| Front page | | Contents | | Previous | | Next |
Life Cycle Assessment of Slurry Management Technologies
3 Reference scenarios
3.1 System Description
The reference scenarios in this report do not represent an average of the slurry management systems in Denmark, but should rather be seen as a representative of “typical” systems. Accordingly, an attempt to identify the most commonly used methods has been made. The reference scenarios do not cover all situations and possibilities. For some of the possibilities, sensitivity analyses of different alternatives have been made in order to clarify the significance of the choice.
For pigs, the reference scenario is based on fattening pigs. Fattening pigs constitute a significant amount of the total number of pigs in Denmark. According to Statistics Denmark (2008a) approximately 42% of all pigs where “Weaned pigs less than 50 kg” and 28% of all pigs where “Weaned pigs at 50 kg and above” in 2007. Hence, in total 70%4 of the pigs in Denmark are fattening pigs (30-100 kg). The Danish Norm data for N, P and K in livestock manure (2001-2008) are all based on the study made by Poulsen et al. (2001), where the category “Fattening pigs (30-100 kg)” is used. The slurry composition for this category has been used in this study.
For cattle, it has been decided to base the reference scenario on dairy cows.
According to Dansk Kvæg (2007 and 2008), dairy cows constituted approximately 35% of the cattle livestock population in Denmark in 2007 and 2006. The category “dairy cows” was the largest single category. In the Danish Norm data for N, P and K in livestock manure (2001-2008) and in Poulsen et al. (2001), the corresponding category is ”1 year cow, heavy race”. (Poulsen et al. (2001) also have a category for Jersey cattle, however, the Jersey cattle production is relatively small according to the statistics in Dansk Kvæg (2007 and 2008)).
The choice of selecting the categories “fattening pigs” and “dairy cows” are supported by the fact that a considerable amount of the international literature in the slurry management area focus on these categories, thus the data availability is considerable for these categories.
A simplified flow diagram for the reference scenarios is shown in figure 3.1
Figure 3.1. A simplified flow diagram for the reference scenarios
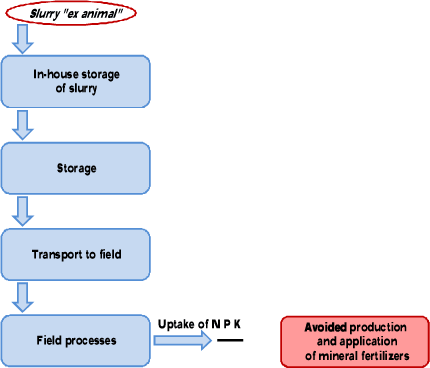
It has been necessary to define the preconditions concerning the reference scenarios regarding e.g. housing units, type of storage, technology for application to the field and reference cropping scenarios. Some of these preconditions are significant for the final results. Hence, sensitivity analysis has been carried out for most of the preconditions in order to estimate the magnitude of the significance.
The main preconditions for the reference scenario for fattening pigs are described below:
- For fattening pigs, the reference scenario is based on a housing system with “Fully slatted floor”. This has been chosen due to the fact that fully slatted floor was the most common housing system for fattening pigs in Denmark in 2006-2007 (approximately half of the housing systems for fattening pigs), according to Hanne Damgaard Poulsen (October 2008, personal communication) and Nielsen (2008) (Annex C).
- From the pre-tank in connection with the housing units the slurry is pumped to the outdoor storage.
- It is common practice that the slurry is stored outdoor in concrete slurry tanks and covered by a floating layer (Christiansen et al., 2003). In Denmark, it is required by law to cover slurry storages in order to reduce ammonia emissions and odour. When storing cattle slurry, a natural floating layer will normally be created. However, for pig slurry, a natural floating layer is less likely to occur, and a cover has to be established by the farmer (Rasmussen et al.,2001) and Christiansen et al, 2003). The use of a PVC roof is also becoming more and more common (Personal communication, S Sommer, 2008). The minimum requirement in the law is a floating layer of straw. When establishing new slurry tanks for pig slurry, the requirement in the law is permanent cover (e.g. a PVC roof) if the distance to neighbours is less than 300 metres. For the reference scenario, a floating layer has been chosen (natural for cattle slurry and by cutted straw for pig slurry) as this is the minimum requirement in the law and as this is the cheapest method (Rasmussen et al., 2001). Of course, probably not all farmers respect the minimum requirements, but the reference scenarios do not cover these situations
