| Bottom | | Front page |
Environmental news No. 80, 2005
Spatial differentiation in LCA impact assessment
Contents
Introduction to the series
Preface to the guideline
Authors' preface
Summary
1 Introduction
2 Global Warming
3 Stratospheric ozone depletion
4 Acidification
5 Terrestrial eutrophication
6 Aquatic eutrophication
7 Photochemical ozone formation
8 Human toxicity
9 Ecotoxicity
10 Example of the application of spatial impact assessment
Introduction to the serie
Life cycle thinking and life cycle assessment are key elements in an integrated product policy. There is a need for thorough and scientific wellfounded methods for life cycle assessment. Parallel to this, there
is a need for simple, easy-understandable methods, which reflect life cycle thinking. Which method to use must depend on the goal and scope in each case inclusive target group, publication strategy etc.
It is common for all life cycle assessments, that they have to give a solid and reliable result. A result, that is a good foundation for the decisions subsequently to be made.
During the last 10 years a number of projects concerning life cycle assessment and life cycle thinking has received financial support.
The main results of projects on life cycle assessments will from 2000 and in the next couple of years be published as a mini-series under the Danish EPA's series Environmental News (Miljønyt).
As the projects are being finalised they will supplement the results of the EDIP-project from 1996. The tools, experience, advice, help and guidance altogether form a good platform for most applications of
life cycle assessments.
Life cycle assessment is a field so comprehensive, that it is not likely to be possible to write one book, that will cover all situations and applications of life cycle assessments. The Danish EPA hopes, that the
LCA-publications together will present the knowledge available to companies, institutions, authorities and others, who wish to use the life cycle approach.
The Danish Environmental Protection Agency, October 2000
Preface to the guideline
This guideline has been prepared within the Danish LCA methodology and consensus-creation project carried out during the period from autumn 1997 to 2003.
The guideline forms part of a series of guidelines dealing with key issues in LCA. These guidelines are planned to be published by the Danish Environmental Protection Agency during 2005.
A primary objective of the guidelines has been to provide advice and recommendations on key issues in LCA at a more detailed level than offered by general literature like the ISO standards, the EDIP
reports, the Nordic LCA project and SETAC publications. The guidelines must be regarded as an elaboration of and supplement to this general literature and not a substitution for this literature. The
guidelines, however, build on the line of LCA methodology known as the EDIP methodology.
It is important to note that the guidelines were developed by a consensus process involving in reality all major research institutions and consulting firms active in the field of LCA in Denmark. The advice given
in the guidelines, thus, be said to represent what is generally accepted as best practice today in the field of LCA in Denmark.
The guidelines are supported by a number of technical reports, which present the scientific discussions and documentation for recommendations offered by the guidelines. These reports are also planned to
be published during 2004. The guidelines and the technical reports are presented in the overview figure below.
The development of the guidelines and the technical reports was initiated and supervised by the Danish EPA Ad Hoc Committee on LCA Methodology Issues in the period 1997-2001.
The research institutions and consulting firms active in the development and consensus process are:
COWI, Consulting Engineers and Planners (Project Management) Institute for Product Development, Technical University of Denmark FORCE Technology
The Danish Technological Institute
Carl Bro
The Danish Building Research Institute DHI - Water and Environment
Danish Toxicology Institute
Rambøll
ECONET
Danish Environmental Research Institute
Guidelines and technical reports prepared within the Danish LCA-methodology and consensusproject
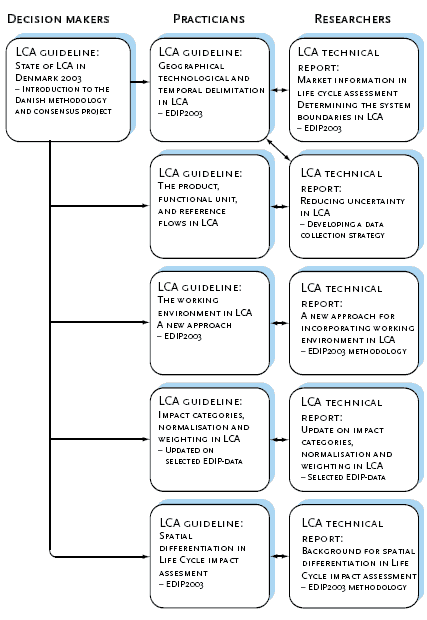
Authors' preface
The work behind this Guideline is documented in the report "Technical background for spatial differentiation in life cycle impact assessment" by José Potting and Michael Hauschild (eds., 2005). In addition
to the impact categories covered by the Guideline, the Technical Report also documents the development of a framework for life cycle impact assessment of noise. It was not possible within the constraints of
the current project to develop it to the level needed for this Guideline. However, a good platform has been created for the further work with this impact category. The Guideline was written by Michael
Hauschild and José Potting but the work behind the recommendations has been performed by the following research teams:
Chapter 4 Acidification
José Potting (Institute of Product Development (IPU), Technical University of Denmark, now the Center for Energy and Environmental Studies IVEM, University of Groningen, the Netherlands)
Wolfgang Schöpp (IIASA, International Institute for Applied Systems Analysis, Laxenburg, Austria)
Kornelis Blok (University of Utrecht, Department of Science, Technology and Society, the Netherlands)
Michael Hauschild (Institute of Product Development (IPU), Technical University of Denmark)
Chapter 5 Terrestrial eutrophication
José Potting
Wolfgang Schöpp Michael Hauschild
Chapter 6 Aquatic eutrophication
José Potting
Arthur Beusen (RIVM, National Institute of Public Health and the Environment, Bilthoven, the Netherlands)
Henriette Øllgaard (The Danish Technological Institute) Ole Christian Hansen (The Danish Technological Institute) Bronno de Haan (RIVM, National Institute of Public Health and the Environment,
Bilthoven, the Netherlands )
Michael Hauschild
Chapter 7 Photochemical ozone formation
Michael Hauschild Annemarie Bastrup-Birk (Danish National Environmental Research Institute)
Ole Hertel (Danish National Environmental Research Institute) Wolfgang Schöpp
José Potting
Chapter 8 Human toxicity
José Potting
Alfred Trukenmüller (Stuttgart University, Institute of Energy Economics and the Rational Use of Energy, Germany) Frans Møller Christensen (Danish Toxicology Center) Hans van Jaarsveld
(RIVM, National Institute of Public Health and the Environment, Bilthoven, the Netherlands)
Stig I. Olsen (Institute of Product Development (IPU), Technical University of Denmark)
Michael Hauschild
Chapter 9 Ecotoxicity
Jens Tørsløv (DHI Water and Environment ) Michael Hauschild
Dorte Rasmussen (DHI Water and Environment)
Chapter 9: Noise nuisance
Per H. Nielsen (Institute of Product Development (IPU), Technical University of Denmark)
Jens E. Laursen (FORCE Technology)
Summary
This Guideline presents the recommendations on characterisation from the Danish LCA Methodology Development and Consensus Creation Project 1997-2003. New characterisation factors and
accompanying normalisation references have been developed for each of the non-global impact categories:
- acidification
- terrestrial eutrophication
- photochemical ozone exposure of plants
- photochemical ozone exposure of human beings
- aquatic eutrophication
- human toxicity via air exposure
- ecotoxicity
For the global impact categories global warming and stratospheric ozone depletion, the characterisation factors are updated with the latest recommendations from IPCC and WMO/UNEP. The new
methodology is referred to as the EDIP2003 life cycle impact assessment methodology.
Compared to the EDIP97 methodology, the models underlying the EDIP2003 characterisation factors take a larger part of the causality chain into account for all the non-global impact categories. The
EDIP2003 factors thus include the modelling of the dispersion of the substance and the subsequent exposure increase. For a number of impact categories, the modelling also includes the background
exposure and vulnerability of the target systems to allow assessment of the exceedance of thresholds. Therefore, the environmental relevance of the calculated impacts is higher – they are expected to be in
better agreement with the actual environmental effects from the substances that are observed, and they are easier and more certain to interpret in terms of environmental damage.
The EDIP2003 factors have been developed in a site-dependent and a site-generic form. The site-generic form disregards spatial variation in dispersion and distribution of the substance and exposure of the
target systems like the EDIP97 methodology, but the results are in the same metrics as the site-dependent EDIP2003 results and can hence be added to these. In the site-dependent form of EDIP2003, the
characterisation factors are spatially resolved at the level of countries allowing the differences in impact from an emission when released in different countries to be a part of characterisation. For most of the
impact categories, the potential spatially determined variation is very large.
The relevance of spatial differentiation depends on the goal of the study. For many applications of LCA, the impact assessment should give the best prediction of the environmental impacts that are caused by
the emissions from the product system, and this is obtained through reduction of the spatially determined variation. There are, however, applications of LCA, where the information provided through inclusion
of spatial differentiation
>may not be relevant to the goal of the study. This can be the case for preparation of environmental product declarations and ecolabel criteria.
The Guideline recommends that the EDIP2003 characterisation methodology be used as an alternative to EDIP97 for performing site-generic characterisation (i.e. disregarding spatial information). For the
non-global impact categories, the environmental relevance of the site-generic EDIP2003 impact potentials is higher, and they provide the option to quantify and reduce the spatial variation not taken into
account.
Further, the Guideline recommends that the EDIP2003 site-dependent factors can be used to identify the main sources of spatially determined variation for the non-global impact categories and to reduce the
variation to the desired level according to the goal of the study.
EDIP97 can of course still be used if a new LCA should be compared with prior results based on EDIP97 methodology and factors.
1. Introduction
It was realised already during the EDIP programme (1991-96) that the exclusion of spatial information from the characterisation in life cycle assessment sometimes leads to obviously erroneous results.
Therefore, the EDIP97 methodology and the accompanying PC tool (beta version 1998) were prepared to take into account spatial differentiation in characterisation, but the concept was not made
operational by then. Spatial information was mainly used in the valuation as a basis for identifying obviously false results that could influence the decision to be based on the LCA.
As part of the Danish LCA Methodology and Consensus-creation Project, the uncertainties posed by refraining from spatial differentiation in characterisation were analysed, and methodology was developed
to allow inclusion of spatial knowledge about sources and the subsequent receiving environment in the life cycle impact assessment. The purpose of this Guideline is to give an operational presentation of the
recommendations following from this project. The new methodology is called EDIP2003. It is presented as an alternative to the EDIP97 methodology as originally presented in Wenzel et al., 1996 and
Hauschild, 1996 and later updated in Wenzel et al., 1997 and Hauschild and Wenzel, 1998a. The main innovation of the EDIP2003, compared to the EDIP97 methodology, lies in the consistent attempt to
include exposure in the characterisation modelling of the main non-global impact categories. This is accomplished through inclusion of a larger part of the causality chain and through introduction of spatial
differentiation regarding the emission and the receiving environment. EDIP2003 can be used both with and without spatial differentiation. In both cases, the inclusion of a larger part of the causality chain gives
the EDIP2003 impact potentials a higher environmental relevance and makes them easier to interpret in terms of damage to the protection areas of the LCA.
It is the hope of the project group that the EDIP2003 methodology will find a natural position as an alternative to the EDIP97 method for life cycle impact assessment and in time, when the users get
acquainted with the advantages that it offers, replace the EDIP97. Apart from increasing the environmental relevance of the results, it is our judgement that the new method considerably improves our
understanding of the spatially determined variation, which underlies the assessment of environmental impacts in LCA, without requiring much additional time and resources.
Guidance to the reader
In this chapter, the EDIP2003 methodology for life cycle impact assessment is introduced and the main differences to the EDIP97 methodology are identified and discussed. First, in Section 1.1, the
Guideline's recommendations on the future use of EDIP2003 and EDIP97 are presented in short form. The rest of the chapter gives the background for the EDIP2003 methodology and the
recommendations. Section 1.2 introduces the general principles of life cycle impact assessment (LCIA) given in the
ISO standard 14042. This is followed by a status on the inclusion of spatial differentiation in current characterisation and normalisation of LCA in Section 1.3. Here, a brief discussion is given of the
possibility to include spatial information in LCIA. In Section 1.4 the EDIP2003 and the EDIP97 methodologies are compared and the main differences identified, and in Section 1.5 a three-step procedure
for the practical application of the new factors is presented. The application of the EDIP2003 methodology is illustrated throughout the Guideline by an example that is introduced at the end of the
introductory chapter in Section 1.6 where an inventory is presented. For each of the impact categories in the following chapters, the use of the EDIP2003 factors is demonstrated on this inventory, and in
Chapter 10 at the end of the Guideline all the results are gathered and the example concluded.
The rest of the Guideline is devoted to the description of how the EDIP2003 methodology handles the environmental impact categories currently made operational within the EDIP methodology. Each impact
category has its own chapter presenting a procedure for the application of the methodology together with the relevant factors for characterisation and normalisation and guidance for interpretation of the
results.
It is the purpose of the Guideline to give an operational presentation of the EDIP2003 methodology for the potential user. The reader looking for a more detailed discussion of the reasoning behind the new
methodology is referred to the documentation given in the background report (Potting and Hauschild, 2005).
1.1 Guidance on the use of EDIP97 and EDIP2003
EDIP2003 can be used both in a site-generic and a site-dependent form. The site-generic form does not take spatial variation into account. EDIP97 is site-generic by nature, and the site-generic form of
EDIP2003 can replace EDIP97 for all applications.
The main reason to continue the use of EDIP97 would be to ensure compatibility of new results with earlier results obtained using EDIP97. Since some of the impact categories are modelled differently in
EDIP97 and EDIP2003, the impact profiles are not directly comparable. On the other hand, the impact profiles of earlier studies can be replaced by EDIP2003
impact profiles by simply applying the new characterisation and normalisation factors to the old inventory. The practical application of the site-generic form of EDIP2003 factors proceeds in the same way as
the application of the EDIP97 factors.
The Danish LCA methodology and consensus-creation project gives the following recommendation on the characterisation part of life cycle impact assessment:
The EDIP2003 characterisation methodology as documented in this Guideline can be used as analternative to EDIP97 for performing site-generic characterisation. For the non-global impact categories EDIP2003 provides the option to quantify and reduce the spatial variation resultingfrom differences in the region of emission.
For the global impact categories global warming and stratospheric ozone formation, the EDIP2003 also involves an update of the characterisation factors from EDIP97.
For new studies, the site-generic form of EDIP2003 should be preferred due to the higher environmental relevance of its impact potentials, and because it offers the possibility of quantifying spatial variation.
Site-dependent characterisation
For the non-global impact categories, regional differences in source and receptor characteristics may strongly influence the impact from an emission. The same emitted amount of a substance may thus cause
quite different impacts depending on where the emission is released. This spatially determined variation can be quantified using the site-generic form of the EDIP2003 methodology. The site-dependent form
of EDIP2003 allows reducing this variation:
Where relevant to the goal of the LCA, the EDIP2003 methodology can be used in its site-dependent form to identify the main sources of spatially determined variation for the non-global
impact categories and to reduce the variation to the desired level.
For the impact categories acidification, photochemical ozone formation and terrestrial eutrophication, the site-dependent EDIP2003 factors can be used directly for characterisation. Until the
methodology has been implemented in a PC tool, the most operational way of performing spatial characterisation will be
- first to apply the EDIP2003 site-generic characterisation factors and then
- to reduce the spatial variation step by step to an acceptable level defined by
- the goal of the study through the use of the site-dependent characterisation factors.
For the impact categories human toxicity, ecotoxicity and aquatic eutrophication the developed spatial characterisation can be applied as part of a sensitivity analysis to examine the spatial
variation in exposure that is disregarded when site-generic characterisation is used.
The practical application of spatial characterisation is outlined in Section 1.4 and described for each of the non-global impact categories in the respective chapters throughout the rest of this Guideline. The
choice of whether or not to apply spatial differentiation in the LCIA must be made according to the goal of the study. For many applications of LCA, it is in line with the goal of
the study that the impact assessment should give the best prediction of the environmental impacts that are caused by the emissions from the product system through reduction of the spatially determined
variation.
There are, however, applications of LCA, where the information provided through inclusion of spatial differentiation may not be relevant to the goal of the study. This can be the case for preparation of
environmental product declarations and ecolabel criteria. Here, the goal may be to guide consumers to buy products from companies that seriously work on emission reductions over the product's life cycle.
Taking into consideration the company's location and the sensitivity of the receiving environment will not contribute to the delivery of that message and may even be misused to obscure it. Therefore, spatial
differentiation in life cycle impact assessment does not conform with the goal of such a study. Similar considerations can be made for the application of LCA for development of ecolabel criteria where
distinction according to the location of the company may be seen as a hidden tradebarrier. For such applications, EDIP2003 in its site-generic form or alternatively EDIP97 should be used.
When applying the EDIP2003 methodology in its site-dependent form, it must be remembered that it has been developed for use in an LCA context where the perspective is reduction of emissions and their
environmental impacts. Here, it offers an improved modelling of the environmental impacts from a product system. The emission reduction perspective is important. The site-dependent EDIP2003
methodology is thus not intended to support impact reduction through transfer of polluting activities to regions where the receiving environment is more robust. Rather it is developed to help prioritising those
processes where emission reduction is most urgent and effective.
Normalisation and weighting
Normalisation in EDIP2003 proceeds in the same way as in EDIP97 just applying the EDIP2003 normalisation references which are given for the different impact categories in the respective chapters of this
Guideline. Until the default EDIP weighting factors, which are based on political reduction targets, have been updated to an EDIP2003 version, the weighting factors based on EDIP97 factors are used also
in EDIP2003.
For the EDIP97 impact categories nutrient enrichment and photochemical ozone formation, the EDIP2003 methodology operates with sub categories. The impact potentials of these sub categories must be
aggregated prior to weighting to allow use of the default EDIP97 weighting factors (based on distance to political targets). The sub category impact potentials are normalised against their respective
normalisation references and the average of the normalised impacts is taken as the impact potential of the main category.
To accommodate future needs for life cycle impact assessment, both EDIP97 and EDIP2003 are planned to be implemented in the officially endorsed PC tool supporting the use of LCA in Denmark.
1.2 Life cycle impact assessment
According to ISO 14042, the assessment phase of an LCA proceeds through several steps from the inventory to the interpretation:
- Classification or assignment of inventory results where the impact categories are defined and the exchanges in the inventory are assigned to impact categories according to their ability to contribute to
different problem areas ("what is the problem for this environmental exchange?").
- Characterisation or calculation of category indicator results where the contributions to impact(s) from each exchange are quantified and then aggregated within each impact category. In this way, the
classified inventory data is converted into a profile of environmental impact potentials or category indicator results, consumption of resources and possibly working environment impact potentials ("how big
is the problem?").
- Normalisation or calculation of the magnitude of the category indicator results relative to reference values where the different indicator results and consumption of resources are expressed on a
common scale through relating them to a common reference, in order to facilitate comparisons across impact categories ("is it much?").
- Weighting where weights are assigned to the different impact categories and resources reflecting the relative importance they are assigned in this study in accordance with the goal of the study ("how
important is it?")
- Interpretation where sensitivity analysis and uncertainty analysis assist interpreting the results of the life cycle assessment according to the goal and scope of the study to reach conclusions and
recommendations.
While classification, characterisation and interpretation are mandatory steps according to ISO 14042, normalisation and weighting are optional.
ISO 14042 also requires that the model for each indicator should be scientifically and technically valid, using a distinct identifiable environmental mechanism and/or reproducible empirical observation. The
model should preferably be internationally accepted i.e. based on an international agreement or approved by a competent international body and value choices and assumptions made during the selection of
impact categories, indicators, and models should be minimised. Furthermore, the indicators should be environmentally relevant
The EDIP2003 methodology meets all of these ISO 14042 requirements and recommendations.
1.3 Spatial differentiation in characterisation and normalisation
This section reviews the background of spatial differentiation in life cycle impact assessment and defines three levels of spatial differentiation.
The impacts caused by an emission depend on and can be predicted from knowledge about
1)the quantity that is emitted
2)the properties of the emitted substance
3)the properties of the emitting source and the receiving environment
In life cycle assessment, the information under 1 is found in the inventory for the product system. The inventory lists the emissions per functional unit and serves as the starting point for the impact assessment
phase.
The properties referred to under 2) could be physico-chemical data like boiling point and molecular weight or biological information regarding the toxicity to specific organisms or the inherent biodegradability
of the substance. This kind of information depends only on the substance and can be determined independently. This kind of data is often found in large substance-databases.
The properties under 3) are specified by the conditions under which the emission takes place and the state of the receiving environment to which the emission contributes (e.g. the simultaneous presence of
other substances or other stressors in the environment which may interact with the emitted substance to create additive or perhaps synergistic or antagonistic effects). The location of the receiving environment
follows from the spatial characteristics of the source, in particular its geographical location.
Some of the early life cycle assessments only included the information under item 1), i.e. all the emissions were simply added and the total emitted quantity was taken as an indicator of the environmental
impact. This came in fact down to nothing more than an extended resource and energy analysis and it was quickly realised that this approach was far too simplistic, and that the outcome made little sense in
an environmental interpretation. Therefore, a life cycle impact assessment developed which is based on information included under both item 1) and 2) by also taking into account the inherent properties of
substances and their maximum capacity to contribute to different environmental impacts with varying strengths. In current practice, the features covered under item 3 are poorly represented, if at all, and
variations in the characteristics of source and the receiving environment have hitherto been neglected for a number of reasons:
- the processes comprised by the product system may be located in many different parts of the world and the conditions of their local environment will often not be known
- the emissions are also spread in time since some of them may have taken place several years ago while emissions from the disposal may continue for decades or centuries into the future
- LCA deals with a functional unit, not the full output from processes.
Due to these reasons, LCA seemed unable to operate with actual concentrations and subsequent risks. In addition, many LCA practitioners have felt that since prediction of actual risks is done using risk
assessment tools, there is no need for inclusion of spatial differentiation in LCA. LCA has historically been seen as a tool for pollution prevention, not avoidance of environmental risks at specific sites.
Some of these points used to be regarded as practical limitations but, as we hope to demonstrate with this Guideline and the technical background report behind it, they do not have to be so any more.
Moreover, there is no discussion in LCA circles that, as long as an impact category is not global, the spatial variation may be large between process emissions of the same substance. Depending on the goal
of the study, it is thus very relevant to include it in the modelling in order to give a correct impression of the impacts caused by the emissions (Udo de Haes et al., 1999). Disregarding spatial variation will
increase the possibility of making wrong conclusions and sub optimisations based on the outcome of the life cycle impact assessment. On the other hand, as mentioned earlier, there are applications where the
goal of the study and the intended use of the results make the inclusion of spatial differentiation unwanted.
To overcome the methodological limitations quoted above, three levels of spatial differentiation in characterisation modelling have been defined:
- site generic modelling (sted-uafhængig): All sources are considered to contribute to the same generic receiving environment. Like in EDIP97, no spatial differentiation in sources and subsequent receiving
environments is performed. However, the modelling may have been expanded to cover a larger part of the causality chain and thereby to ensure compatibility with the next level of spatial differentiation (the
site-generic factors are then calculated as an emission-weighted mean of the site-dependent factors)
- site-dependent modelling (sted-afhængig): Some spatial differentiation is performed by distinguishing between classes of sources and determining their subsequent receiving environment. Source categories
are defined at the level of countries or regions within countries (scale150-500 km). The receiving environment is typically defined at high spatial resolution (scale at maximum 150 km, but usually lower). The
site-dependent characterisation factors thus include the variation within and between the
receiving environments related to each source category in exposure and a priori tolerance to the exposure.
- site-specific modelling (sted-specifik): A very detailed spatial differentiation is performed by considering sources at specific locations. Site-specific modelling allows large accuracy in modelling of the
impact very local to the source. This typically involves local knowledge about conditions of specific ecosystems exposed to the emission. However, since the full impact from a source often covers areas
extending several hundred to thousand kilometres, a detailed assessment of the impact locally around the source may add little accuracy to the quantification of the full impact.
LCA is normally not focused on the local impacts from the product system and furthermore, in LCA it will rarely be possible to operate with site-specific modelling for more than a few processes in the
product system. Therefore, the site-specific level of spatial differentiation is not envisaged to become an integral part of characterisation modelling. It may still be used to provide additional information for the
interpretation step of LCA.
The spatial information available for individual processes in LCA will normally support site-dependent impact modelling. For most processes it will be known at least in which country it is located. This
information is required as part of the system delimitation in order to develop transportation scenarios for the product system. The site-dependent level is the level of spatial differentiation that is suggested for
characterisation modelling in EDIP2003. Incidentally, at least for most airborne emissions, it is also the level of spatial differentiation that is relevant since it represents their typical scale of dispersion. This
means that the site-dependent characterisation factors recommended in Section 1.1 are robust in the sense that the introduction of new uncertainties with the additional fate modelling generally is more than
compensated for by the reduction in the impact potentials' spatially determined variation.
In some life cycle assessments, there will be materials or processes, for which spatial information is not available at all. Maybe the data has been aggregated over several suppliers to hide sensitive information
or to provide average data. For this situation, the EDIP2003 site-generic characterisation factors can be used to provide impact potentials compatible with the site-dependent impact potentials from other
parts of the life cycle. In addition, the use of the EDIP2003 site-generic characterisation factors offers the possibility to quantify the range in the possible impact resulting from ignoring spatial differences in
sources and receiving environments.
1.4 EDIP97 and EDIP2003 – similarities, differences and interpretation
After a brief summary of similarities between EDIP97 and EDIP2003, the main differences between the two methodologies are
presented and guidance is given on the interpretation of the site-generic and the site-dependent EDIP2003 impact potentials.
Similarities between EDIP2003 and EDIP97
The impact assessment methodologies of EDIP97 and EDIP2003 show many similarities. They are both environmental theme-methods in accordance with the requirements of ISO 14042 and proceed
through the same steps – characterisation, normalisation including possible aggregation of sub categories and weighting by the same default weighting factors. They also cover the same impact categories,
though some new sub categories are addressed in EDIP2003. For the impact categories aquatic eutrophication, human toxicity and ecotoxicity, the site-generic characterisation factors of EDIP2003 are
identical to the EDIP97 factors.
EDIP2003 covers a larger part of the causality chain
Apart from the spatially resolved modelling, the main difference between EDIP97 and EDIP2003 lies in the choice of category indicator. In EDIP97 the characterisation modelling is focused rather early in
the environmental mechanisms for some of the impact categories, and the characterisation factors are based exclusively on knowledge of properties of the emitted substance, disregarding properties of the
receiving environment. Where the substance's fate is modelled, a uniform environment is assumed ("unit world"). This reflected state-of-the-art when EDIP97 was developed. In contrast, some of the
EDIP2003 category indicators are chosen later in the causality chain and the characterisation factors also include the (spatially resolved) modelling of the dispersion and distribution of the
substance, the exposure of the target systems and in some cases also the background situation of the target systems to allow assessment of the exceedance of thresholds. This change
reflects the development of environmental modelling since the EDIP97 factors were established in 1994 or 1995 (Wenzel et al., 1996, Hauschild, 1996). The difference is illustrated in Figure 1.1
Modelling the impacts further along the causality chain in EDIP2003 increases the environmental relevance of the calculated impacts – they are often in better agreement with the actual environmental
effects that are observed from the substances, and they are easier and more certain to interpret in terms of environmental damage. Even though EDIP2003 includes a larger part of the causality chain, the
calculated impacts are still predictions and must thus be considered as potentials and not as actual effects. The accuracy of these predictions may be affected by other conditions inherent in the life cycle
assessment approach (e.g. focus on the functional unit and aggregation across time).
EDIP2003 supports quantification of spatially determined variation The EDIP2003 site-generic characterisation factors are calculated as the mean of the site-dependent characterisation factors. While
still supporting site-generic characterisation, EDIP2003 also allows quantification and reduction of the spatially determined variation in impact through the inclusion of spatial variation in emission sources, and subsequent dispersion and receiving environment exposure. Classes of emission sources are typically defined at the national level.
Figure 1.1 Causality chain. For each link the descriptors identify aspects to consider in an environmental model. The EDIP2003 methodology covers the major part of the chain and includes the spatial variations in the
relevant parameters, while the EDIP97 is based on the first links and hence refrains from spatial differentiation.
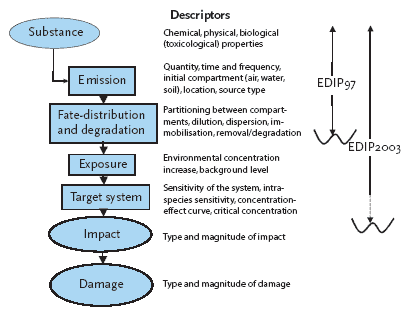
EDIP2003 provides improved modelling of photochemical ozone formation
Some important additional improvements are obtained with the EDIP2003 methodology. For photochemical ozone
formation, the contribution from NOx can now be represented in the site-generic as well as the site-dependent impact potential. The contribution of NOx has hitherto been omitted from the
calculation of the photochemical ozone formation potential but it turns out to be at least the same size as the contribution from the VOCs - hitherto counted as the only source for ozone formation.
EDIP2003 provides improved modelling of acidification
For acidification, the EDIP2003 factors account for the assimilation of nitrogen by ecosystems which in the real world reduces the acidifying properties of nitrogen compounds compared to e.g. SO2. The
EDIP2003 factors thus give a more realistic proportion between the different acidifying compounds than the EDIP97 factors that only reflect the potential for release of protons.
For the EDIP2003 characterisation factors for acidification and photochemical ozone formation, damage to natural ecosystems and human health are chosen as the most sensitive end point. They are also the
end point that current regulation is focused on. Therefore damage to man-made materials is not explicitly addressed by the factors for photochemical ozone formation and acidification (although it will typically be represented indirectly by the other indicators). One might thus
say that some of the damage covered by EDIP97 is no longer covered in EDIP2003 because the impact indicator is chosen further in the causality chain. As an example for acidification, the impact
calculated with the EDIP97 factors represents the number of protons formed per mole of substance emitted. Being defined so early in the cause-effect chain, the EDIP97 impacts in principle represent any
damage potentially caused by the protons (i.e. also damage to man-made materials). On the other hand, the relation between proton-release as such and damage caused is often highly uncertain. If, however,
there is a wish to explicitly include acidification damage to man-made materials, these may be calculated separately using e.g. the EDIP97 factors. It should be noted, however, that the default weighting
factors applied in EDIP97 as well as EDIP2003 represent political reduction targets that for acidification are based on targeted reductions in damage to natural ecosystems. The same holds true for most of
the other impact categories – where the political reduction targets expressed in the weighting factors aim at protection of ecosystems or, where relevant, human health.
The difference in choice of category indicators means that for some of the impact categories, the variation estimates provided with the site-generic EDIP2003 factors are not directly applicable
to the EDIP97 factors.
Different units for EDIP2003 and EDIP97
The difference in choice of end points also means that the impacts calculated using EDIP97 factors and EDIP2003 factors have different units. For EDIP97 most of the impacts are expressed as
quantities of a reference substance which would cause the same size of impact. For EDIP2003, the units express what impact is effectively caused, sometimes up to inclusion of the damage to the target
system. In the example of acidification, the EDIP97 unit is "g SO2-equivalents" while the EDIP2003 is "m2 unprotected ecosystem" expressing the area of ecosystem that is moved from an exposure below
to an exposure above the critical load and thus potentially damaged.
The EDIP2003 impacts could very well be scaled into emissions of reference substances as was done in EDIP97 but we have chosen to keep the original EDIP2003 units for two reasons:
- they give the user an impression of what is actually expressed by the
- EDIP2003 impact potentials
- they emphasize the difference in what is covered by the EDIP97 and EDIP2003 impact potentials and that the two are not immediately comparable.
EDIP2003 optimises the trade-off between environmental relevance and model uncertainty
As characterisation modelling is extended to include more of the causality chain, the uncertainty in interpretation is typically reduced as the environmental relevance of the predicted impact is increased. On
the other hand, the introduction of additional environmental models into the calculation of characterisation factors also introduces some additional sources of uncertainty. Spatial differentiation may further
reduce the impact uncertainty. At the same time, the information about process locations from the inventory analysis that supports spatial characterisation will sometimes be based on assumptions and may
then also be a source of additional uncertainty. Figure 1.2 illustrates this trade-off.
Figure 1.2 As characterisation modelling proceeds along the causality chain to include larger parts of the environmental mechanism, environmental relevance of the calculated impacts is increased and
uncertainty of interpretation is reduced (e.g. through reduction of spatially determined variation). At the same time additional uncertainty is introduced through the applied models and the assumptions made
e.g. in the geographical scoping of the product system (the figure was developed together with professor O. Jolliet, EPFL).
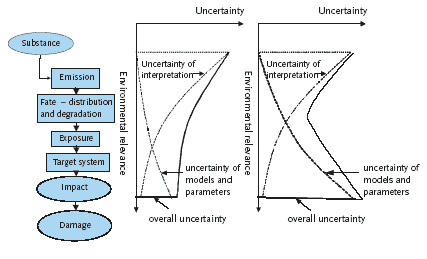
The recommendations given in this Guideline on spatial differentiation in life cycle impact assessment attempt to optimise the trade-off illustrated in Figure 1.2. This is done within the
constraints of the state-ofthe-art in environmental modelling that varies between the different impact categories.
Where detailed integrated assessment models are available, it is possible to develop spatial characterisation factors that incorporate the major part of the spatial variation in emission, exposure and
vulnerability of the exposed environment. Here, the resolving power is increased by orders of magnitude compared to the site-generic characterisation, and the additional uncertainty
introduced by sophisticated modelling is relatively small in comparison. This is the case for the impact categories acidification, terrestrial eutrophication and photochemical ozone formation.
This situation is illustrated by the first of the graphs. For the other non-global impact categories, the state of current environmental modelling is less advanced and as a consequence it has only been possible to include parts of the spatial variation into the new characterisation factors. As a result, the increase in resolving power compared to the existing characterisation is more modest compared to the
additional uncertainty which is introduced. This is the case for the impact categories human toxicity, ecotoxicity and aquatic eutrophication.
EDIP2003 improves interpretation through spatially differentiated impact potentials
The main advantage of the site-generic EDIP2003 characterisation methodology lies in the interpretation phase. The site-generic EDIP2003 factors allow the user to quantify a large part of the spatially
determined variation, which is inherent but unknown in the EDIP97 characterisation factors, and this is valuable input to the sensitivity analysis. Use of the EDIP2003 site-generic factors does not require any
information apart from what is required to use EDIP97. Further sensitivity analysis with the site-dependent factors requires specification of the geographic location of the processes in the product system.
For some processes, this specification will be encumbered by an uncertainty that must also be considered in the sensitivity analysis.
As discussed earlier in this section, the impact potentials calculated with the EDIP2003 factors – site-generic as well as site-dependent - are expected to be in better accordance with the actual impacts on
several accounts:
- The EDIP2003 factors, site-dependent as well as site-generic, are based on the modelling of a larger part of the causality chain between emission and environmental impact than the EDIP97.
- For the links in the causality chain shown in Figure 1.1 that describe environmental fate, resulting exposure, and target system, many descriptors show considerable spatial variation which is nearly
completely disregarded in the modelling of the EDIP97 factors. For most of the impact categories, the new characterisation factors reflect the spatial variation in fate and exposure to varying degrees. For a
number of the impact categories, also spatial variation in the target system sensitivity is represented.
This increased environmental relevance of the EDIP2003 impact potentials should be taken into account in the interpretation, particularly in the case, where they are compared to impact potentials of a lower
environmental relevance (calculated using characterisation factors of the old type, EDIP97 or others). It should also influence the development of weighting factors based on the environmental relevance of
the impact categories (e.g. derived through a panel procedure).
The default EDIP weighting factors, which are based on political reduction targets, should also be updated to an EDIP2003 version using the new characterisation factors on the politically targeted emission
levels. This is not a part of the Danish Method Development and Consensus Creation Project and until it has been done, the updated weighting factors based on the EDIP97 factors are suggested used as
proxies (Stranddorf et al., 2005).
1.5 How is spatial characterisation performed?
Traditionally, the inventory information is aggregated in the sense that all emissions of one substance occurring through the life cycle of the product are summed. In this way the emission of e.g. SO2 is
reported as one total emission of for the whole life cycle and all spatial information about the individual emissions is lost. If site-dependent characterisation is performed directly (i.e. not as part of the
sensitivity analysis following the site-generic characterisation), the life cycle inventory must be passed on to the impact assessment phase in a non-aggregated form in order to make it possible to identify the
geographical location where the different processes take place. This will not be a problem when the EDIP2003 methodology is integrated in an LCA software but may otherwise create some additional work
compared to the site-generic EDIP2003 or EDIP97.
Until the site-dependent form of EDIP2003 is implemented in a PC tool, a practical application of spatial characterisation is described for each of the non-global impact categories in the respective chapters
throughout the rest of this Guideline but in general terms the recommended way of applying EDIP2003 manually is:
For each non-global impact category:
- Calculate the site-generic impact potential and the potential spatially determined variation for the product system using the site-generic EDIP2003 characterisation factors with
accompanying spatial variation estimates
- Identify the processes that contribute most to the site-generic impact and
- subtract their contribution from the site-generic impact potential
- calculate their site-dependent impact potential
- Add the site-dependent contributionsfrom these processers to the adjusted site-generic impact potential
Repeat step 2 until the spatially determined variation is reduced to a suitable level,
i.e. a level where the spatially determined variation can no longer change the conclusion of the study.
The only extra information that is required to use the site-dependent factors of EDIP2003 is the country in which the process is located. This information is often known as part of the scoping. For processes,
where this information is not at hand, the site-generic EDIP2003 factors can be applied. This is also the option for processes taking place outside Europe.
Aggregation of sub categories
For two of the EDIP97 impact categories (nutrient enrichment and photochemical ozone formation), EDIP2003 operates with sub categories which must be aggregated prior to weighting to allow weighting
with the default EDIP97 weighting factors (based on distance to political targets). The aggregation procedure for sub categories that was developed under EDIP97 to prepare the sub categories of
ecotoxicity and human toxicity for weighting is also used here; First the sub category impact potentials are normalised against their respective normalisation references. Then their average is calculated to
represent the impact potential of the main category.
In principle, the default EDIP weighting factors, which are based on political reduction targets, should also be updated using the new characterisation factors for application to impact potentials calculated
using these new factors. This has not been done yet and has not been a part of the Danish Method Development and Consensus Creation Project. It would be relevant with an update for the impact
categories acidification, terrestrial eutrophication and photochemical ozone formation. For the other impact categories, the site-generic version of EDIP2003 is identical to EDIP97 and the weighting factors
are therefore the same. The difference is not expected to be dramatic and until an update is available, it is suggested to use the updated EDIP97 weighting factors as proxies for all impact categories.
1.6 Example on the use of EDIP2003
The following example serves to demonstrate the procedure for application of the EDIP2003 site-generic and site-dependent characterisation factors for all the impact categories. The example has been
constructed to illustrate the use of spatial characterisation. The example is introduced here, and the characterisation is performed and illustrated throughout the chapters on the individual impact categories. A
comparison and discussion of the results is given in Chapter 10.
Functional unit and inventory
In the construction of an office chair, the product developer has to make a choice between the use of zinc and the use of a plastic (polyethylene) as material for a supporting block (a structural element) in the
seat of the chair. The supporting block is flow injection moulded (20 g plastic) or die cast (50 g zinc). A life cycle assessment is performed to compare the environmental impacts from each of the two
alternatives. The functional unit (f.u.) of the study is one component made from either plastic or zinc.
An excerpt from the inventory analysis provides the following results for the life cycle impact assessment:
Table 1.1. Excerpts from inventory for one supporting block made from plastic or zinc
| Plastic part |
Zinc part |
Substance |
Emission, g/f.u. |
Emission, g/f.u. |
Emission to air |
|
|
Hydrogen chloride |
1.16•10-3 |
1.72•10-3 |
Carbon moNOxide |
0.2526 |
0.76 |
Ammonia |
3.61•10-3 |
7.10•10-5 |
Methane |
3.926 |
2.18 |
VOC, power plant |
3.95•10-4 |
3.70•10-4 |
VOC, diesel engine |
2.35•10-2 |
2.70•10-3 |
VOC, unspecified |
0.89 |
0.54 |
Sulphur dioxide |
5.13 |
13.26 |
Nitrogen oxides |
3.82 |
7.215 |
Lead |
8.03•10-5 |
2.601•10-4 |
Cadmium |
8.66•10-6 |
7.45•10-5 |
Zinc |
3.78•10-4 |
4.58•10-3 |
Emissions to water |
|
|
Nitrate-N |
5.49•10-5 |
4.86•10-5 |
Ammonia-N |
4.45•10-4 |
3.04•10-3 |
Ortho phosphate |
1.40•10-5 |
0 |
Zinc |
3.17•10-5 |
2.21•10-3 |
The calculation of site-generic and site-dependent impacts for the inventory in Table 1.1 can be found for each of the impact categories in the respective chapters.
2. Global Warming
Background information for this chapter can be found in:
- Chapter 1 of "Environmental assessment of products. Volume 2: Scientific background" by Hauschild and Wenzel (1998a).
- Chapter 4 of "Guideline in normalisation and weighting – choice of impact categories and selection of normalisation references" by Stranddorf et al., 2005.
2.1 Introduction
The environmental mechanisms underlying global warming, and the climate change associated with it, are global of nature. This means that the impacts caused by an emission are modelled in the same way
regardless where on the surface of the earth, the emission takes place. There is therefore no relevance of including spatial variation in the source and receptor characteristics for this impact category. The
characterisation factors are site-generic by nature and will be valid for EDIP97 (as an update) as well as for EDIP2003.
The atmosphere of the earth absorbs part of the infrared radiation emitted from earth towards space, and is thereby heated. This natural greenhouse effect can be said with certainty to have been increased
over the past few centuries by human activities leading to accumulation of such gases as CO2, N2O, CH4 and halocarbons in the atmosphere. The most import human contribution to the greenhouse effect is
attributed to the combustion of fossil fuels such as coal, oil and natural gas.
The predicted consequences of the man-made greenhouse effect include higher global average temperatures, and changes in the global and regional climates. The world-wide network of meteorological
researchers and atmospheric chemists, the IPCC (Intergovernmental Panel on Climate Change), is following the latest development in our knowledge of the greenhouse effect and issuing regular status
reports. These status reports comprise the basis of the EDIP97 and EDIP2003 methodologies' assessment tool for the global warming.
The endpoint is chosen at the level of increase in the atmosphere's radiative forcing.
2.2 Classification
For a substance to be regarded as contributing to global warming, it must be a gas at normal temperatures in the atmosphere and:
- be able to absorb heat radiation and be stable in the atmosphere for a period of years to centuries,
or
- be of fossil origin and converted to CO2 on breakdown in the atmosphere.
The criteria applied in the EDIP methodologies to determine if a substance contributes to global warming follow the IPCC's recommendation of excluding indirect contributions to the greenhouse effect, i.e.,
contributions attributable to a gas affecting the atmospheric lives of other greenhouse gases already present. At one point the EDIP method goes further than the IPCC's recommendation by including that
contribution from organic compounds and carbon moNOxide of petrochemical origin, which follows from their degradation sooner or later to CO2 in the atmosphere. For emissions of CO2 it is important to
check whether they constitute a net addition of CO2 to the atmosphere, or whether they simply represent a manipulation of part of the natural carbon cycle. If the source of carbon is fossil (coal, oil, natural
gas), conversion to CO2 will mean a net addition. If there is a question of combustion or breakdown of material which does not derive from fossil carbon sources, but e.g. from biomass, there will normally
be no net addition because the material in question was generated recently by fixation of CO2 from the atmosphere, and will sooner or later be broken down to CO2 again (see Hauschild and Wenzel,
1998b, for a more detailed discussion).
