodegradable and non-toxic. The approval of the regulatory authorities overrules the regulations stated in the national law that is applicable for this subject.
Another important aspect when using an extraction and infiltration system is the infiltration of contaminated groundwater. The sole purpose of the system aboveground is to supply an
electron donor to the groundwater and not to remove the contamination before infiltration. In fact, techniques that can be used to clean the groundwater (stripping, activated carbon) alter
the composition of the groundwater, causing a disruption of the degradation process or an increased risk of clogging of the infiltration wells. When it is shown that the extraction and
infiltration of groundwater does not cause an uncontrolled migration of the contamination (for instance by using modelling and monitoring in the field during pilot testing) and the infiltrated
contamination is degraded, infiltration of contaminated groundwater (extracted from the site itself) can be approved.
When implementing a bio-augmentation remediation, specific micro organisms are added besides an electron donor. To `install' the capacity for reductive dechlorination at a contaminated
site, micro-organisms obtained from nature itself are used as inoculum. The required micro organism (Dehalococcoides ethenogenes) has developed by evolution at contaminated sites
instead of genetic engineering in laboratory. When the groundwater is remediated, the necessary electron acceptors for this organism (the chlorinated ethenes) are not present anymore
and the micro organisms will die out. The community of micro organisms that is originally present in the aquifer will be restored. The use of this non-pathogenic micro organism has been
approved in several cases in the Netherlands.
For the Hoogeveen site, where a full-scale bio-augmentation strategy has been used, the effluent of the bioreactor was screened for the presence of known pathogens. The effluent
contained none of these pathogens, and infiltration of groundwater containing dechlorinating biomass was approved.
6. Reference projects in the Netherlands
ERD projects in the Netherlands that are in progress or have been finished are presented in table 5a, b, c and d. In table 5e and f, sites where a ERD approach is in preparation are
presented. The tables do not aim to be comprehensive, but the most important projects are included.
Projects in which Bioclear is involved are marked (*), sufficient data are available to describe the characteristics of the site, approach and, if finished, the results. Other important projects
in the Netherlands are also mentioned, but the available or published data are sometimes limited so the information may not be complete.
Table 5a. ERD projects in the Netherlands, finished or in progress
Klik her for at se tabellen.
Table 5b. ERD projects in the Netherlands, finished or in progress
Table 5c. ERD projects in the Netherlands, finished or in progress
Klik her for at se tabellen.
Table 5d. ERD projects in the Netherlands, finished or in progress
Klik her for at se tabellen.
Table 5e. ERD projects in the Netherlands, in preparation
Klik her for at se tabellen.
Table 5f. ERD projects in the Netherlands, in preparation
Klik her for at se tabellen.
7. Literature
Dijkhuis, J.E., J.B.M. van Bemmel, M.J.C. Henssen, R. van Lotringen. 2003. Protocol for the determination of the sustainability of the natural attenuation (S-NA) of chlorinated ethenes. SKB,
Gouda the Netherlands.
Wiedemeier, T.H., M.A. Swanson, D.E. Moutoux, E.K. Gordon, J.T. Wilson, B.H. Wilson, D.H. Kampbell, P.E. Haas, R.N. Miller, J.E. Hansen, F.H. Chapelle. 1998. Technical protocol for
evaluating natural attenuation of chlorinated solvents in groundwater. US Environmental Protection Agency, Cincinnatti, Ohio USA.
J.J. van der Waarde, M.H.A. van Eekert. 1999. Selection and validation of a practical protocol for anaerobic dechlorination. Phase 1: Inventory of methods and work visit Cornell University USA.
NOBIS-project 97-4-04, CUR/NOBIS Gouda
N.J.P. van Ras, J.J. van der Waarde, G. Schraa. 2001. Selection and validation of a practical protocol for anaerobic dechlorination. Phase 2: validation of selected parameters in batch experiments.
NOBIS-project 97-4-04, CUR/NOBIS Gouda
| Forside | | Indhold | | Forrige> | | Top |
Version 1.0 Februar 2005, © Miljøstyrelsen.
|