- The transport distance from storage to application to fields has been difficult to estimate. Udesen and Rasmussen (2003) estimate a transport distance of 2 km for farmers applying the slurry to their own fields while Rasmussen and Jørgensen (2003) use an estimate of 5 km. Pedersen (2007) has made a study of transport of slurry based on 8 cases, where the slurry was transported over respectively 0.4 km, 0.7 km, 1 km, 4.3 km, 4.5 km, 10 km, 19 km, 30 km, and 32 km. Feenstra et al. (2003) states, that demands in the Action Plan for the Aquatic Environment III 2005-2015 (Vandmiljøplan III) regarding the distribution of the surplus of phosphorus in slurry will induce that huge amounts of slurry have to be transported from areas with surplus of phosphorus (due to a high livestock density) to areas that need phosphorus. However, Feenstra et al. (2003) give no indication of distances. It has not been possible to find estimates on this increased need for transport. Jacobsen et al. (2002) wrote that there are not many Danish assessments of the transport distances for slurry. In the study by Jacobsen et al. (2002), interviews were carried out, and the interviews seemed to support the assumption that the transport distance usually is below 5 km. They found that an increase in the total slurry amount from 2000 tons of slurry to 6000 tons of slurry increased the transport distance from 700 meters to around 1100 meters. A request at the Danish Environmental Protection Agency indicated that they have no information on the average transport distance for slurry in Denmark (personal communication, K S Andersen, December 2008). Most of the alternative technologies, covered by this report, are primarily relevant for large conventional farms with relatively high livestock densities. Accordingly, it could be argued that the focus of this report should be put on relatively long transport distances. For small distances, it is common to use a tractor with trailer. If the transport of slurry to the fields is more than 10 km, transport by truck is required by law in Denmark. In this report, the calculations are based on a transport distance of 10 km. Furthermore, sensitivity analysis is carried out for transport distances of 2 km and 32 km (as 32 km is the maximum distance found by Pedersen (2007)).
- Pig slurry is applied with trail hose tankers to the field in the reference scenarios. According to Landscentret (2008), 68% of all slurry was spread by trail hose application tanker in 2004, and this is still the most common method today (Personal communication with Birkmose (2008) and Pedersen (2008)).
- Relevant soil types for pig production and application of pig slurry are clay soil and sandy soil. The LCAfood project (www.lcafood.dk) operates with two types of soil: “clayey soil” (> 10% clay) “sandy soil” (<10% clay). According to Halberg and Nielsen (2003), pig farms on clay soil constituted 29% of the total Danish pig meat production in 1999, and pig farms on sandy soil constituted 49% of the total Danish pig meat production (The remaining of the pig meat production is farmers that have mixed production with less than 10% of the income from pig production plus a small group of organic pig producers). Accordingly, the reference scenario is set up for both clayey soil and sandy soil for pig slurry. In the modelling, soil type JB3 has been used representing sandy soil and soil type JB6 has been used representing clayey soil. JB6 has been chosen because it is the most common soil type with clay > 10%. JB3 has been chosen because it is considered the best representative of sandy soil (JB1 – JB4), where JB2 is fairly rare in Denmark. 5
- It is assumed that pig slurry is applied to all crops in the crop rotation pattern, with a farm average of 140 kg N ha-¹ y-¹. According to statistics from Nehmdahl (2009) on the distribution of crop types for pig farms and cattle farms, the most common crop type for Danish pig farms is winter wheat (36.4%), followed by spring barley (19.2%) and winter barley (19.2%)(data from 2007). This is verified by experts in the area (Birkmose (2008) and Hermansen (2008)). In order to make a reasonable realistic, though simplified, crop rotation for pig farms, a six year rotation was utilised, with slurry N (kg ha-¹ y-¹) applied in parenthesis: winter barley (133.5) – winter rape (133.5) - winter wheat (133.5) – winter wheat (133.5) – spring barley with catch crop (165) – spring barley (145). According to statistics in Nielsen et al. (2008, page 501), 60% of all slurry in Denmark was applied by trailing hoses in the winter-spring period. It is assumed that the slurry is applied during spring. As mentioned in section 2, the crops are not included within the system boundaries. They are only defined, as the uptake and emissions of N and P in slurry depends on the crop, and in order to model the further fate of the N not removed with harvested products.
The main preconditions for the reference scenario for dairy cows are described below.
- For dairy cows, the reference scenario is based on a “Cubicle housing system with slatted floor (1.2 m channel)” 6. This has been chosen due to the fact that this housing system was the most common housing system for dairy cows in Denmark in 2006-2007(slightly less than half of the housing systems for dairy cows are of the type “cubicle housing system with slatted floor (1.2 m channel) according to Hanne Damgaard Poulsen (October 2008, personal communication). This is supported by Nielsen et al. (2008b).
- The slurry is pumped from the pre-tank in connection with the housing system to the outdoor storage.
- As for pig slurry, it is assumed that the cattle slurry is stored outdoor in concrete slurry tanks. When storing cattle slurry, a natural floating layerwill normally be created by the straw from the housing system, and this is regarded as a sufficient cover (Rasmussen et al., 2001 and Christiansen et al., 2003). Accordingly, the reference scenario for dairy cow slurry is based on the assumption that cut straw is notadded to the slurry tank during storage.