The list of substances estimated to contribute to global warming is manageable and can be regarded as exhaustive. In other words, it is not necessary in practice to check whether a substance fulfils the
criteria above in order to decide whether it is to be regarded as contributing to the greenhouse effect. It is sufficient to consult the list of greenhouse equivalency factors in Table 2.1.
2.3 EDIP2003 and updated EDIP97 characterisation factors
The endpoint for this impact category is chosen at the level of radiative forcing, and the EDIP2003 and revised EDIP97 characterisation factors
are therefore taken from the latest version of the IPCC consensus report. These are complemented by factors for hydrocarbons and partly oxidised or halogenated hydrocarbons of fossil origin, which are
derived from the stoichiometrically determined formation of CO2 by oxidation of the substance. The recommendation for EDIP97 is still to use a time horizon of 100 years and to check the sensitivity to this
choice by applying the other time horizons.
Table 2.1. Factors for characterisation of global warming (in g CO2-equivalents/g). Taken from Albritton and Meira Filho, 2001 except as noted.
Click here to see the Table
2.4 Normalisation
The updated EDIP97 person equivalent for global warming is 8.7 t CO2-eq/pers/yr as found in Stranddorf et al., 2004.
3. Stratospheric ozone depletion
Background information for this chapter can be found in:
- Chapter 2 of "Environmental assessment of products. Volume 2: Scientific background" by Hauschild and Wenzel (1998a).
- Chapter 5 of "Guideline in normalisation and weighting – choice of impact categories and selection of normalisation references" by Stranddorf et al., 2005.
3.1 Introduction
The environmental mechanisms underlying stratospheric ozone depletion are global of nature. This means that the impacts caused by an emission are modelled in the same way regardless where on the
surface of the earth, the emission takes place. There is therefore no relevance of including spatial variation in the source and receptor characteristics for this impact category. The characterisation factors are
site-generic by nature and will be valid for EDIP97 (as an update) as well as for EDIP2003.
The stratospheric content of ozone is disturbed as a consequence of man-made emissions of halocarbons, i.e., CFCs, HCFCs, halons and other long-lived gases containing chlorine and bromine. These
substances increase the breakdown of stratospheric ozone, and the ozone content of the stratosphere is therefore falling, and since 1985 an annually occurring dramatic thinning of the ozone layer has been,
often referred to as the "ozone hole", over the South Pole. In the last few years, the breakdown of ozone has also accelerated over the northern hemisphere. As a consequence of the thinning of the ozone
layer, the intensity of hazardous ultraviolet radiation at the earth's surface has increased over parts of the southern and northern hemispheres. This can have dangerous consequences in the form of increased
frequency of skin cancer in humans and damage to the plants which are the primary producers and hence the foundation of the polar ecosystems.
In spite of a nearly complete abandoning of the main contributors to global warming, the conditions of the stratosphere are not expected to be normalised before the second half of this century.
3.2 Classification
For a substance to be regarded as contributing to stratospheric ozone depletion, it must
- be a gas at normal atmospheric temperatures
- contain chlorine or bromine
- be stable with a life in the atmosphere of a few years to centuries, so that it can be transported up into the stratosphere.
The man-made substances contributing to the stratospheric breakdown of ozone are simple gaseous organic compounds with a substantial content of
chlorine, bromine or possibly fluorine. The most important groups of ozone-depleting halocarbons are the CFCs, the HCFCs, the halons and methyl bromide. In contrast to these, the HFCs are a group of
halocarbons containing neither chlorine nor bromine, and which are therefore not regarded as contributors to the stratospheric breakdown of ozone.
As for the greenhouse gases, the list of compounds considered as contributing to the stratospheric breakdown of ozone is manageable and can be regarded as exhaustive. In practice, it will therefore not be
necessary to check a substance under the above criteria to decide whether it contributes to ozone depletion. It is sufficient to consult the list of ozone depletion equivalency factors in Table 3.1
3.3 EDIP2003 and updated EDIP97 characterisation factors
The endpoint of this impact category is chosen early in the environmental mechanism at the point of disturbance of the ozone content of the
stratosphere, and the EDIP2003 and revised EDIP97 characterisation factors are therefore taken from recommendations of the latest version of the WMO status report. The recommendation for EDIP97 is
still to use an infinite time horizon but to check the importance if a short time horizon (5 or 20 years) is applied (characterisation factors for shorter time horizons provided in Wenzel et al., 1997).
Table 3.1. Factors for characterisation of stratospheric ozone depletion (in g CFC-11-equivalents/g). Taken from Montzka, Frazer et al., 2002 with range representing spread of reported results from models and semi-empirical.
Substance |
Formula |
Lifetime, years |
Total ODP |
ODP range |
|
|
|
g CFC-11 eq/g |
g CFC-11 eq/g |
CFC-11 |
CFCl3 |
45 |
1 |
- |
CFC-12 |
CF2Cl2 |
100 |
1 |
0.82-0.9 |
CFC-113 |
CF2ClCFCl2 |
85 |
1 |
0.9 |
CFC-114 |
CF2ClCF2Cl |
300 |
0.94 |
0.85-1.0 |
CFC-115 |
CF2ClCF3 |
1.700 |
0.44 |
0.40-0.44 |
Tetrachloromethane |
CCl4 |
26 |
0.73 |
0.73-1.20 |
HCFC-22 |
CHF2Cl |
12 |
0.05 |
0.034-0.05 |
HCFC-123 |
CF3CHCl2 |
1.3 |
0.02 |
0.012-0.02 |
HCFC-124 |
CF3CHFCl |
5.8 |
0.02 |
0.02-0.026 |
HCFC-141b |
CFCl2CH3 |
9.3 |
0.12 |
0.037-0.12 |
HCFC-142b |
CF2ClCH3 |
17.9 |
0.07 |
0.014-0.07 |
HCFC-225ca |
C3F5HCl2 |
1.9 |
0.02 |
0.017-0.025 |
HCFC-225cb |
C3F5HCl2 |
5.8 |
0.03 |
0.017-0.03 |
1.1.1-Trichloroethane |
CH3CCl3 |
5 |
0.12 |
0.11-0.15 |
Methyl chloride |
CH3Cl |
1.3 |
0.02 |
- |
Halon 1301 |
CF3Br |
65 |
12 |
12-13 |
Halon 1211 |
CF2ClBr |
16 |
6 |
5-6 |
Halon 1202 |
CF2Br2 |
2.9 |
1.3 |
- |
Halon 2402 |
CF2BrCF2Br |
20 |
<8.6 |
- |
Methyl bromide |
CH3Br |
0.7 |
0.38 |
0.37-0.38 |
3.4 Normalisation
The updated EDIP97 person equivalent for stratospheric ozone depletion is
0.103 kg CFC-11-eq/pers/yr as found in Stranddorf et al., 2005.
4. Acidification
Background information for this chapter can be found in:
- Chapter 4 of the "Environmental assessment of products. Volume 2: Scientific background" by Hauschild and Wenzel (1998a).
- Chapter 3 of the "Background for spatial differentiation in life cycle impact assessment – EDIP2003 methodology" by Potting and Hauschild (2005).
4.1 Introduction
Releases of nitrogen (NOx and NH3) and sulphur (SO2) to air account in most countries for more than 95% of the total acidifying emissions. On a national level, acidifying emissions thus consist mainly of
nitrogen and sulphur. In the life cycle inventory of a product, however, other substances may dominate the total mass of acidifying emissions.
Acidifying emissions are usually dispersed and converted before they are deposited on terrestrial and aquatic systems. The scale of the deposition area depends on the characteristics of the substance and on
regional atmospheric conditions, but the main acidifying substances are transported over several hundred to thousand kilometres. The deposition of acidifying substances may lead to an increase of acidity
(i.e. decrease of pH) in the water or soil matrix. This phenomenon occurs when the base cation of the acid is leaving the system, while the hydrogen ion is left behind. Natural weathering of minerals,
nitrification, fixation of nitrogen in biomass, and fixation or precipitation of compounds of e.g. phosphor in the soil matrix are the main processes to avoid leaching.
Increase of acidity in for instance terrestrial systems leads to increased weathering of (essential) minerals. Weathering of minerals can to some extent neutralise acidifying depositions, though it also leads to an
imbalance of nutrients. When the pH falls to a critical level, toxic aluminium becomes mobile in harmful amounts. The aluminium affects the hair roots and thereby nutrition and water uptake of vegetation. The
resulting decrease in health lowers the ability of trees and other vegetation to cope with stress. The aluminium ions are also toxic to aquatic life in freshwater systems.
4.2 Classification
For a substance to be considered a contributor to acidification it must cause release of hydrogen ions in the environment and the base anions which accompany the hydrogen ions must be leached from the
system.
The number of substances that may contribute to acidification is not large, and in practice Table 4.1 contains all relevant substances that contribute to acidification. Note that emission of organic acids is not
regarded as a contribution to acidification because the base anion is generally degraded rather than leached.
4.3 EDIP97 characterisation factors
Presently, typical characterisation factors for acidification are based on the potential of substances to release hydrogen ions (i.e., the theoretical maximum acidification). The potential of a substance to release
hydrogen ions is expressed as the equivalent emission of sulphur (SO2). One mole of oxidised sulphur can produce two moles of hydrogen ion. The EDIP97 factors are listed in Table 23.5 in Wenzel et al.
(1997).
There are several problems with characterisation factors based on substance potentials to release hydrogen ions. This approach does not take into account that:
- The geographical region of release and regional meteorological conditions determine the relevant deposition pattern of an emission. For every process in the life cycle of a product, the acidifying emission
deposits on a large area containing very many ecosystems. So while geographically close sources have strongly overlapping deposition areas, this is not the case for sources which lie several hundred
kilometres from each other.
- The other way around, the extent to which an ecosystem already receives acidifying depositions from other sources (background deposition) depends on its location in relation to major industrialised and
inhabited areas. Most ecosystems receive acidifying depositions from very many sources, which usually makes the contribution from a single source to the total deposition very small.
- Ecosystems differ in their natural capacity to avoid leaching of base cat-ions and/or to neutralise acidity by weathering of minerals, and the already operative "background" deposition to an ecosystem
determines to what extent its capacity is used and additional deposition is harmful.
As a result, the theoretical maximum capacity of an acidifying substance to release hydrogen ions is usually not determining the acidification impact. Specifically the acidifying impact from nitrogen emissions is
overrated compared to sulphur when the potential to release hydrogen is used as impact indicator, and the final acidifying impact depends on the geographic location where an emission is released.
4.4 EDIP2003 characterisation factors
As argued in the previous section, the potential to release hydrogen is a poor measure to express the acidifying impact of an emission. In EDIP2003, the RAINS model1 is used to establish acidification
factors which overcome most of the identified problems. Site-generic factors have been established (see Table 4.1), as well as site-dependent factors for 44 European countries or regions (see Annex 4.1 to
this chapter). The acidification factors relate an emission by its region of release to the acidifying impact on its deposition areas.
The RAINS model (version 7.2) estimates dispersion and deposition of nitrogen and sulphur compounds on grid elements (150 km resolution), resulting from the emissions from 44 countries or regions in
Europe. The grid consists of 612 elements covering all 44 European regions, including the European part of the former Soviet Union. Total deposition for one grid element is computed by adding up the
contributions from every region and the background contribution for that grid element. The dispersion and deposition estimates are done with source-receptor matrices based on the EMEP model - a
Lagrangian or trajectory model. In this model, an air parcel is followed on its way through the atmosphere along its (horizontal) travel during 96 hours preceding their arrival at a specified grid element.
Figure 4.1 Two dimensional trajectories of atmospheric motion of an air parcel (Alcamo et al. 1990).
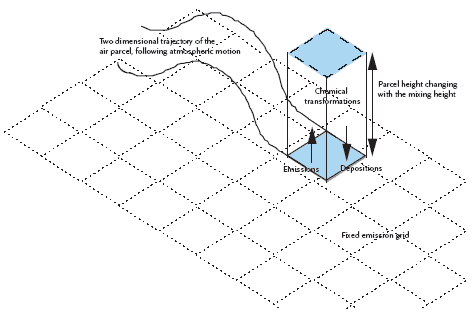
1 RAINS is an integrated assessment model that combines information on national emission levels with information on long range atmospheric transport in order to estimate patterns of deposition and
concentration for comparison with critical loads and thresholds for acidification, terrestrial eutrophication-via-air and tropospheric ozone formation.
The soil capacity to compensate for acid deposition is described by the critical acid load. Critical load functions for acidification of forest soils, heath land, grassland, peatland and freshwater have been
estimated for the grid elements, and cumulative distribution curves for ecosystem sensitivities have been compiled in the RAINS model for all ecosystems within each grid element (for some grid elements
over 30.000 ecosystems have been registered).
The RAINS model calculates the site-dependent characterisation factor for a country by looking at a fixed, but marginal emission of the substance from this country (e.g. 1 ton NOx) on top of the actual
emissions from all countries together. The impact from the resulting deposition increase is the additional area of ecosystem becoming exposed above the critical acidification load. For each grid element, the
impact increment is determined from the cumulative distribution curve of unprotected ecosystems in the grid element. The impact increments for all grid elements within the deposition area are summed and
expressed as the total area of ecosystem becoming unprotected, i.e. exceeding its critical load, as consequence of the emission.
A more detailed description of the RAINS model and its use for calculation of site-dependent characterisation factors can be found in Potting and Hauschild, 2005.
The application of the EDIP2003 site-generic acidification factors is similar to the application of EDIP97 factors which are also site-generic (see next section).
Application of the site-dependent acidification factors is also straightforward (see Section 4.6). Typical life cycle inventories already provide the only additional information which is required for
site-dependent characterisation, namely the geographical region where the emission takes place.
The use of site-dependent acidification factors adds a resolving power of a thousand between highest and lowest ratings, while combined uncertainties in the RAINS model are cancelled out to a large extent
in the characterisation factors due to the large area of ecosystems they cover.
The dependence on the background situation of the receiving environment means that the potential for acidification must be expected to vary with the total emission level and hence in time. To allow
assessment of this variation, the characterisation factors are also calculated for the predicted emission levels for 2010 as shown in Annex 4.1. The factors based on the 1990 emissions are chosen as the
default EDIP2003 characterisation factors but the factors for 2010 allow temporal differentiation for those emissions of the product system that will take place in the future (e.g. from the late use stage of
long-lived products or from the disposal stage). Compared to the spatially determined variation between countries, the temporal variation within countries, determined in this way, is modest.
What do the impacts express?
The site-generic as well as the site-dependent EDIP2003 acidification potentials of an emission from a functional unit are expressed as the area of ecosystem within the full deposition area which is brought to
exceed the critical load of acidification as a consequence of the emission (area of unprotected ecosystem = m2 UES/f.u.).
In comparison, the EDIP97 acidification potential is expressed as the emission of SO2 that would lead to the same potential release of protons in the environment (g SO2-eq/f.u.).
4.5 Site-generic characterisation
The site-generic acidification factors are established as the European average over the 15 EU member countries in EU15 plus Switzerland and Norway, weighted by the national emissions in Table 4.1.
The site-generic acidifying impact of a product can be calculated according to the following formula:

(4.1)
Where: sg-EP(ac) = The site-generic acidification impact, or area of ecosystem that becomes unprotected by the emission from the product system (in 0.01 m2/f.u.).
sg-CF(ac)s = The site-generic
characterisation factor for acidification from Table 4.1 that relates emission of substance (s) to the acidifying impact on its site-generic deposition area (in 0.01 m2/g).
Es = The emission of substance (s) (in g/f.u).
The spatially determined variation which potentially lies hidden within the site-generic acidification impact, can be estimated from the standard deviation given in Table 4.1 for each substance.
Table 4.1. Equivalency factors for site-generic, and for site-dependent characterisation (in 0.01 m2 unprotected ecosystem/g)
| Site-generic
assessment |
Site-dependent
assessment |
|
Site-generic characterisation factors = sg-CF(ac)s |
Site-dependent characterisation factors (factors to be found
in Annex 4.1) |
Substance |
Factor |
standard deviation |
factor = sd-CF(ac)s.i |
|
|
|
|
SO2 |
1.77 |
(2.29) |
sd-CF(ac)|(SO2) |
SO3 |
1.41 |
(1.83) |
0.80•sd-CF(ac)|(SO2) |
H2SO4 |
1.15 |
(1.49) |
0.65•sd-CF(ac)|(SO2) |
H2S |
3.32 |
(4.29) |
1.88•sd-CF(ac)|(SO2) |
NO2 |
0.86 |
(0.72) |
sd-CF(ac)|(NO2) |
NOx |
0.86 |
(0.72) |
sd-CF(ac)|(NO2) |
NO |
1.31 |
(1.11) |
1.53•sd-CF(ac)|(NO2) |
HNO3 |
0.63 |
(0.53) |
0.73•sd-CF(ac)|(NO2) |
NH3 |
2.31 |
(3.04) |
sd-CF(ac)|(NH3) |
HCl |
6.20 |
(9.53) |
(**) 100•sd-CF(ac)|(H+)/36.46 |
HF |
11.30 |
(17.36) |
(**) 100•sd-CF(ac)|(H+)/20.01 |
H3PO4 * |
- |
- |
- |
*Phosphate will normally bind to the soil matrix and then phosphoric acid will not contribute to acidification
**The unit of sd-CF(ac)i(H+) in Annex 4.1 is m2/g, whereas the unit for the factors of the other substances is 0.01 m2/g
4.6 Site-dependent characterisation
The acidifying impact from a product system is often determined by one or a few processes. To avoid unnecessary work, applications where a site-dependent assessment is desired, may therefore start with
calculation of the site-generic acidifying impact of the product as described in the previous section. This site-generic impact can be used to select the processes with the dominating contributions (step 1), and
then to adjust their site-generic impacts with the relevant site-dependent acidification factors (step 2 and 3). This procedure can be seen as a sensitivity analysis-based reduction of those uncertainties in the
site-generic impact which are caused by refraining from site-dependent characterisation.
Step 1
The site-generic acidifying impact of a product, as calculated in the previous section, is broken down into the contributions from the separate processes. These contributions are then ranked from the largest
to the smallest contribution, and the process with the largest acidifying contribution is selected.
Step 2
The site-generic acidifying impact of the product calculated in step 1 is reduced with the contribution of the process selected in step 1. Next, the site-dependent impact from the emissions of this process is
calculated with the relevant site-dependent acidification factors in Annex 4.1.

(4.2)
Where: sd-EP(ac)p= The site-dependent acidifying impact or area of ecosystem that becomes unprotected by the selected process (p) (in m2/f.u.).
sd-CF(ac)s,i= The site-dependent characterisation factor for acidification from Annex 4.1 (default 1990 factors) that relates the emission of substance (s) in country or region
(i) where the selected process (p) is located to the acidifying impact on its deposition area (in m2/g). Emissions from an unknown region or from non-European regions can as a first approach be represented
by the site-generic factors.
Es,p = The emission of substance (s) from the selected process (p) (in g/f.u).
The geographic region in which the emissions take place determines the relevant factors. The impact of emissions from unknown but probably European regions should be calculated with the site-generic
acidification factors. The information about the spatial variation in these factors (see Table
4.1) should be taken into account in the next step. As a first approach, also the emissions from a non-European or unknown region can be calculated with the site-generic acidification factors from Table 4.1.
The standard deviations in Table 4.1 give a range of potential spatial variation for the application of the site-generic factor within Europe. Given the size of the variation in emissions and sensitivities within
Europe, the site-dependent factor is expected to lie within this range for most regions also in the rest of the world. Expert judgement may be used in the interpretation to assess whether the factor for
emissions from processes in non-European regions should be found in the lower or upper end of the range.
Step 3
The site-dependent contributions from the process selected in step 1 are added to the adjusted site-generic contribution from step 2. Step 2 is repeated until the site-dependent contribution from the selected
processes is so large that the residual spatially determined variation in the acidification score can no longer influence the conclusion of the study (e.g. when the site-dependent share is larger than 95% of the
total impact score).
4.7 Normalisation
The EDIP2003 person equivalent for acidification is 2.2?103 m2/pers/yr.
Following the EDIP97 approach, the normalisation reference for acidification is based on the impact caused by the actual emission levels for 1990 (see Hauschild and Wenzel, 1998c and Stranddorf et al.,
2005). Applying the EDIP2003 characterisation factors for acidification, the total area of unprotected ecosystem in Europe is 82?106 ha or 82?1010 m2. The person equivalent is calculated as an average European impact per
person assuming a total European population of 3.70?108 persons.
4.8 Interpretation
The EDIP2003 acidification impact potentials are improved in two aspects compared to the impact potentials calculated using the EDIP97 characterisation factors; the environmental relevance is increased,
and spatial variation in the sensitivity of the receiving environment can be taken into account.
Environmental relevance
The environmental relevance is increased because the exposure of the sensitive parts of the environment as well as the variation in sensitivity of these ecosystems are included in the underlying model, which
now covers most of the causality chain towards the protection area: Ecosystem health. This is particularly important because it increases consistency with weighting factors based on the environmental
relevance. The EDIP default weighting factors for acidification are based on political reduction targets. These targets are also aimed partly at protecting ecosystem health. In comparison, the EDIP97 factors
only cover the potential for release of protons.
Being defined so early in the cause-effect chain, the EDIP97 impacts in principle do not exclude any damage caused by the like the damage to man-made materials. For the EDIP2003 characterisation
factors, damage to natural ecosystems is chosen as the most sensitive end point (and as the end point that current regulation is focused on), and therefore damage to man-made materials is not explicitly
addressed by these factors (although it will at least partly be represented). If there should be a wish to explicitly include acidification damage to man-made materials, these must thus be calculated separately
using e.g. the EDIP97 factors.
Spatial variation
The spatial variation in sensitivity to exposure for acidification is large due to differences in background exposure of ecosystems and their natural resilience to acidifying impacts. The variation in sensitivity
between European regions shows a factor 103 of difference between least and the most sensitive emission countries when expressed on a national scale. This variation is hidden when the EDIP97
characterisation factors or similar site-generic factors are used for characterisation.
4.9 Example
Applying the EDIP2003 factors, characterisation is performed on the inventory presented in Section 1.6.
Site-generic characterisation
As described in Section 4.5, first, the site-generic impacts are calculated. The acidification impacts shown in Table 4.2 are determined using the site-generic factors from Table 4.1.
Table 4.2. Site-generic acidification impacts for one supporting block made from plastic or zinc expressed as area of unprotected ecosystem (UES) per functional unit.
| Emissionto air from
plastic part |
|
Emission to
airfromzinc part |
Site-generic acidification
factors.Table 4.1 |
Site-generic
acidification
impact
of
plastic part |
Site-generic acidification impact
of
zinc part |
Substance |
g/f.u. |
|
g/f.u. |
0.01 m2
UES/g |
0.01
m2
UES/f.u |
0.01 m2
UES/f.u |
|
|
|
|
Middel |
std.afv. |
Middel |
std.afv. |
Middel |
std.afv. |
Hydrogen chloride |
0.001163 |
|
0.00172 |
6.2 |
9.5 |
0.0072 |
0.011 |
0.011 |
0.016 |
Carbon moNOxide |
0.2526 |
|
0.76 |
|
|
|
|
|
|
Ammonia |
0.003605 |
|
0.000071 |
2.31 |
3.04 |
0.0083 |
0.011 |
0.00016 |
0.00022 |
Methane |
3.926 |
|
2.18 |
|
|
|
|
|
|
VOC, power plant |
0.0003954 |
|
0.00037 |
|
|
|
|
|
|
VOC, diesel engines |
0.02352 |
|
0.0027 |
|
|
|
|
|
|
VOC, unspecified |
0.89 |
|
0.54 |
|
|
|
|
|
|
Sulphurdioxide |
5.13 |
|
13.26 |
1.77 |
2.29 |
9.1 |
11.7 |
23.5 |
30.4 |
Nitrogen oxides |
3.82 |
|
7.215 |
0.86 |
0.72 |
3.3 |
2.8 |
6.2 |
5.2 |
Lead |
8.03•10-5 |
|
0.000260 |
|
|
|
|
|
|
Cadmium |
8.66•10-6 |
|
7.45•10-5 |
|
|
|
|
|
|
Zinc |
0.000378 |
|
0.00458 |
|
|
|
|
|
|
Total |
|
|
|
|
|
12.4 |
14.5 |
29.7 |
35.6 |
Using site-generic characterisation factors, the largest acidification impacts are found to be caused by the zinc supporting block. However, the potential spatial variation is so large (as revealed by the spatially
determined standard deviation) that the conclusion is highly uncertain. Therefore, a site-dependent characterisation is performed for those processes which contribute most to the site-generic acidification
impacts in order to reduce the spatially determined uncertainty and strengthen the conclusion.
Site-dependent characterisation
Table 4.2 shows that the predominant contributions to the site-generic acidification impact are caused by emissions of SO2 and NOx. For the zinc component, the main sources for both substances are
identified as the production of zinc from ore which takes place in Bulgaria, the casting of the component which takes place in Yugoslavia, and that part of the transport of
the component, which takes place by truck through Germany (data not shown). For the plastic component, the main sources for both SO2 and NOx are found to be the production of plastic polymer in
Italy, the flow injection moulding of the supporting block in Denmark and the transportation of the component by truck, mainly through Germany (idem). The emissions from these processes contribute
between 91 and 99% the full site-generic impacts of Table 4.2 (data not shown).
In the calculation of the site-dependent impacts for these key processes, the relevant factors from Annex 4.1 are applied. The results are shown in Table
4.3.
Table 4.3. Site-dependent acidification impacts for key processes from either product system.
Zinc part |
|
Emission |
Acidification
factor, Annex 4.1 |
Impact |
|
|
|
g/f.u. |
0.01 m2 UES/g |
0.01 m2UES/f.u |
|
SO2 emissions |
|
|
|
|
|
Zinc production. Bulgaria |
|
9.16 |
0.07 |
0.64 |
|
Zinc casting. Yugoslavia |
|
2.71 |
0.24 |
0.65 |
|
Transport. mainly Germany |
|
1.18 |
2.17 |
2.6 |
|
NOx emissions |
|
|
|
|
|
Zinc production, Bulgaria |
|
0.97 |
0.02 |
0.019 |
|
Zinc casting, Yugoslavia |
|
1.65 |
0.04 |
0.066 |
|
Transport, mainly |
|
4.56 |
0.9 |
4.1 |
|
Total. zinc part |
|
|
|
8.00 |
|
Plastic part |
|
Emission |
Acidification
factor, Annex 4.1 |
Impact |
|
|
|
g/f.u. |
0.01 m2 UES/g |
0.01 m2 UES/f.u |
|
SO2 emissions |
|
|
|
|
|
Plastic production. Italy |
|
2.43 |
0.56 |
1.4 |
|
Flow injection moulding, Denmark |
|
2.11 |
5.56 |
11.7 |
|
Transport, mainly Germany |
|
0.45 |
2.17 |
0.98 |
|
NOx emissions |
|
|
|
|
|
Plastic production, Italy |
|
0.63 |
0.14 |
0.09 |
|
Flow injection moulding, Denmark |
|
0.48 |
2.02 |
0.97 |
|
Transport, mainly Germany |
|
1.74 |
0.9 |
1.6 |
|
Total. plastic part |
|
|
|
16.7 |
|
The site-generic impacts from the key processes are subtracted from the original site-generic impacts in Table 4.2 and the site-dependent impacts from the key processes calculated in Table 4.3 are added.
The acidification impacts thus corrected are found in Table 4.4, and the difference to the original site-generic impacts of Table 4.2 is illustrated in Figure 4.2.
Table 4.4. Acidification impacts from either product system with site-dependent characterisation of key process emissions
| Acidification |
|
0.01 m2 UES/f.u |
Zinc component |
8.8 |
Plastic component |
18.9 |
Around 95% of the resulting impact is calculated using site-dependent characterisation factors for both the zinc-based and the plastic-based component. Even if the site-dependent characterisation was
performed for all the remaining processes in the product system, the result can thus not change significantly, given their modest share in the total and the standard deviation. The spatially conditioned potential
for variation of the impact has largely been cancelled.
As seen from Figure 4.2, the inclusion of spatial differentiation at the level of country of emission reverses the dominance. When the major part of the spatial variation in the dispersion patterns and sensitivity
of the exposed environment is removed, the acidification impact from the plastic component is larger than the acidification impact from the zinc component.
Figure 4.2 Site-generic and site-dependent acidification impacts from the two product systems. For the site-dependent impacts, the site-dependent characterisation factors have only been applied for the
key processes as described above.
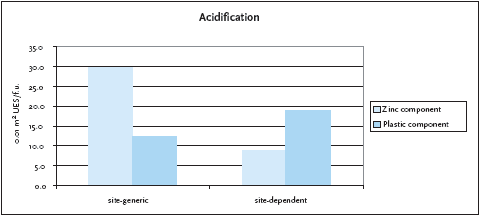
Annex 4.1: Site-dependent characterisation factors for acidification
|
1990 Acidification factors |
2010 Acidification factors |
|
SO2 |
NOx |
NH3 |
H+ eq. |
SO2 |
NOx |
NH3 |
H+ eq. |
Region |
(0.01 m2/g) |
(0.01 m2/g) |
(0.01 m2/g) |
(m2/μeq.) |
(0.01 m2/g) |
(0.01 m2/g) |
(0.01 m2/g) |
(m2/μeq.) |
Albania |
0.02 |
0.00 |
0.01 |
0.00 |
0.01 |
0.00 |
0.00 |
0.00 |
Austria |
1.31 |
0.42 |
3.44 |
2.17 |
1.75 |
0.51 |
4.42 |
1.95 |
Belarus |
4.65 |
4.54 |
5.72 |
0.15 |
0.38 |
0.09 |
0.20 |
0.01 |
Belgium |
1.28 |
0.82 |
1.10 |
6.05 |
1.62 |
0.87 |
2.15 |
0.38 |
Bosnia/Herzegovina |
0.15 |
0.04 |
0.06 |
0.00 |
0.09 |
0.02 |
0.03 |
0.00 |
Bulgaria |
0.07 |
0.02 |
0.05 |
0.00 |
0.03 |
0.01 |
0.02 |
0.00 |
Croatia |
0.30 |
0.12 |
0.17 |
0.06 |
0.28 |
0.10 |
0.15 |
0.01 |
CRFZ |
1.91 |
0.69 |
1.26 |
0.12 |
2.64 |
0.78 |
8.30 |
3.06 |
Denmark |
5.56 |
2.02 |
5.28 |
0.84 |
2.99 |
0.90 |
2.30 |
0.19 |
Estonia |
12.43 |
1.54 |
3.92 |
0.37 |
1.58 |
0.18 |
0.61 |
0.14 |
Finland |
15.14 |
2.42 |
13.40 |
7.33 |
3.53 |
0.30 |
1.33 |
3.28 |
France |
0.79 |
0.47 |
0.74 |
0.50 |
0.90 |
0.53 |
0.89 |
0.03 |
Germany new |
2.17 |
0.90 |
1.89 |
0.33 |
2.39 |
0.87 |
4.52 |
1.11 |
Germany old |
1.94 |
1.42 |
3.31 |
|
2.32 |
1.03 |
4.59 |
|
Greece |
0.01 |
0.00 |
0.01 |
0.00 |
0.01 |
0.00 |
0.00 |
0.00 |
Hungary |
2.08 |
0.37 |
0.90 |
0.13 |
0.48 |
0.16 |
0.47 |
0.05 |
Ireland |
0.78 |
0.57 |
1.11 |
0.04 |
1.54 |
0.89 |
2.50 |
0.04 |
Italy |
0.56 |
0.14 |
0.47 |
0.56 |
0.50 |
0.21 |
1.08 |
0.29 |
Latvia |
2.39 |
1.12 |
1.90 |
0.22 |
0.65 |
0.15 |
0.22 |
0.00 |
Lithuania |
6.85 |
1.00 |
1.67 |
0.43 |
0.63 |
0.16 |
0.26 |
0.01 |
Luxemburg |
0.86 |
0.43 |
1.89 |
0.32 |
1.00 |
0.63 |
1.70 |
0.21 |
Netherlands |
1.24 |
0.97 |
1.55 |
0.04 |
1.47 |
0.88 |
3.04 |
0.57 |
Norway |
10.90 |
2.80 |
14.25 |
6.34 |
6.87 |
1.34 |
10.95 |
6.89 |
Poland |
2.79 |
1.73 |
5.08 |
0.44 |
1.11 |
0.36 |
1.27 |
0.49 |
Portugal |
0.02 |
0.01 |
0.01 |
0.01 |
0.01 |
0.02 |
0.01 |
0.01 |
Moldova |
0.17 |
0.02 |
0.14 |
0.17 |
0.01 |
0.00 |
0.02 |
0.00 |
Rumania |
0.43 |
0.14 |
0.35 |
0.00 |
0.14 |
0.05 |
0.11 |
0.02 |
Kaliningrad region |
1.23 |
0.07 |
0.45 |
3.42 |
0.31 |
0.01 |
0.08 |
2.33 |
Kola, Karelia |
16.45 |
0.21 |
1.12 |
|
28.97 |
0.03 |
0.14 |
|
Remaining Russia |
5.68 |
0.89 |
4.42 |
|
0.22 |
0.03 |
0.06 |
|
St.Petersborg reg. |
11.60 |
1.04 |
3.35 |
|
1.25 |
0.10 |
0.35 |
|
SKRE |
1.36 |
0.47 |
2.68 |
1.70 |
0.60 |
0.21 |
0.63 |
0.16 |
Slovenia |
1.16 |
0.27 |
2.78 |
4.07 |
1.70 |
0.38 |
3.45 |
0.95 |
Spain |
0.13 |
0.04 |
0.04 |
0.08 |
0.14 |
0.06 |
0.07 |
0.06 |
Sweden |
13.82 |
3.03 |
17.68 |
11.89 |
4.31 |
0.78 |
4.61 |
3.14 |
Switzerland |
1.28 |
0.42 |
2.63 |
0.96 |
1.15 |
0.58 |
2.56 |
0.59 |
|
|
|
|
|
|
|
|
|
Ukraine |
1.27 |
1.27 |
1.98 |
0.32 |
0.13 |
0.04 |
0.11 |
0.03 |
United Kingdom |
1.94 |
0.92 |
4.32 |
1.01 |
2.19 |
1.07 |
6.75 |
2.26 |
Yugoslavia |
0.24 |
0.04 |
0.10 |
0.00 |
0.12 |
0.02 |
0.05 |
0.00 |
Atlantic ocean |
0.19 |
0.14 |
|
|
0.38 |
0.22 |
|
|
Mediterranean sea |
0.00 |
0.00 |
|
|
0.00 |
0.00 |
|
|
Baltic sea |
4.48 |
1.77 |
|
|
1.72 |
0.48 |
|
|
North sea |
1.58 |
0.94 |
|
|
1.83 |
0.88 |
|
|
(*) Mean |
1.77 |
0.86 |
2.31 |
2.26 |
1.93 |
0.64 |
2.97 |
3.47 |
(*) Standard deviation |
2.29 |
0.72 |
3.04 |
3.47 |
1.71 |
0.39 |
2.74 |
1.23 |
Minimum |
0.01 |
0.00 |
0.01 |
0.00 |
0.01 |
0.00 |
0.00 |
0.00 |
Maximum |
16.45 |
4.54 |
17.68 |
11.89 |
28.97 |
1.34 |
10.95 |
6.89 |
(*) The mean and standard deviations relate to E15+Norway+Switzerland and are for nitrogen and sulphur weighed with the national emissions of these countries
Footnotes
1 RAINS is an integrated assessment model that combines information on national emission levels with information on long range atmospheric transport in order to estimate patterns of deposition and
concentration for comparison with critical loads and thresholds for acidification, terrestrial eutrophication-via-air and tropospheric ozone formation.
5. Terrestrial eutrophication
Background information for this chapter can be found in:
- Chapter 5 of the "Environmental assessment of products. Volume 2: Scientific background" by Hauschild and Wenzel (1998a).
- Chapter 4 of the "Background for spatial differentiation in life cycle impact assessment – EDIP2003 methodology" by Potting and Hauschild (2005).
5.1 Introduction
Nutrients are essential to ensure (re-)production and subsistence of aquatic and terrestrial systems. Enrichment of ecosystems with nutrients, the literal meaning of the term "eutrophication", is therefore not
harmful until a certain, critical level (critical load) is reached. Each ecosystem and each of its species has its own level of nutrients that relates to optimum growth. Availability of nutrients in excess of this
optimum or critical load leads to a change of the species composition and hence to an unwanted change in the character of the given ecosystem.
5.2 Classification
Normally, biological growth in terrestrial ecosystems is limited by nitrogen. In principle, most compounds containing nitrogen will thus contribute to terrestrial eutrophication, but in practice, Table 5.1 will
cover all emissions in the inventory to be classified as terrestrial eutrophying. For natural soils, atmospheric deposition provides the main man-made supply of nitrogen (and other nutrients).
Free nitrogen (N2) does not contribute to terrestrial eutrophication, even though it is available for certain bacteria and algae. This is because emission of N2 has no additional fertilising effect, inasmuch as the
greater part of the atmosphere already consists of free nitrogen.
In practice, only air-borne emissions will contribute to eutrophication of terrestrial natural ecosystems.
5.3 EDIP97 characterisation factors
Current characterisation factors for eutrophication are typically based on the Redfield ratio. The Redfield ratio refers to the typical composition of aquatic phytoplankton: C106H263O110N16P. The
presently typical eutrophication factors do in most cases not distinguish between aquatic systems and terrestrial systems and actually model both as if they were impacts on aquatic systems. This is also the
case with the EDIP97 factors as listed in Table 23.6 in Wenzel et al. (1997). Only Lindfors et al. (1995) explicitly assess the impact on terrestrial systems by summing nitrogen emissions to air separately.
Additional problems with the current methods for characterisation of terrestrial eutrophication lie in the fact that they disregard the large spatial variations in dispersion patterns and in ecosystem sensitivities
within the deposition areas. These problems are discussed in Section 4.3 under acidification.
5.4 EDIP2003 characterisation factors
Until now, no characterisation factors have been developed specifically for terrestrial eutrophication. In the current context, the RAINS model2 has been used to establish terrestrial eutrophication factors.
Site-generic factors have been established (see Table 5.1), as well as site-dependent factors for 44 European regions (see the Annex 5.1 to this chapter) which through the region of release relates a nitrogen
emission to the terrestrial eutrophication, it causes within its deposition area. The principles of the RAINS-model and its use for calculation of site-dependent characterisation factors are described in Section
4.4.
The application of the EDIP2003 site-generic factors for terrestrial eutrophication is basically similar the application of the likewise site-generic EDIP97 factors (Section 5.5).
Application of the site-dependent factors for terrestrial eutrophication is also straightforward (see Section 5.6). Typical life cycle inventories already provide the only additional information which is required
for site-dependent characterisation, namely the geographical region where the emission takes place. The use of site-dependent terrestrial eutrophication factors adds resolving power of up to a factor
thousand between highest and lowest ratings, while combined uncertainties in the RAINS model to a large extent are cancelled out in the characterisation factors due to the large area of ecosystems they
cover. It has to be mentioned, however, that the critical loads for terrestrial eutrophication are more uncertain than those for acidification.
The dependence on the background situation of the receiving environment means that the potential for terrestrial eutrophication must be expected to vary with the total emission level and hence in time. To
allow assessment of this variation, the characterisation factors are also calculated for the predicted emission levels for 2010 as shown in Annex 5.1. The factors based on the 1990 emissions are chosen as
the default EDIP2003 characterisation factors but the factors for 2010 allow temporal differentiation for those emissions of the product system that will take place in the future (e.g. from the late use stage of
long-lived products or from the disposal stage).
2 RAINS is an integrated assessment model that combines information on national emission levels with information on long range atmospheric transport in order to estimate patterns of deposition and
concentration for comparison with critical loads and thresholds for acidification, terrestrial eutrophication-via-air and tropospheric ozone creation.
Compared to the spatially determined variation between countries, the temporal variation within countries, determined in this way, is less significant.
What do the impacts express?
The site-generic, as well as the site-dependent, EDIP2003 eutrophication potentials of an emission are expressed as the area of terrestrial ecosystem within the full deposition area that is brought to exceed
the critical load of eutrophication as a consequence of the emission (area of unprotected ecosystem = m2 UES).
In comparison, the EDIP97 nutrient enrichment potential aggregates the terrestrial and the aquatic eutrophication potentials. It is expressed as a separate N-potential and P-potential simply reflecting the
content of the two nutrients in the emission. EDIP97 also facilitates aggregation into an NO3--potential reflecting the amount of NO3- that would lead to the same potential eutrophication in aquatic systems
limited by the relevant nutrient (i.e. in the aggregation, N-emissions are assumed emitted to N-limited aquatic systems and P-emissions to P-limited systems).
5.5 Site-generic characterisation
The site-generic terrestrial eutrophication factors are established as the European average over the 15 EU member countries in EU15 plus Switzerland and Norway, weighted by the national emissions in
Table 5.1.
Table 5.1. Factors for site-generic, and for site-dependent characterisation (in
0.01 m2 unprotected ecosystem/g)
|
Site-generic assessmentsg-CF(te)s |
Site-dependent assessmentSite-dependent factors for terrestrial eutrophication(sd-CF(te)s.i factors in Annex 5.1) |
Substance |
Factor |
standard deviation |
|
NO2 | 2.54 | 2.34 | sd-CF(te)|(NO2) |
NOx | 2.54 | 2.34 | sd-CF(te)|(NO2) |
NO | 3.88 | 3.58 | 1.53•sd-CF(te)|(NO2) |
HNO3 | 1.85 | 1.77 | 0.73•sd-CF(te)|(NO2) |
NH3 | 10.10 | 13.11 | sd-CF(te)|(NH3) |
The site-generic terrestrial eutrophication impact of a product can be calculated according to the following formula:

(5.1)
Where: sg-EP(te) = The site-generic terrestrial eutrophication impact, or area of ecosystem that becomes unprotected by the emissions from the product system (in 0.01 m2/f.u.)
sg-CF(te)s = The site-generic characterisation factor for terrestrial eutrophication from Table 5.1 which relates accumulated emission of substance
(s) to the impact on its deposition area in 0.01 m2/g)
Es = The emission of substance (s) (in g/f.u)
The spatially determined variation which potentially lies hidden within the site-generic terrestrial eutrophication impact can be estimated from the standard deviation given in Table 5.1 for each substance.
5.6 Site-dependent characterisation
The terrestrial eutrophying impact from a product system is often determined by one or a few processes. To avoid unnecessary work, applications where a site-dependent assessment is desired, may
therefore start with calculation of the site-generic terrestrial eutrophication impact of the product as described in the previous section. This site-generic impact can be used to select the processes with the
dominating contributions (step 1), and next to adjust their site-generic impacts with the relevant site-dependent terrestrial eutrophication factors (step 2 and 3). This procedure can be seen as a sensitivity
analysis-based reduction of those uncertainties in the site-generic impact which are posed by refraining from site-dependent characterisation.
Step 1
The site-generic terrestrial eutrophication impact from a product, as calculated in the previous section, is broken down into the contributions of the separate processes. These contributions are then ranked
from the largest to the smallest contribution, and the process with the largest contribution is selected.
Step 2
The site-generic terrestrial eutrophication impact calculated in step 1 is reduced with the contribution of the process selected in step 1. Next, the site-dependent impact from the emissions of this process is
calculated with the relevant site-dependent terrestrial eutrophication factors in Annex 5.1.