- The transport distance from storage to application to fields is estimated to 10 km as for pig slurry. Sensitivity analysis is made for 2 km and 32 km.
- Cattle slurry is applied with trail hose tankers to the field, as for pig slurry, with a farm average of 140 kg N ha-¹ y-¹. This is the main application method (Personal communication with Birkmose (2008) and Pedersen (2008)). Part of the cattle slurry is also applied by injection, however, this technology is not included.
- According to Birkmose (2008), cattle are primarily raised on sandy soils in Denmark. This is supported by Halberg and Nielsen (2003), who state that in 1999, 15% of the total milk production came from conventional dairy farms on clay soils, whereas 71% of the milk production came from conventional dairy farms on sandy soils (The remaining 14-15% of the milk production came from farms with mixed production and the milk production was less than 10% of the farm income). However, it has been decided to establish the reference scenarios for both sandy soils and clay soils for cattle slurry.
- According to statistics from Nehmdahl (2009) on the distribution of crop types for pig and cattle farms, the most common crop type for cattle farms is grass (20.6%), closely followed by spring barley (17.2%), and maize (15.3%)(data from 2007). In order to make a reasonable realistic, though simplified, crop rotation for cattle farms, a five year rotation was utilised, with slurry N (kg ha-¹ y-¹) applied in parenthesis: spring barley harvested as whole crop silage (156) – grass clover mixture (182) – grass clover mixture (182) – spring barley with catch crop (0) – spring barley (132). Besides this, 15 % of the area is assumed utilised for continuous silage maize (188). As for pig slurry, it is assumed that the slurry is applied by trailing hoses during spring. For cattle slurry it is assumed that it is applied to all crops.
3.2 Composition of reference slurry
The chemical composition and other characteristics of the slurry in the reference scenarios are needed, as this is the very basis for the comparison between the “traditional slurry management” in the reference scenario with the new technologies in alternative scenarios. A comparison based on different slurry types or different slurry characteristics (e.g. different content of total-N) would give unreliable results, as the emissions of e.g. NH3 are very dependent on the slurry content of N.
Furthermore, the chemical composition of the slurry is needed, as some of the environmental impacts are calculated relatively to the composition of the slurry. For example, the ammonia emissions during storage are calculated as a percentage of the ammonium content in the slurry, the methane emissions are related to the organic matter and the fertiliser value is calculated in relation to the content of N, K and P in the slurry.
However, to define a fair “reference slurry composition” has not been easy, and to relate all the new slurry management technologies to this “theoretical reference slurry composition” is even more complicated. The composition of slurry excreted from the animal depends on the type of animal, diet, the age of the animal etc. The composition of the slurry after the housing system depends to a high degree on the housing system, management, storage time etc. Even slurry from the same farm might have different slurry composition from month to month due to non-controllable factors, e.g. temperature (time of the year) and the microbial decomposition by various micro organisms.
The alternative technologies have to be related to this “reference slurry composition” as far as possible. This is not an easy task, as the environmental data for some of these technologies (e.g. energy consumption, emissions and output products) is based on measurements on slurries with compositions that might be rather different than the “reference slurry composition” defined below. However, as described above, it would give even more unrealistic results to use different slurry compositions for each technology.
The slurry composition in this report is based on the “available data”, rather than a measure of “Danish average values”, which would have been ideal.
Within each category, there are huge variations between minimum values and maximum values. The significance of some of these variations is discussed in the section “Sensitivity analysis”.
Preferably, the chemical composition of the slurry should be described in details. However, it has been difficult to find all the required data. In the reference scenario, the composition of the slurry is described by the following parameters. The parameters include:
- Dry matter (DM)7. DM is the fraction of the manure that is left after water has been evaporated due to heating at 80oC to constant weight or typically 24 hours. It typically constitutes 1 – 10% of the mass (Sommer et al., 2008).
- Ash. Ash is the remains after heating the DM at 550oC for one hour. Typically 20% of DM is ash.
- Volatile solids (VS)8. VS is the fraction of DM that volatilize when heating the DM at 550oC for one hour. This is the fraction lost during incineration. (Sommer et al., 2008). The volatile solids content is equal to the difference between the dry matter and ash (VS = DM – ash). Typically 80% of DM is VS.
- Total-N.
- Total-P
- Potassium (K)
- Carbon (C) (TOC – Total Organic Carbon).
- Copper (Cu)
- Zink (Zn)
- Density
- pH
In the alternative scenarios, it has not been possible to obtain data for all the parameters and some of them have either been based on rough estimates or they have been left out.