(5.2)
Where: sd-EP(te)p = The site-dependent terrestrial eutrophication impact or area of ecosystem that becomes unprotected by the emissions from the selected process (p) (in m2/f.u.)
sd-CF(te)s,i = The site-dependent characterisation factor for terrestrial eutrophication from Annex 5.1 that relates the emission of substance (s) in country or region (i) where the selected process (p) is
located to the impact on its deposition area (in m2/g). Emissions from an unknown region or from non-European regions can as a first approach be represented by the site-generic factors.
Es,p = The emission of substance (s) from the selected process (p) (in g/f.u)
The geographic region in which the emissions take place determines the relevant factors. The impact of emissions from unknown but probably European regions is calculated with the site-generic factors for
terrestrial eutrophication. The information about the spatial variation in these factors (see Table 5.1) should be taken into account in the next step. As a first approach, also the emissions from a non-European
or unknown region can be calculated with the site-generic factors from Table 5.1. The standard deviations in Table 5.1 give a range of potential spatial variation for the application of the site-generic factor
within Europe. Given the size of the variation in emissions and sensitivities within Europe, the site-dependent factor is expected to lie within this range for most regions also in the rest of the world. Expert
judgement may be used in the interpretation to assess whether the factor for emissions from processes in non-European regions should be found in the lower or upper end of the uncertainty range.
Step 3
The site-dependent contributions from the process selected in step 1 are added to the adjusted site-generic contribution from step 2. Step 2 is repeated until the site-dependent contribution of the selected
processes is so large that the residual spatially determined variation in the terrestrial eutrophication score can no longer influence the conclusion of the study (e.g. when the site-dependent share is larger than
95% of the total contribution).
5.7 Normalisation
The EDIP2003 person equivalent for terrestrial eutrophication is
2.1?103 m2/pers/yr.
Following the EDIP97 approach, the normalisation reference for terrestrial eutrophication is based on the impact caused by the actual emission levels for 1990 (see Hauschild and Wenzel, 1998d and
Stranddorf et al., 2005). Applying the EDIP2003 characterisation factors for terrestrial eutrophication, the total area of unprotected ecosystem in Europe is 77•106 ha or 77•101010 m2. The person
equivalent is calculated as an average European impact per person assuming a total European population of 3.70•108 persons.
5.8 Interpretation
The EDIP2003 terrestrial eutrophication impact potentials are improved in two aspects compared to the impact potentials calculated using the EDIP97 characterisation factors; the environmental relevance is
increased and spatial variation in sensitivity of the receiving environment is now included.
Environmental relevance
The environmental relevance is increased because the exposure of the sensitive parts of the terrestrial environment as well as the variation in sensitivity of these ecosystems are included in the underlying
model, which now covers most of the causality chain towards the protection area: Ecosystem health. This is particularly important because it increases consistency with weighting factors based on the
environmental relevance. The EDIP default weighting factors for nutrient enrichment are based on political reduction targets. These targets are also aimed partly at protecting ecosystem health. In comparison,
the EDIP97 factors only cover the potential for release of nutrients and furthermore, the eutrophication of terrestrial ecosystems is treated using aquatic eutrophication factors even though there are important
differences in the two types of eutrophication.
Spatial variation
The spatial variation in natural soil sensitivity to eutrophication can be large due to differences in background exposure and natural nutrient status. The variation in sensitivity between European regions shows
a factor 103 of difference between least and the most sensitive emission countries when expressed on a national scale. This variation is hidden when the EDIP97 factors or similarsite-generic characterisation
factors are used for characterisation.
5.9 Example
Applying the EDIP2003 factors, characterisation is performed on the inventory presented in Section 1.6.
Site-generic characterisation
As described in Section 5.5, first the site-generic impacts are calculated. The terrestrial eutrophication impact shown in Table 5.2 is determined using the site-generic factors from Table 5.1.
Table 5.2. Site-generic terrestrial eutrophication impacts for one supporting block made from plastic or zinc expressed as area of unprotected ecosystem (UES) per functional unit.
Click here to see the Table
Using site-generic characterisation factors, the largest terrestrial eutrophication impacts are found to be caused by the zinc supporting block. However, the potential spatial variation is so large (as revealed by
the spatially determined standard deviation) that the conclusion is highly uncertain. Therefore, a site-dependent characterisation is performed for those processes that contribute the most to the site-generic
terrestrial eutrophication impacts in order to reduce the spatially determined uncertainty and strengthen the conclusion.
Site-dependent characterisation
Table 5.2 shows that the predominant contributions to the site-generic terrestrial eutrophication impact are caused by the emissions of NOx.A minor contribution from NH3 is negligible in the overall impact.
For the zinc component, the main sources for NOx emission are identified as the production of zinc from ore which takes place in Bulgaria, the casting of the component which takes place in Yugoslavia, and
that part of the transport of the component, which takes place by truck through Germany (data not shown). For the plastic component, the main sources for NOx are found to be the production of plastic polymer in Italy, the flow injection moulding of the supporting block in Denmark and the transportation
of the component by truck, mainly through Germany (idem). The emissions from these processes contribute 99% and 75% of the full site-generic impacts of Table
5.2 for the zinc component and the plastic component respectively (data not shown).
In the calculation of the site-dependent impacts for these key processes, the relevant site-dependent factors from Annex 5.1 are applied. The results are shown in Table 5.3.
The site-generic impacts from the key processes are subtracted from the original site-generic impacts in Table 5.2 and the site-dependent impacts from the key processes calculated in Table 5.3 are added.
The terrestrial eutrophication impacts thus corrected are found in Table 5.4, and the difference to the original site-generic impacts of Table 5.2 is illustrated in Figure 5.1.
Table 5.3. Site-dependent terrestrial eutrophication impacts for key processes from either product system.
Zink part |
|
|
Emissions.
g/f.u. |
Terr. Eutr. impact,
Annex 5.10.01 m2 UES/g |
Impact0.01 m2 UES/f.u |
NOx emissions |
|
|
|
|
|
Zinc production, Bulgaria |
|
|
0.97 |
1.02 |
0.99 |
Zinc casting, Yugoslavia |
|
|
1.65 |
5.55 |
9.16 |
Transport, mainly Germany |
|
|
4.56 |
2.04 |
9.30 |
Total, zinc part |
|
|
|
|
19.4 |
Plastic part |
|
|
Emissions. g/f.u. |
Terr. Eutr. impact,
Annex 5.1 |
Impact |
|
|
|
|
0.01 m2 UES/g |
0.01 m2 UES/f.u |
NOx emissions |
|
|
|
|
|
Plastic production. Italy |
|
|
0.63 |
1.12 |
0.71 |
Flow injection moulding, Denmark |
|
|
0.48 |
5.33 |
2.56 |
Transport, mainly Germany |
|
|
1.74 |
2.04 |
3.55 |
Total, plastic part |
|
|
|
|
6.8 |
Table 5.4. Terrestrial eutrophication impacts from either product system with site-dependent characterisation of key process emissions
| Terrestrialeutrophication |
|
0.01 m2 UES/f.u |
Zinc component |
19.5 |
Plastic component |
9.2 |
Site-dependent characterisation hardly influences the size of the terrestrial eutrophication impacts. The zinc component has the largest impact in both cases. Around 99% of this impact is calculated using
site-dependent characterisation factors for the zinc-based component while the site-dependent share for the plastic-based component is around 75%. Even if the site-dependent characterisation were
performed for all the remaining processes in the product system, the result would thus not change significantly for the zinc-based component, given their modest share in the total and the standard deviation.
The spatially conditioned potential for variation of the impact has largely been cancelled. For the plastic component it might be required to include one or two processes more to obtain the needed robustness
of the result but this would hardly change the dominance of the zinc component.
Figure 5.1 Site-generic and site-dependent terrestrial eutrophication impacts from the two product systems. For the site-dependent impacts, the site-dependent characterisation factors have only been
applied for the key processes as described above.
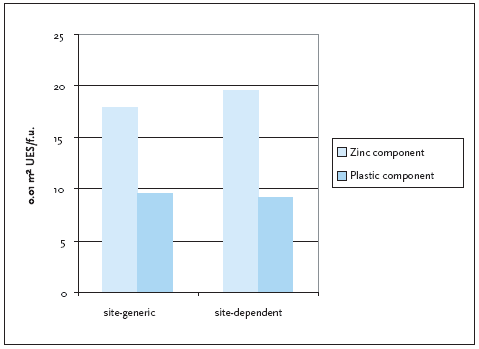
Annex 5.1: Site-dependent characterisation factors for terrestrial eutrophication
| 1990 factors |
2010 factors |
|
(in 0.01 m2 UES/gram) |
(in 0.01 m2 UES/gram) |
Region |
NOx |
NH3 |
NOx |
NH3 |
Albania |
1.58 |
6.91 |
0.80 |
3.12 |
Austria |
1.03 |
3.38 |
2.86 |
28.62 |
Belarus |
1.67 |
2.81 |
0.98 |
2.45 |
Belgium |
1.44 |
1.10 |
1.78 |
2.45 |
Bosnia/Herzegovina |
2.97 |
13.33 |
6.61 |
30.29 |
Bulgaria |
1.02 |
9.06 |
1.18 |
17.50 |
Croatia |
1.52 |
6.21 |
5.99 |
25.36 |
CRZF |
1.68 |
2.52 |
2.62 |
9.70 |
Denmark |
5.33 |
9.80 |
2.13 |
6.04 |
Estonia |
6.63 |
42.02 |
2.89 |
9.29 |
Finland |
11.29 |
91.69 |
3.40 |
79.00 |
France |
2.93 |
9.15 |
9.10 |
20.03 |
Germany new |
2.15 |
3.64 |
2.36 |
8.00 |
Germany old |
2.04 |
4.86 |
3.01 |
12.66 |
Greece |
0.56 |
15.67 |
0.42 |
2.04 |
Hungary |
1.70 |
5.67 |
7.33 |
20.73 |
Ireland |
0.37 |
0.51 |
0.15 |
0.19 |
Italy |
1.12 |
13.26 |
2.16 |
14.28 |
Latvia |
3.92 |
7.69 |
2.31 |
13.05 |
Lithuania |
3.23 |
5.72 |
2.11 |
14.98 |
Luxemburg |
0.10 |
0.16 |
1.30 |
3.61 |
Netherlands |
1.91 |
2.30 |
1.69 |
3.01 |
Norway |
6.29 |
10.11 |
1.09 |
0.75 |
Poland |
2.15 |
4.39 |
2.41 |
9.97 |
Portugal |
3.11 |
30.74 |
9.40 |
27.66 |
Moldova |
0.16 |
1.18 |
0.23 |
1.05 |
Romania |
1.29 |
5.18 |
2.09 |
7.02 |
Kaliningrad region |
0.21 |
0.92 |
0.62 |
2.80 |
Kola, Karelia |
0.72 |
5.07 |
0.21 |
1.73 |
Remaining Russia |
0.55 |
0.57 |
0.13 |
0.22 |
St.Petersborg reg. |
3.37 |
5.93 |
1.47 |
7.82 |
SKRE |
1.34 |
6.27 |
2.69 |
30.27 |
Slovenia |
1.09 |
10.22 |
2.38 |
21.83 |
Spain |
2.44 |
13.40 |
3.71 |
16.02 |
Sweden |
11.97 |
70.06 |
2.75 |
6.24 |
Switzerland |
0.90 |
5.76 |
2.65 |
24.78 |
Region |
NOx |
NH3 |
NOx |
NH3 |
Macedonia |
0.25 |
13.66 |
0.26 |
10.82 |
Ukraine |
0.62 |
3.42 |
0.47 |
3.40 |
United Kingdom |
1.77 |
3.14 |
0.84 |
0.89 |
Yugoslavia |
5.55 |
35.96 |
3.74 |
15.16 |
Atlantic ocean |
0.96 |
|
0.39 |
|
Baltic sea |
6.20 |
|
2.72 |
|
Mediterranean sea |
0.08 |
|
0.02 |
|
North sea |
1.86 |
|
1.15 |
|
(*) Mean |
2.54 |
10.10 |
3.25 |
13.51 |
(*) Standard deviation |
2.34 |
13.11 |
3.25 |
10.10 |
Minimum |
0.10 |
0.16 |
0.15 |
0.19 |
Maximum |
11.97 |
91.69 |
9.40 |
79.00 |
(*) The mean and standard deviations relate to E15+Norway+ Switzerland and are weighed with the national emissions of these countries
Footnotes
2 RAINS is an integrated assessment model that combines information on national emission levels with information on long range atmospheric transport in order to estimate patterns of deposition and
concentration for comparison with critical loads and thresholds for acidification, terrestrial eutrophication-via-air and tropospheric ozone creation.
6. Aquatic eutrophication
Background information for this chapter can be found in:
- Chapter 5 of the "Environmental assessment of products. Volume 2: Scientific background" from Hauschild and Wenzel (1998a).
- Chapter 5 of the "Background for spatial differentiation in life cycle impact assessment – EDIP2003 methodology" by Potting and Hauschild (2005)
6.1 Introduction
Eutrophication literally means "the process of becoming rich of nutrients". The eutrophying impact typically characterised in life cycle impact assessment relates implicitly to eutrophication of aquatic
ecosystems. This follows from the modelling of impact which in life cycle assessment usually takes its bases in the composition of aquatic biomass (Wenzel et al. 1997, Heijungs et al. 1992, Lindfors et al.
1995). Aquatic eutrophication is the subject of this chapter while impact assessment methodology for terrestrial eutrophication can be found in Chapter 5.
6.2 Classification
The nutrients, which normally limit biological growth in aquatic ecosystems, are nitrogen and phosphorus. For a compound to be regarded as contributing to aquatic eutrophication, it must thus contain
nitrogen or phosphorus in a form which is biologically available. In practice, Table 6.1 will cover all emissions in the inventory to be classified as aquatic eutrophying.
Free nitrogen (N2) is not regarded as a contributor to aquatic eutrophication, even if it is available for certain bacteria and algae. This is because emission of N2 has no additional eutrophying effect, inasmuch
as the greater part of the atmosphere already consists of free nitrogen.
Aquatic eutrophication can be caused by emissions to air, water and soil.
6.3 EDIP97 characterisation factors
Current characterisation factors for eutrophication allow adding contributions from nitrogen and phosphorus based on the Redfield ratio. These factors do in most cases not distinguish between aquatic
systems and terrestrial systems and model both as if they were impacts on aquatic systems. Also in EDIP97, terrestrial and aquatic eutrophication are taken together as one impact category called nutrient
enrichment. However, the Redfield ratio refers to the typical composition of aquatic phytoplankton:
C106H263O110N16P. A too large growth of phytoplankton, as a result of eutrophication, pushes aquatic ecosystems out of balance and starts a chain of ecological effects.
The EDIP97 factors from Wenzel et al. (1997) are listed in Table 6.1.
Table 6.1. Characterisation factors for eutrophication from Wenzel et al. (1997)
Substance |
Formula |
CF(N)
(g N-eq./g substance) |
CF(P)
(g P-eq./g substance) |
CF(ne)
(g NO3--eq/g substance) |
Nitrogen |
|
|
|
|
Nitrate |
NO3- |
0.23 |
0 |
1.00 |
Nitrogen dioxide |
NO2 |
0.30 |
0 |
1.35 |
Nitrite |
NO2- |
0.30 |
0 |
1.35 |
Nitrogen oxides |
NOx |
0.30 |
0 |
1.35 |
Nitrious oxide |
N2O |
0.64 |
0 |
2.82 |
Nitric oxide |
NO |
0.47 |
0 |
2.07 |
Ammonia |
NH3 |
0.82 |
0 |
3.64 |
Cyanide |
CN- |
0.54 |
0 |
2.38 |
Total Nitrogen |
N |
1.00 |
0 |
4.43 |
Phosphorus |
|
|
|
|
Phosphate |
PO43- |
0 |
0.33 |
10.45 |
Pyrophosphate |
P2O72- |
0 |
0.35 |
11.41 |
|
|
|
|
|
TotalPhosphorus |
P |
0 |
1.00 |
32.03 |
Wenzel et al. (1997) propose to aggregate impacts from nitrogen emissions (3rd column) and from phosphorus emissions (4th column) separately, but nevertheless also provide factors to add impacts from
phosphorus and nitrogen (5th column). The preferred separate aggregation of the two nutrients is due to the fact that phosphorus is typically limiting the growth of biomass in inland waters (rivers and lakes)
while nitrogen usually is the limiting nutrient in marine waters.
There are several problems with characterisation factors based on the Redfield ratio. Such factors do not take into account that:
- The hydrogeological conditions in the region of application determine the transport of nutrients from agriculture – by surface runoff/erosion (nitrogen and phosphorus), and groundwater drainage (nitrogen) –
to surface water.
- Phosphorus will usually not be removed again (at most temporarily stored in bottom sediment), but nitrogen does to some extent leave the aquatic system through denitrification (nitrate is used for respiration
in anaerobic biomass decomposition resulting in release of N2). The amount of nitrogen available for biomass growth over time is thus smaller than the amount entering the aquatic system.
- All nutrients remaining in the aquatic system will finally end up in marine waters, but some releases are directly to sea while most are first to inland waters and then through river transport reaching the sea.
- Aquatic ecosystems differ in their capacity to cope with eutrophication and subsequent phytoplankton growth, and the already operative "background" loading determines to what extent this capacity is used
and additional input of nutrient is harmful.
6.4 EDIP2003 characterisation factors
Until now, typical characterisation factors for aquatic eutrophication are based on the theoretical maximum amount of biomass that can be produced from a substance. As elaborated in the previous section,
the maximum potential for biomass growth is a poor measure of the eutrophying impact of emissions to aquatic ecosystems. In the current context, the CARMEN model3 is used to establish exposure factors
for aquatic eutrophication that overcome some of the identified problems (those relating to the fate of nutrients). The factors calculated by means of the Carmen model express the fraction of a nutrient
emission from agricultural soil or wastewater treatment plant that will reach and expose inland waters or marine waters, i.e. they relate emissions of nutrients to the resulting nutrient enrichment of
natural waters.
The CARMEN model (version 1.0) calculates the change in nutrient loads in ground water, inland waters (river catchment) and coastal seas from changes in input of nutrients. The nutrient inputs modelled by
CARMEN are atmospheric deposition of nitrogen on soil and coastal seas, phosphorus and nitrogen supply to agricultural soils, and phosphorus, and nitrogen discharged with municipal wastewater (see
Figure 6.1).
CARMEN models the transport of nutrients to surface water from agricultural supply, through groundwater drainage and surface runoff and through atmospheric deposition with a high spatial resolution
based on 124320 grid-elements of 10x10 minutes (roughly 100-250km2, depending on the longitude and latitude location of the grid-element). The nitrogen and phosphorus sources have been allocated to
each grid-element on the basis of the distribution of land uses in the given grid-element (arable land, grassland, permanent crops, forest, urban area, inland waters, others). The transport of nutrient by rivers
to sea is modelled rather straightforward in CARMEN assuming fixed removal rates of N and P in freshwater systems. The water flow is the main transport mechanism that brings nutrients from soil to
surface water. The routes modelled are deep groundwater drainage (nitrogen), runoff (nitrogen) or topsoil erosion (phosphorus) followed by river transport to coastal waters.
3 CARMEN is an acronym for CAuse effect Relation Model to support Environmental Negotiations. It is an integrated assessment model to analyse and evaluate strategies to reduce nutrient loading of
inland waters and coastal seas in Europe. The model does not contain an assessment of ecological effects, but calculates the change in nutrient loads in ground water, inland waters (river catchments) and
coastal seas from changes in nutrient emissions and supplies (i.e. the causes).
Figure 6.1 Main sources for nitrogen (continuous arrow) and phosphorus (dashed arrow) to soil, groundwater, surface waters and coastal seas addressed in the CARMEN model (Beusen not published).
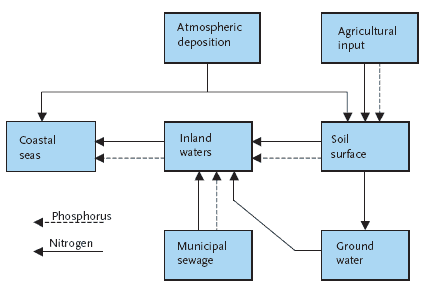
The eutrophication factors per country are calculated by changing the total amount of either nitrogen or phosphorus from a given source category in one country (other emissions for all countries and other
source categories remaining the same). Next, the increases in loading caused by this change of one country are accumulated over all river catchments and seas to obtain the factors which express what share
of the emission contributes to eutrophication of respectively inland waters and seas (in kg per kg released). For each source category, the change in eutrophying loads is calculated with a spatial resolution
over 101 river catchments and 32 coastal seas. In addition, the calculations for coastal seas also address atmospheric deposition as a nitrogen source. For atmospheric deposition, the CARMEN model
does not consider the relationships between country of origin and depositions to coastal seas. The model is therefore supplemented by data on the nitrogen deposited on European seas as a ratio of the
emission from their country of release.
A more detailed description of the CARMEN model and its use for calculation of site-dependent characterisation factors can be found in Potting et al., 2004a.
Site-generic exposure factors for Europe are given in Table 6.2, and Appendix 6.1 provides site-dependent exposure factors for 32 European regions relating nitrogen and phosphorus emissions by their
country of release to their eutrophication of inland waters and marine waters. The inland waters include all freshwater systems: lakes, streams, rivers and catchments while marine waters include the coastal
areas, brackish waters and open sea.
The site-dependent eutrophication factors express which share of an amount of nutrient released in a given country will contribute to eutrophication of
European inland waters and coastal seas. The CARMEN model does not include an assessment of the effects, i.e. whether this nutrient loading actually results in increased biomass growth and which effect
this has on the ecological quality of the water. The calculated factors thus represent the highest potential contribution to biomass growth (realistic worst case in the sense that removal of nutrients before the
reach the water has been taken into account). Compared to the EDIP2003 factors developed for terrestrial eutrophication and acidification, they thus cover a shorter part of the cause-impact chain and
should be seen as fate or exposure factors rather than as expressing an ecological effect in terms of eutrophication and biomass growth. The present state-of-the-art in integrated assessment modelling of
aquatic eutrophication does not allow such an effect assessment.
Being exposure factors, the EDIP2003 factors do not replace the EDIP97 factors which represent the relative content of nutrients in different compounds. Instead, they are to be used in combination with the
EDIP97 factors for separate characterisation of nitrogen compounds and phosphorus compounds. In the temperate and sub tropic regions of Europe, inland freshwaters are typically phosphorus limited while
marine waters typically are nitrogen limited (in the tropics, it may be the opposite). Respecting this distinction, inland waters and marine waters are treated as two sub-categories under the new impact
category aquatic eutrophication.
Application of both the EDIP2003 site-generic and the EDIP2003 site-dependent exposure factors in combination with the EDIP97 factors is straightforward (see Sections 6.5 and 6.6). The typical life
cycle inventories already provide the only additional information required for site-dependent assessment, namely the geographical region where the emission takes place.
The use of site-dependent exposure factors, however, adds only a moderate resolving power of at most a factor 7 difference between highest and lowest ratings. The moderate range found between the
highest and lowest site-dependent exposure factors justifies a certain reluctance in site-dependent assessment, considering that the modelling in itself also brings uncertainty into the site factors.
The main use of the site-dependent exposure factors for aquatic eutrophication is for sensitivity analysis.
What do the impacts express?
The EDIP2003 aquatic eutrophication potentials of a nutrient emission express the maximum exposure of aquatic systems that it can cause. In this respect they are similar to the EDIP97 nutrient enrichment
potential and they are also expressed in the same units, namely as N- or P-equivalents. However, compared to the EDIP97 impacts, a larger part of the fate of the substances is modelled, and the EDIP2003
impact potential thus represents
the fraction of the emission which can actually be expected to reach different aquatic systems. Where EDIP97 impacts represented a worst case eutrophication potential, the EDIP2003 eutrophication
potential can thus be seen as realistic worst case.
6.5 Proper inventory data
It is common practice in life cycle assessment to consider the topsoil of agricultural fields as part of the technosphere. The data in life cycle inventory for nutrient supply in agriculture therefore usually refer to
the amount of nutrient available for leaving the topsoil after plant uptake and binding. In case this figure is not known, Annex 6.3 provides factors which can be used to estimate the combined leaching and
run-off of nutrients from the agricultural soil if the applied quantity of fertiliser is known (i.e. before plant uptake and binding in the soil).
Similarly, the exposure factors of Table 6.2 and Annex 6.1 refer to the emission of nutrients with waste water, i.e. after the relevant waste water treatment. In case, the waste water treatment has not been
modelled as part of the inventory analysis, Potting et al., 2005a provides typical removal efficiencies for nutrients with different types of waste water treatment in Europe.
6.6 Site-generic characterisation
The moderate range between highest and lowest site-dependent exposure factors found in Annex 6.1 means that there is only little motivation for performing a full site-dependent exposure assessment for this
impact category since the additional resolution that is obtained is small.
There is, however, still good reason to perform site-generic characterisation using the new site-generic exposure factors from Table 6.2 in combination with the EDIP97 factors as described below. The
procedure is the same for both of the subcategories:
Inland waters and marine waters.
The site-generic aquatic eutrophication impact on inland waters and marine waters from a product system can be calculated according to the following formula:

(6.1)
Where: sg-EP(ae) = The site-generic aquatic eutrophying impact from an emission to inland waters or marine waters (in either N-equivalents or P-equivalents)
sg-AEEFs = The site-generic exposure factor from Table 6.2 that relates the emission of substance (s) by the given source category to the eutrophying impact on either inland waters or marine waters
CF(ne)s = The characterisation factor from Table 6.1 that allows aggregating substance (s) with other substances belonging to the same group (nitrogen or phosphorus compounds)
Es = The emission of substance (s) (in g/f.u)
Table 6.2. Factors for site-generic characterisation, and for site-dependent characterisation of eutrophication of inland waters and marine waters
Emission to inland
waters
|
Site-generic assessment
Site-
generic exposure
=
sg-AEEF(s)
|
Site-dependent assessment
Site-dependent exposure factors(factor
to be found in Annex 6.1) |
Substance/source category |
Factor |
(standarddeviation) |
factor = sd-AEEFi,s |
P-agricultural (*) |
0.06 |
(0.03) |
sd-AEEF|(P-agricultural) |
P-wastewater (**) |
0.88 |
(0.15) |
sd-AEEF |(P-wastewater) |
N-agricultural (*) |
0.53 |
(0.08) |
sd-AEEF |(N-agricultural) |
N-wastewater (**) |
0.59 |
(0.15) |
sd-AEEF |N-wastewater) |
Emission to marine waters |
Site-generic assessment |
Site-dependent assessment |
|
Site-generic exposure factors |
Site-dependent exposure factors |
Substance/source category |
= sg-AEEF(s) |
|
(factor to be found in Annex 6.1) |
|
Factor |
(standarddeviation) |
factor = sd-AEEF |(s) |
P-agricultural (*) |
0.06 |
(0.03) |
sd-AEEF |(P-agricultural) |
P-wastewater (**) |
1.00 |
|
sd-AEEF |(P-wastewater) |
N-agricultural (*) |
0.54 |
(0.08) |
sd-AEEF |(N-agricultural) |
N-wastewater (**) |
0.70 |
|
sd-AEEF |(N-wastewater) |
*These factors relate to nutrient emissions after plant uptake
**The factors for wastewater basically express what share is released directly to marine waters or indirectly through rivers in a European average
situation
The standard deviations given for each substance in Table 6.2 represent the spatial variation underlying the site-generic exposure factors.
6.7 Site-dependent characterisation
Given the moderate range between the highest and the lowest site-dependent exposure factors in Annex 6.1, there is, as stated earlier, only little motivation for performing a full site-dependent exposure
assessment for aquatic eutrophication. Instead, the site-dependent factors should be seen as an information for a sensitivity analysis and possibly also for reduction of the potential spatial variation in the
site-generic impact.
If site-dependent characterisation is performed, a three-step procedure has been developed. The aquatic eutrophying impact from a given product is in many cases determined by one or a few processes.
These are identified from a calculation of the site-generic impact as described in Section 6.6 (step 1), and, if wanted, the site-generic impact can be adjusted with the relevant site-dependent factors (step 2
and 3).
Step 1
The site-generic aquatic eutrophication impact from a product system, as calculated in the previous section, is broken down into the contributions from the separate processes. These contributions are then
ranked from the largest to the smallest contribution, and the process with the largest contribution is selected.
Step 2
The site-generic aquatic eutrophication impact from step 1 is reduced with the contribution of the process selected in step 1. Next, the site-dependent impact from the emissions of this process is calculated
with the relevant site-dependent aquatic exposure factors.

(6.2)
Where: sd-EP(ae)p = The site-dependent aquatic eutrophication impact from process (p) on inland waters or marine waters (in either N-equivalents or P-equivalents)
sd-AEEFs,i= The site-dependent exposure factor from Annex 6.1 that relates the emission of substance (s) by the relevant source category in country or region (i) where process
(p) takes place to the eutrophying impact on either inland waters or marine waters. Emissions from an unknown region or from non-European regions can as a first approach be represented by the
site-generic factors.
CF(ne)s = The characterisation factor from Table 6.1 that allows aggregating substance (s) with other substances belonging to the same group (nitrogen or phosphorus compounds)
Es,p = The emission of substance (s) from the selected process (p) (in g/f.u).
The exposure factors in Annex 6.1 for wastewater cover situations where the country of emission is known. As regards the distribution of waste water emissions between inland waters and marine waters,
the exposure factors of Annex 6.1 reflect the average situation in the given country. If an emission of nutrients is known to be released fully to inland waters, the factor should be
0.7 for nitrogen and 1.0 for phosphorus instead of the value found in Annex
6.1. If the full emission is to marine waters, the factors for inland waters should be 0 for both nitrogen and phosphorus.
The geographic region in which the emissions take place determines the relevant factors. As a first approach, emissions from a non-European or unknown region can be characterised using the site-generic
factors from Table 6.2. The standard deviations for these factors in Table 6.2 give a range for spatial variation covered by the site-generic factor within Europe. Given the size of the variation in emissions and
sensitivities within Europe, the site-dependent factor is expected to lie within this range for most regions also in the rest of the world. Expert judgement may be used in the interpretation to assess whether the
factor for emissions from processes in non-European regions should be found in the lower or upper end of the range.
Step 3
The site-dependent contributions from the process selected in step 1 are added to the adjusted site-generic contribution from step 2. Step 2 is repeated until the site-dependent contribution of the selected
processes is so large that the spatial variation can no longer influence the conclusion of the study (e.g. when the site-dependent share is larger than 95% of the total contribution).
6.8 Normalisation
The EDIP2003 person equivalents for aquatic eutrophication using the EDIP2003 exposure factors are 12 kg N-eq/person/year and 0.41 kg P-eq/person/year or in aggregated form 58 kg NO3
--eq/person/year.
Following the EDIP97 approach, the normalisation reference for aquatic eutrophication is based on the impact caused by the actual emission levels for 1995 (see Hauschild and Wenzel 1998d and
Stranddorf et al., 2005). Applying the EDIP2003 exposure factors for aquatic eutrophication together with the characterisation factors from EDIP97, the total impact from the European emissions is 4467 kt
N-eq/year and 151 kt P-eq/year or in aggregated form 21467 kt NO3--eq/year The person equivalent is calculated as an average European impact per person assuming a total European population of
3.70?108 persons. The calculation of the normalisation reference is documented in Annex 6.4.
6.9 Interpretation
Considering the moderate range found between the highest and lowest site-dependent exposure factors the main interest of the established site-dependent exposure factors lies in their use for representing
this part of spatial variation in a sensitivity analysis.
The exposure factors express the share of the emission that will contribute to eutrophication of respectively inland waters and seas (in kg per kg released). Combined with the EDIP97 or similar
characterisation factors for aquatic eutrophication, the exposure factors indicate the amount of phytoplankton that at maximum can be produced from the nutrient emission. Compared to the factors
developed for terrestrial eutrophication and acidification, the factors for aquatic eutrophication cover a shorter part of the cause-impact chain and are thus fate or exposure factors rather than factors which
express the ecological effect in terms of eutrophication and biomass growth.
Inland waters are typically phosphorus limited and marine waters mostly nitrogen limited. This should be reflected in the interpretation of the assessed eutrophying impact. The present state-of-the-art in
integrated assessment modelling of aquatic eutrophication does not allow a closer assessment of ecological effect. Annex 6.2 contains an overview of the ecological quality of rivers in a number of countries.
This overview may be used for a qualitative evaluation.
In Potting et al., 2005a the site-dependent exposure factors are also within the countries spatially resolved over a total of 101 river catchments and 42 marine waters. This information can be used, if desired,
for a qualitative effect evaluation in the interpretation.
6.10 Example
Applying the EDIP2003 factors, characterisation is performed on the inventory presented in Section 1.6.
Site-generic characterisation
As described in Section 6.6, first the site-generic impacts are calculated. The aquatic eutrophication impact shown in Table 6.3 is determined using the EDIP97 factors from Table 6.1 and the site-generic
exposure factors from Table 6.2.
Table 6.3. Site-generic aquatic eutrophication impacts expressed as N- and P-equivalents for one supporting block made from plastic or zinc.
Click here to see the Table
Using site-generic characterisation factors, the zinc supporting block has the largest N-equivalent while only the plastic block has any contributions to aquatic eutrophication with P. For both components the
aquatic nutrient enrichment is predominantly caused by NOx-emissions deposited to marine areas, while the contribution from the waterborne emissions is negligible. For the site-generic impacts, the potential
spatial variation is so large (as revealed by the spatially determined standard deviation) that the conclusion might change if spatial variation were to be included.
Therefore, a site-dependent characterisation is performed for those processes that contribute the most to the site-generic aquatic eutrophication impacts with N in order to reduce the spatially determined
uncertainty and strengthen the conclusion.
Site-dependent characterisation
Table 6.3 shows that the predominant contributions to the site-generic aquatic eutrophication impact are caused by the emissions of NOx to air. The contributions from emissions of NH3 to air and
NO3—N and NH4+-N to water are negligible in the overall impact. For the zinc component, the main sources for NOx emission are identified as the production of zinc from ore which takes place in
Bulgaria, the casting of the component which takes place in Yugoslavia and that part of the transport of the component, which takes place by truck through Germany (data not shown). For the plastic
component the main sources for NOx are found to be the production of plastic polymer in Italy, the flow injection moulding of the supporting block in Denmark and the transportation of the component by
truck, mainly through Germany (idem). The emissions from these processes contribute 99% and 75% of the full site-generic impacts of Table 6.3 for the zinc component and the plastic component
respectively (data not shown).
In the calculation of the site-dependent impacts for these key processes, the relevant site-dependent factors from Annex 6.1 are applied. The results are shown in Table 6.4.
Table 6.4. Site-dependent aquatic eutrophication impacts for key processes from either product system.
Zinc part |
Emissiong/f.u. |
Characterisation
factor,
Table 6.1g N-eq./g |
Aq. eutr. factor,
Annex 6.1g/g |
Impact sd-EP(ae)g N-eq/f.u. |
NOx emissions |
|
|
|
|
Zinc production, Bulgaria |
0.97 |
0.3 |
0.31 |
0.09 |
Zinc casting, Yugoslavia |
1.65 |
0.3 |
0.19 |
0.09 |
Transport,mainly Germany |
4.56 |
0.3 |
0.23 |
0.31 |
Total. zinc part |
|
|
|
0.5 |
Plastic part |
Emission |
Characterisation factor, Table 6.1 |
Aq. eutr. factor, Annex 6.1 |
Impactsd-EP(ae) |
|
g/f.u. |
g N-eq./g |
g/g |
g N-eq/f.u. |
NOx emissions |
|
|
|
|
Plastic production, Italy |
0.63 |
0.3 |
0.40 |
0.08 |
Flow injection moulding, Denmark |
0.48 |
0.3 |
0.41 |
0.06 |
Transport,mainly Germany |
1.74 |
0.3 |
0.23 |
0.12 |
|
|
|
|
|
Total, plastic part |
|
|
|
0.3 |
The site-generic impacts from the key processes are subtracted from the original site-generic impacts in Table 6.3 and the site-dependent impacts of Table 6.4 are added. The thus corrected aquatic
eutrophication impacts are found in Table 6.5 and the difference to the original site-generic impacts of Table 6.3 is illustrated in Figure 6.2.
Site-dependent characterisation reduces the size of the aquatic eutrophication impact with N slightly and reduces the dominance of the zinc component. For the zinc-based component, around 99% of this
impact is calculated using site-dependent characterisation factors, while the site-dependent share for the plastic-based component is around 75%. Even if the site-dependent characterisation was performed
for all the remaining processes in the product system, it is not likely that the result would change significantly, given their modest share in the total and the standard deviation. The spatially conditioned potential
for variation of the impact has largely been cancelled for both components.
Table 6.5. Aquatic eutrophication impacts as N-equivalents from either product system with site-dependent characterisation of key process emissions
| Aquatic eutrophication,
sd-EP(ae)
g N-eq./f.u |
Zinc component | 0.50 |
Plastic component | 0.35 |
Figure 6.2 Site-generic and site-dependent aquatic eutrophication impacts with N from the two product systems. For the site-dependent impacts, the site-dependent exposure factors have only been
applied for the key processes as described above.
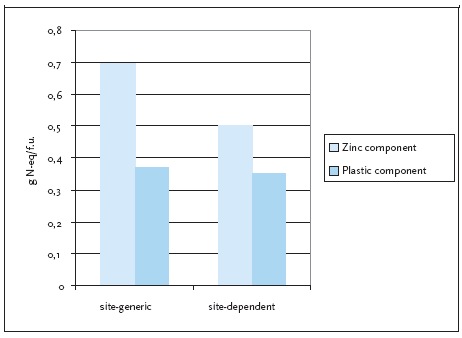
Annex 6.1: Site-dependent exposure factors for eutrophication of inland and marine waters
| Inlandwaters |
Marinewaters |
|
|
Nitrogen |
Phosphorus |
Nitrogen |
Phosphorus |
|
Agri |
WW |
Agri |
WW |
Agri |
WW |
NH3 |
NO2 |
Agri |
WW |
Albania |
0.53 |
0.57 |
0.1 |
0.81 |
0.53 |
0.7 |
0.29 |
0.32 |
0.1 |
1 |
Austria |
0.6 |
0.7 |
0.15 |
1 |
0.6 |
0.7 |
0.06 |
0.18 |
0.15 |
0.98 |
Baltic countries |
0.51 |
0.63 |
0.05 |
0.9 |
0.52 |
0.71 |
0.19 |
0.2 |
0.05 |
1 |
Belarus |
0.45 |
0.7 |
0.04 |
1 |
0.45 |
0.71 |
|
|
0.04 |
1 |
Belgium & Luxemburg |
0.56 |
0.66 |
0.05 |
0.94 |
0.58 |
0.7 |
0.19 |
0.27 |
0.06 |
1 |
Bulgaria |
0.56 |
0.7 |
0.03 |
0.99 |
0.55 |
0.7 |
0.13 |
0.31 |
0.03 |
1 |
Caucasus |
0.53 |
0.59 |
0.06 |
0.88 |
0.54 |
0.7 |
|
|
0.06 |
1 |
Czechia& Slovakia |
0.64 |
0.7 |
0.07 |
1 |
0.64 |
0.7 |
0.07 |
0.16 |
0.06 |
0.99 |
Denmark |
0.34 |
0.35 |
0.02 |
0.48 |
0.44 |
0.7 |
0.45 |
0.41 |
0.03 |
1 |
Finland |
0.57 |
0.46 |
0.04 |
0.64 |
0.64 |
0.71 |
0.29 |
0.32 |
0.04 |
1 |
France |
0.57 |
0.65 |
0.06 |
0.93 |
0.59 |
0.7 |
0.28 |
0.34 |
0.06 |
1 |
Germany, east |
0.53 |
0.66 |
0.03 |
0.94 |
0.55 |
0.7 |
0.16 |
0.23 |
0.03 |
1 |
Germany, west |
0.52 |
0.68 |
0.06 |
0.97 |
0.53 |
0.71 |
0.16 |
0.25 |
0.06 |
1 |
Greece |
0.51 |
0.42 |
0.04 |
0.63 |
0.51 |
0.7 |
0.38 |
0.55 |
0.04 |
1 |
Hungary |
0.5 |
0.7 |
0.03 |
1 |
0.51 |
0.69 |
0.07 |
0.16 |
0.02 |
0.99 |
Iceland |
0.64 |
0.59 |
0.09 |
0.88 |
0.64 |
0.7 |
|
|
0.09 |
1 |
Ireland |
0.62 |
0.64 |
0.13 |
0.91 |
0.62 |
0.7 |
0.51 |
0.69 |
0.13 |
1 |
Italy |
0.52 |
0.55 |
0.06 |
0.79 |
0.52 |
0.7 |
0.29 |
0.4 |
0.06 |
1 |
Moldavia |
0.5 |
0.7 |
0.02 |
1 |
0.51 |
0.68 |
0.1 |
0.2 |
0.02 |
0.98 |
the Netherlands |
0.26 |
0.31 |
0.03 |
0.37 |
0.36 |
0.72 |
0.27 |
0.38 |
0.03 |
1 |
Norway |
0.56 |
0.5 |
0.08 |
0.71 |
0.64 |
0.71 |
0.52 |
0.51 |
0.09 |
1 |
Poland |
0.47 |
0.69 |
0.03 |
0.98 |
0.47 |
0.7 |
0.11 |
0.18 |
0.03 |
1 |
Portugal |
0.62 |
0.52 |
0.06 |
0.75 |
0.62 |
0.7 |
0.37 |
0.44 |
0.06 |
1 |
Rumania |
0.57 |
0.7 |
0.04 |
1 |
0.57 |
0.7 |
0.08 |
0.18 |
0.04 |
1 |
Russia |
0.55 |
0.6 |
0.04 |
0.86 |
0.55 |
0.7 |
0.18 |
0.38 |
0.04 |
1 |
Spain |
0.61 |
0.6 |
0.03 |
0.86 |
0.61 |
0.7 |
0.25 |
0.41 |
0.03 |
1 |
Sweden |
0.52 |
0.56 |
0.04 |
0.83 |
0.59 |
0.71 |
0.37 |
0.38 |
0.04 |
1 |
Switzerland |
0.63 |
0.7 |
0.12 |
1 |
0.65 |
0.7 |
0.06 |
0.19 |
0.12 |
1 |
Turkey |
0.53 |
0.59 |
0.06 |
0.88 |
0.54 |
0.7 |
|
|
0.06 |
1 |
Ukraine |
0.49 |
0.68 |
0.03 |
0.97 |
0.5 |
0.7 |
0.11 |
0.17 |
0.03 |
1 |
United Kingdom |
0.53 |
0.58 |
0.08 |
0.84 |
0.6 |
0.71 |
0.48 |
0.57 |
0.09 |
1 |
Yugoslavia |
0.59 |
0.69 |
0.09 |
0.99 |
0.59 |
0.69 |
0.08 |
0.19 |
0.09 |
0.98 |
Mean |
0.53 |
0.59 |
0.06 |
0.88 |
0.54 |
0.7 |
0.23 |
0.32 |
0.06 |
1 |
Standard deviation |
0.08 |
0.15 |
0.03 |
0.15 |
0.08 |
|
0.15 |
0.14 |
0.03 |
|
Minimum |
0.26 |
0.05 |
0.02 |
0.37 |
|
|
0.06 |
0.16 |
|
|
Maximum |
0.64 |
0.07 |
0.15 |
1 |
0.65 |
|
0.52 |
0.69 |
0.15 |
|
Annex 6.2: Percentage of river reaches in various European countries classified as being of good, fair, poor or bad quality River reaches of good quality have nutrient-poor water, low levels of
organic matter, saturated with dissolved oxygen, rich invertebrate fauna, and suitable spawning ground for salmonid fish. River reaches with moderate organic pollution and nutrient content, good oxygen
conditions, rich flora and fauna, large fish population are classified as fair. Poor quality river reaches have heavy organic pollution, usually low oxygen concentrations, locally anaerobic sediment, occasional
mass occurrence of organisms insensitive to oxygen depletion, small or absent fish population, periodic fish kill. Of bad quality are those rivers with excessive organic pollution, prolonged periods of very low
oxygen concentration or total deoxygenation, anaerobic sediment, severe toxic input, devoid of fish (Kristensen and Hansen 1994). It should be noted that The inventory given in the table is based on very
different numbers of rivers and river stations in each country, and the classification is based on the specific countries own measurements, which have not been intercalibrated.
Country |
Good |
Fair |
Poor |
Bad |
Austria (1991) |
14 |
82 |
3 |
1 |
Belgian Flandern (1989-1990) |
17 |
31 |
15 |
37 |
Bulgaria (1991) |
25 |
33 |
31 |
11 |
Croatia |
15 |
60 |
15 |
10 |
Czech republic |
12 |
33 |
27 |
28 |
Denmark (1989-1991) |
4 |
49 |
35 |
12 |
England/Wales (1990) |
64 |
25 |
9 |
2 |
Finland (1989-1990) |
45 |
52 |
3 |
0 |
Former west Germany (1995) |
44 |
40 |
14 |
2 |
Iceland |
99 |
1 |
0 |
0 |
Ireland (1987-1990) |
77 |
12 |
10 |
1 |
Italy |
27 |
31 |
34 |
8 |
Latvia |
10 |
70 |
15 |
5 |
Lithuania |
2 |
97 |
1 |
0 |
Luxemburg |
53 |
19 |
17 |
11 |
The Netherlands |
5 |
50 |
40 |
5 |
Northen Ireland (1990) |
72 |
24 |
4 |
0 |
Poland |
10 |
33 |
29 |
28 |
Romania |
31 |
40 |
24 |
5 |
Russian Federation |
6 |
87 |
5 |
2 |
Scotland (1990) |
97 |
2 |
1 |
0 |
Slovenia (1990) | 12 | 60 | 27 | 1 |
Annex 6.3: Leaching and surface run off of nutrients from soil
The factors are used for estimation of combined leaching and surface run off of nutrients from agricultural soil to surface waters after plant uptake and binding (the exposure factors of Table 6.1 relate to this
kind of inventory information). To be applied in the case where inventory information regards the quantity of nutrients applied rather than the emission from the soil.
|
Nitrogen after plant uptake andbinding
(in kg/kg applied) |
Phosphorus after plant uptake
(in kg/kg applied) |
|
Grassland<100 kg Nappl./ha |
Grassland>100 kg Nappl./ha |
Arable &Natural land |
All land types |
Sand |
0.00 |
0.15 |
0.25 |
0.10 |
Loam |
0.00 |
0.10 |
0.18 |
0.10 |
Clay |
0.00 |
0.05 |
0.10 |
0.10 |
Peat |
0.00 |
0.01 |
0.05 |
0.10 |
Annex 6.4: Normalisation reference for aquatic eutrophication
Based on national emission inventories of nutrient emissions for a number of European countries provided by Larsen, 2005a, European normalisation reference is calculated for aquatic eutrophication
applying the EDIP2003 exposure factors and the EDIP97 characterisation factors according to Equation 6.2 for site-dependent aquatic eutrophication impact:

A number of assumptions have been made:
1. For the EU15 countries, the inventories of waterborne emissions of N and P report the quantity from each country which annually reaches the sea (Baltic, North Sea/Atlantic ocean or Mediterranean).
There is thus no need for application of an exposure factor since the removal, which occurs between emission from agriculture or waste water treatment plant and the sea, has already occurred.
2. A correction is performed to assess the man-made share of the total nutrient load reaching the sea. Danish results show that 85-90% of the waterborne emissions of nitrogen and phosphorus are
man-made. Assuming that these figures are valid for Europe, the total emission inventories are multiplied by a factor 0.88 to arrive at the total annual man-made emissions reaching the seas around Europe.
3. For the national emission inventories of the air-borne nutrient emissions of NOx and NH3, the relevant national AEEFs are found in Annex 6.1 and applied together with the nutrient enrichment
characterisation factors from EDIP97.
The calculation of the aquatic eutrophication normalisation reference is shown in the table below.
The normalisation references are calculated by dividing the total EU-15 impacts for 1994 by the total population in the EU15 countries in 1994:
Aquatic eutrophication normalisation
references |
Nutrient |
EU15 emission1994 |
EU15 populationMillion persons in 1994 |
Normalisation reference1994 |
Nitrogen |
4467 kt N-eq./year |
|
12 |
|
|
370 |
kg N-eq./person/year |
Phosphorus |
151 kt P-eq./year |
|
0.41 |
|
|
|
kg P-eq./person/year |
N and P |
21467 kt NO3- |
|
58 |
|
-eq./person/year |
|
kg NO3--eq./person/year |
Country |
Population |
Riverine discharges |
|
|
Total N |
Total P |
Anthropogenic N |
Anthropogenic P |
|
(Mio) |
(kt/year) |
(kt/year) |
(kt/year) |
(kt/year) |
Germany |
81.1 |
376.4 |
13.1 |
331.2 |
11.5 |
United Kingdom |
58.2 |
376 |
36 |
331 |
32 |
France |
57.7 |
185 |
8 |
163 |
7 |
Italy |
57 |
346 |
29 |
304 |
26 |
Spain |
39.1 |
185 |
11 |
163 |
9.7 |
Netherlands |
15.3 |
490 |
27.5 |
431 |
24.2 |
Greece |
10.4 |
117 |
7 |
103 |
6.2 |
Belgium |
10.1 |
47 |
2 |
41 |
1.8 |
Portugal |
9.9 |
15.7 |
14.2 |
13.8 |
12.5 |
Sweden |
8.7 |
137.8 |
5 |
121.3 |
4.4 |
Austria |
8 |
|
|
0 |
0 |
Denmark |
5.2 |
140.6 |
4.5 |
123.7 |
4.0 |
Finland |
5.1 |
66.1 |
3.6 |
58.2 |
3.2 |
Ireland |
3.6 |
179.1 |
10.5 |
157.6 |
9.2 |
Luxemburg |
0.4 |
|
|
0 |
0 |
EU-15 |
369.8 |
2661.7 |
171.4 |
2342.3 |
150.8 |
Country |
Population |
Airborne emissions |
|
|
NOx |
AEEF for N0x to air |
NH3 |
AEEF for NH3 to air |
|
(Mio) |
(kt/Year) |
(g/g) |
(kt/Year) |
(g/g) |
Germany |
81.1 |
2266 |
0.24 |
623 |
0.16 |
United Kingdom |
58.2 |
2387 |
0.57 |
320 |
0.48 |
France |
57.7 |
1682 |
0.35 |
667 |
0.28 |
Italy |
57 |
2157 |
0.4 |
389 |
0.29 |
Spain |
39.1 |
1223 |
|
344 |
|
Netherlands |
15.3 |
530 |
0.38 |
172 |
0.27 |
Greece |
10.4 |
357 |
0.55 |
445 |
0.38 |
Belgium |
10.1 |
374 |
0.27 |
79 |
0.19 |
Portugal |
9.9 |
249 |
0.44 |
93 |
0.37 |
Sweden |
8.7 |
444 |
0.38 |
51 |
0.37 |
Austria |
8 |
171 |
0.18 |
86 |
0.06 |
Denmark |
5.2 |
276 |
0.41 |
94 |
0.45 |
Finland |
5.1 |
288 |
0.32 |
41 |
0.29 |
Ireland |
3.6 |
117 |
0.69 |
125 |
0.51 |
Luxemburg |
0.4 |
23 |
0.27 |
7 |
0.19 |
EU-15 |
369.8 |
12544 |
|
3536 |
|
Country |
Population |
Site-dependent aquatic eutrophication potential |
|
(Mio) |
kt N-eq./year |
kt P-eq./year |
kt NO3--eq. /year |
Germany |
81.1 |
576.1 |
11.5 |
2684.84 |
United Kingdom |
58.2 |
865 |
32 |
4192.75 |
France |
57.7 |
492.6 |
7. |
2269.32 |
Italy |
57 |
655.8 |
26 |
3190.94 |
Spain |
39.1 |
162.8 |
9.7 |
822.36 |
Netherlands |
15.3 |
530 |
24.2 |
2604 |
Greece |
10.4 |
301 |
6.2 |
1401 |
Belgium |
10.1 |
84 |
1.8 |
393 |
Portugal |
9.9 |
75 |
12.5 |
465 |
Sweden |
8.7 |
187 |
4.4 |
880 |
Austria |
8 |
13 |
0 |
60 |
Denmark |
5.2 |
192 |
4.0 |
896 |
Finland |
5.1 |
96 |
3.2 |
458 |
Ireland |
3.6 |
234 |
9.2 |
1136 |
Luxemburg |
0.4 |
3 |
0 |
13 |
EU-15 |
369.8 |
4467 |
151 |
21467 |
Footnotes
3 CARMEN is an acronym for CAuse effect Relation Model to support Environmental Negotiations. It is an integrated assessment model to analyse and evaluate strategies to reduce nutrient loading of
inland waters and coastal seas in Europe. The model does not contain an assessment of ecological effects, but calculates the change in nutrient loads in ground water, inland waters (river catchments) and
coastal seas from changes in nutrient emissions and supplies (i.e. the causes).
7. Photochemical ozone formation
Background information for this chapter can be found in:
- Chapter 3 of the "Environmental assessment of products. Volume 2: Scientific background" by Hauschild and Wenzel (1998a).
- Chapter 6 of the "Background for spatial differentiation in life cycle impact assessment – EDIP2003 methodology" by Potting and Hauschild (2005).
7.1 Introduction
When solvents and other volatile organic compounds are released to the atmosphere, most of them are degraded within a few days to weeks. Initiated by sunlight, nitrogen oxides (NOx) and volatile organic
compounds (VOCs) react to form ozone. The nitrogen oxides are not consumed during the ozone formation, but have a catalyst-like function. Depending on the nature of the VOCs, the reactions may take
hours or days. Since the process is initiated by sunlight, it is called photochemical ozone formation. It takes place in the troposphere, the lower layers of the atmosphere, where it is the primary source of
ozone.
The formed ozone is an unstable gas but nevertheless, it has a half-life of a few weeks in the troposphere. This does mean, however, that the ozone formed in the troposphere cannot rise to the stratosphere
and remedy the thinning of the ozone layer there. In the troposphere, it is widely dispersed, and ozone measured at a particular location may have arisen from VOC and NOx emissions many hundreds of
kilometres away. The ozone concentration in the troposphere rises by about 1% a year over most of the northern hemisphere, where the largest emissions of VOC and NOx occur. Over the southern
hemisphere, the ozone concentration in the troposphere is practically constant.
Due to its high reactivity, ozone attacks organic substances present in plants and animals or materials exposed to air. This leads to an increased frequency of humans with problems in the respiratory tract
during periods of photochemical smog in cities, and the steadily increasing tropospheric concentration of ozone causes a reduced agricultural yield. For Denmark, the loss is estimated to cost about 10% of
the total agricultural production.
7.2 Classification
The substances contributing to photochemical ozone formation are:
- volatile organic compounds (VOC)
- nitrogen oxides (NOx)
- carbon moNOxide (CO)
- methane (CH4)
Volatile organic compounds
Applying the definition of EDIP97, a volatile organic compound is defined as
an organic compound with a boiling point below 250° C. In addition to being volatile, the compound must contain hydrogen or double bonds between carbon atoms to be able to undergo oxidation
with ozone formation. Due to the exceptionally long lifetime of methane (CH4) and consequent low ozone formation potential, a distinction is often made between this compound and the rest of the VOCs
which are sometimes referred to as NMVOCs (non-methane VOCs). If nothing else is specified, in this Guideline, VOCs should be taken as non-methane VOCs. VOCs may be reported in life cycle
inventories as individual substances or as mixtures. The main sources of VOC emissions are combustion processes and use of organic solvents.
Nitrogen oxides
NOx designates the sum of NO and NO2. The two oxides are easily interconverted through oxidation or reduction and their relative prominence depends on the redox conditions of the surrounding air.
Therefore, they are usually reported as the sum; NOx. The main source of NOx is combustion processes where it is formed from atmospheric nitrogen N2and oxygen O2.
Carbon moNOxide
Even though it is not an organic compound, CO also contributes to photochemical ozone formation. The main source of CO is incomplete combustion.
Methane
The contribution of CH4 to ozone formation is important at the global scale rather than the regional scale, due to its long life time in the troposphere, and methane is considered an important greenhouse gas.
The main man-made sources of methane are combustion processes and biogenic sources like rice paddies and the digestive systems of livestock.
7.3 EDIP97 characterisation factors
Most current life cycle impact assessment methodologies apply photochemical ozone creation potentials, POCPs, to characterise the photochemical ozone formation potential of VOCs. The POCP factors
applied in EDIP97 express the potential for formation of ozone during the first 4-9 days after emission at standard concentrations of hydroxyl radical and NOx and at typical atmospheric conditions. The
POCP factors are found in Table 23.3 and 23.4 in Wenzel et al., 1997. The ozone formation potential of a substance is expressed relative to that of ethylene (C2H4) which is used as a reference compound.
As discussed in Hauschild and Wenzel, 1998e, ozone formation is strongly dependent on local conditions like the simultaneous presence of other VOCs and NOx, and the solar radiation intensity, all of
which may vary strongly from location to location. This is the reason why a preliminary spatial differentiation was introduced in EDIP97 through the distinction between emissions occurring in regions with low
and high background levels of NOx.
The definition of the POCP factors excludes the possibility of representing the contribution of NOx to photochemical ozone formation. This means that only the contribution from VOCs is considered. As
mentioned already in the documentation of EDIP97, this is quite unfortunate since NOx may in some cases be the main contributor to the formation of photochemical ozone. Nonetheless the contribution
from NOx can not be represented using the EDIP97 methodology.
7.4 EDIP2003 characterisation factors
The inability to give a satisfactory representation of the spatial variability of the ozone formation is the main motivation behind developing the new spatial characterisation factors which allow a much higher
degree of spatial differentiation revealing rather large differences which are averaged out in the EDIP97 approach.
In addition, the EDIP2003 factors hold the following advantages over the POCP approach employed in EDIP97:
- the resulting impact potential is more straightforward to interpret in terms of environmental damage since it is modelled further along the impact chain to include exposure of human beings and vegetation
instead of just predicting the potential formation of ozone
- the dependence on surrounding conditions means that the potential for ozone formation must be expected to vary from year to year. The EDIP2003 factors are calculated for the emission levels of three
different years (1990, 1995 and predicted emission levels for 2010) for average meteorological conditions which allows judging their sensitivity to this temporal variation. Only the factors for 1995 are shown
here – the others can be found in Hauschild et al., 2005.
The EDIP2003 characterisation factors for photochemical ozone formation have been developed using the RAINS model which was also used for development of characterisation factors for acidification
and terrestrial eutrophication. Site-generic factors have been established (see Table 7.1), as well as site-dependent factors for 41 European countries or regions (see Annex 7.1 to this chapter). The
photochemical ozone formation factors relate an emission by its region of release to the ozone exposure and impact on vegetation or human beings within its deposition areas. The principles of the RAINS
model are described in Section 4.4. It was originally developed for modelling of acidification (N- and S-compounds) and air-borne eutrophication (N-compounds) but it is intended to support the
development of cost-effective European abatement strategies for different types of air pollution and has therefore recently been expanded to include the precursors of photochemical ozone formation (NOx
and VOCs). For the modelling of ozone formation, RAINS applies a meta-model which has been statistically derived from a mechanistic model of the highly complex reaction schemes behind the formation
of ozone and other photo-oxidants.
Such highly complex models are used for calculation of the POCPs which are used as characterisation factors in EDIP97 but they will not be feasible in an integrated assessment model where
source-receptor relationships must also be modelled well. Instead, RAINS builds on a computationally efficient `reduced-form' model of ozone formation which acts as a meta-model based on the complex
mechanistic model, using statistical regression methods to summarise the behaviour of the a more complex model.
The ozone formation is influenced by the presence of other VOCs as determined by the concomitant emission patterns of the European countries. The factors may therefore vary in time and in order to reveal
temporal variation, they are calculated for the registered or projected emissions of three reference years 1990, 1995 and 2010. The factors based on the 1995 emissions are chosen as the default EDIP2003
characterisation factors but the factors for the other years are given in Hauschild et al., 2005 to allow checking the temporal sensitivity of the factors and, if wanted, to allow temporal differentiation for those
emissions of the product system, which will take place in the future (e.g. from the late use stage of long-lived products or from the disposal stage). The site-generic factors only show minor temporal
variations but for some countries, the change in the site-dependent factors may be considerable over time, for exposure of humans.
The ozone formation is also influenced by the meteorological conditions which may fluctuate from year to year. To reduce the influence of annual variations in meteorological conditions, the characterisation
factors for each of the emission years 1990, 1995 and 2010 are derived as the average of five different calculations using the meteorological data for the years 1989, 1990, 1992, 1993 and 1994
respectively.
Due to its long life time, the contribution of methane to ozone creation is rather low on a regional level. This is why it is not included in the RAINS model, which has been adapted for calculating the
EDIP2003 characterisation factors. Instead, it is suggested to base the characterisation factors for methane on the site-generic factors developed for VOCs and correct for the fact that due to the long
lifetime of methane, a large part of the ozone formed will expose ocean areas and hence not contribute to exposure of vegetation or humans. A correction factor of 0.5 is proposed.
Human health and ecosystem health are the LCA protection areas which can be influenced by the photochemical ozone formation. Human beings and vegetation show clear differences in their sensitivity and
thresholds to ozone exposure, and the exposure of humans and vegetation is therefore modelled separately. The damage to materials caused by ozone is not modelled explicitly but it is taken to be reflected
by the exposure of humans since the geographical distribution of man-made materials will follow the distribution of humans.
As part of the new methodology for characterisation of photochemical ozone formation, the impact category is thus divided into two subcategories which represent the exposure of human beings and
materials, and the exposure of vegetation above their respective thresholds. For each of these two subcategories, an impact potential is calculated.
The impact potential for vegetation exposure is expressed as the AOT40, the product of the area of vegetation exposed above the threshold of chronic effects, 40 ppb (m2), the annual duration of the
exposure above the threshold (hours), and the exceeding of the threshold concentration (ppb). The unit of the impact potential for vegetation is m2?ppm?hours. The impact potential for human exposure is
expressed as the AOT60, the product of the number of persons exposed above the threshold of chronic effects, 60 ppb (pers), the annual duration of the exposure above the threshold (hours), and the
exceeding of the threshold concentration (ppb). The unit of the impact potential for human exposure is pers?ppm?hours.
What do the impacts express?
The site-generic and the site-dependent EDIP2003 photochemical ozone formation potentials of an emission are expressed in the same units. For vegetation, the impact is expressed as the AOT40, the
accumulated exposure (duration times exceedance of threshold) above the threshold of 40 ppb times the area that is exposed as a consequence of the emission. The threshold of 40 ppb is chosen as an
exposure level below which no or only small effects occur. The unit for vegetation exposure is m2?ppm?hours. For humans the impact is expressed as the AOT60, the accumulated exposure above the
threshold of 60 ppb times the number of persons which are exposed as a consequence of the emission. No threshold for chronic exposure of humans to ozone has been established. Instead, the threshold of
60 ppb is chosen as the long-term environmental objective for the EU ozone strategy proposed by the World Health Organisation, WHO. The unit for human exposure is pers?ppm?hours.
In comparison, the EDIP97 photochemical ozone formation potential is expressed as the emission of C2H4 that would lead to the same potential formation of ozone in the environment.
7.5 Site-generic characterisation
The site-generic characterisation factors have been developed as emission-weighted European averages of the site-dependent.
The site-generic photochemical ozone formation impacts of a product can be calculated according to the following formulas:
Click here to see the Formula
(7.1)
Where:
sg EP(po,veg) is the site-generic photochemical ozone formation impact on vegetation expressed as area exposed above threshold (in m2?ppm•hours/f.u.)
sg EP(po,hum) is the site-generic photochemical ozone formation impact on human health expressed as persons exposed above threshold (in pers?ppm?hours/f.u.)
sg CF(po,veg)VOC is the site-generic photochemical ozone formation factor from Table 7.1 that relates emission of VOCs or CO to the impact on vegetation in the deposition area (in m2•ppm•hours/g).
sg CF(po,veg)NOx is the site-generic photochemical ozone formation factor for from Table 7.1 that relates emission of NOx to the impacts on vegetation in the deposition area
(in m2•ppm•hours/g).
sg CF(po,hum)VOC is the site-generic photochemical ozone formation factor from Table 7.1 that relates emission of VOCs or CO to the impacts on human health in the deposition area (in
pers•ppm•hours/g).
sg CF(po,hum)NOx is the site-generic photochemical ozone formation factor from Table 7.1 that relates emission of NOx to the impacts on human health in the deposition area
(in pers•ppm•hours/g).
sg CF(po,veg)CH4 is the site-generic photochemical ozone formation factor for from Table 7.1 that relates emission of CH4 to the impacts on vegetation in the deposition area
(in m2•ppm•hours/g).
sg CF(po,hum)CH4 is the site-generic photochemical ozone formation factor from Table 7.1 that relates emission of CH4 to the impacts on human health in the deposition area
(in pers•ppm•hours/g).
ηi is a substance-specific efficiency factor from Annex 7.2 expressing the ozone creation potential of the individual volatile organic compound or CO (s) relative to the ozone creation potential of the
European average VOC (dimensionless).
Ei is the emission of NOx, CH4 or individual or source-specified VOC or CO (s) according to index (in g/f.u.)
For each of the two sub categories, the procedure for calculating the site-generic impact potential is:
- multiply the NOx emission by the relevant site-generic characterisation factor for NOx from Table 7.1
- multiply the emissions of individual VOCs, source-specified VOC mixtures or CO by their efficiency factor from Annex 7.2 and add them to the emissions of unspecified VOCs to get the sum-VOC
emission
- multiply the sum-VOC emission by the site-generic characterisation factor for VOCs and CO from Table 7.1
- multiply the CH4 emission by the site-generic characterisation factor for CH4 from Table 7.1
- Sum the impact potentials thus calculated for NOx, VOC, CO and CH4 to get the impact potential for each of the two sub categories.
The spatially determined variation which potentially lies hidden in the site-generic photochemical ozone impacts, can be estimated from the standard deviation given in Table 7.1 for each substance.
7.6 Site-dependent characterisation
The photochemical ozone formation impact from a product is often dominated by a few processes. To avoid unnecessary work, applications where a site-dependent assessment is desired, may therefore
start with calculation of the site-generic photochemical ozone formation impacts of the product as described in the previous section. Based on the site-generic impact, the processes with the dominating
contributions can then be identified (step 1) and their site-generic impacts be adjusted with the relevant site-dependent characterisation factors (step 2 and 3) using the procedure described below. This
procedure can be seen as a sensitivity analysis-based reduction of those uncertainties in the site-generic impact which are posed by refraining from site-dependent characterisation.
Step 1
For each of the sub categories calculate the site-generic photochemical ozone formation impact as described in the previous section, and on this basis identify the processes with the dominating contributions
or decide to do site-dependent characterisation for all processes. Order the contributions from the largest to the smallest and select the process with the largest photochemical ozone formation contribution.
Table 7.1. Factors for site-generic characterisation of photochemical ozone formation impacts on vegetation and human health
Substance |
Impacts on vegetation
(m2•ppm•hour/g) |
Impacts on human
health(pers•ppm•hour/g) |
|
Factor |
standard deviation |
Factor |
standard deviation |
NOx |
1.8 |
2.9 |
1.2•10-4 |
2.7•10-4 |
VOC. CO |
0.73 |
1.2 |
5.9•10-5 |
1.3•10-4 |
CH4 |
0.36 |
0.6 |
2.9•10-5 |
6.3•10-5 |
Step 2
Reduce each of the two site-generic photochemical ozone formation impacts of the product calculated in step 1 with the contribution of the process selected in step 1. Calculate the site-dependent impact
potentials from the emissions of this process with the relevant site-dependent characterisation factors from Annex 7.1 using the following formulas:
Click here to see the Formula
(7.2)
Where:
sd EP(po,veg)p is the site-dependent photochemical ozone formation impact on vegetation expressed as area exposed above threshold by the selected process (p)
(in m2•ppm•hours/f.u.)
sd EP(po,hum)p is the site-dependent photochemical ozone formation impact on human health expressed as persons exposed above threshold by the selected process (p)
(in pers•ppm•hours/f.u.)
sd CF(po,veg)NOx,i is the site-dependent photochemical ozone formation factor from Annex 7.1 that relates emission of NOx from country or region (i), where the selected process (p) is located, to the
impacts on vegetation in the deposition area (in m2•ppm•hours/g).
sd CF(po,veg)VOC,i is the site-dependent photochemical ozone formation factor from Annex 7.1 that relates emission of VOCs or CO from country or region (i), where the selected process (p) is located,
to the impact on vegetation in the deposition area (in m2•ppm•hours/g).
sg CF(po,veg)CH4 is the site-generic photochemical ozone formation factor CH4 from Table 7.1 that relates emission of CH4 to the
impacts on vegetation in the deposition area
(in m2•ppm•hours/g).
sd CF(po,hum)NOx,i is the site-dependent photochemical ozone formation factor from Annex 7.1 that relates emission of NOx from country or region (i), where the selected process (p) is located, to the
impacts on human health in the deposition area (in pers•ppm•hours/g).
sd CF(po,hum)VOC,p is the site-dependent photochemical ozone formation factor from Annex 7.1 that relates emission of VOCs or
CO from country or region (i), where the selected process (p) is located, to the impacts on human health in the deposition area (in pers•ppm•hours/g).
sg CF(po,hum)CH4 is the site-generic photochemical ozone formation factor from Table 7.1 that relates emission of CH4 to the impacts on human health in the deposition area (in pers•ppm•hours/g).
ηi is a substance-specific efficiency factor from Annex 7.2 expressing the ozone creation potential of the individual volatile organic compound or CO (s) relative to the ozone creation potential of the
European average VOC (dimensionless).
Ep,i is the emission of NOx, CH4 or individual or source-specified VOC or CO (s), according to index, from process (p) (in g/f.u.)
For both sub categories, the procedure for calculating the site-dependent impact potential is:
For each process:
- determine in which country the process is located to select the relevant site-dependent characterisation factors in Annex 7.1
- multiply the NOx emission by the relevant site-dependent characterisation factor for NOx from Annex 7.1
- multiply the emissions of individual VOCs, source-specified VOC mixtures or CO by their efficiency factor from Annex 7.2 and add them to the emissions of unspecified VOCs to get the sum-VOC
emission for the process
- multiply the sum-VOC emission by the relevant site-dependent characterisation factor for VOCs and CO from Annex 7.1
- multiply the CH4 emission by the site-generic characterisation factor for CH4 from Table 7.1
- sum the impact potentials thus calculated for NOx, VOC, CO and CH4 to find the impact potential for the process for each of the two photochemical ozone formation sub categories
As a first approach, also the emissions from a non-European or unknown region can be calculated with the site-generic factors from Table 7.1. The standard deviations on the site-generic factors in Table
7.1 give a range of potential spatial variation for the application of the site-generic factor within Europe. Given the size of the variation in emissions and sensitivities within Europe, the site-dependent factor is
expected to lie within this range for most regions, also in the rest of the world. Expert judgement may be used in the interpretation to assess whether the factor for emissions from processes in non-European
regions should be found in the lower or upper end of the range.
Step 3
Add the site-dependent contributions from the process selected in step 1 to the adjusted site-generic contribution from step 2. Repeat step 2 until the site-dependent contribution of the selected processes is
so large that the spatially determined variation in the photochemical ozone impact score can no longer influence the conclusion of the study (e.g. when the site-dependent share is larger than 95% of the total
impact score)
7.7 Normalisation
The EDIP2003 person equivalents for photochemical ozone formation are:
Impacts on vegetation: 1.4•105 m2•ppm•hours/person/year Impacts on human health
and materials: 10 pers•ppm•hours/person/year
Following the EDIP97 approach, the normalisation references for photochemical ozone impact on vegetation and human health are based on the impacts caused by the actual emission levels for 1995 (see
Hauschild and Wenzel 1998e and Stranddorf et al., 2005). Applying the EDIP2003 characterisation factors for photochemical ozone formation, the total exposure of vegetation and humans above the
respective threshold values in Europe is 5.3•1013 m2?ppm•hours and 3.7•109 pers?ppm?hours respectively. The person equivalent is calculated as an average European impact per person assuming a total
European population of 3.70•108 persons.
Due to lack of national European emission estimates for the emissions of CO and CH4, these have not been included in the normalisation references. Based on data collected for Europe and Denmark for the
EDIP97 normalisation references, they are not expected to contribute more than 5% altogether.
7.8 Interpretation
The EDIP2003 photochemical ozone formation impact potentials are improved in two aspects compared to the impact potentials calculated using the EDIP97 characterisation factors; the environmental
relevance is increased, and a part of the spatial variation in sensitivity of the receiving environment is now taken into account.
Environmental relevance
The environmental relevance is increased because the exposure of the sensitive parts of the environment (vegetation or human beings) is included in the underlying model which now covers most of the
causality chain towards the LCA protection areas: Ecosystem health and human health. This is particularly important because it increases consistency with weighting factors based on the environmental
relevance.
The EDIP default weighting factors for photochemical ozone formation are based on political reduction targets. These targets are also aimed partly at protecting human and ecosystem health. In comparison,
the EDIP97 factors only cover the potential for formation of ozone.
In addition, the contribution of NOx is now included in the impact potentials. The significance of this novelty depends for a specific product system on the quantities of NOx and VOCs emitted. From the
calculation of the normalisation references, it is known that on a European level, NOx contributes around twice as much as VOC to photochemical ozone formation, and on average the characterisation
factor for NOx is more than three times as high as the characterisation factor of VOCs.
Spatial variation
The spatial variation in exposure for photochemical ozone formation can be large, even at the very local scale. The variation in sensitivity between European regions is now presented on a national scale
showing a factor 15-20 of difference between least and the most sensitive emission countries for exposure of vegetation, and a factor of around 400 times of difference for exposure of humans (the latter
reflecting the variation in population density in the deposition areas). This variation is hidden when the EDIP97 factors or similar site-generic factors are used for characterisation.
7.9 Example
Applying the EDIP2003 factors, characterisation is performed on the inventory presented in Section 1.6.
Site-generic characterisation
As described in Section 7.5, first, the site-generic impacts are calculated. The photochemical ozone formation impacts on vegetation and human health in Table 7.2 and 7.3 are determined using the
site-generic factors from Table
7.1 and the substance-specific efficiency factors for different VOCs and CO from Annex 7.2.
Table 7.2. Site-generic photochemical ozone impacts on vegetation for one supporting block made from plastic or zinc (mean and standard deviation representing spatial variation)
| Emission to air fromplastic part |
Emission to air from zinc part |
Site-generic ozonimpact, vegetation,
Table 7.1 |
Efficiency factor,Annex 7.1 |
Substance |
g/f.u. |
g/f.u. |
m2ppm•hour/g |
|
|
|
|
|
mean |
std. dev |
|
Hydrogen chloride |
0.001163 |
0.00172 |
|
|
|
Carbon moNOxide |
0.2526 |
0.76 |
0.73 |
1.2 |
0.075 |
Ammonia |
0.003605 |
0.000071 |
|
|
|
Methane |
3.926 |
2.18 |
0.36 |
0.6 |
0.018 |
VOC, power plant |
0.0003954 |
0.00037 |
0.73 |
1.2 |
1.3 |
VOC, diesel engines |
0.02352 |
0.0027 |
0.73 |
1.2 |
1.5 |
VOC, unspecified |
0.89 |
0.54 |
0.73 |
1.2 |
1 |
Sulphur dioxide |
5.13 |
13.26 |
|
|
|
Nitrogen oxides |
3.82 |
7.215 |
1.8 |
2.9 |
|
Lead |
8.03•10-5 |
0.000260 |
|
|
|
Cadmium |
8.66•10-6 |
7.45•10-5 |
|
|
|
Zinc |
0.000378 |
0.00458 |
|
|
|
Total |
|
|
|
|
|
|
Site-generic ozoneimpac,
|
Site-generic ozone impact, |
|
vegetation, plastic |
vegetation, zinc part |
Substance |
m2ppm•hour/f.u. |
m2ppm•hour/f.u. |
|
Mean |
std.dev. |
Mean |
std.dev. |
Hydrogen chloride |
|
|
|
|
Carbon moNOxide |
0.014 |
0.023 |
0.042 |
0.068 |
Ammonia |
|
|
|
|
Methane |
0.025 |
0.042 |
0.014 |
0.024 |
VOC, power plant |
3.8•10-4 |
6.2•10-4 |
3.5•10-4 |
5.8•10-4 |
VOC, diesel engines |
0.026 |
0.042 |
0.0030 |
0.0049 |
VOC, unspecified |
0.65 |
1.1 |
0.39 |
0.65 |
Sulphur dioxide |
|
|
|
|
Nitrogen oxides |
6.9 |
11.1 |
13.0 |
20.9 |
Lead |
|
|
|
|
Cadmium |
|
|
|
|
Zinc |
|
|
|
|
Total |
7.66 |
12.33 |
13.44 |
21.77 |
Table 7.3. Site-generic photochemical ozone impacts on human health for one supporting block made from plastic or zinc (mean and standard deviation representing spatial variation)
|
Emissionto air from plastic part |
Emissionto air from zinc part |
Site-generic ozone impact, human health,
Table 7.1 |
Efficiency factor,Annex 7.1 |
Substance |
g/f.u. |
g/f.u. |
pers•ppm•hours |
|
|
|
|
|
mean |
std.dev. |
|
Hydrogen chloride |
0.001163 |
0.00172 |
|
|
|
Carbon moNOxide |
0.2526 |
0.76 |
5.9•10-5 |
1.3•10-4 |
0.075 |
Ammonia |
0.003605 |
0.000071 |
|
|
|
Methane |
3.926 |
2.18 |
2.9•10-5 |
3.6•10-5 |
0.018 |
VOC, power plant |
0.0003954 |
0.00037 |
5.9•10-5 |
1.3•10-4 |
1.3 |
VOC, diesel engines |
0.02352 |
0.0027 |
5.9•10-5 |
1.3•10-4 |
1.5 |
VOC, unspecified |
0.89 |
0.54 |
5.9•10-5 |
1.3•10-4 |
1 |
Sulphur dioxide |
5.13 |
13.26 |
|
|
|
Nitrogen oxides |
3.82 |
7.215 |
1.2•10-3 |
2.7•10-4 |
|
Lead |
8.03•10-5 |
0.000260 |
|
|
|
Cadmium |
8.66•10-6 |
7.45•10-5 |
|
|
|
Zinc |
0.000378 |
0.00458 |
|
|
|
Total |
|
|
|
|
|
|
Site-generic ozone impact,
human health, plastic part |
Site-generic ozone impact,
human health, zinc part |
Substance |
pers•ppm•hours/f.u |
pers•ppm•hours/f.u |
|
mean |
std.dev. |
mean |
std.dev. |
Hydrogen chloride |
|
|
|
|
Carbon moNOxide |
1.1•10-6 |
2.5•10-6 |
3.4•10-6 |
7.4•10-6 |
Ammonia |
|
|
|
|
Methane |
2.0•10-6 |
2.5•10-6 |
1.1•10-6 |
1.4•10-6 |
VOC, power plant |
3.0•10-8 |
6.7•10-8 |
2.8•10-8 |
6.3•10-8 |
VOC, diesel engines |
2.1•10-6 |
4.6•10-6 |
2.4•10-7 |
5.3•10-7 |
|
|
|
|
|
VOC, unspecified |
5.3•10-5 |
1.2•10-4 |
3.2•10-5 |
7.0•10-5 |
Sulphur dioxide |
|
|
|
|
Nitrogen oxides |
4.6•10-3 |
1.0•10-3 |
8.7•10-3 |
1.9•10-3 |
Lead |
|
|
|
|
Cadmium |
|
|
|
|
Zinc |
|
|
|
|
Total |
4.66•10-3 |
1.22•10-3 |
8.77•10-3 |
2.00•10-3 |
Using site-generic characterisation factors, the largest impacts are found to be caused by the zinc supporting block for both of the two photochemical ozone sub categories. In both cases, the impacts are 2-3
times higher for the zinc supporting block than the impacts calculated for the plastic block. However, the potential spatial variation is so large (as revealed by the spatially determined standard deviation) that
the conclusion is highly uncertain. Therefore, a site-dependent characterisation is performed for those processes which contribute the most to the site-generic impacts, in order to reduce the spatially
determined uncertainty and strengthen the conclusion.
Site-dependent characterisation
Table 7.2 and 7.3 show that the impacts on vegetation as well as on human health are dominated by the contribution from NOx, while an emission of unspecified VOCs is also noticeable. The main NOx
sources for the zinc component, are identified as the production of zinc from ore which takes place in Bulgaria, the casting of the component which takes place in Yugoslavia and that part of the transport of
the component, which takes place by truck through Germany (data not shown). For the plastic component, the main sources for NOx are found to be the production of plastic polymer in Italy, the flow
injection moulding of the supporting block in Denmark and the transportation of the component by truck, mainly through Germany (idem). The unspecified VOC-emission from the plastic component is
caused by the plastic polymer production in Italy, and for the zinc component, it comes from the casting process in Yugoslavia (data not shown). The emissions from these processes contribute more than
99% for the zinc component and 75% for the plastic component for impacts on vegetation (Table 7.2) as well as impacts on human health (Table 7.3).
In the calculation of the site-dependent impacts for these key processes, the relevant factors from Annex 7.1 (photochemical ozone formation) are applied. The results are shown in Table 7.4.
Table 7.4. Site-dependent photochemical ozone impacts on vegetation and human health for key processes from either product system.
Zinc part |
Emission |
Ozone formation factor,
vegetation, Annex 7.2 |
Ozone formation factor, human health,Annex 7.2 |
Impact, vegetation |
Impact, human health |
|
g/f.u. |
m2ppm•hours/g |
pers•ppm•hours/g |
m2ppm•hours/f.u. |
pers•ppm•hours/f.u. |
NOx emissions |
|
|
|
|
|
Zinc production, Bulgaria |
0.97 |
1.4 |
2.20•10-6 |
1.4 |
2.1•10-6 |
Zinc casting, Yugoslavia |
1.65 |
1.6 |
2.20•10-6 |
2.6 |
3.6•10-6 |
Transport, mainly Germany |
4.56 |
2.9 |
1.70•10-4 |
13.2 |
7.8•10-4 |
VOC emissions |
|
|
|
|
|
Zinc casting, Yugoslavia |
0.53 |
0.2 |
1.40•10-5 |
0.1 |
7.4•10-6 |
Total. zinc part |
|
|
|
17.33 |
7.99•10-4 |
Plastic part |
Emission |
Ozone formation factor,
vegetation, Annex 7.2 |
Ozone formation factor, human health,Annex 7.2 |
Impact, vegetation |
Impact, human health |
|
g/f.u. |
m2 ppm•hours/g |
pers•ppm•hours |
m2 ppm•hours/f.u. |
pers•ppm•hours/f.u. |
NOx emissions |
|
|
|
|
|
Plastic production, Italy |
0.63 |
1.5 |
2.00•10-4 |
0.9 |
1.3•10-4 |
Flow injection moulding, Denmark |
0.48 |
1.5 |
3.40•10-5 |
0.7 |
1.6•10-5 |
Transport, mainly Germany |
1.74 |
2.9 |
1.70•10-4 |
5.0 |
3.0•10-4 |
VOC emissions |
|
|
|
|
|
Plastic polymer production, Italy |
0.87 |
0.7 |
1.00•10-4 |
0.6 |
8.7•10-5 |
Total. plastic part |
|
|
|
7.33 |
5.33•10-4 |
The site-generic impacts from the key processes are subtracted from the original site-generic impacts in Table 7.2 and 7.3, and the site-dependent impacts from the key processes calculated in Table 7.4 are
added. The photochemical ozone impacts thus corrected are found in Table 7.5, and the differences to the original site-generic impacts of Table 7.2 are illustrated in Figure 7.1.
Table 7.5. Photochemical ozone impacts from either product system with site-dependent characterisation of key process emissions
| Ozone, vegetation |
Ozone, human health |
| m2ppm•hours/f.u. | pers•ppm•hours/f.u. |
Zinc component | 17.6 | 8.80•10-4 |
Plastic component | 10.9 | 2.90•10-3 |
For photochemical ozone formation impact on vegetation, more than 99% of the impacts for the zinc component in Table 7.5 include the spatial information. Even if the site-dependent characterisation was
performed for all the remaining processes in the product system, the result will thus not change significantly, given their modest share in the total and the standard deviation. The spatially conditioned
uncertainty of the impact has largely been cancelled. For the plastic component, however, the figure is 85% for impacts on vegetation and for impacts on human health it is as low as 60%. This means that for
the plastic component, particularly the figure for impacts on human health may still change if further spatial characterisation is performed. More key processes need thus to be included in order to cancel the
spatially determined uncertainty of the conclusion.
Click here to see the Figure
Figure 7.1 summarises the difference between the site-generic and the site-dependent impacts.
Figure 7.1 Site-generic and site-dependent photochemical ozone impacts on vegetation and human health from the two product systems. For the site-dependent impacts, the site-dependent characterisation
factors have only been applied for key processes as described above.