It was the intention to include and follow the slurry content of ammonium (NH4+) or TAN” (total available Nitrogen i.e. NH4+ + NH3) from the slurry was excretion until its application of the slurry to the field. Poulsen et al. (2001, page 130) and DJF (2008b) estimate that NH4+-N content in pig slurry is approximately 75% of total-N and approximately 60% for cattle slurry, however, this is not used in their calculations (personal communication, H Damgaard Poulsen, January 2008). The estimate by Poulsen et al. (2001) is the content in the outdoor storage tank. Hansen et al. (2008) carried out measurements on more than 500 slurry samples coming from slurry after storage right before its application to the field. They measured a content of NH4+-N corresponding to 79% of the total N in the slurry “ex storage” for fattening pigs and as 58% of the total N for dairy cows. However, it is not reasonable to assume that these NH4+ contents in the slurry after storage can be used as estimates for the NH4+ content in the slurry right after excretion and the same applies as regarding the slurry in the slurry pits in the housing units. This is because the content of NH4+ and/or TAN is, to a great degree, affected by biological processes, including nitrification (transformation of NH4+ to NO3-), denitrification (transformation of NO3- to N2), mineralization (transformation of organic N to NH4+) or immobilization (the opposite of mineralization). These biological processes depend on a range of factors, e.g. the temperature and the C:N ratio of the slurry, and it has not been possible to establish reliable balances for NH4+ nor TAN.
Accordingly, it has not been possible to include the content of NH4+ nor TAN in the slurry composition.
Due to lack of data, it has only been possible to include data on volatile solids (VS) in the reference scenario and not in the scenarios for the technologies for slurry management.
Originally, it was the intention to include sulphur (S) and emissions of hydrogen sulphide (H2S). However, it was difficult to obtain data on hydrogen sulphide emissions for a majority of the processes. The budget of the project limited the amount of effort that could be allocated to an extensive search for data and accordingly hydrogen sulphide emissions have not been included and mass balances on sulphur could not be established. The additional amount of sulphur provided by the acidification technology is included for the acidification scenario, as it has special relevance for this specific scenario.
Data on the content of magnesium, calcium and sodium has not been collected, as they are regarded as insignificant for the overall environmental results of the life cycle assessment.
The composition of the slurry in the housing units is based on the Danish Normative system for assessing manure composition (Poulsen et al. (2001) and DJF (2008a)). Poulsen et al. (2001) established the technical background report, and the yearly updated values are published by Danmarks Jordbrugs Forskning (DJF). These data are combined with data from the literature.
The “reference slurry” is defined “ex animal”, i.e. right after the animals have excreted the slurry components. This is chosen as the reference point as this is where the system boundaries start, as described above. The composition of the slurry in the reference scenario is calculated at three points:
- Slurry “ex animal”, i.e. right after excretion
- Slurry “ex housing”, i.e. in the slurry pit under the animals right before flushing to the pretank
- Slurry “ex storage”, i.e. after months of covered outdoor storage, measured right before application to field.
See figure 3.2.
Figure 3.2. The reference slurry defined “ex housing”.
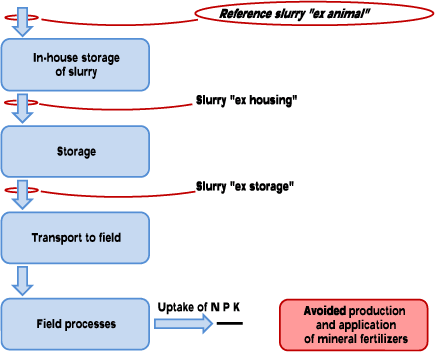
The chemical composition of pig slurry is given in table 3.1. The composition of dairy cow slurry is given in table 3.2. The explanations for the composition are given in Annex A. The “ex storage” values are lower than the “ex housing” values due to the dilution with rain water during the outdoor storage and due to degradation. The number of digits should not be seen as a measure of the precision, but is only included as the values are the foundation for further calculations. The data for dry matter (DM), nitrogen (N), phosphorus (P) and potassium (K) are based on the Danish Normative system for assessing manure composition (Poulsen et al. (2001), DJF (2008a) and DJF (2008b)). The rest of the data is based on various references, see Annex A.
Table 3.1. Characteristics of slurry from fattening pigs in the reference scenario. Per 1000 kg of slurry “ex animal”, “ex housing” and “ex storage”.
|
Ex
Animal |
Ex
housing |
Ex
storage |
Total mass |
1000 kg
Slurry
ex animal |
1000 kg
Slurry
ex housing |
1000 kg
Slurry
ex storage |
Dry matter (DM) |
77.4 kg |
69.7 kg |
61 kg |
Ash content |
13.2 kg |
13.2 kg |
12.2 kg |
Volatile solids (VS) |
64.2 kg |
56.5 kg |
48.8 kg |
Of total VS:
- easily degradable |
41.7 kg |
34.0 kg |
28.1 kg |
- heavyly degradable |
22.5 kg |
22.5 kg |
20.7 kg |
Total-N (DJF, 2008) |
6.60 kg |
5.54 kg |
5.00 kg |
Total-N in this study |
6.60 kg |
5.48 kg |
4.80 kg |
NH4+-N |
No data |
No data |
3.60 kg |
Total-P |
1.13 kg |
1.13 kg |
1.04 kg |
Potassium (K) |
2.85 kg |
2.85 kg |
2.60 kg |
Carbon (C) |
37.0 kg |
33.3 kg |
29.2 kg |
Copper (Cu) |
30.0 g |
30.0 g |
27.6 g |
Zinc (Zn) |
89.4 g |
89.4 g |
82.4 g |
Density |
1053 kg per m³ |
1053 kg per m³ |
1053 kg per m³ |
pH |
7.8 |
7.8 |
7.8 |
Table 3.2. Characteristics of slurry from dairy cows in the reference scenario. Per 1000 kg of slurry “ex animal”, “ex housing” and “ex storage”.