As seen from Figure 7.1, the inclusion of spatial differentiation at the level of country of emission reverses the dominance in ozone impact on human health. When a major part of the spatial variation in the
dispersion patterns and sensitivity of the exposed environment (i.e. population density) is eliminated, the impact from the plastic component is larger than the impact from the zinc component. For ozone
impacts on vegetation, the ranking of the two alternatives remains the same also after spatial characterisation. Considering that for the plastic component, the ozone impact on human health still comprises a
significant potential for spatial variation, no conclusion can be drawn yet for this impact category
Annex 7.1: Site-dependent photochemical ozone formation factors
Factors for site-dependent characterisation of photochemical ozone formation impacts on vegetation and human health
Country | Vegetation | Human health |
| NOx | VOC | NOx | VOC |
| m2•ppm•hours/g | pers•ppm•hours/g |
Albania | 1.1 | 0.2 | 9.40E-06 | 4.00E-06 |
Austria | 3 | 0.5 | 7.00E-05 | 4.70E-05 |
Belarus | 1.6 | 0.4 | 2.50E-06 | 7.20E-09 |
Belgium | 1.6 | 1.1 | 3.80E-04 | 2.20E-04 |
Bosnia/ Herzegovina | 1.6 | 0.2 | 1.30E-05 | 3.50E-05 |
| | | | |
Bulgaria | 1.4 | 0.3 | 2.20E-06 | 2.20E-06 |
Croatia | 2.4 | 0.3 | 3.80E-05 | 1.20E-04 |
Czech Republic | 2.4 | 0.9 | 2.10E-04 | 6.20E-07 |
Denmark | 1.5 | 0.8 | 3.40E-05 | 2.70E-05 |
Estonia | 0.2 | 0.6 | 1.20E-06 | 9.40E-06 |
Finland | 0.4 | 0.3 | 8.50E-07 | 5.20E-07 |
France | 3.4 | 0.9 | 2.20E-04 | 1.20E-04 |
Germany-new | 2.9 | 1.5 | 1.70E-04 | 1.10E-04 |
Germany-old | 2 | 1.3 | 3.30E-04 | 1.90E-04 |
Greece | 0.5 | 0.1 | 1.90E-05 | 1.10E-05 |
Hungary | 4.3 | 0.8 | 3.00E-05 | 2.40E-05 |
Ireland | 0.2 | 0.1 | 1.20E-05 | 8.50E-06 |
Italy | 1.5 | 0.7 | 2.00E-04 | 1.00E-04 |
Latvia | 0.4 | 0.3 | 2.50E-06 | 1.00E-06 |
Lithuania | 1.1 | 0.6 | 4.20E-06 | 1.30E-06 |
Luxemburg | 0.8 | 0.1 | 1.10E-04 | 5.80E-05 |
Macedonia | 0.5 | 0.2 | 4.30E-06 | 3.80E-05 |
Moldova | 0.7 | 0.5 | 1.50E-06 | 1.80E-06 |
Netherlands | 0.8 | 0.9 | 2.30E-04 | 1.30E-04 |
Norway | 0.2 | 0.1 | 2.10E-06 | 1.50E-06 |
Poland | 2.5 | 1.2 | 1.10E-04 | 6.90E-05 |
Portugal | 3.5 | 1.1 | 1.30E-04 | 6.70E-05 |
Romania | 2.1 | 0.3 | 5.00E-06 | 5.00E-06 |
Russia-Kaliningrad | 0.2 | 0 | 3.60E-06 | 4.70E-06 |
| | | | |
Remaining Russia | 0.9 | 0.2 | 2.10E-06 |
2.90E-0 |
Slovakia | 3.4 | 0.7 | 5.60E-05 | 1.50E-06 |
Slovenia | 1.2 | 0.2 | 2.60E-05 | 2.70E-06 |
Spain | 2.3 | 0.6 | 4.60E-05 | 2.40E-05 |
Sweden | 1 | 0.4 | 1.20E-05 | 8.30E-06 |
Switzerland | 2.2 | 0.4 | 9.80E-05 | 6.10E-05 |
Ukraine | 2 | 0.7 | 3.70E-06 | 2.10E-07 |
United Kingdom | 1 | 1.3 | 9.90E-05 | 6.00E-05 |
Yugoslavia | 1.6 | 0.2 | 2.20E-06 | 1.40E-05 |
Atlantic Ocean | 0.5 | 0 | 1.40E-05 | 0.00E+00 |
Baltic Sea | 0.5 | 0 | 1.50E-06 | 0.00E+00 |
North Sea | 1.1 | 0.2 | 7.80E-05 | 0.00E+00 |
Annex 7.2: Efficiency factors for individual VOCs and source-specified VOCs
Individual VOCsand methane |
Efficiencyfactor ηs |
Individual VOCs,carbon moNOxide and source specified VOCs |
Effiencyfactor ηs |
Alkanes |
1 |
Aldehydes |
1.5 |
|
|
formaldehyde |
1.1 |
ethane |
0.21 |
acetaldehyde |
1.3 |
propane |
1.1 |
propionaldehyde |
1.5 |
n-butane |
1 |
butyraldehyde |
1.4 |
isobutane |
0.79 |
isobutyraldehyde |
1.6 |
n-pentane |
1 |
valeraldehyde |
1.7 |
isopentane |
0.74 |
acrolein |
2 |
n-hexane |
1.1 |
Ketones |
1 |
2-methylpentane |
1.3 |
acetone |
0.45 |
3-methylpentane |
1.1 |
methyl ethyl ketone |
1.1 |
2.2-dimethylbutane |
0.63 |
methyl isobutyl ketone |
1.6 |
2.3-dimethylbutane |
0.96 |
Alcohols |
0.66 |
n-heptane |
1.3 |
methanol |
0.31 |
2-methylhexane |
1.2 |
ethanol |
0.67 |
3-methylhexane |
1.2 |
isopropanol |
0.5 |
n-octane |
1.2 |
butanol |
1 |
2-methylheptane |
1.2 |
isobutanol |
0.75 |
n-nonane |
1.2 |
butan-2-diol |
0.75 |
2-methyloctane |
1.3 |
Ethers |
1 |
n-decane |
1.2 |
dimethyl ether |
0.75 |
2-methylnonane |
1.1 |
propylene glycol methyl ether |
1.3 |
n-undecane |
1.1 |
Esters |
0.51 |
n-dodecane |
1 |
methyl acetat (=dimethyl ester) |
0.06 |
Alkenes |
2.2 |
ethyl acetate |
0.55 |
ethylene |
2.5 |
isopropyl acetate |
0.54 |
propylene |
2.6 |
n-butyl acetate |
0.81 |
1-butene |
2.4 |
isobutyl acetate |
0.83 |
2-butene (trans) |
2.5 |
Propylene glycol methyl ether-acetate |
0.25 |
Isobutene |
1.5 |
Chloro-alkanes |
0.011 |
2-pentene (trans) |
2.3 |
methylen chloride |
0.023 |
1-pentene |
2.6 |
chloroform |
0.0075 |
2-methylbut-1-ene |
1.9 |
methyl chloroform |
0.0025 |
3-methylbut-1-ene |
2.2 |
Chloro-alkenes |
0.64 |
2-methylbut-2-ene |
1.9 |
trichloroethylene |
0.17 |
2-methylpropene |
1.6 |
tetrachloroethylene |
0.01 |
Isoprene |
2 |
allyl chloride |
1.8 |
The dimensionless efficiency factor is representing the efficiency of individual VOCs relative to the European average VOC in contributing to ozone formation. It is derived as the quotient between the
respective POCP-factors for 4-9 days in high NOx-areas (the EDIP97 characterisation factors for high NOx-areas).
Annex 7.2: Efficiency factors for individual VOCs and source-specified VOCs
Individual VOCsand methane | Efficiencyfactor ηs | Individual VOCs, carbon moNOxide andsource specified VOCs | Efficiencyfactor ηs |
Alkynes | | Inorganic compounds | |
acetylene | 0.42 | carbon moNOxide | 0.075 |
Aromatics | 1.9 | | |
benzene | 0.47 | Source-specified VOC mixtures | |
toluene | 1.4 | Petrol-powered car, exhaust | 1.5 |
o-xylene | 1.7 | Petrol-powered car, vapour | 1.3 |
m-xylene | 2.5 | Diesel-powered car, exhaust | 1.5 |
p-xylene | 2.2 | Power plants | 1.3 |
ethylbenzene | 1.5 | Combustion of wood | 1.5 |
n-propylbenzene | 1.2 | Food industry | 1 |
isopropylbenzene | 1.4 | Surface coating | 1.3 |
1.2.3-trimethylbenzene | 2.9 | Chemical cleaning of clothes | 0.75 |
1.2.4-trimethylbenzene3 | | Refining and distribution of oil | 1.3 |
1.3.5-trimethylbenzene | 2.9 | Natural gas leakage | 0.05 |
o-ethyltoluene | 1.7 | Coal mining | 0.018 |
m-ethyltoluene | 2 | Farming | 1 |
p-ethyltoluene | 1.8 | Landfilling of household waste | 0.018 |
8. Human toxicity
Background information for this chapter can be found in:
- Chapter 7 of the "Environmental assessment of products. Volume 2: Scientific background" by Hauschild and Wenzel (1998a).
- Chapter 8 of the "Background for spatial differentiation in life cycle impact assessment – EDIP2003 methodology" by Potting and Hauschild (2005).
8.1 Introduction
Nearly all substances are in principle toxic to human beings. It is only the dose as determined by the exposure that can prevent a substance from exerting its human toxic potential. There are three main routes
of human exposure to environmental pollutants: (1) inhalation with air, (2) ingestion with food and water (and sometimes also soil), and (3) penetration of the skin after contact with air (sometimes also soil or
water) or polluted surfaces. The exposure of humans to environmental pollutants usually occurs via more than one route at the same time (multi-route exposure), but one exposure route often dominates over
the others. Exposure trough inhalation results in most cases directly from emissions to air. Exposure through ingestion is usually the result of re-distribution between different environmental media and the food
chain. The intake of food is dominating the exposure through ingestion but to some extent, emission to soil and water may also result in direct exposure by ingestion of soil (pica, contaminated vegetables) and
water (as drinking water).
Typically, characterisation of human toxicity focuses on inhalation and ingestion. The methodology presented in this chapter focuses on inhalation since this is the exposure route where spatial differentiation is
anticipated to be of the largest relevance.
8.2 Classification
For the classification of substances contributing to human toxicity, a screening tool has been developed as part of EDIP97 based on the substance characteristics that dispose a substance for toxicity (Wenzel
et al., 1997). It is recommended to use this tool in combination with some of the existing lists of priority pollutants like the list of undesirable substances and the effect list from the Danish EPA (2000a and b).
8.3 EDIP97 characterisation factors
Characterisation of human toxicity can be based on quite different types of modelling. Presently, characterisation factors can roughly be divided into factors based on multi-media full-fate modelling, and
factors based on some-fate modelling. The EDIP97 characterisation factors are representative of the latter group. The strategy behind the fate modelling of the EDIP97 method has been to identify those
properties that are important for the substance's potential for human toxicity and then combine these in a transparent way in the expression of the characterisation factor. This is seen as preferable to basing it on adaptation of one of the existing multimedia models which have been developed and used for something else, namely
generic risk assessment of chemicals, and which are generally of little transparency. The EDIP97 factors are retained in the new characterisation factors to characterise the site-generic human toxic impact.
Characterisation of human toxicity is complex because of the large number of relevant substances involved, and the various interacting environmental processes leading to exposure. Though spatial
differentiation may play a role in all processes, it was not further explored for exposures through ingestion. It was considered more important for inhalation exposures directly resulting from emissions to air
since these are known to be strongly influenced by spatial variation:
- The stack height together with local and regional meteorological conditions determine the pattern of concentration increases resulting from an emission. The size of the area of concentration increases differs
between substances, but has a radius of several hundreds kilometres (short-lived substances) to thousands of kilometres (long-lived substances). The exposure in the local area surrounding the source is most
important for short-lived substances, while the exposure in the long range dominates for long living substances.
- Population densities show considerable spatial variation within the exposed area, as well as between exposure areas, for emissions released at different geographic locations.
- The extent to which an area is already exposed to concentration increases from other sources (background concentration) depends on its location in relation to major industrial and inhabited areas. Most
areas receive pollutants from very many sources, which usually means that the contribution from any single source is very small at the regional level. At the local level, the concentration increase from the
source will be larger but in general, if regulated properly, as is usually the case in industrialised countries, not large enough on its own to cause no-effect-levels of toxic substances to be exceeded.
As a result, not all substance emitted will result in adverse human exposure. The final human exposure depends on the geographic location where an emission is released.
8.4 EDIP2003 factors for human toxicity
The EDIP2003 site-generic factors do not replace the EDIP97 characterisation factors. Rather, they should be considered as exposure factors to be used in combination with the EDIP97 factors which are
maintained to characterise the site-generic impact on human toxicity from emissions. The EDIP97 characterisation factors are listed in Annex 8.1 to
8.3.
The EDIP2003 exposure factors have been established to evaluate spatially determined variations in the increase of human exposure4 through inhalation resulting directly from air emissions. The exposure
factors have been established for combinations of the following situations:
- Emitted substance: a short-lived (hydrogen chloride) and a long-lived (benzene) model substance
- Different heights of emission
- Different geographical locations
- Actual variation in atmospheric conditions
- Actual variation in regional and local population densities
The range of variation in the site-dependent exposure factors, which can be found by varying these parameters, provides insight in the potential variation in the site-generic human toxicity impact potential.
The accumulated exposure increase has been calculated for a long-lived substance (benzene, residence time of about one week) and a short-lived substance (hydrogen chloride, residence time of about 7
hours). These two substances have been selected because the residence time, and thereby the accumulated exposure increase, for emissions of most substances will lie between those of hydrogen chloride
and benzene. The source strength is kept at one gram per second continuously, but the influence of the height of the release point is investigated (1m, 25m, and 150m). The accumulated human exposure
increase from a release is the product of concentration increase and population density integrated over the whole surface.
The site-dependent exposure factor consists of two parts, one quantifying the exposure close to the source (0-10km), and one quantifying exposure over longer distances from the source (>10km).
Concentrations local to the source are estimated with the EUtrend model5, while the WMI model6 is used for estimating concentration increases at longer distances from the source.
4 The term "exposure" in the remainder of this chapter is equivalent with the term "increase of accumulated exposure" in Chapter 6 in Potting and Hauschild (2004).
5 The EUTREND model follows a
Gaussian plume approach to calculate concentration spatially resolved over the European grid. A specific strength of EUTREND is its capacity to accurately model the local dimension of emission dispersion
by using 1990 meteorology (annual statistics mean).
6 The Wind rose Model Interpreter (WMI) is part of the integrated assessment model EcoSense. WMI follows trajectory modelling based on region dependent atmospheric conditions (1990 annual
statistics mean).
The EUTREND model describes the mixing of the plume with the surrounding air after a substance is released from its source. Within a few hundred meters, the plume usually results in concentration
increases at ground level. Wind speeds largely determine how fast the plume dilutes, whereas the release height also influences how fast the plume reaches ground level. EUTREND models the resulting
concentration increases at ground level with a Gaussian plume approach applying region-dependent atmospheric conditions. The calculations are performed for the three different release heights and for
sources located in different climates:
- A maritime climate (approximated by atmospheric conditions in the Netherlands
- Climate in North Europe (approximated by atmospheric condition in Finland)
- Climate in Central Europe (approximated by atmospheric conditions in Austria)
- Climate in South Europe (approximated by atmospheric conditions in Italy)
The EUTREND results show a modest difference in local accumulated exposure between the maritime and North European climate regions on the one hand, and the South and Central European climates on
the other hand. The influence of source height on local accumulated exposure is more moderate than anticipated, but nevertheless considerable for tall sources. The climate region becomes more important
with lower release heights due to the considerable difference in wind velocities between the regions. Low wind velocities give slower dilution and subsequently higher ground concentrations than high wind
speeds. In addition, low wind velocities are usually accompanied by modest mixing heights for the plume. Wind velocities in the south and central climate regions are on average lower than in the maritime and
northern climate regions.
At longer distances from the source, the plume attains a homogeneous vertical distribution in the mixing layer of the atmosphere. Trajectory or one-dimensional Lagrangian modelling is an often-used way to
trace concentration increases resulting from substance transport and removal at long ranges. The Wind rose Model Interpreter (WMI) has been adapted for our purpose from the EcoSense integrated
assessment model (Krewitt et al. 1997). For any receptor point, it models the input from outside the grid cell differentiating between twenty-four sectors of the wind rose, such that from each sector a
straight-line trajectory arrives at the receptor point. Concentrations at the receptor point are obtained by averaging over the dispersion results from these trajectories, suitably weighted by the frequencies of
winds in each 15° sector. WMI supports modelling of substance fate along these trajectories based on region-dependent atmospheric conditions (1990 annual statistics mean).
For the present study, it has been employed to set up a single layer model with a horizontal resolution of 150•150 km2 on the EMEP7 grid, assuming a constant mean mixing height.
The WMI results show that while high wind speeds cause dilute concentrations and thus decrease human exposure close to the source, they increase the distances over which a substance is transported.
Transport over longer distance results in more people being exposed but to a lower concentration. The direct net effect of high wind speed on accumulated exposure is therefore usually small. Spatial
variability of precipitation is also considered in the model. While wet deposition is of minor importance for benzene, hydrogen chloride is removed from the atmosphere with every shower due to its high
affinity for water. Precipitation varies strongly over the grid from 2000–3200 mm•a-1 in grid-squares at the Norwegian coast around Bergen down below 200 mm•a-1 in the Sahara Desert, parts of Turkey,
Southeast Russia and Kazakhstan. Due to its longer lifetime, the accumulated exposure to benzene is less dependent on local than on regional population density. The model domain (Europe) is actually too
small to trace benzene concentrations over their full residence time. Approximately 40% of the benzene emitted at the Central European site and almost 60% of the benzene emitted at the North European
site is subject to atmospheric transport beyond the edges of the model grid. An extrapolation has been performed to cover the exposure taking place outside the European grid (see Potting et al., 2005b).
Spatially resolved European population data from Tobler et al. (1995; see Annex 8.4) is used in the models to estimate the exposure which is expressed as the product of the concentration increase and the
population exposed to it (pers•μg/m3/g emitted).
The site-dependent factors for regional human exposure show a difference between highest rating area (South-Eastern Netherlands) and the very low ratings (in some very sparsely populated areas in the far
North) of less than a factor 20 for the long-lived benzene, but almost a factor 100 for the short-lived hydrogen chloride. While the uncertainties in the modelling underlying those factors are acceptable, the
spatial variation of the impact potential is thus considerable at the regional level.
The site-dependent factors for local human exposure (0-10km) show that exposure close to the source is less than a factor 2 higher from a release height of 1m than from a release height of 25m. The
exposure from a release height of 25m is a factor 6 to 10 higher than exposure from a release height of 150m. In comparison to the regional situation, these differences are moderate.
7 Co-operative Program for Monitoring and Evaluation of the long range transmission of air pollutants in Europe.
Application of the EDIP2003 exposure factors to evaluate the spatial variation in the human toxicity impact from inhalation is simple but does require additional information (see Section 8.6) on the emission
height and the geographical location where the emission takes place. Typical life cycle inventories already provide data about the region where an emission is released, but usually no information is available
on the height of the emission point and whether the emission is released in the vicinity of built-up areas. The latter two are of importance for the exposure local to the source.
Though the geographical region of release is often known, this information will not always be available, and for some applications it is also preferable to refrain from site-dependent characterisation. The
moderate range found between the highest and lowest site-dependent factors for local exposure moreover justifies being reluctant in applying these. Together with the fact that the exposure factors have only
been calculated for two model substances this means that the main interest of the established site-dependent local and regional exposure factors will be for sensitivity analysis to help quantify
the possible spatial variation underlying the site-generic impact potentials.
What do the impacts express?
The EDIP2003 human toxicity exposure factors for air-borne emissions express the exposure of human beings within the predicted deposition area as the product of the concentration increase and the
number of people exposed to it (g/m3•person), integrated over the full deposition area within Europe. The EDIP97 human toxicity characterisation factors for exposure via air represent the substance's
inherent ability to cause human toxicity via air exposure. They are calculated as the reciprocal of a fate-corrected human reference dose or –concentration and are thus really effect factors or severity factors
which inherently assume that an exposure takes place
(m3/g(/person)). The exposure factor for an emission and the effect factor of the substance are multiplied to calculate the human toxic impact potential. The exposure-corrected impact potential is
dimensionless.
In comparison, the EDIP97 factors express the volume of environmental compartment (air, water, soil) which can be polluted up to the human reference concentration or –dose, the level not expected to
cause effects on lifelong exposure (m3/g).
8.5 Site-generic characterisation, all exposure routes
Factors have been developed to evaluate exposure via inhalation for hydrogen chloride (atmospheric residence time of the substance around one day)
and benzene (atmospheric residence time around one week or longer). These two substances are intended to represent the dispersion pattern of short-lived and relatively long-lived pollutants respectively.
The site-generic human toxicity impact potential for exposure via air is calculated using the site-generic (European average) exposure factors in Table 8.1 in combination with the EDIP97 characterisation
factors for human toxicity via air from Wenzel et al. (1997) according to the following expression:

(8.1)
Where: sg-EP(hta)= The site-generic human toxicity impact from the product (dimensionless) through inhalatory exposure from atmospheric emissions
sg-HEFregional, s
= The site-generic exposure factor (person•μg/m3) from Table 8.1, which relates the emission of substance (s) (represented by HCl or benzene) to exposure at the regional level
sg-HEFlocal, s = The site-generic exposure factor (person•μg/m3) from Tale 8.1, which relates the emission of substance (s) (represented by HCl or benzene) to exposure at
the local level
CF(hta)s= The EDIP97 characterisation factor for human toxicity (in m3/g) from Annex 8.1, which relates the emission of substance (s) into air to the impact for
exposure via air
E(a)s = The emission of substance (s) to air (in g per functional unit).
The EDIP97 characterisation factors for human toxicity via air are found in Annex 8.1.
Table 8.1. Factors for site-generic, and for site-dependent human exposure assessment (in person•μg/m3 per gram emitted)
Regional |
Site-generic assessment
Site-generic exposure factors =
sg-HEF(s) |
Site-dependent assessment
Site-dependent exposure factors |
|
|
|
(factor to be found in Annex 8.5-8.7) |
Substance |
Factor |
standard deviation |
factor = sd-HEF(s)i |
C6H12 |
50000 |
33000 |
sd-HEFregional(C6H12)i |
HCl-25m (*) |
2460 |
1600 |
sd-HEFregional (HCl)| |
HCl-1m |
2190 |
1420 |
sd-HEFregional (HCl)| |
HCl-150m |
3200 |
2080 |
sd-HEFregional (HCl)| |
Local |
Site-generic assessment
Site-generic exposure factors
=
sg-HEF(s) |
|
Site-dependent assessment
Site-dependent exposure factors |
|
|
|
(factor to be found in Annex 8.5-8.7) |
Substance |
Factor |
standard deviation |
factor = sd-HEF(s)i |
C6H12-25m (**) |
6970 |
|
PDi•sd-HEFlocal (C6H12)i |
HCl-25m (**) |
3620 |
|
PDi•sd-HEFlocal (HCl)i |
*The value for a release height of 25m is taken as default
**These values refer to southern Europe, and a population density of 100 persons/km2
For exposure via inhalation, the potential spatial variation of the exposure and the resulting human toxicity impact can be estimated from the standard deviation in the site-generic exposure factors in Table
8.1.
8.6 Site-dependent characterisation
The human toxicity impact from a given product is in many cases dominated by one or a few processes. Even for applications, where a site-dependent assessment is preferred, it is therefore advised to start
with calculation of the site-generic impact of a product as described in the previous section, taking into account exposure in a site-generic situation. This site-generic impact can be used to select the
processes with the dominating contributions (step 1), and next to evaluate the actual spatial variation in the contribution from these processes by applying the relevant site-dependent factors (step 2 and
3).
Step 1
The site-generic human toxicity impact by inhalation resulting directly from air emissions, as calculated in the previous section, is broken down into the contributions from the separate processes. These
contributions are then ranked from the largest to the smallest contribution, and the process with the largest contribution is selected.
Step 2
The site-generic human toxicity impact from step 1 is reduced with the contribution of the process selected in step 1. Next, the site-dependent impact from the emissions of this process is calculated using the
relevant site-dependent factors.
Click here to see the Formula
(8.2)
Where:
sd-EP(hta)p = The site-dependent human toxicity impact (dimensionless) from process (p) through the inhalatory exposure from atmospheric emissions
d-HEFregional(h)s,i = The site-dependent exposure factor (person•μg/m3) which relates the emission of substance (s) (represented by HCl or benzene) released at height (h) in country or region (i) (where process (p) is located) to exposure at the regional level. The
site-dependent factors for regional exposure can be found in Annex 8.5 for hydrogen chloride and Annex 8.6 for benzene.
sd-HEFlocal(h)s,i = The site-dependent exposure factor (person•μg/m3) which relates the emission of substance (s) (represented by HCl or benzene) released at height (h) in country or region (i) (where process (p) is located) to exposure at the local level. The
site-dependent factors for local exposure can be determined from Annex 8.7.
PDi = The local population density in country or region (i) where process (p) is located. The local population density can be estimated from Annex
8.4, or roughly be taken as 100 person/km2 for rural areas, 500 person/km2 for urbanised areas, 1000-5000 person/km2 for built-up areas, and >10,000 person/km2 for city-centres
CF(hta)s = The EDIP97 factor for human toxicity (in m3/g) from Annex 8.1 which relates the emission of substance(s) into air to the impact from an exposure via air
E(a)s,p = The emission to air of substance (s) from process (p) (in g per functional unit).
The geographic region in which the emissions take place determines the relevant regional and local factors of the source. The impact of emissions from unknown but probably European regions can be
calculated with the site-generic exposure factors (see previous section). The information about the potential spatial variation in these factors (see table 8.1) should be taken into account in the next step.
As a first approach, also the emissions from a non-European or unknown region can be calculated with the site-generic exposure factors from previous section. The standard deviations for the site-generic
factors in Table 8.1 give a range for their spatial variation within Europe. Given the size of the variation in emissions and sensitivities within Europe, the site-dependent factor is expected to lie within this range
for most regions also in the rest of the world. Expert judgement may be used in the interpretation to assess whether the factor for emissions from processes in non-European regions should be found in the
lower or upper end of the range.
Step 3
The site-dependent contributions from the process selected in step 1 are added to the adjusted site-generic contribution from step 2. Step 2 is repeated until the site-dependent contribution from the selected
processes is so large that the residual spatial variation in the human toxicity score can no longer influence the conclusion of the study (e.g. when the site-dependent share is larger than 95% of the total impact
score).
8.7 Normalisation
The EDIP2003 person equivalent for human toxicity via air using the EDIP2003 exposure factors is 1.7•108 yr-1
Following the EDIP97 approach, the normalisation reference for human toxicity via air is based on the impact caused by the actual emission levels for 1994 (see Hauschild and Wenzel 1998f and Stranddorf
et al., 2005). Applying the EDIP2003 exposure factors for human toxicity via air together with the characterisation factors from EDIP97, the total impact from the emissions in a representative number of
European countries, for which relevant air emission data is found, is 4.4•1016. The person equivalent is calculated as an average European impact per person assuming a population in these countries of
2.55•108 persons. The calculation of the normalisation reference is documented in Annex 8.8.
8.8 Interpretation
Considering the moderate range found between the highest and lowest site-dependent exposure factors and acknowledging the fact that the exposure factors have only been calculated for two model
substances, the main interest of the established site-dependent exposure factors lies in their use for representing this part of spatial variation in a sensitivity analysis to help quantify the possible spatial variation
underlying the site-generic impact potentials.
The exposure factors relate emissions of toxic substances to the increase in human exposure. Combined with the EDIP97 or similar site-generic characterisation factors for human toxicity, the exposure
factors indicate the increase in human toxic pressure from the emission. The total human exposure to the given substance is unknown, since the full emissions of the process are unknown (the inventory relates
to the functional unit), as are the environmental background concentrations of the given substance. Compared to the factors developed for terrestrial eutrophication and acidification, the factors for human
toxicity thus cover a shorter part of the cause-impact chain. The present state-of-the-art in integrated assessment modelling of human toxicity does not allow a closer assessment of toxic effect.
To assist interpretation of the exposure estimates, a review is given with a selection of typical situations where background concentrations are near or above no-effect-levels for a number of important air
pollutants (see Annex
8.9). This review provides information to help evaluate whether no-effect-levels are likely to be exceeded by the emission of a given process. Such an evaluation must be very rough, given the limited data
available about background concentrations. Nevertheless, it is a first step in the interpretation for identifying those processes for which concentration increases may exceed no-effect-levels.
8.9 Example
Applying the EDIP2003 factors, characterisation is performed on the inventory presented in Section 1.6.
Site-generic characterisation
As described in Section 8.5, first the site-generic impacts for exposure via air are calculated. The human toxicity impact shown in Table 8.2 is determined using the EDIP97 factors from Annex 8.1 and the
site-generic exposure factors from Table 8.1 according to Equation 8.1. Among the airborne emissions for which EDIP97 factors exist, the metals (which are particle-bound), NOx and carbon moNOxide
are judged to have atmospheric residence times close to benzene (one week). In the characterisation they are therefore represented by the site-generic exposure factors for benzene. The residence time of
SO2 is expected to lie closer to the residence time of HCl (one day), and for SO2, the site-generic exposure factors of HCl are therefore chosen. For HEFregional, a release height of 25m is assumed
because the emissions are of industrial origin.
Table 8.2. Site-generic impact potentials for human toxicity via air exposure for one supporting block made from plastic or zinc. Expressed as area of unprotected ecosystem.
Click here to see the Table
Using site-generic exposure factors, the zinc supporting block has the largest human toxicity impact potentials. For both supporting blocks, SO2, NOx, and lead are important contributors while also the
cadmium emission contributes significantly for the zinc component. However, the potential spatial variation is so large (as revealed by the spatially determined standard deviation) that the conclusion might
change if spatial variation were to be included. Therefore, a site-dependent characterisation is performed for those processes that contribute the most to the site-generic impacts in order to reduce the
spatially determined uncertainty and strengthen the conclusion.
Site-dependent characterisation
Table 8.2 reveals that the predominant contributions to the human toxicity impact via air are caused by SO2, NOx, Pb and (for the zinc component) Cd. For the zinc component, the main sources for SO2
and NOx emissions are identified as the production of zinc from ore which takes place in Bulgaria, the casting of the component which takes place in Yugoslavia and that part of the transport of the
component, which takes place by truck through Germany. Both the lead and zinc emissions are nearly exclusively caused by the production of zinc from ore in Bulgaria (data not shown). For the plastic
component the main sources for SO2 and NOx are found to be the production of plastic polymer in Italy, the flow injection moulding of the supporting block in Denmark, and the transportation of the
component by truck, mainly through Germany. The lead emissions come from the consumption of electricity which takes place at a number of places throughout Europe. For the latter it is thus chosen to
retain the site-generic characterisation (idem). The emissions from the selected processes contribute a good 80% and 95% of the full site-generic impacts of Table 8.2 for the zinc component and the plastic
component respectively (data not shown).
In the calculation of the site-dependent impacts for these key processes, the relevant site-dependent regional exposure factors are read from the maps of Annex 8.5 and annex 8.6. The midpoint of the given
intervals is applied. The local exposure factors are found in Annex 8.7 covering the range up to 10 km distance. The population density in the local area is taken as rural (100 persons/km2). The results of the
site-dependent characterisation are shown in Table 8.3.
Exposure factors for HCl and benzene were used to represent substances of short respectively long residence times in the atmosphere. To check the robustness of the results for the choice of model
substance (HCl or benzene) in the best estimate calculation in Table 8.3, the lower and upper bond due to residence time of the substance is determined. The calculation of the site-dependent impacts is
repeated applying the HCl factors for all emissions (lower bond) and the benzene factors for all emissions (upper bond) (calculation not shown). For all three calculations, the site-generic impacts from the
key processes are subtracted from the original site-generic impacts in Table 8.2 and the site-dependent impacts (in Table 8.3 for the best estimate) are added. The thus corrected human toxicity impacts via
air are found in Table 8.4 and the difference to the original site-generic impacts of Table 8.2 is illustrated in Figure 8.1.
Table 8.3. Site-dependent impact potentials for human toxicity via air for key processes from either product system.
Zinc part |
|
EF(hta) |
HEFregional |
HEFlocal |
PD |
|
Toxic impact EP(hta) |
|
g/f.u. |
m3 air/g |
person•μg/m3/g |
person•μg/m3/g |
|
|
SO2 emissions |
|
|
|
|
|
|
|
Zinc production, Bulgaria |
9,16 |
1,30•103 |
1500 |
0,52 |
|
100 |
61 |
Zinc casting, Yugoslavia |
2,71 |
1,30•103 |
1500 |
0,52 |
|
100 |
18 |
Transport, mainly Germany |
1,18 |
1,30•103 |
3500 |
0,68 |
|
100 |
13 |
NOx emissions |
|
|
|
|
|
|
|
Zinc production, Bulgaria |
0,97 |
8,60•103 |
22500 |
1 |
|
100 |
246 |
Zinc casting, Yugoslavia |
1,65 |
8,60•103 |
22500 |
1 |
|
100 |
418 |
Transport, mainly Germany |
4,56 |
8,60•103 |
22500 |
1,75 |
|
100 |
1361 |
Lead emissions |
|
|
|
|
|
|
|
Zinc production, Bulgaria |
1,75•10-4 |
1,00•108 |
22500 |
1 |
|
100 |
516 |
Cadmium emissions |
|
|
|
|
|
|
|
Zinc production, Bulgaria |
6,50•10-5 |
1,10•108 |
22500 |
1 |
|
100 |
211 |
Total, zinc part |
|
|
|
|
|
|
2843 |
Plastic part |
|
|
|
|
|
|
|
SO2 emissions |
|
|
|
|
|
|
|
Plastic production, Italy |
2,43 |
1,30•103 |
1500 |
0,52 |
|
100 |
16 |
Flow injection moulding, Denmark |
2,11 |
1,30•103 |
1500 |
0,28 |
|
100 |
9 |
Transport, mainly Germany |
0,45 |
1,30•103 |
3500 |
0,68 |
|
100 |
5 |
NOx emissions |
|
|
|
|
|
|
|
Plastic production, Italy |
0,63 |
8,60•103 |
22500 |
1 |
|
100 |
160 |
Flow injection moulding, Denmark |
0,48 |
8,60•103 |
22500 |
0,42 |
|
100 |
105 |
Transport, mainly Germany |
1,74 |
8,60•103 |
22500 |
1,75 |
|
100 |
519 |
|
|
|
|
|
|
|
|
Total, plastic part |
|
|
|
|
|
|
814 |
Site-dependent characterisation reduces the size of the human toxicity impact via air for both components but strengthens the dominance of the zinc component. For the zinc-based component around 75%
of this impact is calculated using site-dependent characterisation factors while the site-dependent share for the plastic-based component is around 85%. Even if the site-dependent characterisation were
performed for all the remaining processes in the product system, the result will thus not change significantly, given their modest share in the total and the standard deviation. The major part of the spatially
conditioned potential for variation of the impact has been cancelled for both components. The upper and lower bonds calculated in Table 8.4 also reveal that the dominance of the zinc component in this
impact category is relatively insensitive to the residence time of the substance involved. Site is more important than residence time within the boundaries of the investigated model substances HCl and
benzene.
Table 8.4. Human toxicity impacts via air from either product system with site-dependent characterisation of key process emissions, best estimate (using exposure factors for HCl and benzene as judged
most appropriate), lower bond (exposure factors for HCl for all emissions) and upper bond (exposure factors for benzene for all emissions).
|
Human toxicity via air, EP(hta) Best estimat |
Human toxicity via air, EP(hta) Lower bond |
Human toxicity via air, EP(hta) Upper bond |
Zinccomponent |
3403 |
1216 |
3819 |
Plastic component |
1291 |
672 |
1443 |
Figure 8.1 Site-generic and site-dependent human toxicity impacts via air from the two product systems. For the site-dependent impacts, the site-dependent exposure factors have only been applied for the
key processes as described above.