|
Ex
Animal |
Ex
housing |
Ex
storage |
Total mass |
1000 kg slurry ”ex animal” |
1000 kg slurry ”ex housing” |
1000 kg slurry
”ex storage” |
Dry matter (DM) |
125.7 kg |
113.2 kg |
103 kg |
Ash content |
21.5 kg |
21.5 kg |
20.6 kg |
Volatile solids (VS) |
104.2 kg |
91.7 kg |
82.4 kg |
Of total VS:
- easily degradable |
50.0 kg |
37.5 kg |
30.5 kg |
- heavy degradable |
54.2 kg |
54.2 kg |
51.9 kg |
Total-N (DJF, 2008) |
6.87 kg |
6.41 kg |
6.02 kg |
Total-N in this study |
6.87 kg |
6.34 kg |
5.79 kg |
NH4+-N |
No data |
No data |
3.47 kg |
Total-P |
1.02 kg |
1.03 kg |
0.98 kg |
Potassium (K) |
5.81 kg |
5.90 kg |
5.65 kg |
Carbon (C) |
55.2 kg |
49.7 kg |
45.2 kg |
Copper (Cu) |
12.1 kg |
12.1 kg |
11.6 g |
Zinc (Zn) |
23.4 kg |
23.4 kg |
22.4 g |
Density |
1053 kg per m³ |
1053 kg per m³ |
1053 kg per m³ |
pH |
7.8 |
7.8 |
7.8 |
3.3 Data for the reference scenario
Data for the reference scenario is to a high degree based on two main references: Data from the Danish Normative system for assessing manure composition (Poulsen et al. (2001), DJF (2008a) and DJF (2008b)) and IPCC (2006). These data should be regarded as rather “static and rough estimates”. These data have been used as these are widely used (e.g. for national and international statistics for Green House Gas calculations and for the yearly publications from Plantedirektoratet containing the requirements for the farmers’ fertiliser accounts according to Danish Law (Plantedirektoratet, 2008). Furthermore, the budget for this study could not include sophisticated modelling of the emissions. However, it is has not been without problems using the Danish Normative system for assessing manure composition in combination with the data from IPCC (2006). First of all, the two references are not in accordance regarding mass balances – the loss of C due to CH4 emissions in IPCC (2006) is not in accordance with the DM loss estimated by DJF (2008b). Secondly, the data are rather “static”. The Danish Normative system do not specify the retention time in the housing units, the pre-tank or the outdoor storage. Furthermore, there is no specification of the emissions from the pre-tank (accordingly, these are included in the data for outdoor storage in this study).
The main problem using data from IPCC (2006) is that the data are very “generic and static”. As example could be mentioned the N2O emission from application of slurry to field, which is 1% of the N applied – regardless of soil type and local conditions (see Annex A). The IPCC estimate for N2O is used for the Green House Gas calculations worldwide, and special Danish conditions are not taken into account. Another problem that could be mentioned is the in-house CH4 emissions. In IPCC (2006), the emission factors (in kg CH4 per kg VS) is 5.67 times higher for “storage > 1 month” than for “storage > 1 month” which is an unrealistic jump. The emission of CH4 should rather have been modelled as a function of time. The CH4 emissions depend on a range of factors, among these the retention time in the housing units, temperature and on the biological activity. As the CH4 emissions for the slurry management technologies are calculated relative to the CH4 emissions in this reference scenario, the significance of the uncertainty is reduced slightly for the comparisons to the new technologies.
3.4 Results of the Impact Assessment
3.4.1 Overall results of the impact assessment for the reference scenarios
The relative contributions to the environmental impact categories and resource consumptions for the reference scenario for pig slurry are shown in figure 3.3 and for dairy cow slurry in figure 3.4.
The contributions to each impact category are explained in the following sections.
The positive values (to the right) are the contributions to the environmental impacts and resource consumptions by the management of the pig slurry and dairy cow slurry.
The negative values (to the left) are “avoided environmental impacts”, because the nutrient content of pig and dairy cow slurry replace mineral fertilisers (if slurry was not applied to the field, the farmer would apply mineral fertilisers to the field instead). When the fertilisers are replaced, the production and application of these are avoided, and accordingly the system obtains a “deduction” when the mineral fertilisers are subtracted from the system.
The numbering of processes (“A2” and “A3” refers to the number of the section in Annex A, where the processes are described).
Figure 3.3. environmental impacts and resource consumption from the reference scenario for pig slurry. soil type “JB3” and “JB6”. 10 and 100 years time horizon for global warming and for aquatic eutrophication (N).
Click here to see Figure 3.3
Figure 3.4. environmental impacts and resource consumption from the reference scenario for dairy cow slurry. soil type “JB3” and “JB6”. 10 and 100 years time horizon for global warming and for aquatic eutrophication (N).
Click here to see Figure 3.4
3.4.2 Normalised results
In figure 3.5, the “normalised” environmental impacts for pig slurry and dairy cow slurry are shown. The environmental impacts are normalised by use of the EDIP method, i.e. dividing the contributions from the slurry by the yearly average contribution from a person. The unit for the normalised impacts are “Person Equivalents”, and the normalised impacts can be interpreted as “a percent of the average yearly contribution by a person”. As can be seen at figure 3.5, the contribution from 1000 kg pig slurry contributes to the global warming by approximately 3.3 % of one person’s yearly contribution to the global warming.
It has not been possible to normalise the contributions to “respiratory inorganics”, as the factors for these are based on the IMPACT 2002+ method, as explained in section 2.7.
The category “Non-renewable energy” is also based on the IMPACT 2002+ method. The IMPACT 2002+ method includes a normalisation factor for this category, based on the average energy consumption by an “average European”. The exact value should be taken with care, as it is not part of the EDIP method used for the normalisation of all the other categories. However, it provides an indication of the magnitude.
The normalisation factor for phosphorus is based on Nielsen and Wenzel (2005), who calculated that the average usage of phosphate rock is in the order of 22 kg per world citizen per year. There is significant uncertainty on this figure. However, it provides an indication of the magnitude.
When interpreting the normalised results, it should be kept in mind, that normalised results are not a measure of the relative importance of the environmental impacts. However, the normalised results are very close to the weighted results by the EDIP method, as the weighting factors are all in the range of 1.1-1.4 (except for ozone layer depletion, which is not included in this study).
From the normalised data in figure 3.5, it can be seen that:
- Slurry management has a relatively large contribution to “Aquatic eutrophication (nitrogen compounds)” compared to the other impact categories. The contribution by the slurry is partly counterbalanced by the avoided contribution from the replaced mineral N fertilisers, and when regarding “net contributions” (contribution minus avoided), the contribution to aquatic eutrophication (N) is at the same magnitude as most of the other environmental impacts.
- The contribution to “Aquatic eutrophication (phosphorus)” is also realitively large. However, this is counterbalanced by the avoided contributions from mineral P fertilisers.
- The phosphorous resources, which is saved due to the use of slurry at the field replacing mineral P fertiliser is relatively large.
- The normalised consumption of non-renewable energy is relativity small compared to the other categories. It means that the energy consumption of the systems is not a “big issue”.
Figure 3.5. Normalised contributions to environmental impacts from the reference scenario for pig slurry and dairy cow slurry. soil type “JB3” and 10 years time horizon for global warming and for aquatic eutrophication (N).
Click here to see Figure 3.5
3.4.3 Global warming
For pig slurry as well as dairy cow slurry, the main contributions to global warming come from the emissions from the field processes, the outdoor storage of slurry and the emissions from the indoor storage of slurry, see figure 3.3 and 3.4. The contributions from the indoor storage and the outdoor storage are totally dominated by contributions from CH4. The contributions from the field are caused by CO2 and N2O emissions.
The “negative contributions” to the global warming (to the left in figure 3.3 and 3.4) is due to the avoided fertilisers (predominantly due to the avoided N mineral fertiliser). As described in Annex A, the slurry contains N, P and K nutrients, which replace application and production of mineral fertilisers. As the application of mineral N fertiliser leads to N2O emissions from the soil, and as the production of N mineral fertiliser leads to N2O emission during the production of nitric acid from ammonia, these are avoided. The application of slurry also leads to N2O emission. However, due to the huge uncertainty on the N2O emission factors from both slurry and mineral N fertiliser, it is not possible to clearly express whether the application of slurry leads to a net reduction of the total N2O emission or opposite. In this study, the same N2O emission factor has been used for the field emissions of N2O from slurry and from mineral N fertilisers per kg N.
In table 3.3 the contributions to Global Warming from management of pig slurry in the reference scenario is shown. The contributions from dairy cow slurry are shown in table 3.4. The % should be taken with care due to the high uncertainties – it is only a very rough estimate!
Table 3.3. Contributions to global warming from pig slurry in the reference scenario.
Per 1000 kg of slurry “ex animal”. The % should be taken with care due to the high uncertainties – it is rather rough estimates! The number of digits is not an expression of the uncertainty.