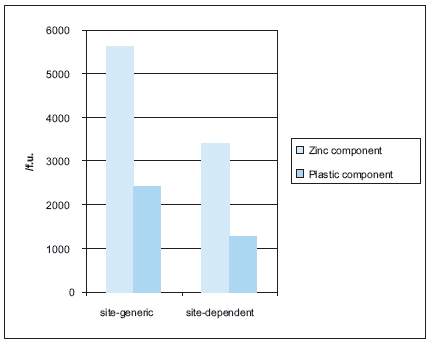
Annex 8.1: EDIP97 characterisation factors for human toxicity assessment for emissions to air (Wenzel et al., 1997)
Emissions to air as first compartment |
Substance | CAS no. | EF(hta)m3/g | EF(htw)m3/g | EF(hts)m3/g |
| | | | |
1.1.1-Trichloroethane | 71-55-6 | 9.2E+02 | 9.9E-04 | 2.0E-03 |
1.2-Benzo-isothiazolin-3-one | 2634-33-5 | 2.8E+04 | 0 | 0 |
1.2-Dichlorobenzene | 95-50-1 | 8.3E+03 | 0.37 | 7.0E-03 |
1.2-Dichloroethane | 107-06-2 | 5.0E+04 | 3.9E-03 | 7.5E-02 |
1.2-Propylen oxide | 75-56-9 | 3.3E+04 | 2.9E-06 | 1.1E-03 |
1-Butanol | 71-36-3 | 1.3E+04 | 1.4E-03 | 1.4E-01 |
2.3.7.8-Tetrachlorodi-benzo-p-dioxin | 1746-01-6 | 2.9E+10 | 2.2E+08 | 1.4E+04 |
2.4-Dinitrotoluene | 121-14-2 | 1.1E+02 | 5.8E-03 | 9.6E-04 |
2-Chlorotoluene | 95-49-8 | 2.2E+03 | 0.98 | 1.9E-02 |
2-Ethyl hexanol | 104-76-7 | 1.8E+03 | 0 | 0 |
2-Ethylhexyl acetate | 103-09-3 | 9.5E+03 | 0 | 0 |
2-Propanol | 67-63-0 | 1.2E+02 | 7.5E-06 | 2.8E-03 |
3-Chlorotoluene | 108-41-8 | 2.2E+03 | 0.71 | 2.4E-02 |
4-Chlorotoluene | 106-43-4 | 2.2E+03 | 0.79 | 2.2E-02 |
Acetaldehyde | 75-07-0 | 3.7E+03 | 0 | 0 |
Acetic acid | 64-19-7 | 1.0E+04 | 3.3E-06 | 1.6E-03 |
Acetone | 67-64-1 | 3.2E+04 | 8.5E-06 | 4.1E-03 |
Acrylic acid | 79-10-7 | 6.7E+05 | 6.3E-05 | 1.6E-02 |
Acrylic acid, 2-hydroxyethyl ester | 818-61-1 | 2,0E+02 | 0 | 0 |
Anthracene | 120-12-7 | 9.5E+02 | 0 | 0 |
Antimony | 7440-36-0 | 2.0E+04 | 64 | 17 |
Arsenic | 7440-38-2 | 9.5E+06 | 7.4 | 1.0E+02 |
Atrazine | 1912-24-9 | 1.4E+05 | 0 | 0 |
Benzene | 71-43-2 | 1.0E+07 | 2.3 | 14 |
Benzo(a)pyrene | 50-32-8 | 5.0E+07 | 0 | 0 |
Benzotriazole | 95-14-7 | 1.3E+03 | 9.3E-04 | 2.0E-02 |
Biphenyl | 92-52-4 | 2.3E+05 | 1.4 | 2.9E-03 |
Butyl diglycol acetate | 124-17-4 | 1.3E+04 | 0 | 0 |
Cadmium | 7440-46-9 | 1.1E+08 | 5.6E+02 | 4.5 |
Carbon monoxide | 630-08-0 | 8.3E+02 | 0 | 0 |
Chlorine | 7782-50-5 | 3.4E+04 | 0 | 0 |
Annex 8.1: EDIP97 characterisation factors for human toxicity assessment for emissions to air (Wenzel et al., 1997)
Emissions to air as first compartment |
Substance |
CAS no. |
EF(hta)m3/g |
EF(htw)m3/g |
EF(hts)m3/g |
|
|
|
|
|
Chlorbenzene |
108-90-7 |
2.2E+05 |
0.27 |
4.6E-02 |
Chloroform |
67-66-3 |
1.0E+05 |
5.4E-02 |
0.20 |
Chromium |
7440-47-3 |
1.0E+06 |
3.6 |
1.1 |
Cobalt |
7440-48-4 |
9.5E+03 |
2.5E-03 |
0.17 |
Copper |
7440-50-8 |
5.7E+02 |
3.4 |
4.0E-03 |
DibutyltiNOxide |
818-08-6 |
1.4E+05 |
3.7E-03 |
4.2E-03 |
Diethanolamine |
111-42-2 |
4.0E+04 |
0 |
0 |
Diethylaminoethanol |
100-37-8 |
2.7E+04 |
0 |
0 |
Diethylene glycol |
111-46-6 |
2.5E+05 |
0 |
0 |
Diethylene glycol mono-n-butyl ether |
112-34-5 |
2.0E+06 |
0 |
0 |
Ethanol |
64-17-5 |
1.1E+02 |
2.9E-07 |
1.5E-04 |
Ethyl acetate |
141-78-6 |
6.9E+02 |
8.9E-06 |
1.2E-03 |
Ethylene glycol |
107-21-1 |
8.3E+05 |
1.4E-03 |
2.0E-05 |
Ethylene glycol acetate |
111-15-9 |
3.7E+03 |
0 |
0 |
Ethylene glycol mono-n-butyl ether |
111-76-2 |
2.1E+04 |
0 |
0 |
Ethylenediamine tetraacetic acid, EDTA |
60-00-4 |
3.7E+02 |
0 |
0 |
Ethylenediamin, 1,2-ethanediamine |
107-15-3 |
2.0E+04 |
0 |
0 |
Fluoride |
16984-48-8 |
9.5E+04 |
0 |
0 |
Formaldehyde |
50-00-00 |
1.3E+07 |
2.2E-05 |
5.8E-03 |
Glycerol |
56-81-5 |
70 |
0 |
0 |
Hexamethylene diisocyanate, HDI |
822-06-0 |
7.1E+05 |
12 |
0.56 |
Hexane |
110-54-3 |
1,6E+03 |
0.34 |
9.7E-04 |
Hydrogen cyanide |
74-90-8 |
1.4E+05 |
1.5E-03 |
0.71 |
Hydrogene sulphide |
7783-06-04 |
1.1E+06 |
8.1E-04 |
0.26 |
Iron |
7439-89-6 |
3.7E+04 |
9.6E-03 |
0.77 |
Isobutanol |
78-83-1 |
1.0E+07 |
2.8E-05 |
3.7E-03 |
Isopropylbenzene, cumene |
98-82-8 |
1.0E+04 |
0.21 |
2.1E-02 |
Lead |
7439-92-1 |
1.0E+08 |
53 |
8.3E-02 |
Maleic acid, dibutyl ester |
105-76-0 |
7.7E+03 |
0 |
0 |
Manganese |
7439-96-5 |
2.5E+06 |
5.3E-03 |
0.42 |
Annex 8.1: EDIP97 characterisation factors for human toxicity assessment for emissions to air (Wenzel et al., 1997)
Emissions to air as first compartment |
Substance |
CAS no. |
EF(hta)m3/g |
EF(htw)m3/g |
EF(hts)m3/g |
|
|
|
|
|
Mercury |
7439-97-6 |
6.7E+06 |
1.1E+05 |
81 |
Methacrylic acid |
79-41-4 |
4.5E+04 |
0 |
0 |
Methanol |
67-56-1 |
2.5E+03 |
3.0E-04 |
3.1E-04 |
Methyl isobutyl ketone |
108-10-1 |
3.3E+03 |
3.6E-03 |
0.12 |
Methyl methacrylate |
80-62-6 |
1.0E+07 |
0 |
0 |
Methylenebis (4-phenylisocyanate), MDI |
101-68-8 |
5.0E+07 |
0 |
0 |
Molybedum |
7439-98-7 |
1.0E+05 |
5.3E-02 |
1.5 |
Monoethanolamin |
141-43-5 |
2.7E+04 |
0 |
0 |
Morpholine |
110-91-8 |
1.3E+04 |
0 |
0 |
n-Butyl acetate |
123-86-4 |
1.1E+03 |
7.0E-03 |
5.0E-02 |
Nickel |
7440-02-0 |
6.7E+04 |
3.7E-03 |
0.12 |
Nitrilotriacetate |
139-13-9 |
3.8E+04 |
0 |
0 |
Nitrobenzenesulpho nic acid, sodium salt |
127-68-4 |
2.6E+03 |
1.7E-07 |
3.9E-05 |
Nitrogen dioxide and other NOx |
10102-44-0 |
8.6E+03 |
0 |
0 |
Nitrous oxide, N2O |
10024-97-2 |
2.0E+03 |
0 |
0 |
Ozone |
10028-15-6 |
5.0E+04 |
0 |
0 |
Phenol |
108-95-2 |
1.4E+06 |
0 |
0 |
Phosgene |
75-44-5 |
2.0E+06 |
0 |
0 |
Propylene glycol, 1,2-propanediol |
57-55-6 |
1.5E+03 |
0 |
0 |
Selenium |
7782-49-2 |
1.5E+06 |
28 |
4.4E-02 |
Silver |
7440-22-4 |
2.0E+05 |
5.3E-02 |
4.2 |
Sodium benzoate |
532-32-10 |
1.4E+04 |
4.0E-07 |
1.4E-04 |
Sodium hypochlorite |
7681-52-9 |
2.0E+03 |
0 |
0 |
Styrene |
100-42-5 |
1.0E+03 |
0 |
0 |
Sulphamic acid |
5329-14-6 |
9.0E+03 |
2.1E-09 |
9.7E-06 |
Sulphur dioxide |
7446-09-5 |
1.3E+03 |
0 |
0 |
Tetrachlorethylene |
127-18-4 |
2.9E+04 |
0.36 |
4.0E-02 |
Thallium |
7440-28-0 |
5.0E+05 |
1.3E+04 |
10 |
Titanium |
7440-32-6 |
1.8E+04 |
4.7E-03 |
0.38 |
Annex 8.1: EDIP97 characterisation factors for human toxicity assessment for emissions to air (Wenzel et al., 1997)
Emissions to air as first compartment |
Substance |
CAS no. |
EF(hta)m3/g |
EF(htw)m3/g |
EF(hts)m3/g |
|
|
|
|
|
Toluene |
108-88-3 |
2.5E+03 |
4.0E-03 |
1.0E-03 |
Toluene diisocyanate 2.4/2.6 mixture |
26471-62-5 |
7.1E+05 |
2.1 |
1.2E-02 |
Toluene-2.4-diamine |
95-80-7 |
1.4E+03 |
0 |
0 |
Trichloroethylene |
79-01-6 |
1.9E+04 |
9.1E-04 |
6.9E-04 |
Triethanolamine |
102-71-6 |
1.3E+04 |
0 |
0 |
Triethylamine |
121-44-8 |
1.4E+05 |
0 |
0 |
Vanadium |
7440-62-2 |
1.4E+05 |
3.7E-02 |
0.96 |
Vinylchloride |
75-01-4 |
3.9E+05 |
0.40 |
4.0 |
Xylenes, mixed |
1330-20-7 |
6.7E+03 |
1.1E-03 |
6.7E-05 |
Zinc (as dust) |
7440-66-6 |
8.1E+04 |
4.1 |
1.3E-02 |
Annex 8.2: EDIP97 characterisation factors for human toxicity assessment for emissions to water (Wenzel et al., 1997)
Emissions to water as first
compartment |
Substance |
CAS no. |
EF(hta) |
EF(htw) |
EF(hts) |
|
|
m3/g |
m 3/g |
3m/g |
|
|
|
|
|
1,1,1-Trichloroethane |
71-55-6 |
9.2E+02 |
9.9E-04 |
2.0E-03 |
1,2-Benzoiso thiazolin-3-one |
2634-33-5 |
0 |
1.3E-04 |
0 |
1,2-Dichlorobenzene |
95-50-1 |
8.3E+03 |
0.37 |
7.0E-03 |
1,2-Dichloroethane |
107-06-2 |
0 |
2.0E-02 |
0 |
1,2-Propylene oxide |
75-56-9 |
0 |
1.5E-05 |
0 |
1-Butanol |
71-36-3 |
0 |
7.1E-03 |
0 |
2.3.7.8-Tetrachloro dibenzo-p-dioxin |
1746-01-6 |
0 |
1.1E+09 |
0 |
2,4-Dinitrotoluene |
121-14-2 |
0 |
2.9E-02 |
0 |
2-Chlorotoluene |
95-49-8 |
2.2E+03 |
0.98 |
1.9E-02 |
2-Ethyl hexanol |
104-76-7 |
0 |
2.8E-02 |
0 |
2-Ethylhexyl acetate |
103-09-3 |
9.5E+03 |
0 |
0 |
2-Propanol |
67-63-0 |
0 |
3.7E-05 |
0 |
3-Chlorotoluene |
108-41-8 |
2.2E+03 |
0.71 |
2.4E-02 |
4-Chlorotoluene |
106-43-4 |
2.2E+03 |
0.79 |
2.2E-02 |
Acetaldehyde |
75-07-0 |
0 |
7.1E-06 |
0 |
Acetic acid |
64-19-7 |
0 |
1.6E-05 |
0 |
Acetone |
67-64-1 |
0 |
4.3E-05 |
0 |
Acrylic acid |
79-10-7 |
0 |
3.1E-04 |
0 |
Acrylic acid, 2-hydroxyethyl ester |
818-61-1 |
0 |
6.4E-04 |
0 |
Anthracene |
120-12-7 |
0 |
11 |
0 |
Antimony |
7440-36-0 |
0 |
3.2E+02 |
0 |
Arsenic |
7440-38-2 |
0 |
37 |
0 |
Atrazine |
1912-24-9 |
0 |
1.1 |
0 |
Benzene |
71-43-2 |
1.0E+07 |
2.3 |
14 |
Benzo(a)pyrene |
50-32-8 |
0 |
3.2E+02 |
0 |
Benzotriazole |
95-14-7 |
0 |
4.6E-03 |
0 |
Biphenyl |
92-52-4 |
0 |
7.1 |
0 |
Butyl diglycol acetate |
124-17-4 |
0 |
3.3E-02 |
0 |
Cadmium |
7440-46-9 |
0 |
2.8E+03 |
0 |
Carbon monoxide |
630-08-0 |
8.3E+02 |
0 |
0 |
Chlorine |
7782-50-5 |
3.4E+04 |
0 |
0 |
Chlorobenzene |
108-90-7 |
2.2E+05 |
0.27 |
4.6E-02 |
Chloroform |
67-66-3 |
1.0E+05 |
5.4E-02 |
0.20 |
Annex 8.2: EDIP97 characterisation factors for human toxicity assessment for emissions to water (Wenzel et al., 1997)
Emissions to water as first
compartment |
Substance |
CAS no. |
EF(hta) |
EF(htw) |
EF(hts) |
|
|
m3/g |
3m/g |
m3/g |
|
|
|
|
|
Chromium |
7440-47-3 |
0 |
18 |
0 |
Cobalt |
7440-48-4 |
0 |
1.2E-02 |
0 |
Copper |
7440-50-8 |
0 |
17 |
0 |
DibutyltiNOxide |
818-08-6 |
0 |
1.9E-02 |
0 |
Diethanolamine |
111-42-2 |
0 |
3.9E-05 |
0 |
Diethylaminoethanol |
100-37-8 |
0 |
3.2E-03 |
0 |
Diethylene glycol |
111-46-6 |
0 |
3.1E-06 |
0 |
Diethylene glycol mono-n-butyl ether |
112-34-5 |
0 |
3.4E-03 |
0 |
Ethanol |
64-17-5 |
0 |
1.5E-06 |
0 |
Ethyl acetate |
141-78-6 |
0 |
4.4E-05 |
0 |
Ethylene glycol |
107-21-1 |
0 |
7.0E-03 |
0 |
Ethylene glycol acetate |
111-15-9 |
0 |
1.5E-03 |
0 |
Ethylene glycol mono-n-butyl ether |
111-76-2 |
0 |
8.4E-05 |
0 |
Ethylenediamine tetraacetic acid, EDTA |
60-00-4 |
0 |
6.7E-09 |
0 |
Ethylenediamine,1.2-ethanediamine |
107-15-3 |
0 |
1.4E-05 |
0 |
Fluoride |
16984-48-8 |
0 |
1.2E-02 |
0 |
Formaldehyde |
50-00-00 |
0 |
1.1E-04 |
0 |
Glycerol |
56-81-5 |
0 |
1.3E-06 |
0 |
Hexamethylene diisocyanate, HDI |
822-06-0 |
0 |
61 |
0 |
Hexane |
110-54-3 |
1.6E+03 |
0.34 |
9.7E-04 |
Hydrogen cyanide |
74-90-8 |
1.4E+05 |
1.5E-03 |
0.71 |
Hydrogen sulphide |
7783-06-04 |
0 |
4.1E-03 |
0 |
Iron |
7439-89-6 |
0 |
4.8E-02 |
0 |
Isobutanol |
78-83-1 |
0 |
1.5E-05 |
0 |
Isopropylbenzene, cumene |
98-82-8 |
1.0E+04 |
0.21 |
2.1E-02 |
Lead |
7439-92-1 |
0 |
2.6E+02 |
0 |
Maleic acid, dibutyl ester |
105-76-0 |
0 |
14 |
0 |
Manganese |
7439-96-5 |
0 |
2.7E-02 |
0 |
Mercury |
7439-97-6 |
6.7E+06 |
1.1E+05 |
81 |
Methacrylic acid |
79-41-4 |
0 |
6.0E-03 |
0 |
Annex 8.2: EDIP97 characterisation factors for human toxicity assessment for emissions to water (Wenzel et al., 1997)
Emissions to water as first
compartment |
Substance |
CAS no. |
EF(hta) |
EF(htw) |
EF(hts) |
|
|
m3/g |
3m/g |
m3/g |
|
|
|
|
|
Methanol |
67-56-1 |
0 |
1.5E-03 |
0 |
Methyl isobutyl ketone |
108-10-1 |
0 |
1.8E-02 |
0 |
Methyl methacrylate |
80-62-6 |
0 |
4.9E-03 |
0 |
Methylenebis(4-phenylisocyanate), MDI |
101-68-8 |
0 |
2.8E+02 |
0 |
Molybdenum |
7439-98-7 |
0 |
0.27 |
0 |
Monoethanolamine |
141-43-5 |
0 |
3.5E-05 |
0 |
Morpholine |
110-91-8 |
0 |
1.0E-04 |
0 |
n-Butyl acetate |
123-86-4 |
0 |
3.5E-02 |
0 |
Nickel |
7440-02-0 |
0 |
1.9E-02 |
0 |
Nitrilotriacetate |
139-13-9 |
0 |
8.2E-14 |
0 |
Nitrobenzenesulpho nic acid, sodium salt |
127-68-4 |
2.6E+03 |
1.7E-07 |
3.9E-05 |
Nitrogen dioxide and other NOx |
10102-44-0 |
0 |
3.7E-05 |
0 |
Nitrious oxide, N2O10024-97-2 |
|
2.0E+03 |
0 |
0 |
Ozone |
10028-15-6 |
5.0E+04 |
0 |
0 |
Phenol |
108-95-2 |
0 |
3.4E-02 |
0 |
Phosgene |
75-44-5 |
2.0E+06 |
0 |
0 |
Propylene glycol,1.2-propanediol |
57-55-6 |
0 |
4.8E-06 |
0 |
Selenium |
7782-49-2 |
0 |
1.4E+02 |
0 |
Silver |
7440-22-4 |
0 |
0.27 |
0 |
Sodium benzoate |
532-32-10 |
0 |
2.0E-06 |
0 |
Sodium hypochlorite |
7681-52-9 |
0 |
2.6E-04 |
0 |
Styrene |
100-42-5 |
1.0E+03 |
0 |
0 |
Sulphamic acid |
5329-14-6 |
0 |
1.1E-08 |
0 |
Sulphur dioxide |
7446-09-5 |
1.3E+03 |
0 |
0 |
Tetrachlorethylene |
127-18-4 |
2.9E+04 |
0.36 |
4.0E-02 |
Thallium |
7440-28-0 |
0 |
6.5E+04 |
0 |
Titanium |
7440-32-6 |
0 |
0.02 |
0 |
Toluene |
108-88-3 |
2.5E+03 |
4.0E-03 |
1.0E-03 |
Toluene diisocyanate 2.4/2.6 mixture |
26471-62-5 |
0 |
10 |
0 |
Toluene-2.4-diamine |
95-80-7 |
0 |
1.3E-04 |
0 |
Trichloroethylene |
79-01-6 |
1.9E+04 |
9.1E-04 |
6.9E-04 |
Annex 8.2: EDIP97 characterisation factors for human toxicity assessment for emissions to water (Wenzel et al., 1997)
Emissions to water as first
compartment |
Substance |
CAS no. |
EF(hta) |
EF(htw) |
EF(hts) |
|
|
m3/g |
3m/g |
m3/g |
|
|
|
|
|
Triethanolamine |
102-71-6 |
0 |
8.4E-05 |
0 |
Triethylamine |
121-44-8 |
0 |
0.23 |
0 |
Vanadium |
7440-62-2 |
0 |
0.19 |
0 |
Vinylchloride |
75-01-4 |
3.9E+05 |
0.40 |
4.0 |
Xylenes, mixed |
1330-20-7 |
6.7E+03 |
1.1E-03 |
6.7E-05 |
Zinc (as dust) |
7440-66-6 |
0 |
21 |
0 |
Annex 8.3: EDIP97 characterisation factors for human toxicity assessment for emissions to soil (Wenzel et al., 1997)
Emissions to soil as first compartment |
Substance |
CAS no. |
EF(hta) |
EF(htw) |
EF(hts) |
|
|
m3/g |
3m/g |
3m/g |
|
|
|
|
|
1.1.1-Trichloroethane |
71-55-6 |
9.2E+02 |
9.9E-04 |
2.0E-03 |
1.2-Benzoiso thiazolin-3-one |
2634-33-5 |
0 |
0 |
0.32 |
1.2-Dichlorobenzene |
95-50-1 |
8.3E+03 |
0.37 |
7.0E-03 |
1.2-Dichloroethane |
107-06-2 |
0 |
0 |
9.4E-02 |
1.2-Propylene oxide |
75-56-9 |
0 |
0 |
1.4E-03 |
1-Butanol |
71-36-3 |
0 |
0 |
0.18 |
2.3.7.8-Tetrachloro dibenzo-p-dioxin |
1746-01-6 |
0 |
0 |
1.8E+04 |
2.4-Dinitrotoluene |
121-14-2 |
0 |
0 |
1.2E-03 |
2-Chlorotoluene |
95-49-8 |
2.2E+03 |
0.98 |
1.9E-02 |
2-Ethyl hexanol |
104-76-7 |
0 |
0 |
1.5E-03 |
2-Ethylhexyl acetate |
103-09-3 |
9.5E+03 |
0 |
0 |
2-Propanol |
67-63-0 |
0 |
0 |
3.5E-03 |
3-Chlorotoluene |
108-41-8 |
2.2E+03 |
0.71 |
2.4E-02 |
4-Chlorotoluene |
106-43-4 |
2.2E+03 |
0.79 |
2.2E-02 |
Acetaldehyde |
75-07-0 |
0 |
0 |
9.2E-04 |
Acetic acid |
64-19-7 |
0 |
0 |
2.0E-03 |
Acetone |
67-64-1 |
0 |
0 |
5.2E-03 |
Acrylic acid |
79-10-7 |
0 |
0 |
2.0E-02 |
Acrylic acid, 2-hydroxyethyl ester |
818-61-1 |
0 |
0 |
7.6E-02 |
Anthracene |
120-12-7 |
0 |
0 |
1.1E-04 |
Antimony |
7440-36-0 |
0 |
0 |
21 |
Arsenic |
7440-38-2 |
0 |
0 |
1.3E+02 |
Atrazine |
1912-24-9 |
0 |
0 |
4.2E-02 |
Benzene |
71-43-2 |
1.0E+07 |
2,3 |
14 |
Benzo(a)pyrene |
50-32-8 |
0 |
0 |
1.8E-03 |
Benzotriazole |
95-14-7 |
0 |
0 |
2.5E-02 |
Biphenyl |
92-52-4 |
0 |
0 |
3.6E-03 |
Butyl diglycol acetate |
124-17-4 |
0 |
0 |
0.27 |
Cadmium |
7440-46-9 |
0 |
0 |
5.6 |
Carbon moNOxide |
630-08-0 |
8.3E+02 |
0 |
0 |
Annex 8.3: EDIP97 characterisation factors for human toxicity assessment for emissions to soil (Wenzel et al., 1997)
Emissions to soil as compartment
first |
Substance |
CAS no. |
EF(hta) |
EF(htw) |
EF(hts) |
|
|
m3/g |
3m/g |
3m/g |
|
|
|
|
|
Chlorine |
7782-50-5 |
3.4E+04 |
0 |
0 |
Chlorobenzene |
108-90-7 |
2.2E+05 |
0.27 |
4.6E-02 |
Chloroform |
67-66-3 |
1.0E+05 |
5.4E-02 |
0.20 |
Chromium |
7440-47-3 |
0 |
0 |
1.4 |
Cobalt |
7440-48-4 |
0 |
0 |
0.21 |
Copper |
7440-50-8 |
0 |
0 |
5.0E-03 |
DibutyltiNOxide |
818-08-6 |
0 |
0 |
5.3E-03 |
Diethanolamine |
111-42-2 |
0 |
0 |
5.9E-03 |
Diethylaminoethanol |
100-37-8 |
0 |
0 |
0.30 |
Diethylene glycol |
111-46-6 |
0 |
0 |
4.7E-04 |
Diethylene glycol mono-n-butyl ether |
112-34-5 |
0 |
0 |
0.16 |
Ethanol |
64-17-5 |
0 |
0 |
1.8E-04 |
Ethyl acetate |
141-78-6 |
0 |
0 |
1.5E-03 |
Ethylene glycol |
107-21-1 |
0 |
0 |
2.5E-05 |
Ethylene glycol acetate |
111-15-9 |
0 |
0 |
6.6E-02 |
Ethylene glycol mono-n-butyl ether |
111-76-2 |
0 |
0 |
3.5E-03 |
Ethylenediamine tetraacetic acid, EDTA |
60-00-4 |
0 |
0 |
2.5E-06 |
Ethylenediamine, 1,2-ethanediamine |
107-15-3 |
0 |
0 |
1.5E-03 |
Fluoride |
16984-48-8 |
0 |
0 |
6.4E-04 |
Formaldehyde |
50-00-00 |
0 |
0 |
7.2E-03 |
Glycerol |
56-81-5 |
0 |
0 |
1.7E-04 |
Hexamethylene diisocyanate, HDI |
822-06-0 |
0 |
0 |
0.70 |
Hexane |
110-54-3 |
1.6E+03 |
0.34 |
9.7E-04 |
Hydrogen cyanide74-90-8 |
|
1.4E+05 |
1.5E-03 |
0.71 |
Hydrogene sulphide |
7783-06-04 |
1.1E+06 |
0 |
0 |
Iron |
7439-89-6 |
0 |
0 |
0.96 |
Isobutanol |
78-83-1 |
0 |
0 |
4.6E-03 |
Isopropylbenzene, cumene |
98-82-8 |
1.0E+04 |
0.21 |
2.1E-02 |
Lead |
7439-92-1 |
0 |
0 |
0.10 |
Annex 8.3: EDIP97 characterisation factors for human toxicity assessment for emissions to soil (Wenzel et al., 1997)
Emissions to soil as compartment
first |
Substance |
CAS no. |
EF(hta) |
EF(htw) |
EF(hts) |
|
|
m3/g |
3m/g |
3m/g |
|
|
|
|
|
Maleic acid, dibutyl ester |
105-76-0 |
0 |
0 |
3.4E-03 |
Manganese |
7439-96-5 |
0 |
0 |
0.53 |
Mercury |
7439-97-6 |
6.7E+06 |
1.1E+05 |
81 |
Methacrylic acid |
79-41-4 |
0 |
0 |
0.22 |
Methanol |
67-56-1 |
0 |
0 |
3.9E-04 |
Methyl isobutyl ketone |
108-10-1 |
0 |
0 |
0.15 |
Methyl methacrylate |
80-62-6 |
0 |
0 |
3.2E-02 |
Methylenebis(4-phenylisocyanate), MDI |
101-68-8 |
0 |
0 |
4.0E-04 |
Molybdenum |
7439-98-7 |
0 |
0 |
1.9 |
Monoethanolamine |
141-43-5 |
0 |
0 |
5.4E-03 |
Morpholine |
110-91-8 |
0 |
0 |
1.6E-02 |
n-Butyl acetate |
123-86-4 |
0 |
0 |
6.2E-02 |
Nickel |
7440-02-0 |
0 |
0 |
0.15 |
Nitrilotriacetate |
139-13-9 |
0 |
0 |
5.1E-05 |
Nitrobenzenesulphonic acid, sodium salt |
127-68-4 |
2.6E+03 |
1.7E-07 |
3.9E-05 |
Nitrogen dioxide and other NOx |
10102-44-0 |
0 |
0 |
3.7E-03 |
Nitrous oxide |
10024-97-2 |
2.0E+03 |
0 |
0 |
Ozone |
10028-15-6 |
5.0E+04 |
0 |
0 |
Phenol |
108-95-2 |
0 |
0 |
6.4E-05 |
Phosgene |
75-44-5 |
2,0E+06 |
0 |
0 |
Propylene glycol,1.2-propanediol |
57-55-6 |
0 |
0 |
7.7E-04 |
Selenium |
7782-49-2 |
0 |
0 |
5.5E-02 |
Silver |
7440-22-4 |
0 |
0 |
5.3 |
Sodium benzoate |
532-32-10 |
0 |
0 |
1.7E-04 |
Sodium hypochlorite |
7681-52-9 |
0 |
0 |
2.5E-02 |
Styrene |
100-42-5 |
1.0E+03 |
0 |
0 |
Sulphamic acid |
5329-14-6 |
0 |
0 |
1.2E-05 |
Sulphur dioxide |
7446-09-5 |
1.3E+03 |
0 |
0 |
Annex 8.3: EDIP97 characterisation factors for human toxicity assessment for emissions to soil (Wenzel et al., 1997)
Emissions to soil as compartment
first |
Substance |
CAS no. |
EF(hta) |
EF(htw) |
EF(hts) |
|
|
m3/g |
3m/g |
3m/g |
|
|
|
|
|
Tetrachlorethylene |
127-18-4 |
2.9E+04 |
0.36 |
4.0E-02 |
Thallium |
7440-28-0 |
0 |
0 |
13 |
Titanium |
7440-32-6 |
0 |
0 |
0,47 |
Toluene |
108-88-3 |
2.5E+03 |
4.0E-03 |
1.0E-03 |
Toluene diisocyanate 2.4/2.6 mixture |
26471-62-5 |
0 |
0 |
1.5E-02 |
Toluene-2.4-diamine |
95-80-7 |
0 |
0 |
1.1E-02 |
Trichloroethylene |
79-01-6 |
1.9E+04 |
9.1E-04 |
6.9E-04 |
Triethanolamine |
102-71-6 |
0 |
0 |
1.4E-02 |
Triethylamine |
121-44-8 |
0 |
0 |
1.2 |
Vanadium |
7440-62-2 |
0 |
0 |
1.2 |
Vinylchloride |
75-01-4 |
3.9E+05 |
0.40 |
4.0 |
Xylenes, mixed |
1330-20-7 |
6.7E+03 |
1.1E-03 |
6.7E-05 |
Zinc (as dust) |
7440-66-6 |
0 |
0 |
1.6E-02 |
Annex 8.4: Populations densities spatially resolved over Europe (Tobler et al. 1995)
Estimate of population densities for 1994 from Tobler et al. (1995). Locations of the Northern, Central, Southern European and maritime sites are indicated with capital letters.
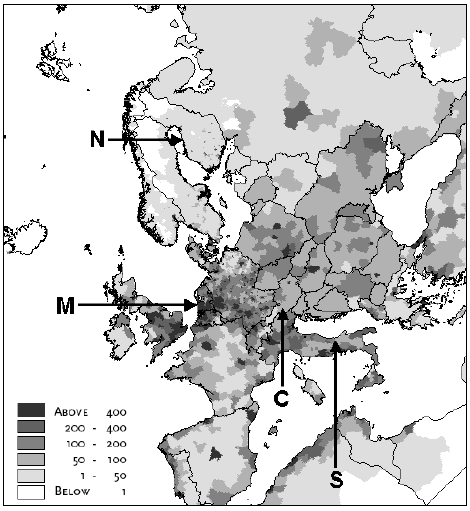
Annex 8.5: Regional exposure to hydrogen chloride
The regional exposure (in person•μg•m-3) over the total receiving area (from 10km to several hundred to thousand kilometres) caused by the release of one gram hydrogen chloride gas at a height of 25m in
the source grid-square. The mean exposure is 2460 person•μg•m-3 per gram emitted, and the standard deviation is 1600 person•μg•m-3 (both weighted for population density). The exposure caused by a
similar emission released at a height of 150m can be obtained by multiplying with a factor 1.30 (stdev. 0.02). The exposure caused by a release at 1m can be obtained by multiplying with a factor 0.89 (stdev. 0.04). The large capitals in the figure indicate the point for which local exposures in Annex 8.7 have been calculated.
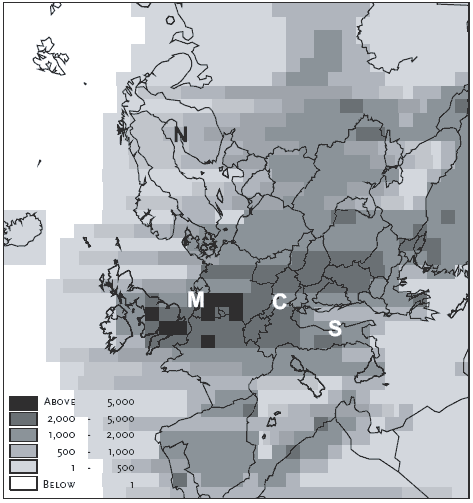
Annex 8.6: Regional exposure to benzene
The regional exposure (in person•μg•m-3) over the total receiving area (from 10km to several hundred to thousand kilometres) caused by a release of one gram benzene at a height of 25m in the source
grid-square. The mean exposure is 50000 person•μg•m-3 per gram emitted, and the standard deviation is 33000 person•μg•m-3 (both weighted for population density). The exposure increase is extrapolated
outside the European grid to cover transport distances up to the level where all benzene is removed from the atmosphere (see Potting et al., 2005b). The height of release hardly influences the resulting
exposure due to the long lifetime of benzene, and therefore no calculations are made for the other release heights of benzene. The large capitals in the figure indicate the points for which local exposures in
Annex 8.7 have been calculated.
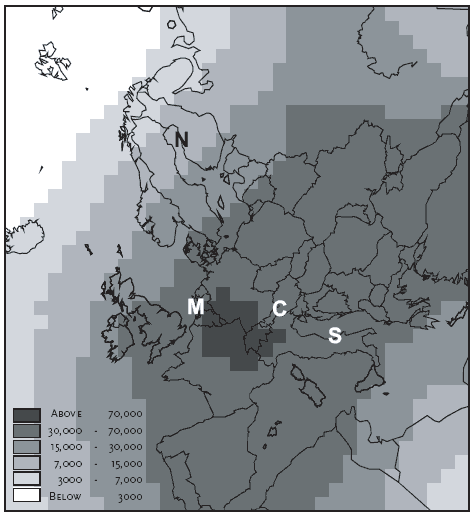
Annex 8.7: Local exposure to hydrogen chloride and benzene
The table shows the exposure from an emission of one gram of benzene and hydrogen chloride local to the source (0-10km) and released at different heights (1m, 25m and 150m) and in different climate
regions in Europe. Also given are the exposures at smaller distances from the source (0.5km, 5km and 10km). The exposures are expressed as a proportion of the accumulated benzene exposure at 10km
distance (20•20km2) resulting from a release at a height of 25m in South Europe (69.7 person•μg•m-3). The population density is in all cases one person•km-2. The locations chosen to represent the four
European regions in the table are indicated on the maps of Annex 8.5 and 8.6.
| |
Benzene |
|
Hydrogen chloride |
|
|
|
|
0.5km |
5km |
10km |
|
0.5km |
5km |
10km |
|
|
R |
150m |
|
0.02 |
0.05 |
Maritime |
Similar as for benzene |
150m |
R |
E |
|
|
0.02 |
0.04 |
North europe |
|
E |
L |
|
|
0.03 |
0.07 |
Central europe |
|
L |
E |
|
|
0.04 |
0.08 |
South europe |
|
E |
A |
25m |
0.02 |
0.2 |
0.31 |
Maritime |
|
0.16 |
0.23 |
25m |
A |
S |
|
0.03 |
0.25 |
0.42 |
North europe |
|
0.2 |
0.28 |
|
S |
EH |
|
0.04 |
0.53 |
0.93 |
Central europe |
|
0.36 |
0.5 |
|
EH |
E |
|
0.04 |
0.57 |
1 |
South europe |
|
0.38 |
0.52 |
|
E |
I |
1m |
0.24 |
0.49 |
0.59 |
Maritime |
0.2 |
0.33 |
0.37 |
1m |
I |
G |
|
0.25 |
0.53 |
0.67 |
North europe |
0.2 |
0.33 |
0.38 |
|
G |
H |
|
0.68 |
1.41 |
1.75 |
Central europe |
0.45 |
0.63 |
0.68 |
|
H |
T |
|
0.75 |
1.55 |
1.91 |
South europe |
0.47 |
0.63 |
0.67 |
|
T |
Annex 8.8: EDIP2003 normalisation reference for human toxicity via air
Based on national emission inventories for a number of European countries provided by Christensen, 2005, a European normalisation reference is calculated for human toxicity via air applying the EDIP2003
exposure factors and the EDIP97 characterisation factors according to Equation 8.2 for site-dependent human toxicity impact.

A number of assumptions have been made:
1. In the absense of a complete set of national emission inventories for the EU countries, the normalisation reference has been based on inventories which cover relatively few priority emissions and which
have been available for 11 European countries. The inclusion of the missing EU countries is not expected to change the resulting European normalisation reference significantly.
2. For every substance in the national emission inventory, it has been decided whether the atmospheric residence time is best represented using hydrogen chloride or benzene as model compound.
3. The regional site-dependent exposure factor (sd-HEFregional(h)s,i) has been assumed constant for the whole emission country and determined as the midpoint of the interval which covers the largest part
of the country in the maps in Annex 8.5 and Annex 8.6 (for hydrogen chloride like and benzene like substances respectively).
4. For nearly all substances in the national emission inventories, it has been assumed that the emission source is industrial and that the emission height is 25 m. For the emissions of NOx and PM10, the main
emission source has been assumed to be transport processes where an emission height of 1 m is more appropriate. For the HCl-like emissions the exposure factors given in Annex 8.5 are multiplied by a
factor 0.89. For benzene-like substances no the exposure is only insignificantly influenced by the emission height. The influence of the assumed emission height on the normalisation reference is modest.
5. The site-generic local exposure factor (sg-HEFlocal(h)s,i) is determined from Annex 8.7 where it is tabulated for benzene-like and HCl-like substances as a function of European region and emission
height. The site-dependent local exposure factor is found by multiplying by the population density (PDi) in the surroundings of the emission point. In the lack of such specific information for the individual
emissions behind the national emission
inventories, the average population densities of the respective countries have been assumed (varying from 20 persons/km2 in Norway to 456 persons/km2 in the Netherlands). Particularly for the short-lived
HCl-like substances this may be a significant source of error.
The total impact for Europe as well as for the 11 individual countries is calculated in the the table below and the person equivalent is calculated using the size of the population in the 11 countries together.
|
|
|
|
Austria |
|
Denmark |
|
|
Main source |
Benzene orHCl |
Char. factor |
Emission |
Impact potential |
Emission |
Impact potential |
|
|
|
(EDIP97) |
1994 |
sd-EP(HTA) |
1994 |
sd-EP(HTA) |
|
|
|
EF(hta) |
ton/Year |
|
ton/year |
|
Total impact (/year) |
|
|
|
|
4.48E+14 |
|
3.96E+14 |
|
|
|
|
|
|
|
|
Population (persons) |
|
|
|
|
8.00E+06 |
|
5.13E+06 |
|
|
|
|
|
|
|
|
Normalisation reference(/year/person) |
|
|
|
|
5.60E+07 |
|
7.71E+07 |
Substance |
|
|
|
|
|
|
|
SO2 |
industry |
Benzene |
1.30E+03 |
5.49E+04 |
4.01E+12 |
1.58E+05 |
1.10E+13 |
NOx |
transport |
HCl |
8.60E+03 |
1.71E+05 |
1.13E+13 |
2.76E+05 |
1.08E+13 |
N2O |
industry |
Benzene |
2.00E+03 |
1.27E+04 |
1.43E+12 |
1.22E+04 |
1.31E+12 |
CO |
industry |
Benzene |
8.30E+02 |
1.18E+06 |
5.51E+13 |
7.15E+05 |
3.18E+13 |
nmVOC |
industry |
HCl |
1.00E+04 |
2.90E+05 |
1.99E+13 |
1.54E+05 |
5.95E+12 |
Cd |
industry |
Benzene |
1.10E+08 |
2.72E+00 |
1.68E+13 |
1.19E+00 |
7.01E+12 |
As |
industry |
Benzene |
9.50E+06 |
3.26E+00 |
1.74E+12 |
7.42E-01 |
3.77E+11 |
Cr(VI) |
industry |
Benzene |
1.00E+06 |
6.62E+00 |
3.72E+11 |
3.49E+00 |
1.87E+11 |
Hg |
industry |
Benzene |
6.70E+06 |
2.18E+00 |
8.21E+11 |
7.58E+00 |
2.72E+12 |
Ni |
industry |
Benzene |
6.70E+04 |
3.55E+01 |
1.34E+11 |
2.21E+01 |
7.93E+10 |
Pb |
industry |
Benzene |
1.00E+08 |
2.43E+01 |
1.37E+14 |
3.95E+01 |
2.11E+14 |
Se |
industry |
Benzene |
1.50E+06 |
4.71E+00 |
3.97E+11 |
1.32E-01 |
1.06E+10 |
Cu |
industry |
Benzene |
5.70E+02 |
9.24E+00 |
2.96E+08 |
1.06E+01 |
3,24E+08 |
Zn |
industry |
Benzene |
8.10E+04 |
2.08E+02 |
9.47E+11 |
1.18E+02 |
5,12E+11 |
Formaldehyde |
industry |
HCl |
1.30E+07 |
|
0.00E+00 |
|
0.00E+00 |
Benzene |
industry |
Benzene |
1.00E+07 |
|
0.00E+00 |
7.33E+01 |
3.92E+13 |
Phenol |
industry |
HCl |
1.40E+06 |
|
0.00E+00 |
|
0.00E+00 |
Styrene |
industry |
HCl |
1.00E+03 |
|
0.00E+00 |
|
0.00E+00 |
Toluene |
industry |
HCl |
2.50E+03 |
|
0.00E+00 |
1.84E+02 |
1.78E+09 |
Xylenes |
industry |
HCl |
6.70E+03 |
|
0.00E+00 |
7.33E+01 |
1.90E+09 |
PAH |
industry |
Benzene |
5.00E+07 |
4.58E+02 |
1.29E+15 |
3.70E+01 |
9.91E+13 |
Fluoranthen |
industry |
Benzene |
n.a. |
|
|
2.30E+01 |
|
Benzo(b)fluoranthen |
industry |
Benzene |
n.a. |
|
|
3.62E+00 |
|
Benzo(k) fluoranthen |
industry |
Benzene |
n.a. |
|
|
1.36E+00 |
|
Benzo(a) pyren |
industry |
Benzene |
n.a. |
|
|
2.67E+00 |
|
Benzo(g,h,i)per ylene |
industry |
Benzene |
n.a. |
|
|
4.29E+00 |
|
Indino(1.2.3-c,d)pyren |
industry |
Benzene |
n.a. |
|
|
2.29E+00 |
|
PAH-eq. (benzo(a) pyren) |
industry |
Benzene |
5.00E+07 |
5.43E+01 |
1.53E+14 |
4.38E+00 |
1.17E+13 |
Dioxin |
industry |
Benzene |
2.90E+10 |
2.90E-05 |
4.73E+10 |
1.40E-05 |
2.17E+10 |
PCP |
industry |
Benzene |
8.30E+03 |
|
0.00E+00 |
|
0.00E+00 |
Hexachlor-benzene (HCB) |
industry |
Benzene |
8.30E+03 |
|
0.00E+00 |
6.26E+03 |
2.78E+12 |
Tetrachlorometh ane (TCM) |
industry |
Benzene |
2.90E+04 |
|
0.00E+00 |
3.00E-01 |
4.66E+08 |
Trichloro-ethylene (TRI) |
industry |
Benzene |
1.90E+04 |
|
0.00E+00 |
4.78E+02 |
4.86E+11 |
Tetrachloroethy lene (PER) |
industry |
Benzene |
2.90E+04 |
|
0.00E+00 |
3.54E+02 |
5.50E+11 |
Trichloro-benzene (TCB) |
industry |
Benzene |
8.30E+03 |
|
0.00E+00 |
4.06E+02 |
1.80E+11 |
Trichloro-ethane (TCE) |
industry |
Benzene |
9.20E+02 |
|
0.00E+00 |
1.00E+01 |
4.93E+08 |
Hexachloro-hexane (HCH) |
industry |
Benzene |
8.30E+03 |
|
0.00E+00 |
9.20E+00 |
4.09E+09 |
Chlorbenzenes |
industry |
Benzene |
8.30E+03 |
|
0.00E+00 |
1.41E+03 |
6.27E+11 |
Vinylchloride |
industry |
Benzene |
3.90E+05 |
|
0.00E+00 |
|
0.00E+00 |
Particulate matter (PM10) |
transport |
Benzene |
2.00E+04 |
3.70E+04 |
4.57E+13 |
5.10E+04 |
5.68E+13 |
|
|
|
|
|
|
|
|
Total |
|
|
|
|
4.48E+14 |
|
3.96E+14 |
Exposure factor (person•μg/m3/g) |
egional, sd-HEFreg(h) |
|
|
|
|
|
|
-benzene |
|
|
|
50000 |
|
50000 |
|
-HCl |
|
|
|
3500 |
|
1500 |
|
Exposure factor (person•μg/m3/g) |
ocal, sd-HEFloc(h) |
|
|
|
|
|
|
-benzene |
|
|
|
0.93 |
|
0.42 |
|
-HCl |
|
|
|
0.5 |
|
0.28 |
|
Population density |
(pers/km2) |
|
|
96 |
|
121 |
|
Click here to see the Table
Click here to see the Table
| United Kingdom |
|
Europe |
|
Emission |
Impact potential |
|
|
1994 |
sd-EP(HTA) |
|
|
ton/Year |
|
|
Total impact (/year) |
|
1.13E+16 |
4.37E+16 |
Population (persons) |
|
5.82E+07 |
2.55E+08 |
Normalisation reference(/year/person) |
|
1.95E+08 |
1.71E+08 |
|
|
|
|
Substance |
|
|
|
SO2 |
2.70E+06 |
1.94E+14 |
|
NOx |
2.39E+06 |
1.95E+14 |
|
N2O |
9.95E+04 |
1.10E+13 |
|
CO |
5.97E+06 |
2.73E+14 |
|
|
|
|
|
nmVOC |
2.35E+06 |
1.73E+14 |
|
|
|
|
|
Cd |
2.35E+01 |
1.43E+14 |
|
|
|
|
|
As |
1.12E+02 |
5.87E+13 |
|
|
|
|
|
Cr(VI) |
6.33E+01 |
3.49E+12 |
|
|
|
|
|
Hg |
1.95E+01 |
7.21E+12 |
|
|
|
|
|
Ni |
4.67E+02 |
1.73E+12 |
|
|
|
|
|
Pb |
1.75E+03 |
9.66E+15 |
|
|
|
|
|
Se |
9.93E+01 |
8.22E+12 |
|
|
|
|
|
Cu |
7.92E+01 |
2.49E+09 |
|
|
|
|
|
Zn |
1.31E+03 |
5.86E+12 |
|
|
|
|
|
Formaldehyde |
|
0.00E+00 |
|
|
|
|
|
Benzene |
|
0.00E+00 |
|
|
|
|
|
Phenol |
|
0.00E+00 |
|
|
|
|
|
Styrene |
|
0.00E+00 |
|
|
|
|
|
Toluene |
|
0.00E+00 |
|
|
|
|
|
Xylenes |
|
0.00E+00 |
|
|
|
|
|
PAH |
7.64E+02 |
2.11E+15 |
|
|
|
|
|
Fluoranthen |
|
|
|
Benzo(b)fluoranthen |
|
|
|
Benzo(k)fluoranthen |
|
|
|
Benzo(a)pyren |
|
|
|
Benzo(g,h,i)perylene |
|
|
|
Indino(1.2.3-c,d)pyren |
|
|
|
PAH-eq. (benzo(a)pyren) |
9.05E+01 |
2.50E+14 |
|
Dioxin |
7.93E-04 |
1.27E+12 |
|
PCP |
5.55E+02 |
2.54E+11 |
|
Hexachlorobenzene (HCB) |
1.20E+00 |
5.50E+08 |
|
Tetrachloromethane (TCM) |
3.19E+03 |
5.11E+12 |
|
Trichloroethylene (TRI) |
2.03E+04 |
2.13E+13 |
|
Tetrachloroethylene (PER) |
1.13E+04 |
1.81E+13 |
|
Trichlorobenzene (TCB) |
6.29E+02 |
2.88E+11 |
|
Trichloroethane (TCE) |
2.47E+04 |
1.25E+12 |
|
Hexachlorohexane (HCH) |
1.14E+02 |
5.22E+10 |
|
Chlorobenzenes |
|
0.00E+00 |
|
Vinylchloride |
|
0.00E+00 |
|
Particulate matter (PM10) |
2.70E+05 |
2.98E+14 |
|
Total |
|
1.13E+16 |
|
Exposure factor regional, sd-HEFreg(h) (person• μg/m3/g) |
|
|
|
-benzene |
50000 |
|
|
-HCl |
3500 |
|
|
Exposure factor local, sd-HEFloc(h) (person• μg/m3/g) |
|
|
|
-benzene |
0.31 |
|
|
-HCl |
0.23 |
|
|
Population density(pers/km2) |
240 |
|
|
Annex 8.9: Typical situations where background concentrations are near or above no-effect-levels for selected air pollutants The information may be used as a first step in the interpretation to
help evaluate whether no-effect-levels are likely to be exceeded by the emission of a given process.