Contributions to Global warming |
kg CO2 eq |
Uncertainty
Range
kg CO2 eq. |
% of total positive contribution |
Comments and reference on uncertainty |
CH4 |
|
|
|
|
CH4 from in-house storage |
75.7 |
[ 7 – 150 ] |
25.3% |
Based on IPCC (2006). If IPCC data for < 1 month storage had been chosen, the emissions would be a factor 5.67 times lower. In addition, an uncertainty on a factor 2 is assumed as on the other data from IPCC (2006), see below. |
CH4 from “Storage” (in pre-tank and outdoor storage) |
44.6 |
[ 15 – 134 ] |
14.9% |
Data based on IPCC (2006). IPCC (2006) does not estimate the uncertainty. The uncertainty must be high – the emissions depend on storage time which is not included in the IPCC model. Estimated uncertainty: A factor 3. |
N2O |
|
|
|
|
N2O from in-house storage |
11.2 |
[ 5.6 – 22] |
3.7% |
IPCC (2006) estimates that the uncertainty for this is a factor 2. |
N2O from storage (pre-tank and outdoor storage) |
16.0 |
[ 8 – 32 ] |
5.3% |
IPCC (2006) estimates that the uncertainty for this is a factor 2. |
N2O from field |
34.9 |
[ 12 – 105 ] |
11.7% |
IPCC (2006) estimates that the uncertainty for this is a factor 3. |
Avoided N2O due to avoided production of mineral fertilisers and application of these to field |
-32.0 |
[ - 11 – - 96] |
-10.7% |
IPCC (2006) estimates that the uncertainty for this is a factor 3. The uncertainties on the N2O emission from production of mineral N fertiliser in the SimaPro database is at the same magnitude (see Annex A). |
CO2 |
|
|
|
|
CO2 from electricity for pumping and stirring |
3.6 |
[1.8 – 7.3 ] |
1.2% |
Estimate: Á factor 2 (mainly on the electricity consumption by the gear) |
CO2from transport |
3.1 |
[ 1.5 - 6 ] |
1.0% |
Estimate: A factor 2. |
Avoided CO2 due to avoided production of mineral fertilisers |
-17.5 |
[ -9- -26 ] |
-5.9% |
Change if other marginal N and P fertiliser was chosen, see Annex A.
Sensitivity analysis in SimaPro. |
CO2 from field processes from avoided mineral N fertiliser ¹ (biogenic CO2) |
14.3 |
[ 9- 20 ] |
4.8% |
Estimate of the uncertainty: ± 40% |
CO2 from field processes (during 10 years) (biogenic CO2)) |
88.7 |
[53-124] |
29.6% |
Estimate of the uncertainty: ± 40% |
Residue |
|
|
|
|
Residue from other processes |
5.6 |
Not estimated |
1.9% |
|
TOTALS |
|
|
|
|
Total POSITIVE contributions |
299.3 |
[150-600] |
100% |
Rough estimate: A factor 2 |
Total AVOIDED contributions |
-51.2 |
[-25--100] |
-17.1% |
Rough estimate: A factor 2 |
Total NET contribution
(= positive - negative) |
248.0 |
[125-500] |
82.9% |
Rough estimate: A factor 2 |
1 As explained in table A.18 in Annex A, application of mineral N fertiliser gives rise to extra soils C storage during the 10 years time horizon due to more residues from a larger crop (C-tool). This means that application of extra mineral N fertiliser saves emissions of CO2, and opposite: Application of less mineral N fertiliser means that extra C is not stored in the soil, accordingly it corresponds to a positive contribution to global warming.
Table 3.4. Contributions to global warming from dairy cow slurry in the reference scenario. Per 1000 kg of slurry “ex animal”. The % should be taken with care due to the high uncertainties – it is rather rough estimates! The number of digits is not an expression of the uncertainty.