Substance |
Recommendedtreshold level(s)# |
Sources |
Typical exposure situations and exposure levels in relation
to treshold
level, focus on"near or above treshold"& |
NOx |
one hour daily maximum 200 μg/m3(0.11 ppm) |
Outdoor
Mainly combustionprocesses:Traffic (50%), industry
(20%), other mobile sources(10-15%) |
Outdoor, air
>40μg /m3 (annual average)
in large American, European, and Asian cities>50 μg/m3 (annual
average) for 40% of European urban population>400 μg/m3 (1-h)
in some megacities(e.g. Cairo, Delhi, London, Los Angeles, Sao Paulo) Regional
conc. may reach 60-70μg/m3 (24-h) in most of Central europe |
40μg/m3 (0.023 ppm) annual average |
Indoor Gas stoves, unven-ted gas
space |
150 μg/m3 |
24 hour average |
heaters, waterheaters etc.Outdoor sources |
Indoor, air>100 μg/m3 (average over 1-2 weeks)
in 50% of homes and >480 μg/m3 in
8%of homes with kerosene heaters >100 μg/3 (average over 1-2 weeks) in70%
of homes and >480 μg/m3 in
20%of homes with unvented gas spaceheaters> 100 μg/m3 (average
over 1-2 weeks)in some homes with gas coolers andgas stoves 849 μg/m3 (peak
1-h) in homes with kerosene heaters Indoor exposure especially highduring winter
(high heatproduction and low ventilation) |
Substance |
Recommendedtreshold level(s)# |
Sources |
Typical exposure situations and exposure levels in relation
to treshold
level, focus on"near or above treshold"& |
SO2 |
500 μg/m3
10 min. average |
OutdoorMajor sources:
Fuel combustion (especially energyproduction
and manufacturing industries) |
Outdoor, air
> 6000 μg/m3 (short
term) in somehighly industrialised areas> 700 μg/m3 (peak-concentrations)
in
some megacities> 100 μg/m3 (24-h) for 70% of
European urban population100-150 μg/m3 (24-h)
during smogperiods in some parts of Central/East Europe. No indication of
whether
the annual average will be
exceeded.> 150 μg/m3 (annual average)
in
some megacities (e.g. Beijing. MexicoCity and Seoul)50-100 μg/m3 (annual
average) in
some other megacities (e.g. Rio deJaneiro and Shanghai) |
350 μg/m3one hour average |
125 μg/m324 hour average |
Other sources:
Industrial processes
and road traffic |
50 μg/m3annual average |
Particles. |
|
Outdoor |
Outdoor, air |
PM10 |
No human threshold mechanism (noWHO nor EU Guideline) Recommended
UKlimit value:50 μg/m324 hour average |
Combustion processes (especially dieselengines )Natural
sources |
50 μg/m3 (24-h) exceeded extensive-ly
in many European cities Regional concentrations up to 25μg/m3 (annual
average) in certain parts of Central/North EasternEurope 200-600 μg/m3 (annual
average) in12 megacities (mainly Asian, butalso Mexico City and Cairo) |
CO |
100 μg/m3 |
Main source: |
Outdoor, air |
|
15 min. average |
Road traffic |
up to 67 μg/m3 (1-h) in Mexico City30-60 μg/m3 (1-h),
10-20 μg/m3 (8-h) in some megacities (Cairo, Jakarta, London, Los
Angeles, Moscow, NewYork, Sao Paulo) Often > 10 μg/m3 (8-h)
in 10-15worst European cities. No indication of whether one houraverage levels
will be exceeded. |
60 μg/m330 min. average |
30 μg/m3one hour average |
10 μg/m38 hour average |
Substance |
Recommended treshold level(s)# |
Sources |
Typical exposure situations andexposure levels in
relation totreshold level, focus on"near or above treshold"& |
NMVOCs |
Substance specific. |
Outdoor |
Outdoor, air |
|
Road traffic (30%)Solvent and otherproduct handling |
Potential problem close to pointsources (e.g. solvent
industry)Traffic (mainly benzene, see below) |
|
|
The different VOCshave different threshold levels. Therefore
it is problematic thatthe substances are usually measured and the resultsreported
be the group parameter`VOC'. Site characterisation must be performedbased on
the individual substances. |
(30%)Agriculture,forestry, etc. (20%)Other non-combustionprocesses
(10%) |
|
Indoor, airPotential problem. see `sources'column |
|
|
Indirect exposure via theenvironment (drinking water
andfood stuff)Needs case-to-case assessment.Especially a problem for bio-accumulating
and difficultdegradable substances |
Indoor |
Office machinesCleaning agentsTobacco smoke MicrobialformationBio
effluents (fromhumans)Cosmetics Building materials Stripped from tapwater duringshowering,
toiletflush. etc. |
|
|
Substance |
Recommendedtreshold
level(s)# |
Sources |
Typical exposure situations andexposure levels in relation totreshold
level, focus on"near or above treshold"& |
Benzene |
6 μg/m3 (life time) |
Outdoor
Non combusted benzene in petrol |
Outdoor. air
up to 100 μg/m3 in urban
areas withhigh traffic intensity |
|
Drinking water:10 μg/l |
Point sources (e.g.petrol fillingstations and other fuel
handling facilities )Underground petroleum tanks (in relation to drinking watercontamination) |
5-30 μg/m3 general urban populationmay > 6 μg/m3 in
someindustrialised areas3.2 -10 μg/m3 (= 3200 -10.000 μg/m3)during
petrol filling (short term!) |
|
Indirect exposure via the environment (drinking
water and food stuff)Up to 330 μg/l has been measured in drinking water locally Levels
usually below 10 μg/l |
|
Indoor
Cigarette smoke Building materials Stripped
from tapwater during showering. toiletflush. etc. |
|
Indoor. air
Cigarette smokers have a high intake758-1670 μg/m3 (short
term) has been measured in the shower stallduring showering366-498 μg/m3 (short
term) hasbeen measured in bathroom duringshowering |
|
Substance |
Recommendedtreshold level(s)# |
Sources |
Typical exposure situations and exposure levels in relation
totreshold
level, focus on"near or above treshold"& |
Chloro-form |
TDI: 8-10 μ g/kg bw/day |
Outdoor |
Outdoor, air |
|
|
Manufacturing and further processing of the substance Reactions
between organic matter and chlorine (paperbleaching.chlorination of drinking
water. chlorination
of cooling water.chlorination of waste water) Decomposition of other chlorinated compounds |
0.1 - 0.25 μg/m3 in remote cleanareas
in the US 0.3 - 9.9 μg/m3 in urban US areas 4.1 - 160 μg/m3 occasionally
near US point sources< 1 μg/m3 (general exposure level) for
Dutch and German conditions |
(23 μg/m3 over lifetime)
See footnote!! |
|
|
Indirect exposure via the environment (drinking
water and food
stuff)
Drinking water: Occasionally up to 60 μg/l (equalling approx. 2 μg/kg
bw day assuming 2 l water consumption a day and 64 kg body weight) in theUS
Op
til 14 μg/l
in Germany
Op til 18-36 μg/l in Japan |
|
Indoor
Stripped from tapwater during showering,
toilet flush, etc. |
|
Indoor, air
1-10 μg/m3 (general indoor level) 100 μg/m3 is
common in swimmingpools |
|
Substance |
Recommendedtreshold level(s)# |
Sources |
Typical exposure situations andexposure levels in relation totreshold
level, focus on"near or above treshold"& |
HCB |
TDI: 0.11 μg/kg bw/dag |
Outdoor |
Outdoor. air |
|
|
Chlorinated pesticides Incomplete combustion Old
dump sites Waste managemen tof chlorinated solvents and pesticides |
few ng/m3 (or less) distant frompoint
sourcesHigher near point sources |
(0.47 μg/m3 lifetime)≠
See footnote!! |
|
|
Indirect exposure via the environment (drinking
water and food stuff) 0.0004-0.003 μg/kg bw./day; estimated usual US intake
(<<TDI) Critical
exposure levels may be reached in population groups with a diet high in wild
life animals. HCB
accumulates in breast milk. where baby exposures of 0.0018-5.1μg/kg bw/day
have been reported |
|
Dioxins |
TDI: 10 pg/kg bw*/day |
Outdoor |
Outdoor. air |
|
|
Combustion processes (wastes. fossils and wood) Production.
use and disposal of certain chemicals (e.g.chlorinated pesticides and benzenes) Pulp
bleaching Recycling of metals |
Critical exposure levels can be reached near combustion
plants State-of-the-art incinerators with proper air pollution prevention devices
should not pose significant risk |
|
Indirect exposure via the environment (drinking
water and
food stuff)
0.3-3.0 pg/kg bw/day - general population
Critical exposure levels
may be reached in breast milk and populations eating many wild life fish |
|
Substance |
Recommended treshold level(s)# |
Sources |
Typical exposure situations and exposure levels in relation
to treshold level,
focus on"near or above treshold"& |
Lead. Pb |
0.5 μg/m3annual average |
Outdoor |
Outdoor. air |
|
Mining and smelting of lead
Lead in petroladditives Handling
of products containing lead (batteries, cables. pigments,solder, steelproducts) Oil
and coalcombustion Natural sources (volcanicactivity and geological weathering) |
Threshold may be exceeded in areas with a high traffic
intensity in countries where lead is still used as a petrol additive Highexposure
levels may be reached close to point sources (e.g. in the vicinity of lead smelters) |
|
Drinking water:0.05 μg/l |
|
|
Indirect exposure via theenvironment (drinking water
andfood stuff)
Drinking water levels usually < 5μg/l. but may exceed 100 μg/l
(0.1μg/l) in taps with lead plumbing Average US adult intake is 56.5mg/day
mainly from food stuff (dairy products, meat, fish,poultry, grain & cereal
products, vegetables. fruits and beverages). Levels in food stuff rely on background
concentration/production site and lead intakelevels may locally be critical Especially
high intakes may occur for "soil-eating" children playing at contaminated
sites |
|
Substance |
Recommended treshold level(s)# |
Sources |
Typical exposure situations and exposure levels in relation
to treshold
level, focus on"near or above treshold"& |
Cadmium, Cd |
5 μg/m3 (life time) |
Outdoor |
Outdoor. air |
|
|
Metal mining and production (zinc, cadmium, copper,lead)
Phosphate
fertiliser manufacture |
Elevated levels close topollution sources maycontribute
significantly to thetotal intake |
|
Drinking water:0.005 μg/l
Provisional intake:0.4-0.5 μg/week |
Indirect exposure via the environment (drinking
waterand
food stuff)
Average US adult intake is 0.21-0.23 μg/week mainly from food stuff
(grain, cereal products, potatoes and other vegetables) Levels in food stuff
rely on background concentration/production site and cadmiumintake levels may
locally be above the recommended weekly intake level |
|
Cement manufacture |
|
|
Wood combustion |
|
Natural sources (volcanic activity and geological weathering) |
|
Other routesIntake via smoking |
Other routes
Smokers may obtain inhalation intake
levels comparable to the provisional intake |
|
Substance |
Recommended treshold level(s)# |
Sources |
Typical exposure situations and exposure levels in relation
to treshold
level, focus on"near or above treshold"& |
Mercury. Hg |
1 μg/m3annual average |
Outdoor |
Outdoor. air |
|
|
MiningIndustrial processes incl. Hg (e.g. chlor-alkali)Coal
and otherfossil fuelcombustion Cement production
Waste incineration |
Air intakes usually of minor importance |
|
Drinking water0.001 μg/l (organic Hg) |
Indirect exposure via the environment (drinking
water and
food stuff) General intake levels 0.22-0.86μg/kg/week Critical levels may
be reached in population groups with a high consumption of marine mammals (mainly
fish) and in particular breast feed children (due to Hg accumulation in milk) |
|
Provisional intake5 μg/kg bw*/week (total Hg)3 μg/kg
bw*/week (CH3Hg) |
|
|
Other routes
Dental amalgam |
|
Other routes
Dental amalgam may contribute about 10 μg/day (equalling about 1 μg/kg/week) |
|
≠ To be used with caution. Has been derived from the TDI assuming: 64 kg body weight, inspiration of 22 m3 per day and the same bioavailability/uptake via oral and inhalation exposure. Especially the
latter assumption may be questioned.
* `bw': Abbreviation for `body weight'.
# In relation to LCA, attention must be paid to substances with non-threshold mechanisms, e.g. benzene and particles. For these substances, any elevation in exposure will result in an elevated risk. The
recommended threshold levels are therefore less relevant in relation to site characterisation. & For industrialised countries, regulation of point sources will often aim at protecting the surrounding area from
above threshold exposure situations. This assumption may often be interpreted as default, but exceptions may occur.
Footnotes
4 The term "exposure" in the remainder of this chapter is equivalent with the term "increase of accumulated exposure" in Chapter 6 in Potting and Hauschild (2004).
5 The EUTREND model follows a
Gaussian plume approach to calculate concentration spatially resolved over the European grid. A specific strength of EUTREND is its capacity to accurately model the local dimension of emission dispersion
by using 1990 meteorology (annual statistics mean).
6 The Wind rose Model Interpreter (WMI) is part of the integrated assessment model EcoSense. WMI follows trajectory modelling based on region dependent atmospheric conditions (1990 annual
statistics mean).
7 Co-operative Program for Monitoring and Evaluation of the long range transmission of air pollutants in Europe.
9. Ecotoxicity
Background information for this chapter can be found in:
- Chapter 6 of the "Environmental assessment of products. Volume 2: Scientific background" by Hauschild and Wenzel (1998).
- Chapter 8 of the "Background for spatial differentiation in life-cycle impact assessment – the EDIP2003 methodology" by Potting and Hauschild (2005).
9.1 Introduction
Chemical emissions contribute to ecotoxicity if they affect the function and structure of the ecosystems through toxic effects on the organisms living in them. Ecotoxicity involves many different mechanisms of
toxicity and compared to the other environmental impacts included in life cycle impact assessment, ecotoxicity has the character of a composite category which includes all substances with a direct effect on
the health of the ecosystems. On this basis, the list of substances classified as contributing to ecotoxicity will be much more comprehensive than the corresponding lists of the other environmental impacts
(apart from human toxicity which is of a similar nature), and it will include many different types of substances with widely differing chemical characteristics. For a substance to be classified as ecotoxic, it must
be toxic to some of the natural organisms, but toxicity is a relative concept, and paraphrasing the ancient Swiss physician Paracelsus, all substances are toxic if the dose ingested is large enough. Apart from
the substance's toxicity, properties like persistence (low degradability in the environment), and ability to bioaccumulate or be transported to sensitive parts of the environment, therefore determine, which
substances are considered to be ecotoxic. Together with the direct toxicity, these properties are of decisive significance for whether the dose is large enough to result in the occurrence of ecotoxic effects.
9.2 Classification
For the classification of substances contributing to ecotoxicity, a screening tool has been developed as part of EDIP97 based on the substance characteristics discussed above. It is recommended to use this
tool in combination with some of the existing lists of priority pollutants like the List of Undesirable Substances and the Effect List (Danish EPA, 2000a and b).
9.3 EDIP97 characterisation factors
The EDIP97 method (Wenzel et al., 1997, Hauschild et al., 1998) is a simplified version of what has later been called a modular approach to ecotoxicity assessment. Rather than basing it on adaptation of
one of the existing multimedia models developed and used for generic risk assessment of chemicals, the approach behind the EDIP97 method is to identify those properties that are important for the
substance's potential for ecotoxicity and then include these in a transparent and relevant way in the expression of the characterisation factor.
Ecotoxicity is considered in aquatic ecosystems (acute and chronic), in terrestrial ecosystems (chronic exposure) and in wastewater treatment plants. For each endpoint, a simplified fate modelling is applied
based on a modular approach where redistribution between the environmental compartments and potential for biodegradation are represented as separate factors. The characterisation factor for chronic
ecotoxicity in environmental compartment (n) from an emission of substance (i) to compartment (m) is determined as:
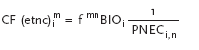
(9.1)
.... where the redistribution factor, fmn expresses which fraction of the
emission, upon redistribution from the initial compartment (m), reaches the final compartment (n), where the ecotoxic impact is modelled. BIO represents the potential for biodegradation as determined from
standardised tests for ready and inherent biodegradability. The toxicity is expressed as the inverse predicted no effect concentration (PNEC) for the ecosystems of compartment (n).
As described in the introduction to this Guideline, the EDIP97 methodology is prepared for inclusion of spatial differentiation for all the non-global impact categories through site factors SF intended to
modify the site-generic characterisation factors. For ecotoxicity assessment, the expression becomes
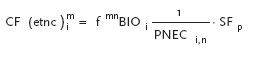
(9.2)
The site-generic impact potential in EDIP97 is interpreted as the largest impact to be expected from the emission and the site factor is seen as the spatially determined probability that the full impact will
occur, i.e. SF ranges between 0 and 1. The EDIP data format is open for inclusion of spatial aspects into the characterisation, and Wenzel and co-authors give guidance on the quantification and use of the
SF without making the site factor really operational (Wenzel et al., 1997).
The EDIP methodology for ecotoxicity assessment also involves other possibilities for spatial differentiation. For the fraction of airborne emissions that deposit, the redistribution factor, fmn is set at "a" when
(n) is the aquatic compartment and 1-a when (n) is the terrestrial compartment. EDIP97 allows "a" to be chosen according to the conditions of the region where the emission takes place. For Danish
conditions, a=0.5 is proposed while a global default is set at a=0.2.
Furthermore, in EDIP97, spatial information in the form of initial dilution data for waterborne emissions, is suggested included as technical information in the weighting of the potential contribution to acute
aquatic ecotoxicity to reflect the differences in dilution potential (and hence the probability of acute effects) for different types of aquatic systems.
9.4 EDIP2003 factors for ecotoxicity
The EDIP2003 factors do not replace the EDIP97 characterisation factors. Rather, they should be considered as exposure factors to be used in combination with the EDIP97 factors which are maintained to
characterise the site-generic impact on ecotoxicity from emissions. This means that the parts of the fate and effect factors which are not spatially differentiated are maintained as they were defined in EDIP97.
For inclusion of spatial variation, the site factor framework of the EDIP97 has been attempted made operational. Since no integrated assessment model has been found for adoption to spatial differentiation in
the modelling of substance fate, the simplified modular approach employed in EDIP97 has been extended into the field of the exposure assessment instead. Based on an analysis of the causality chain for
ecotoxicity, the main spatial characteristics influencing the environmental fate or ecotoxic effect of substances have been identified and the possibility for including them in the characterisation of ecotoxicity has
been examined.
A framework has been developed for inclusion of the spatial variation in average ambient temperature (biodegradation), frequency of natural ecosystems in soil and water (target systems) and sorption and
sedimentation conditions between fresh water and salt water systems (removal). The framework has been made operational for four European regions: North, East, West and South.
Exposure factors for ecotoxicity
The already existing site-generic characterisation factor are interpreted as representing the impact from the substance assuming that exposure takes place. The spatial characterisation is thus performed by
multiplying the site-generic characterisation factor and an exposure factor, which is seen as a modifier expressing the degree to which exposure actually occurs. The ecotoxicity exposure factor is abbreviated
EEF for the sake of consistency with the exposure factors for other impact categories defined in EDIP2003. It depends both on the substance properties and the spatial characteristics of the process and is
expressed as a product of the following variables:
- SFemis representing the spatial variation of the parameters (descriptors) of the emission part of the cause-impact chain
- SFbio representing the spatial variation of the biodegradation and other transformation parameters in the fate part of the cause-impact chain
- SFsed representing the spatial variation of the sorption and sedimentation parameters in the fate part of the cause-impact chain (only relevant for aquatic ecotoxicity).
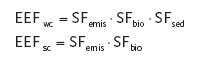
(9.3)
Exposure factors are calculated for ecotoxicity in water and soil in Annex
9.4.
9.5 Site-generic characterisation
The site-generic exposure factors for ecotoxicity are taken as the average values from Tables 9.1 (for aquatic ecotoxicity) and 9.2 (for terrestrial ecotoxicity). The site-generic ecotoxicity impact potentials
are calculated using these factors in combination with the relevant EDIP97 characterisation factors for ecotoxicity from Wenzel et al. (1997), according to the following expression:

(9.4)
Where:
sg-EP(etn) = The site-generic ecotoxicity impact from the product (in m3/f.u.) in environmental compartment n
sg-EEFs = The site-generic exposure factor (dimensionless) relating the emission of substance (s) to the exposure, sg-EEFwc = 1.3 for organic substances and 0.91 for metals and sg-EEFwc = 0.33, determined as
the average values for the European regions in Table 9.1 and 9.2.
CF(etm,n)s = The EDIP97 characterisation factor for ecotoxicity (in m3/g) from Annex 9.1, 9.2 or 9.3 which relates the emission of substance (s) into the initial media (m) to the impact in compartment n
E(m)s = The emission of substance (s) to the initial media (m) (in g/f.u).
9.6 Site-dependent characterisation
The exposure factors are modifying factors representing the severity of the exposure similar to the factors developed for the impact categories human toxicity and aquatic eutrophication. Given the moderate
range between the highest and the lowest site-dependent exposure factors for organic substances in Table 9.1, and given that considerable uncertainties accompany the exposure factors developed for
ecotoxicity, it is found that the additional uncertainties may well exceed the variation given by these exposure factors.
On this background, there is only little motivation for performing a full site-dependent exposure assessment for ecotoxicity in soil or water. Rather, the site-dependent factors should be seen as an information
for a sensitivity analysis and possibly also for reduction of the potential spatial variation in the site-generic impact.
The Guideline will recommend that their application will be for sensitivity analysis to help quantify the possible spatial variation underlying the site-generic impact potentials
For emission of metals to water, the situation is a bit different. Here, the type of receiving environment strongly influences the loss through sedimentation for the most adsorbing metals, in the sense that
emissions to rivers and lakes have a much lower exposure factor due to sedimentation in lakes. This deviation from the general pattern of the exposure factors does not alter the overall recommendation that
the ecotoxicity exposure factors are used only in a sensitivity analysis context and not in a routine site-dependent characterisation.
The ecotoxicity impact from a given product is in many cases dominated by one or a few processes. Even for applications, where a site-dependent assessment is preferred, it is therefore advised to start with
calculation of the site-generic impact of a product as described in the previous section. This site-generic impact can be used to select the processes with the dominating contributions (step 1), and next to
evaluate the actual spatial variation in the contribution from these processes by applying the relevant site-dependent factors (step 2 and 3).
Step 1
The site-generic ecotoxicity impact in water or soil, as calculated in the previous section, is broken down into the contributions from the separate processes. These contributions are then ranked from the
largest to the smallest contribution, and the process with the largest contribution is selected.
Step 2
The site-generic ecotoxicity impact from step 1 is reduced with the contribution of the process selected in step 1. Next, the site-dependent impact from the emissions of this process is estimated with the
relevant site-dependent factors.

(9.5)
Where:
sd-EP(etn)p = The site-dependent ecotoxicity impact in compartment (n) from process (p)
EEF(etn)s,i = The site-dependent exposure factor (dimensionless) relating the emission of substance
(s) in situation (i) as relevant for process (p) (described by geographical region and location in the hydrological cycle) to exposure at the regional level. The site-dependent exposure factor is found in Annex
9.4 in Table 9.9 of for organic substances and in Table 9.10 for metals.
CF(etm,n)s = The EDIP97 characterisation factor for ecotoxicity (in m3/g) from Annex 9.1,
9.2 or 9.3 which relates the emission of substance
(s) into the initial media (m) to the impact in compartment n
E(m)s = The emission of substance (s) to the initial media (m) (in g/f.u).
The determining parameters are the region of emission (Northern, Western, Eastern, and Southern Europe) and type of receiving water for emissions to water (river or lake, estuary, sea, influencing SFsed)
and a number of substance characteristics (biodegradability, lipophilicity and volatility).
For emissions of organic substances or metals to air, the part which ends up in water is assumed to deposit mainly in the sea and EEFwc for sea (in Table
9.9 or Table 9.10) is therefore chosen for air-borne emissions.
Step 3
The site-dependent contributions from the process selected in step 1 are added to the adjusted site-generic contribution from step 2. Step 2 is repeated until the site-dependent contribution from the selected
processes is so large that the residual spatial variation in the ecotoxicity score can no longer influence the conclusion of the study (e.g. when the site-dependent share is larger than 95% of the total impact
score).
9.7 Interpretation
For the exposure factors tabulated in Annex 9.4, the ranges (min-max value) are shown in Table 9.1 for aquatic and terrestrial ecotoxicity of organic substances and in Table 9.2 for metals.
Table 9.1. Ranges (min-max value) and medians of exposure factors for organic substances.
Region |
Aquatic ecotoxicity |
|
Terrestrial ecotoxicity |
Max value |
Min value |
Median value |
|
Northen europe |
1.95 |
0.14 |
1.91 |
0.65 |
(1.5•1.3•1) |
(1.5•1.3•0.07) |
(1.5•1.3•0.98) |
(0.5•1.3) |
Vestern europe |
1 |
0.07 |
0.98 |
0.25 |
(1•1•1) |
(1•1•0.07) |
(1•1•0.98) |
(0.25•1) |
Eastern europe |
2 |
0.14 |
1.96 |
0.25 |
(2•1•1) |
(2•1•0.07) |
(2•1•0.98) |
(0.25•1) |
Sourthen europe |
1.4 |
0.098 |
1.37 |
0.18 |
(2•0.7•1) |
(2•0.7•0.07) |
(2•0.7•0.98) |
(0.25•0.7) |
Overall median |
1.39 |
0.25 |
Overall average standard deviation |
1.30 |
0.33 |
0.54 |
0.22 |
For organic substances it is found that the largest variation, which can be introduced by using this framework for spatial characterisation of aquatic ecotoxicity, is a factor 28 (1.95:0.07 between the value for
a not biodegradable substance emitted directly to the sea in Northern Europe and a strongly lipophilic substance emitted to a river in Western Europe). For substances of less extreme lipophilicity
(logKow<4), the largest variation is a factor 6.5, found between the same two situations. For terrestrial ecotoxicity, the largest variation is a factor 3.7 (0.65:0.18 between the value for any substance emitted
to soil in Northern Europe and any substance emitted to soil in Southern Europe).
The variation between highest and lowest exposure factor is thus quite modest, even for extremely lipophilic substances. Indeed, the developed exposure factor is expected to represent only a minor part of
the actual spatially determined variation in the fate and resulting exposure of ecosystems to chemicals within Europe since:
- A large number of parameters which potentially contribute to spatial variation could not be included in the framework as explained in Tørsløv et al. (2005). In general, their inclusion was not feasible due to
the low availability of environmental data or the current modest state of ecotoxicity modelling. This is the case for differences in ecosystem sensitivities and differences in background loads throughout Europe.
If it had been possible to include more of these parameters, it is anticipated that the modelled spatial variation as expressed through the exposure factor EEF would have been larger.
- As argued in Tørsløv et al. (2005), it must be expected that the size of the variation in nature parameters between regions is reduced when the size of the region is increased (the larger the region, the larger
the differences within the region and the lower the variation between regions).
This trend will propagate to the exposure factors calculated from selected nature parameters. In the present methodology, for feasibility reasons, Europe has been split into just four regions, and it is
foreseeable that if the framework had been based on individual countries rather than such large geographical regions, the modelled spatial variation would have been larger.
For metals, the picture in Table 9.2
is somewhat different from what was observed for organic substances in Table 9.1 mainly due to the occurrence of extremely low exposure factors for the strongly adsorbing metals, particularly lead and tin
when emitted to freshwater systems (river, lake) where their removal through adsorption and sedimentation is efficient. For the rest of the metals, the pattern is similar to the pattern for organic substances.
Overall, it is judged that considerable uncertainties accompany the exposure factors developed for ecotoxicity, and that these uncertainties may well exceed the variation given by the factors. On this
background, the authors do not find it recommendable to apply the developed exposure factors in an attempt to perform spatial characterisation of ecotoxicity in LCIA.
Furthermore, the emission data for calculating European normalisation references lack the required spatial differentiation for most substances (Stranddorf et al., 2005), and therefore it has not been possible
to calculate EDIP2003 normalisation references for any of the ecotoxicity sub categories.
Currently, work is underway in the OMNIITOX project under the fifth Frame Programme of EU on development of a European consensus method for characterisation of ecotoxicity in LCA. This method
involves a comprehensive multimedia fate model with the option of spatial differentiation at the level of countries.
Table 9.2. Ranges (min-max value), averages and medians of exposure factors for metals.
Region |
Aquatic ecotoxicity |
|
Terrestrial ecotoxicity |
Max value |
Min value |
Median |
|
NorthernEurope |
1.93 |
6.3?10-6 |
1.30 |
0.65 |
(1.5•1.3•0.99) |
(1.5•1.3•3.2?10-6) |
(1.5•1.3•0.67) |
(0.5•1.3) |
Western Europe |
0.99 |
3.2?10-6(1•1•3.2?10-6) |
0.67 |
0.25 |
(1•1•0.99) |
|
(1•1•0.67) |
(0.25•1) |
Eastern Europe |
1.98 |
6.5?10-6(2•1•3.2?10-6) |
1.34 |
0.25 |
(2•1•0.99) |
|
(2•1•0.67) |
(0.25•1) |
SouthernEurope |
1.39 |
4.5?10-6(2•0.7•3.2?10-6) |
0.89 |
0.18 |
(2•0.7•0.99) |
|
(2•0.7•0.67) |
(0.25•0.7) |
|
|
|
|
|
Overall median |
0.92 |
0.25 |
Overall average |
0.91 |
0.33 |
Standard deviation of overall average |
|
|
0.62 |
0.22 |
The reader with interest in spatial characterisation of ecotoxicity is referred to the results of this work which will be available towards the end of 2004 (www.OMNIITOX.net.).
9.8 Example
In spite of the recommendation given in Section 9.7, the EDIP2003 exposure factors have been applied in a characterisation of the inventory presented in Section 1.6 to illustrate their use.
Site-generic characterisation
As described in Section 9.5, first the site-generic impacts are calculated. The ecotoxicity impacts shown in Table 9.3 are determined according to Equation
9.4, using the EDIP97 factors from Annex 9.1 and 9.2 (for emissions to air and water respectively) and site-generic exposure factors taken as the averages in Table 9.1 and 9.2.
Among the air- and waterborne emissions, EDIP97 factors exist only for the metals but these are also expected to be the strongest contributors to ecotoxicity in water and soil.
Table 9.3.
Site-generic impact potentials for chronic ecotoxicity in water and soil for one supporting block made from plastic or zinc, (expressed as volume of exposed compartment).
Substance |
Emission forplastic part |
Emission forzinc part | EF(etwc) |
EF(etsc) |
sg-EEFwc |
sg-EEFsc |
|
g/f.u. |
g/f.u. |
m3/g |
m3/g |
|
|
|
|
|
|
|
|
|
Emissions to air |
|
|
|
|
|
|
Hydrogen chloride |
0.001163 |
0.00172 |
|
|
|
|
Carbon moNOxide |
0.2526 |
0.76 |
|
|
|
|
Ammonia |
0.003605 |
0.000071 |
|
|
|
|
Methane |
3.926 |
2.18 |
|
|
|
|
VOC, powerplant |
0.0003954 |
0.00037 |
|
|
|
|
VOC, diesel engines |
0.02352 |
0.0027 |
|
|
|
|
VOC, unspecified |
0.89 |
0.54 |
|
|
|
|
Sulphur dioxide |
5.13 |
13.26 |
|
|
|
|
Nitrogen oxides |
3.82 |
7.215 |
|
|
|
|
Lead |
0.00008031 |
0.0002595 |
400 |
0.01 |
0.91 |
0.33 |
Cadmium |
0.00000866 |
0.00007451 | 2.40•104 |
1.8 |
0.91 |
0.33 |
Zinc |
0.000378 |
0.00458 |
200 |
0.005 |
0.91 |
0.33 |
Emissions to water |
0 |
0 |
|
|
|
|
NO3--N |
0.00005487 |
0.0000486 |
|
|
|
|
NH4+-N |
0.0004453 |
0.003036 |
|
|
|
|
PO43- |
0.000014 |
0 |
|
|
|
|
Zinc |
0.00003171 |
0.002209 | 1.00•103 |
0 |
0.91 |
|
|
|
|
|
|
|
|
Total |
|
|
|
|
|
|
Substance |
Plastic part |
|
Zinc part |
|
|
|
|
|
|
|
sg-EP(etwc) |
sg-EP(etsc) |
sg-EP(etwc) |
sg-EP(etsc) |
|
m3/f.u. |
m3/f.u. |
m3/f.u. |
m3/f.u. |
|
|
|
|
|
Emissions to air |
|
|
|
|
Hydrogen chloride |
0 |
0 |
0 |
0 |
Carbon moNOxide |
0 |
0 |
0 |
0 |
Ammonia |
0 |
0 |
0 |
0 |
Methane |
0 |
0 |
0 |
0 |
VOC, power plant |
0 |
0 |
0 |
0 |
VOC, diesel engines |
0 |
0 |
0 |
0 |
VOC, unspecified |
0 |
0 |
0 |
0 |
Sulphur dioxide |
0 |
0 |
0 |
0 |
Nitrogen oxides |
0 |
0 |
0 |
0 |
Lead |
0.029233 |
2.7•10-7 |
0.094458 |
8.56•10-7 |
Cadmium |
0.189134 |
5.1•10-6 |
16.272.984 |
4.4310-5 |
Zinc |
0.068796 |
6.2•10-7 |
0.83356 |
7.56•10-6 |
Emissions to water |
0 |
0 |
0 |
0 |
NO3--N |
0 |
0 |
0 |
0 |
NH4+-N |
0 |
0 |
0 |
0 |
PO43- |
0 |
0 |
0 |
0 |
Zinc |
0.028856 |
0 |
201.019 |
0 |
Total |
0.32 |
6.0•10-6 |
4.6 |
5.3•10-5 |
|
|
|
|
|
Using the site-generic EDIP97 characterisation factors, the zinc supporting block has the largest chronic ecotoxicity impact potential in both water and soil. For both supporting blocks, cadmium and zinc
emissions to air are the most important contributors to ecotoxicity in water and soil while the waterborne zinc emission also contributes significantly to ecotoxicity in water for the zinc component. In order to
shed some light on the influence of the potential spatial variation, site-dependent characterisation is performed for those processes that contribute the most to the site-generic impacts.
Site-dependent characterisation
Table 9.3 reveals that the predominant contributions to the ecotoxicity impact are caused by Cd and Zn to air and (for the zinc component) Zn to air. For the zinc component, the main sources for Cd and Zn
emissions to air and water are identified as the production of zinc from ore which takes place in Bulgaria and, for Zn to air, the casting of the component which takes place in Yugoslavia (data not shown).
For the plastic component, the Cd and Zn emissions to air originate mainly from the generation of electricity which takes place at a number of places throughout Europe. For the latter it is thus chosen to
retain the site-generic characterisation. The emissions from the selected processes contribute about 80% and 95% of the full site-generic impacts of Table 9.3 for the zinc component and the plastic component respectively (data not shown).
In the calculation of the site-dependent impacts for the key processes for the zinc component, the relevant site-dependent regional exposure factors are found in Table 9.10 of Annex 9.4. All the main
processes take place in Southern Europe. The results of the site-dependent characterisation are shown in Table 9.4.
Table 9.4.
Site-dependent impact potentials for chronic ecotoxicity in water and soil for key processes from the zinc component product system.
Zinc part |
|
EF(etwc) |
EF(etsc) |
EEFwc |
EEFsc |
sd-EP(etwc) |
sd-EP(etsc) |
|
g/f.u. |
m3/g |
m3/g |
|
|
m3/f.u. |
m3/f.u. |
Zinc emissions to air |
|
|
|
|
|
|
|
Zinc production, Bulgaria |
2.77•10-3 | 2.00•102 |
0.005 |
1.11 |
0.175 |
0.614 |
2.42•10-6 |
Zinc casting, Yugoslavia |
1.34•10-3 | 2.00•102 |
0.005 |
1.11 |
0.175 |
0.297 |
1.17•10-6 |
Lead emissions to air |
|
|
|
|
|
|
|
Zinc production, Bulgaria |
1.75•10-4 |
400 |
0.01 |
0.66 |
0.175 |
4.62•10-2 |
3.06•10-7 |
Cadmium emissions to air |
|
|
|
|
|
|
|
Zinc production, Bulgaria |
6.50•10-5 | 2.40•104 |
1.8 |
1.28 |
0.175 |
2.00 |
2.05•10-5 |
Zinc emissions to water |
|
|
|
|
|
|
|
Zinc production, Bulgaria |
2.17•10-3 | 1.00•103 |
0 |
0.93 |
0.175 |
2.02 |
0.00 |
Total, zink part |
|
|
|
|
|
5.0 |
2.4•10-5 |
The site-generic impacts from these key processes are subtracted from the original site-generic impacts in Table 9.3 and the site-dependent impacts of Table 9.4 are added. The thus corrected ecotoxicity
impacts via air are found in Table 9.5 and the difference to the original site-generic impacts of Table
9.3 is illustrated in Figure 9.1.
Table 9.5. Chronic ecotoxicity impacts in water and soil from the zinc component product system with site-dependent characterisation of key process emissions
|
Chronic aquaticecotoxicity |
Chronic terrestrialecotoxicity |
|
m3/f.u. |
m3/f.u. |
Zinc component |
5.3 |
3.1•10-5 |
Plastic component |
0.32 |
6.0•10-6 |
Site-dependent characterisation slightly increases the size of the aquatic ecotoxicity impact and reduces the terrestrial ecotoxicity impact, but it does not influence the strong dominance of the zinc component
over the plastic component. For the zinc-based component more than 90 % of this impact has now been calculated using site-dependent characterisation factors. Even if the site-dependent characterisation
was performed for all the remaining processes in the product system, the result would thus not change significantly. The major part of the spatially conditioned potential for variation of the impact has been
cancelled.
Figure 9.1 Site-generic and site-dependent ecotoxicity impacts in water and soil.For the site-dependent impacts, the site-dependent exposure factors have only been applied for the key processes as described above.
Click here to see the Figure
Annex 9.1: EDIP97 characterisation factors for ecotoxicity assessment for emissions to air (Wenzel et al., 1997)
Emissions to air as firstcompartment |
Substance |
CAS no. |
EF(etwc) |
EF(etwa) |
EF(etsc) |
|
|
3m/g |
3m/g |
3m/g |
|
|
|
|
|
1.2-Propylene oxide |
75-56-9 |
1.2 |
0 |
11 |
1.2-Dichlorobenzene |
95-50-1 |
10 |
0 |
0.49 |
1.2-Dichloroethane |
107-06-2 |
20 |
0 |
61 |
1-Butanol |
71-36-3 |
0.01 |
0 |
0.09 |
2.3.7.8-Tetrachloro-dibenzo-p-dioxin |
1746-01-6 |
5.6E+08 |
0 |
1.2E+04 |
|
|
|
|
|
2.4-Dinitrotoluene |
121-14-2 |
150 |
0 |
190 |
2-Chlorotoluene |
95-49-8 |
200 |
0 |
10 |
2-Ethyl hexanol |
104-76-7 |
0 |
0 |
0 |
2-Propanol |
67-63-0 |
0.05 |
0 |
0.46 |
3-Chlorotoluene |
108-41-8 |
200 |
0 |
14 |
4-Chlorotoluene |
106-43-4 |
200 |
0 |
12 |
Acetic acid |
64-19-7 |
0.08 |
0 |
0.79 |
Acetone |
67-64-1 |
4,0E+03 |
0 |
3,8E+04 |
Anionic detergent (worst case) |
n.a. |
4.0 |
0 |
33 |
|
|
|
|
|
Anthracene |
120-12-7 |
0 |
0 |
0 |
Arsenic |
7440-38-2 |
380 |
0 |
0.27 |
Atrazine |
1912-24-9 |
0 |
0 |
0 |
Benzene |
71-43-2 |
4.0 |
0 |
3.6 |
Benzotriazole |
95-14-7 |
4.0 |
0 |
13 |
Biphenyl |
92-52-4 |
200 |
0 |
2,8 |
Cadmium |
7440-46-9 |
2.4E+04 |
0 |
1.8 |
Chlorobenzene |
108-90-7 |
200 |
0 |
38 |
Chloroform |
67-66-3 |
20 |
0 |
25 |
Chromium (VI) |
7440-47-3 |
130 |
0 |
0.01 |
Cobalt |
7440-48-4 |
400 |
0 |
9.1 |
Copper |
7440-50-8 |
2.5E+03 |
0 |
0,02 |
DibutyltiNOxide |
818-08-6 |
2.0E+04 |
0 |
530 |
Diethanolamine |
111-42-2 |
0 |
0 |
0 |
Diethylaminoethanol |
100-37-8 |
0 |
0 |
0 |
Diethylene glycol |
111-46-6 |
0 |
0 |
0 |
Annex 9.1: EDIP97 characterisation factors for ecotoxicity assessment for emissions to air (Wenzel et al., 1997)
Emissions to air as first |
compartment |
|
|
|
Substance |
CAS no. |
EF(etwc) |
EF(etwa) |
EF(etsc) |
|
|
m3/g |
m3/g |
m3/g |
Diethylene glycol mono-n-butyl-ether |
112-34-5 |
0 |
0 |
0 |
|
|
|
|
|
Ethanol |
64-17-5 |
0.001 |
0 |
0.01 |
Ethyl acetate |
141-78-6 |
0.08 |
0 |
0.59 |
Ethylene glycol |
107-21-1 |
0.001 |
0 |
0.010 |
Ethylenediamine tetraacetic acid, EDTA |
60-00-4 |
0 |
0 |
0 |
|
|
|
|
|
Ethylenediamine, 1.2-ethanediamine |
107-15-3 |
0 |
0 |
0 |
|
|
|
|
|
Formaldehyde |
50-00-00 |
24 |
0 |
200 |
Hexane |
110-54-3 |
150 |
0 |
2.5 |
Hydrogen cyanide |
74-90-8 |
800 |
0 |
7.6E+03 |
Hydrogene sulphide |
7783-06-4 |
0 |
0 |
0 |
Iron |
7439-89-6 |
20 |
0 |
0.53 |
Isopropylbenzene, cumene |
98-82-8 |
2.9 |
0 |
0.08 |
|
|
|
|
|
Lead |
7439-92-1 |
400 |
0 |
0.01 |
Manganese |
7439-96-5 |
71 |
0 |
1.9 |
Mercury |
7439-97-6 |
4.0E+03 |
0 |
5.3 |
Methanol |
67-56-1 |
0.01 |
0 |
0.10 |
Methyl methacrylate |
80-62-6 |
0 |
0 |
0 |
|
|
|
|
|
Molybdenum |
7439-98-7 |
400 |
0 |
3.9 |
Monoethanolamine |
141-43-5 |
0 |
0 |
0 |
|
|
|
|
|
n-Butyl acetate |
123-86-4 |
0.56 |
0 |
1.0 |
Nickel |
7440-02-0 |
130 |
0 |
0.05 |
Nitrilotriacetate |
139-13-9 |
0 |
0 |
0 |
Nitrobenzenesulphonic acid |
127-68-4 |
0.09 |
0 |
0.84 |
|
|
|
|
|
Phenol |
108-95-2 |
0 |
0 |
0 |
Propylene glycol, 1.2-propanediol |
57-55-6 |
0 |
0 |
0 |
|
|
|
|
|
Selenium |
7782-49-2 |
4.0E+03 |
0 |
106 |
Sodium benzoate |
532-32-10 |
0.63 |
0 |
6.2 |
Sodium-hypochlorite |
7681-52-9 |
0 |
0 |
0 |
|
|
|
|
|
Strontium |
7440-24-6 |
2,0E+03 |
0 |
53 |
Styrene |
100-42-5 |
0 |
0 |
0 |
Sulphamic acid |
5329-14-6 |
2.8 |
0 |
28 |
Tetrachlorethylene |
127-18-4 |
20 |
0 |
1.1 |
|
|
|
|
|
Thallium |
7440-28-0 |
670 |
0 |
18 |
Thorium |
7440-29-1 |
330 |
0 |
8.9 |
Titanium |
7440-32-6 |
27 |
0 |
0.73 |
Toluene |
108-88-3 |
4.0 |
0 |
0.97 |
Triethanolamine |
102-71-6 |
0 |
0 |
0 |
Triethylamine |
121-44-8 |
0 |
0 |
0 |
Vanadium |
7440-62-2 |
40 |
0 |
0.34 |
Xylenes, mixed |
1330-20-7 |
4.0 |
0 |
0.40 |
|
|
|
|
|
Zinc |
7440-66-6 |
200 |
0 |
0.005 |
Annex 9.2: EDIP97 characterisation factors for ecotoxicity assessment for emissions to water (Wenzel et al., 1997)
Emissions to water as firstcompartment |
Substance | CAS no. | EF(etwc) | EF(etwa) | EF(etsc) |
| | m3/g | m3/g | m3/g |
1.2-Propylene oxide | 75-56-9 | 5.9 | 0.59 | 0 |
1.2-Dichlorobenzene | 95-50-1 | 10 | 10 | 0.49 |
1.2-Dichloroethane | 107-06-2 | 100 | 10 | 0 |
1-Butanol | 71-36-3 | 0.07 | 0.04 | 0 |
2.3.7.8-Tetrachlorodibenzo-pdioxin | 1746-01-6 | 2.8E+09 | 2.8E+08 | 0 |
2.4-Dinitrotoluene | 121-14-2 | 770 | 77 | 0 |
2-Chlorotoluene | 95-49-8 | 200 | 100 | 10 |
2-Ethyl hexanol | 104-76-7 | 2.7 | 1.3 | 0 |
2-Propanol | 67-63-0 | 0.25 | 0.13 | 0 |
3-Chlorotoluene | 108-41-8 | 200 | 100 | 14 |
4-Chlorotoluene | 106-43-4 | 200 | 100 | 12 |
Acetic acid | 64-19-7 | 0.40 | 0.20 | 0 |
Acetone | 67-64-1 | 2.0E+04 | 10 | 0 |
Anionic detergent (worst case) | n.a. | 20 | 10 | 0 |
Anthracene | 120-12-7 | 5.0E+04 | 1.0E+04 | 0 |
Arsenic | 7440-38-2 | 1.9E+03 | 190 | 0 |
Atrazine | 1912-24-9 | 6.7E+03 | 670 | 0 |
Benzene | 71-43-2 | 4.0 | 10 | 3.6 |
Benzotriazole | 95-14-7 | 20 | 2.0 | 0 |
Biphenyl | 92-52-4 | 1.0E+03 | 100 | 0 |
Cadmium | 7440-46-9 | 1.2E+05 | 1.2E+04 | 0 |
Chlorobenzene | 108-90-7 | 200 | 100 | 38 |
Chloroform | 67-66-3 | 20 | 10 | 25 |
Chromium | 7440-47-3 | 670 | 67 | 0 |
Cobalt | 7440-48-4 | 2.0E+03 | 200 | 0 |
Copper | 7440-50-8 | 1.3E+04 | 1.3E+03 | 0 |
DibutyltiNOxide | 818-08-6 | 1.0E+05 | 1.0E+04 | 0 |
Diethanolamine | 111-42-2 | 0.91 | 0.45 | 0 |
Diethylaminoethanol | 100-37-8 | 13 | 1.3 | 0 |
Diethylene glycol | 111-46-6 | 0.03 | 0.02 | 0 |
Diethylene glycol monon-butyl ether | 112-34-5 | 0.19 | 0.19 | 0 |
Ethanol | 64-17-5 | 0.005 | 0.003 | 0 |
Ethyl acetate | 141-78-6 | 0.41 | 0.21 | 0 |
Ethylene glycol | 107-21-1 | 0.005 | 0.002 | 0 |
Ethylendiamine tetraacetic acid, EDTA | 60-00-4 | 1.8 | 0.18 | 0 |
Ethylendiamine, 1.2-ethandiamine | 107-15-3 | 0.87 | 0.43 | 0 |
Formaldehyde | 50-00-00 | 120 | 60 | 0 |
Hexane | 110-54-3 | 150 | 74 | 2.5 |
Hydrogen cyanide | 74-90-8 | 800 | 2.0E+03 | 7.6E+03 |
Hydrogen sulphide | 7783-06-4 | 0 | 3.3E+03 | 0 |
Iron | 7439-89-6 | 100 | 10 | 0 |
Isopropylbenzene, cumene | 98-82-8 | 2.9 | 7.1 | 0.08 |
Lead | 7439-92-1 | 2.0E+03 | 200 | 0 |
Manganese | 7439-96-5 | 360 | 36 | 0 |
Mercury | 7439-97-6 | 4.0E+03 | 2.0E+03 | 5.3 |
Methanol | 67-56-1 | 0.05 | 0.03 | 0 |
Methyl methacrylate | 80-62-6 | 0.54 | 0.27 | 0 |
Molybdenum | 7439-98-7 | 2.0E+03 | 200 | 0 |
Monoethanolamine | 141-43-5 | 27 | 13 | 0 |
n-Butyl acetate | 123-86-4 | 2.8 | 0.56 | 0 |
Nickel | 7440-02-0 | 667 | 67 | 0 |
Nitrilotriacetate | 139-13-9 | 0.15 | 0.08 | 0 |
Nitrobenzenesulphonic acid, sodium salt | 127-68-4 | 0.09 | 0.04 | 0.84 |
Phenol | 108-95-2 | 44 | 22 | 0 |
Propylene glycol, 1.2-propanediol | 57-55-6 | 0.02 | 0.01 | 0 |
Selenium | 7782-49-2 | 2.0E+04 | 1.4E+03 | 0 |
Sodium benzoate | 532-32-10 | 3.2 | 1.6 | 0 |
Sodium-hypochlorite | 7681-52-9 | 267 | 27 | 0 |
Strontium | 7440-24-6 | 1.0E+04 | 1.0E+03 | 0 |
Styrene | 100-42-5 | 0 | 40 | 0 |
Sulphamic acid | 5329-14-6 | 14 | 7.0 | 0 |
Tetrachlorethylene | 127-18-4 | 20 | 10 | 1.1 |
Thallium | 7440-28-0 | 3.3E+03 | 330 | 0 |
Thorium | 7440-29-1 | 1.7E+03 | 1.7E+03 | 0 |
Titanium | 7440-32-6 | 140 | 14 | 0 |
Toluene | 108-88-3 | 4.0 | 10 | 0.97 |
Triethanolamine | 102-71-6 | 5.6 | 1.1 | 0 |
Triethylamine | 121-44-8 | 100 | 10 | 0 |
Vanadium | 7440-62-2 | 200 | 20 | 0 |
Xylenes, mixed | 1330-20-7 | 4.0 | 10 | 0.40 |
Zinc | 7440-66-6 | 1.0E+03 | 100 | 0 |
Annex 9.3: EDIP97 characterisation factors for ecotoxicity assessment for emissions to soil (Wenzel et al., 1997)
Emissions to soil as firstcompartment |
Substance |
CAS no. |
EF(etwc) |
EF(etwa) |
EF(etsc) |
|
|
m3/g |
m3/g |
m3/g |
1.2-Propylene oxide |
75-56-9 |
0 |
0 |
13 |
1.2-Dichlorobenzene |
95-50-1 |
10 |
0 |
0.49 |
1.2-Dichloroethane |
107-06-2 |
0 |
0 |
76 |
1-Butanol |
71-36-3 |
0 |
0 |
0.11 |
2.3.7.8-Tetrachloro-dibenzo-p-dioxin |
1746-01-6 |
0 |
0 |
1.5E+04 |
2.4-Dinitrotoluene |
121-14-2 |
0 |
0 |
235 |
2-Chlorotoluene |
95-49-8 |
200 |
0 |
10 |
2-Ethyl hexanol |
104-76-7 |
0 |
0 |
0.16 |
2-Propanol |
67-63-0 |
0 |
0 |
0.58 |
3-Chlorotoluene |
108-41-8 |
200 |
0 |
14 |
4-Chlorotoluene |
106-43-4 |
200 |
0 |
12 |
Acetic acid |
64-19-7 |
0 |
0 |
0.99 |
Acetone |
67-64-1 |
0 |
0 |
4.7E+04 |
Anionic detergent (worst case) |
n.a. |
0 |
0 |
41 |
Anthracene |
120-12-7 |
0 |
0 |
59 |
Arsenic |
7440-38-2 |
0 |
0 |
0.33 |
Atrazine |
1912-24-9 |
0 |
0 |
528 |
Benzene |
71-43-2 |
4.0 |
0 |
3.6 |
Benzotriazole |
95-14-7 |
0 |
0 |
16 |
Biphenyl |
92-52-4 |
0 |
0 |
3.5 |
Cadmium |
7440-46-9 |
0 |
0 |
2.2 |
Chlorobenzene |
108-90-7 |
200 |
0 |
38 |
Chloroform |
67-66-3 |
20 |
0 |
25 |
Chromium |
7440-47-3 |
0 |
0 |
0.01 |
Cobalt |
7440-48-4 |
0 |
0 |
11 |
Copper |
7440-50-8 |
0 |
0 |
0.02 |
DibutyltiNOxide |
818-08-6 |
0 |
0 |
665 |
Diethanolamine |
111-42-2 |
0 |
0 |
2.2 |
Diethylaminoethanol |
100-37-8 |
0 |
0 |
28 |
Diethylene glycol |
111-46-6 |
0 |
0 |
0.07 |
Diethylene glycol monon-butyl ether |
112-34-5 |
0 |
0 |
0.37 |
Ethanol |
64-17-5 |
0 |
0 |
0.01 |
Ethyl acetate |
141-78-6 |
0 |
0 |
0.73 |
Ethylene glycol |
107-21-1 |
0 |
0 |
0.01 |
Ethylene diamine tetra acetic acid, EDTA |
60-00-4 |
0 |
0 |
4.5 |
Ethylenediamine, 1.2-ethanediamine |
107-15-3 |
0 |
0 |
2.1 |
Formaldehyde |
50-00-00 |
0 |
0 |
254 |
Hexane |
110-54-3 |
150 |
0 |
2.5 |
Hydrogen cyanide |
74-90-8 |
800 |
0 |
7.6E+03 |
Hydrogen sulphide |
7783-06-4 |
0 |
0 |
0 |
Iron |
7439-89-6 |
0 |
0 |
0.66 |
Isopropylbenzene, cumene |
98-82-8 |
2.9 |
0 |
0.08 |
Lead |
7439-92-1 |
0 |
0 |
0.01 |
Manganese |
7439-96-5 |
0 |
0 |
2.4 |
Mercury |
7439-97-6 |
4.0E+03 |
0 |
5.3 |
Methanol |
67-56-1 |
0 |
0 |
0.12 |
Methyl methacrylate |
80-62-6 |
0 |
0 |
0.48 |
Molybdenum |
7439-98-7 |
0 |
0 |
4.8 |
Monoethanolamine |
141-43-5 |
0 |
0 |
66 |
n-Butyl acetate |
123-86-4 |
0 |
0 |
1.3 |
Nickel |
7440-02-0 |
0 |
0 |
0.07 |
Nitrilotriacetate |
139-13-9 |
0 |
0 |
0.38 |
Nitrobenzenesulphonic acid, sodium salt |
127-68-4 |
0.09 |
0 |
0.84 |
Phenol |
108-95-2 |
0 |
0 |
110 |
Propylene glycol, 1.2-pro-panediol |
57-55-6 |
0 |
0 |
0.05 |
Selenium |
7782-49-2 |
0 |
0 |
130 |
Sodium benzoate |
532-32-10 |
0 |
0 |
7.8 |
Sodium hypochlorite |
7681-52-9 |
0 |
0 |
610 |
Strontium |
7440-24-6 |
0 |
0 |
66 |
Styrene |
100-42-5 |
0 |
0 |
0.0 |
Sulphamic acid |
5329-14-6 |
0 |
0 |
35 |
Tetrachlorethylene |
127-18-4 |
20 |
0 |
1.1 |
Thallium |
7440-28-0 |
0 |
0 |
22 |
Thorium |
7440-29-1 |
0 |
0 |
11 |
Titanium |
7440-32-6 |
0 |
0 |
0.91 |
Toluene |
108-88-3 |
4.0 |
0 |
0.97 |
Triethanolamine |
102-71-6 |
0 |
0 |
14 |
Triethylamine |
121-44-8 |
0 |
0 |
80 |
Vanadium |
7440-62-2 |
0 |
0 |
0.43 |
Xylenes, mixed |
1330-20-7 |
4.0 |
0 |
0.40 |
Zinc |
7440-66-6 |
0 |
0 |
0.007 |
Annex 9.4: EDIP2003 exposure factors for ecotoxicity in water and soil
The exposure factors are calculated applying Equation 9.3:
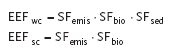
The individual factors of the exposure factor are discussed below and on this basis the exposure factors are calculated. Background information for the calculation of the individual factors is found in Tørsløv
et al., 2005.
The emission component, SFemis
For emissions to air or emissions to water or soil which are found to evaporate, the SFemis factor reflects the fraction of the deposited part of the emission which will expose water or soil ecosystems.
In connection to EDIP97, SFemis is defined as
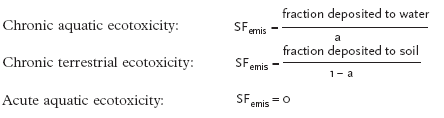
(9.6)
Where a is the fraction assumed to deposit to land in the calculation of the site-generic EDIP97 ecotoxicity factor.
SFemis is calculated based on fractions deposited to water and soil natural areas for the four European regions, assuming the use of a global default value of 0.2 for a in the EDIP97 characterisation factors
in Annex 9.1-9.3.
The biodegradation and transformation component, SFbio
The SFbio factor reflects the variation of biodegradability with the average temperature of the region, where the fate of the substance takes place. It is relevant for both aquatic and terrestrial systems. The
annual average temperature over Europe varies around 10° C between the Nordic region and the Southern region with the Western and Eastern regions in-between. Assuming that the current site-generic
fate modelling (in EDIP97 or other LCIA methodology) corresponds to an average mid-European situation, the SFbio factor is determined as:
Table 9.6. SFemis for emissions occurring in different regions of Europe.
Region |
Aquatic ecotoxicity |
Terrestrial ecotoxicity |
Nordic countries |
1.5 |
0.5 |
Western countries |
1 |
0.25 |
Eastern countries |
2 |
0.25 |
Southern countries |
2 |
0.25 |
Southern countries: SFbio= 0.7
Nordic countries: SFbio= 1.3
East and Western European countries: SFbio = 1
The sorption and sedimentation component, SFsed
The SFsed factor must reflect the spatial variation in the relative importance of sedimentation as a removal process for substances adsorbing to particulate material in different aquatic systems. The SFsed
factor is only relevant for substances emitted to or ending in the aquatic compartment of the environment.
In EDIP97, there is no consideration of removal due to sedimentation. This is equivalent to operating with a removal factor with the value 1 (just like no potential for biodegradation is represented by a BIO
factor value of 1).
The removal by sedimentation depends on:
1)The net-sedimentation rate of suspended material in different aquatic systems.
2)The position of the emission point in the hydrogeological cycle.
3)The biodegradability and thus how long time the substance can be expected to be present in the environment.
4)The sorption behaviour of the substance
These parameters are included in the values for SFsed for organic substances presented in Table 9.7 and for metals in Table 9.8.
Table 9.7. SFsed representing removal by the combined effect of sedimentation and biodegradation for readily biodegradable, inherently biodegradable and not biodegradable organic substances of different
lipophilicity for the three emission scenarios: emission to river and from there through lake to estuary and sea, emission through estuary to sea and emission directly to sea.
logKow | River-lake-estuary-sea |
Estuary-sea |
Sea |
|
|
|
|
|
|
|
|
|
|
|
Ready |
Inherent |
N.B. |
Ready |
Inherent |
I.B. |
Ready |
Inherent |
N.B. |
-3 |
0.30 |
0.60 |
0.99 |
0.79 |
0.91 |
1.00 |
1.00 |
1.00 |
1.00 |
-2 |
0.30 |
0.60 |
0.99 |
0.79 |
0.91 |
1.00 |
1.00 |
1.00 |
1.00 |
-1 |
0.30 |
0.60 |
0.99 |
0.79 |
0.91 |
1.00 |
1.00 |
1.00 |
1.00 |
0 |
0.30 |
0.60 |
0.99 |
0.79 |
0.91 |
1.00 |
1.00 |
1.00 |
1.00 |
1 |
0.30 |
0.60 |
0.99 |
0.79 |
0.91 |
1.00 |
1.00 |
1.00 |
1.00 |
2 |
0.30 |
0.60 |
0.99 |
0.79 |
0.91 |
1.00 |
1.00 |
1.00 |
1.00 |
3 |
0.30 |
0.60 |
0.99 |
0.79 |
0.91 |
1.00 |
1.00 |
1.00 |
1.00 |
4 |
0.30 |
0.59 |
0.98 |
0.79 |
0.90 |
1.00 |
1.00 |
1.00 |
1.00 |
5 |
0.26 |
0.52 |
0.86 |
0.79 |
0.90 |
0.99 |
1.00 |
1.00 |
1.00 |
6 |
0.07 |
0.14 |
0.24 |
0.72 |
0.82 |
0.90 |
0.98 |
0.98 |
0.98 |
N.B.: Not biodegradable
Table 9.8. SFsed representing removal by sedimentation for different metals in the three emission scenarios: emission to river and from there through lake to estuary and sea, emission through estuary to sea
and emission directly to sea.
Metal |
Kd m3 |
/kg |
River-lake-estuary-sea |
Estuary-sea |
Sea |
|
|
|
|
|
|
As |
10 |
|
0.73 |
0.98 |
0.99 |
Cd |
50 |
|
0.21 |
0.86 |
0.92 |
Co |
50 |
|
0.21 |
0.86 |
0.92 |
Cr(III) |
126 |
|
0.02 |
0.67 |
0.79 |
Cu |
50 |
|
0.21 |
0.86 |
0.92 |
Hg |
200 |
|
0.00 |
0.52 |
0.69 |
Ni |
40 |
|
0.28 |
0.89 |
0.94 |
Pb |
398 |
|
0.00 |
0.27 |
0.47 |
Se |
16 |
|
0.61 |
0.96 |
0.98 |
Sn |
398 |
|
0.00 |
0.27 |
0.47 |
Zn |
126 |
|
0.02 |
0.67 |
0.79 |
Ecotoxicity exposure factors, EEF
Based on the SF-values given above, the aquatic ecotoxicity exposure factors are calculated and tabulated for organic substances and metals in Table 9.9 and Table 9.10, and the terrestrial ecotoxicity
exposure factors in Table 9.11.
Spatial differentiation in Life Cycle impact assessment - The EDIP2003 methodology
Table 9.9. Site-dependent exposure factors for aquatic ecotoxicity (EEFwc) of organic substances depending on region of emission, lipophilicity, biodegradability (ready, inherent or not biodegradable) and
point of emission in the hydrological chain (to river, estuary or sea).
Northern Europe |
logKow |
river-lake-estuary-sea |
estuary-sea |
Sea |
|
|
|
|
|
|
|
|
|
|
|
Ready |
Inherent |
n.b. |
Ready |
Inherent |
n.b. |
Ready |
Inherent |
n.b. |
-3 |
0.59 |
1.17 |
1.94 |
1.55 |
1.77 |
1.95 |
1.95 |
1.95 |
1.95 |
-2 |
0.59 |
1.17 |
1.94 |
1.55 |
1.77 |
1.95 |
1.95 |
1.95 |
1.95 |
-1 |
0.59 |
1.17 |
1.94 |
1.55 |
1.77 |
1.95 |
1.95 |
1.95 |
1.95 |
0 |
0.59 |
1.17 |
1.94 |
1.55 |
1.77 |
1.95 |
1.95 |
1.95 |
1.95 |
1 |
0.59 |
1.17 |
1.94 |
1.55 |
1.77 |
1.95 |
1.95 |
1.95 |
1.95 |
2 |
0.59 |
1.17 |
1.94 |
1.55 |
1.77 |
1.95 |
1.95 |
1.95 |
1.95 |
3 |
0.59 |
1.16 |
1.93 |
1.55 |
1.77 |
1.95 |
1.95 |
1.95 |
1.95 |
4 |
0.58 |
1.15 |
1.91 |
1.55 |
1.76 |
1.95 |
1.95 |
1.95 |
1.95 |
5 |
0.51 |
1.01 |
1.68 |
1.53 |
1.75 |
1.93 |
1.95 |
1.95 |
1.95 |
6 |
0.14 |
0.28 |
0.47 |
1.40 |
1.60 |
1.76 |
1.91 |
1.91 |
1.91 |
Western Europe |
logKow |
river-lake-estuary-sea |
estuary-sea |
Sea |
|
|
|
|
|
|
|
|
|
|
|
Ready |
Inherent |
n.b. |
Ready |
Inherent |
n.b. |
Ready |
Inherent |
n.b. |
-3 |
0.30 |
0.60 |
0.99 |
0.79 |
0.91 |
1.00 |
1.00 |
1.00 |
1.00 |
-2 |
0.30 |
0.60 |
0.99 |
0.79 |
0.91 |
1.00 |
1.00 |
1.00 |
1.00 |
-1 |
0.30 |
0.60 |
0.99 |
0.79 |
0.91 |
1.00 |
1.00 |
1.00 |
1.00 |
0 |
0.30 |
0.60 |
0.99 |
0.79 |
0.91 |
1.00 |
1.00 |
1.00 |
1.00 |
1 |
0.30 |
0.60 |
0.99 |
0.79 |
0.91 |
1.00 |
1.00 |
1.00 |
1.00 |
2 |
0.30 |
0.60 |
0.99 |
0.79 |
0.91 |
1.00 |
1.00 |
1.00 |
1.00 |
3 |
0.30 |
0.60 |
0.99 |
0.79 |
0.91 |
1.00 |
1.00 |
1.00 |
1.00 |
4 |
0.30 |
0.59 |
0.98 |
0.79 |
0.90 |
1.00 |
1.00 |
1.00 |
1.00 |
5 |
0.26 |
0.52 |
0.86 |
0.79 |
0.90 |
0.99 |
1.00 |
1.00 |
1.00 |
6 |
0.07 |
0.14 |
0.24 |
0.72 |
0.82 |
0.90 |
0.98 |
0.98 |
0.98 |
Eastern Europe |
logKow |
river-lake-estuary-sea |
estuary-sea |
Sea |
|
|
|
|
|
|
|
|
|
|
|
Ready |
Inherent |
n.b. |
Ready |
Inherent |
n.b. |
Ready |
Inherent |
n.b. |
-3 |
0.60 |
1.20 |
1.99 |
1.59 |
1.81 |
2.00 |
2.00 |
2.00 |
2.00 |
-2 |
0.60 |
1.20 |
1.99 |
1.59 |
1.81 |
2.00 |
2.00 |
2.00 |
2.00 |
-1 |
0.60 |
1.20 |
1.99 |
1.59 |
1.81 |
2.00 |
2.00 |
2.00 |
2.00 |
0 |
0.60 |
1.20 |
1.99 |
1.59 |
1.81 |
2.00 |
2.00 |
2.00 |
2.00 |
1 |
0.60 |
1.20 |
1.99 |
1.59 |
1.81 |
2.00 |
2.00 |
2.00 |
2.00 |
2 |
0.60 |
1.19 |
1.99 |
1.59 |
1.81 |
2.00 |
2.00 |
2.00 |
2.00 |
3 |
0.60 |
1.19 |
1.98 |
1.59 |
1.81 |
2.00 |
2.00 |
2.00 |
2.00 |
4 |
0.59 |
1.18 |
1.96 |
1.59 |
1.81 |
2.00 |
2.00 |
2.00 |
2.00 |
5 |
0.52 |
1.04 |
1.72 |
1.57 |
1.79 |
1.98 |
2.00 |
2.00 |
2.00 |
6 |
0.15 |
0.29 |
0.48 |
1.43 |
1.64 |
1.80 |
1.96 |
1.96 |
1.96 |
Southerneurope |
logKow |
river-lake-estuary-sea |
estuary-sea |
Sea |
|
|
|
|
|
|
|
|
|
|
|
Ready |
Inherent |
n.b. |
Ready |
Inherent |
n.b. |
Ready |
Inherent |
n.b. |
-3 |
0.42 |
0.84 |
1.39 |
1.11 |
1.27 |
1.40 |
1.40 |
1.40 |
1.40 |
-2 |
0.42 |
0.84 |
1.39 |
1.11 |
1.27 |
1.40 |
1.40 |
1.40 |
1.40 |
-1 |
0.42 |
0.84 |
1.39 |
1.11 |
1.27 |
1.40 |
1.40 |
1.40 |
1.40 |
0 |
0.42 |
0.84 |
1.39 |
1.11 |
1.27 |
1.40 |
1.40 |
1.40 |
1.40 |
1 |
0.42 |
0.84 |
1.39 |
1.11 |
1.27 |
1.40 |
1.40 |
1.40 |
1.40 |
2 |
0.42 |
0.84 |
1.39 |
1.11 |
1.27 |
1.40 |
1.40 |
1.40 |
1.40 |
3 |
0.42 |
0.84 |
1.39 |
1.11 |
1.27 |
1.40 |
1.40 |
1.40 |
1.40 |
4 |
0.42 |
0.82 |
1.37 |
1.11 |
1.27 |
1.40 |
1.40 |
1.40 |
1.40 |
5 |
0.37 |
0.73 |
1.21 |
1.10 |
1.26 |
1.38 |
1.40 |
1.40 |
1.40 |
6 |
0.10 |
0.20 |
0.34 |
1.00 |
1.15 |
1.26 |
1.37 |
1.37 |
1.37 |
Table 9.10. Site-dependent exposure factors for aquatic ecotoxicity (EEFwc) of individual metals depending on region of emission, and point of emission in the hydrological chain (to river, estuary or sea).
Northern europe |
Metal |
river-lake-estuary-sea |
estuary-sea |
Sea |
As |
1.43 |
1.91 |
1.93 |
Cd |
0.40 |
1.67 |
1.79 |
Co |
0.40 |
1.67 |
1.79 |
Cr(III) |
0.036 |
1.30 |
1.55 |
Cu |
0.40 |
1.67 |
1.79 |
Hg |
0.0035 |
1.02 |
1.34 |
Ni |
0.56 |
1.73 |
1.82 |
Pb |
6.30•10-6 |
0.53 |
0.92 |
Se |
1.19 |
1.87 |
1.91 |
Sn |
6.30•10-6 |
0.53 |
0.92 |
Zn |
0.04 |
1.30 |
1.55 |
Western europe |
Metal |
river-lake-estuary-sea |
estuary-sea |
Sea |
As |
0.73 |
0.98 |
0.99 |
Cd |
0.21 |
0.86 |
0.92 |
Co |
0.21 |
0.86 |
0.92 |
Cr(III) |
0.018 |
0.67 |
0.79 |
Cu |
0.21 |
0.86 |
0.92 |
Hg |
0.0018 |
0.52 |
0.69 |
Ni |
0.28 |
0.89 |
0.94 |
Pb |
3.23•10-6 |
0.27 |
0.47 |
Se |
0.61 |
0.96 |
0.98 |
Sn |
3.23•10-6 |
0.27 |
0.47 |
Zn |
0.02 |
0.67 |
0.79 |
Eastern europe |
Metal |
river-lake-estuary-sea |
estuary-sea |
Sea |
As |
1.47 |
1.95 |
1.98 |
Cd |
0.41 |
1.71 |
1.83 |
Co |
0.41 |
1.71 |
1.83 |
Cr(III) |
0.037 |
1.34 |
1.59 |
Cu |
0.41 |
1.71 |
1.83 |
Hg |
0.0036 |
1.05 |
1.38 |
Ni |
0.57 |
1.77 |
1.87 |
Pb |
6.46•10-6 |
0.55 |
0.94 |
Se |
1.22 |
1.92 |
1.96 |
Sn |
6.46•10-6 |
0.55 |
0.94 |
Zn |
0.04 |
1.34 |
1.59 |
Southerneurope |
Metal |
river-lake-estuary-sea |
estuary-sea |
Sea |
As |
1.03 |
1.37 |
1.39 |
Cd |
0.29 |
1.20 |
1.28 |
Co |
0.29 |
1.20 |
1.28 |
Cr(III) |
0.026 |
0.93 |
1.11 |
Cu |
0.29 |
1.20 |
1.28 |
Hg |
0.0025 |
0.73 |
0.96 |
Ni |
0.40 |
1.24 |
1.31 |
Pb |
4.52•10-6 |
0.38 |
0.66 |
Se |
0.85 |
1.34 |
1.37 |
Sn |
4.52•10-6 |
0.38 |
0.66 |
Zn |
0.03 |
0.93 |
1.11 |
For emissions of organic substances or metals to air, the part which ends up in water is assumed to deposit mainly in the sea and EEFwc for sea is therefore chosen for air-borne emissions.
Table 9.11. Site-dependent exposure factors for terrestrial ecotoxicity (EEFsc) of organic substances and metals depending on region of emission.
Region |
EEFsc |
Nordic countries |
0.65 |
Western countries |
0.25 |
Eastern countries |
0.25 |
Southern countries |
0.175 |
10. Example of the application of site -dependent impact assessment
Examples in Chapters 4-9 have demonstrated the application of the EDIP2003 site-generic and site-dependent characterisation factors on the same example. The example which is introduced in Section 1.6
concerns an LCA-based comparison of the use of zinc and the use of plastic (polyethylene) as material for a supporting block (a structural element) in the seat of an office chair.
Figure 10.1 summarises the difference between the site-generic and the site-dependent impacts for the different impact categories.
As seen from Figure 10.1, the inclusion of spatial differentiation at the level of country of emission influences the size of the impact potential to a larger or minor extent for all impact categories. Sometimes the
site-dependent impacts are higher than the site-generic impacts, and sometimes they are lower, but the dominance of the zinc component over the plastic component is rather stable. Sometimes it is
strengthened a bit (human toxicity via air), sometimes it is weakened a little (aquatic eutrophication) but only for two of the impact categories does site-dependent characterisation reverse the dominance of
the zinc component. This is the case for the categories acidification and ozone impact on human health, where the elimination of part of the spatial variation in the dispersion patterns and sensitivity of the
exposed environment means that the impact from the plastic component becomes larger than the impact from the zinc component. As stated in the ozone example in Section 7.9, the ozone impact on human
health from the plastic component still comprises a significant potential for spatial variation, and therefore no conclusion can be drawn for this impact category without extending the site-dependent
characterisation to comprise additional key processes.
Figure 10.1 Site-generic and site-dependent impact potentials for acidification, terrestrial eutrophication, photochemical ozone impacts on vegetation and human health, aquatic eutrophication, human health
via air exposure and chronic ecotoxicity in water and soil from the two product systems. For the site-dependent impacts, the site-dependent characterisation factors have only been applied for the key
processes as described in the respective chapters.
Click here to see the Figure
10.1 Normalisation
In order to further investigate the importance of the findings described above, the site-dependent impacts calculated throughout the example are normalised in Table 10.1 using the relevant site-dependent
EDIP2003 normalisation references for the different impact categories (site-dependent normalisation references are not available for the ecotoxicity categories).
The normalised site-dependent impact profile is shown in Figure 10.2. It is clear from the figure that compared to the background load of society, the different impact potentials have roughly the same size
across the impact categories, except for the ozone impact to human health for the plastic component which is about twice the size of the other normalised impact potentials and the human toxicity impact via
air which is lower than the others.
10.2 Interpretation
The potential spatial variation in the characterisation is large for many of the impact categories as revealed by the standard deviation on the site-generic characterisation factors throughout the example. In the
example, spatially determined differences in sensitivity are considered relevant to the decisions that shall be based on the results of the LCA. Therefore, the spatial variation can be seen as an uncertainty on
the conclusion. This uncertainty is so large, that based on the site-generic impact assessment, it is not possible to conclude dominance for one of the two supportive blocks for any of the investigated impact
categories.
Table 10.1. Normalised site-dependent impact scores for the zinc and plastic components
Impact |
Site-dependent |
impact |
Site-dependent |
Normalised |
site- |
category |
|
|
normalisation |
dependent impact |
|
|
|
|
reference |
|
|
|
Zinc |
Plastic |
|
Zinc |
Plastic |
|
component |
component |
|
component |
component |
Acidification |
0.088 m2 |
0.189 m |
2.2.103 m2 |
0.040 mPE |
0.086 mPE |
|
|
|
|
|
|
Terrestrial |
|
|
|
|
|
eutrophication |
0.195 m2 |
0.092 m2 |
2.1.103 m2/person/yr |
0.093 mPE |
0.044 mPE |
Aquatic |
|
|
|
|
|
eutrophication |
0.50 g N-eq. |
0.35 g N-eq. |
12.103g N-eq./person/yr |
0.042 mPE |
0.029 mPE |
Photochemical |
17.6 |
10.9 m2ppm•hours |
1.4.105 m2•ppm•hours/ |
0.13 mPE |
0.08 mPE |
ozone impacts |
m2ppm.hours |
|
person/year |
|
|
|
|
|
|
|
|
on vegetation |
|
|
|
|
|
Photochemical |
8.8.10-4 |
2.9.10-3 |
10 pers•ppm•hours/ |
0.088 mPE |
0.29 mPE |
ozone impacts |
pers•ppm•hours |
pers•ppm•hours |
person/year |
|
|
on humans |
|
|
|
|
|
Human |
3.4.103 |
1.3.103 |
1.7.108 /person/year |
0.020 mPE |
0.008 mPE |
toxicity |
|
|
|
|
|
Figure 10.2 Normalised impact potentials for the two product systems. Except for the ozone impact on human health, the major spatial variation in dispersion and exposure has been eliminated from the
impacts.
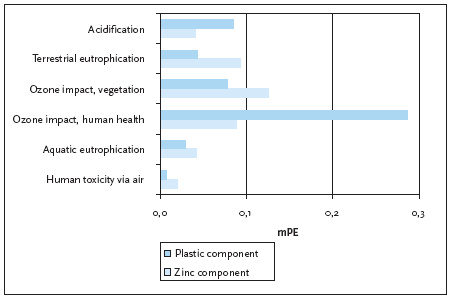
A site-dependent characterisation removes the major part of this uncertainty for all impacts for the zinc component and for all impacts except photochemical ozone impact on human health for the plastic
component. Compared to the site-generic characterisation, the dominance is reversed for two of the impacts – acidification and ozone impact on human health.
The normalised site-dependent impact potentials in Figure 10.2 show a trade-off where the zinc component is superior for acidification and ozone impact on human health while the plastic component has the
lowest impact in the rest of the catgories. A weighting is needed to decide which alternative is preferable from an environmental perspective. If the ozone impact on human health turns out to be decisive, the
site-dependent characterisation must be performed for an additional part of the processes in the plastic component's product system.
The weighting goes beyond the scope of this Guideline but it should be noted that in order to use the EDIP97 default weighting factors (based on political reduction targets), the two subcategories for
photochemical ozone formation must be aggregated into one photochemical ozone formation impact potential. According to the EDIP97 methodology this is done by taking the average of the normalised
impact potentials for the sub categories. The same holds true for the sub categories on eutrophication.
11. References
Albritton, D.L. and Meira Filho, L.G. (eds.): Climate Change 2001: The scientific basis. ISBN: 0521014956, Earthprint Ltd. U.K., 2001.
Alcamo, J., R. Shaw and L. Hordijk (eds.). The RAINS model of acidification. Science and strategies in Europe. Kluwer Academic Publishers, Dordrecht, the Netherlands, 1990.
Beusen, A: User manual of CARMEN1 (Draft). National Institute of Public Health and Environmental Protection (RIVM), Bilthoven, the Netherlands, not published.
Christensen, F.M.: Human toxicity. Chapter 9 in Stranddorf, H., Hoffmann,
L. and Schmidt, A.: Update of selected impact categories, normalisation and weighting in LCA. Environmental project no. 995, the Danish Environmental Protection Agency, Copenhagen, 2005.
Danish EPA: List of undesirable substances. Environmental Review no. 15, Danish Environmental Protection Agency, Copenhagen, 2000a.
Danish EPA: The effect list. Environmental Review no. 6 (in Danish), Danish Environmental Protection Agency, Copenhagen, 2000b.
Hauschild, M. (ed.): Background of the environmental assessment of products (in Danish), 670 pp. ISBN 87-7810-543-9, Ministry of Environment and Energy and Confederation of Danish Industries,
Copenhagen, 1996.
Hauschild, M. and Wenzel, H.: Acidification as a criterion in the environmental assessment of products. In Hauschild, M.Z. and Wenzel, H.: Environmental assessment of products. Vol. 2 - Scientific
background, 565 pp. Chapman & Hall, United Kingdom, ISBN 0412 80810 2, Kluwer Academic Publishers, Hingham, MA. USA, 1998c.
Hauschild, M. and Wenzel, H.: Global warming as a criterion in the environmental assessment of products. In Hauschild, M.Z. and Wenzel, H.: Environmental assessment of products. Vol. 2 - Scientific
background, 565 pp. Chapman & Hall, United Kingdom, ISBN 0412 80810 2, Kluwer Academic Publishers, Hingham, MA. USA, 1998b.
Hauschild, M. and Wenzel, H.: Nutrient enrichment as a criterion in the environmental assessment of products. In Hauschild, M.Z. and Wenzel, H.: Environmental assessment of products. Vol. 2 - Scientific
background, 565 pp. Chapman & Hall, United Kingdom, ISBN 0412 80810 2, Kluwer Academic Publishers, Hingham, MA. USA, 1998d.
Hauschild, M. and Wenzel, H.: Photochemical ozone formation as criterion in environmental assessment of products. Chapter 3 of Hauschild, M. and Wenzel, H.: Environmental assessment of products. Vol.
2 - Scientific background, Chapman & Hall, United Kingdom, Kluwer Academic Publishers, ISBN 0412 80810 2, Hingham, MA., USA, 1998e.
Hauschild, M., Bastrup-Birk, A., Hertel, O., Schöpp, W., and Potting, J.: Photochemical ozone formation. Chapter 6 in Potting, J. and Hauschild, M. (eds.): Background for spatial differentiation in life cycle
impact assessment – the EDIP2003 methodology. Environmental project no. 996, Danish Environmental Protection Agency, Copenhagen, 2005.
Hauschild, M., Olsen, S.I. and Wenzel, H.: Human toxicity as criterion the environmental assessment of products. In Hauschild, M.Z. and Wenzel, H.: Environmental assessment of products. Vol. 2 -
Scientific background, 565 pp. Chapman & Hall, United Kingdom, ISBN 0412 80810 2, Kluwer Academic Publishers, Hingham, MA. USA, 1998f.
Hauschild, M., Wenzel, H., Damborg, A. and Tørsløv, J.: Ecotoxicity as criterion in environmental assessment of products. Chapter 6 of Hauschild,
M. and Wenzel, H.: Environmental assessment of products. Vol. 2 - Scientific background, Chapman & Hall, United Kingdom, ISBN 0412 80810 2, Kluwer Academic Publishers, Hingham, MA. USA,
1998g.
Hauschild, M.Z. and Wenzel, H.: Environmental assessment of products. Vol. 2 - Scientific background, 565 pp. Chapman & Hall, United Kingdom, ISBN 0412 80810 2, Kluwer Academic Publishers,
Hingham, MA. USA, 1998a.
Heijungs, R., J. Guinée, g. Huppes, R.M. Lankreijer, H.A. Udo de Haes, A. Wegener Sleeswijk, A.M.M. Ansems, P.G. Eggels, R. Van Duin en H.P. de Goede. Environmental life cycle assessment of
products. Guide and background (ISBN 90-5191-064-9). Centre of Environmental Science of Leiden University, Leiden, the Netherlands, 1992.
ISO 14042: Environmental management – Life cycle assessment - Life cycle impact assessment. International Organisation for Standardisation, 1999.
Krewitt, W., P. Mayerhofer, R. Friedrich, A. Trukenmüller, T. Heck, A. Greßmann, F. Raptis, F. Kaspar, J. Sachau, K. Rennings, J. Diekmann, B. Praetorius. ExternE – Externalities of energy. National
implementation in Germany (EUR 18271). Directorate-General XII for Science, Research and Development of the European Commission, 1997.
Kristensen, P. and H. O. Hansen: European rivers and lakes. Assessment of their environmental state. EEA environmental monographs 1, European Environmental Agency, Copenhagen, 1994.
Larsen, J.: Nutrient enrichment. Chapter 8 in Stranddorf, H., Hoffmann, L. and Schmidt, A.: Update of selected impact categories, normalisation and weighting in LCA. Environmental project no. 995, the
Danish Environmental Protection Agency, Copenhagen, 2005.
Lindfors, L-G, K. Christiansen, L. Hoffman,Y. Virtanen, V. Juntilla, O-J. Hanssen, A. Rønning, T. Ekval and G. Finnveden.: Nordic Guidelines on life cycle assessment (Nord 1995; 20). Nordic Council of
Ministers, Copenhagen, 1995.
Montzka, S.A., Frazer, P.J. and coauthors: Controlled substances and other source gases. Chapter 1 in: Ajavon, A.N., Albritton, D.L., Mégie, G., and Watson, R.T. (eds.): Scientific assessment of ozone
depletion : 2002. World Meteorological Organisation Global Ozone Research and Monitoring Project – report no. 47, WMO Geneva, 2002.
Potting, J. and Hauschild, M. (eds.): Background for spatial differentiation in life-cycle impact assessment – the EDIP2003 methodology. Environmental project no. 996, Danish Environmental Protection
Agency, Copenhagen, 2005.
Potting, J., Beusen, A., Øllgaard, H., Hansen, O.C., de Haan, B. and Hauschild, M.: Aquatic eutrophication. Chapter 5 in Potting, J. and Hauschild, M. (eds.): Background for spatial differentiation in life
cycle impact assessment – the EDIP2003 methodology. Environmental project no. 996, Danish Environmental Protection Agency, Copenhagen, 2005a.
Potting, J., Trukenmüller, A., Christensen, F.M., van Jaarsveld, H., Olsen, S.I. and Hauschild, M.: Human toxicity. Chapter 7 in Potting, J. and Hauschild,
M. (eds.): Background for spatial differentiation in life cycle impact assessment – the EDIP2003 methodology. Environmental project no. 996, Danish Environmental Protection Agency, Copenhagen,
2005b.
Stranddorf, H., Hoffmann, L. and Schmidt, A.: Impact categories, normalisation and weighting in LCA. Environmental News no. 78 from the Danish Environmental Protection Agency, Copenhagen, 2005.
Tobler, W., U. Deichmann, J. Gottsegen and K. Maloy. The Global Demography Project (Technical Report TR-95-6 [online]). Santa Barbara (United States of America), dept. of Geography, University of
California of the National Centre for Geographic Information and Analysis, 1995 [cited 11 August 1999]. Available from Internet http://www.ciesin.org/datasets/gpw/globldem.doc.html.
Tørsløv, J., Hauschild, M., Rasmussen, D. and Potting, J.: Ecotoxicity. Chapter 8 in Potting, J. and Hauschild, M. (eds.): Background for spatial differentiation in life-cycle impact assessment – the
EDIP2003 methodology. Environmental project no. 996, Danish Environmental Protection Agency, Copenhagen, 2005.
Udo de Haes, H.A., Jolliet, O., Finnveden, G., Hauschild, M., Krewitt, W. and Müller-Wenk, R.: Best available practice regarding impact categories and category indicators in life cycle impact assessment.
International Journal of Life Cycle Assessment, 4(2), 1-15, 1999
Wenzel, H., Hauschild M.Z. and Alting, L.: Environmental assessment of products. Vol. 1 - Methodology, tools, techniques and case studies, 544 pp. Chapman & Hall, United Kingdom, ISBN 0 412
80800 5, Kluwer Academic Publishers, Hingham, MA. USA., 1997.
Wenzel, H., Hauschild M.Z. and Alting, L.: Environmental assessment of products. Vol. 1 - Methodology, tools, techniques and case studies, 544 pp. Chapman & Hall, United Kingdom, ISBN 0 412
80800 5, Kluwer Academic Publishers, Hingham, MA. USA., 1997.
Wenzel, H., Hauschild, M. and Rasmussen, E.: Environmental assessment of products (in Danish), 335 pp. ISBN 87-7810-542-0, Ministry of Environment and Energy and Confederation of Danish
Industries, Copenhagen, 1996.
| Top | | Front page |
Version 1.0 January 2006, © Danish Environmental Protection Agency
|