Contributions to Global warming |
kg CO2 eq |
Uncertainty
Range
kg CO2 eq. |
% of total positive contribution |
Comments and reference on uncertainty |
CH4 |
|
|
|
|
CH4 from in-house storage |
65.6 |
[ 6 – 131 ] |
19.2% |
Based on IPCC (2006). If IPCC data for < 1 month storage had been chosen, the emissions would be a factor 5.67 times lower. In addition, an uncertainty on a factor 2 is assumed as on the other data from IPCC (2006), see below. |
CH4 from “Storage” (in pre-tank and outdoor storage) |
38.6 |
[ 13 – 116 ] |
11.3% |
Data based on IPCC (2006). IPCC (2006) does not estimate the uncertainty. The uncertainty must be high – the emissions depend on storage time which is not included in the IPCC model. Estimated uncertainty: A factor 3. |
N2O |
|
|
|
|
N2O from in-house storage |
9.3 |
[ 4.7 – 19] |
2.7% |
IPCC (2006) estimates that the uncertainty for this is a factor 2. |
N2O from storage (pre-tank and outdoor storage) |
16.6 |
[ 8 – 33 ] |
4.8% |
IPCC (2006) estimates that the uncertainty for this is a factor 2. |
N2O from field |
39.8 |
[ 13 – 119 ] |
11.6% |
IPCC (2006) estimates that the uncertainty for this is a factor 3. |
Avoided N2O due to avoided production of mineral fertilisers and application of these to field |
-34.7 |
[-12 – - 104] |
-10.1% |
IPCC (2006) estimates that the uncertainty for this is a factor 3. The uncertainties on the N2O emission from production of mineral N fertiliser in the SimaPro database is at the same magnitude (see Annex A). |
CO2 |
|
|
|
|
CO2 from electricity for pumping and stirring |
2.6 |
[ 1.3 – 5.2 ] |
0.8% |
Estimate: Á factor 2 (mainly on the electricity consumption by the gear) |
CO2from transport |
2.9 |
[ 1.5 - 6 ] |
0.9% |
Estimate: A factor 2. |
Avoided CO2 due to avoided production of mineral fertilisers |
-19.5 |
[ -10- -30 ] |
-5.7% |
Change if other marginal N and P fertiliser was chosen, see Annex A.
Sensitivity analysis in SimaPro. |
CO2 from field processes from avoided mineral N fertiliser ¹ (biogenic CO2) |
15.5 |
[ 9- 22 ] |
4.5% |
Estimate of the uncertainty: ± 40% |
CO2 from field processes (during 10 years) (biogenic CO2)) |
132 |
[79-185] |
38.6% |
Estimate of the uncertainty: ± 40% |
Residue |
|
|
|
|
Residue from other processes |
17.3 |
Not estimated |
5.1% |
|
TOTALS |
|
|
|
|
Total POSITIVE contributions |
342.1 |
[170-685] |
100% |
Rough estimate: A factor 2 |
Total AVOIDED contributions |
-56.0 |
[-28-112] |
-16.4% |
Rough estimate: A factor 2 |
Total NET contribution
(= positive - negative) |
286.1 |
[140-570] |
83.6% |
Rough estimate: A factor 2 |
1 As explained in table A.18 in Annex A, application of mineral N fertiliser gives rise to extra soils C storage during the 10 years time horizon due to more residues from a larger crop (C-tool). This means that application of extra mineral N fertiliser saves emissions of CO2, and opposite: Application of less mineral N fertiliser means that extra C is not stored in the soil, accordingly it corresponds to a positive contribution to global warming.
In order to assess the significance of some of the assumptions made, and as some of the data used for this Life Cycle Assessment has a high uncertainty, sensitivity analysis has been carried out for a variety of parameters:
From table 3.3 and table 3.4 it can be seen that:
- When taking the uncertainties into consideration, there is no statistical difference between the contributions from pig slurry and dairy cow slurry, except for the field processes.
- The main contributions come from CH4 in the housing units, CH4 during storage, N2O from field processes and biogenic CO2 from field processes.
- The emissions of CH4 are generally found to have a very high uncertainty, as described in Annex A. They are based on rough estimates rather than “well founded scientific research”. A critical point to mention is the calculation of the CH4 emissions from indoor storage. As mentioned in Annex A, the uncertainty on the CH4 emissions from the housing units is high. The emission factors (in kg CH4 per kg VS) is 5.67 times higher for “storage > 1 month” than for “storage < 1 month” which is an unrealistic jump. The emission of CH4 should rather have been modelled as a function of time. In this study, the high emission factor from IPCC (2006) has been used as a conservative estimate. It has not been possible to improve the estimates - the area needs more scientific research.
- The CO2 emissions from the housing units and the outdoor storage are based on mass balances, based on the rough estimate of DM loss in the Danish Norm data (Poulsen et al., 2001) and DJF (2008). However, as the CH4 emissions have a global warming potential that is 23 times higher than CO2, the uncertainties of CH4 are more important.
- The CO2 emissions from the field have a relatively high contribution to global warming (approximately at the same level as the CH4 emissions from the housing units). Hence these are also important. The CO2 emissions from the field are modelled by the use of C-TOOL. The CO2 emissions are very dependent on the time horizon. In this study the calculation is based on a 10 years perspective, but if using a 100 years perspective, the CO2 emissions will be 22% higher for both pig slurry and dairy cow slurry.
- The N2O emissions from fields are very important, and the factors are very uncertain. The N2O emissions depend to a great extent on the soil conditions and could be modelled in advanced models. However, this has not been possible within the budget and frames of this study. The direct N2O emissions are based on IPPC (2006, table 11.1).
- Sensitivity analysis on the transport shows that transport of slurry from the outdoor storage tank to the fields has no significance for the overall contributions to global warming.
- Furthermore, the electricity consumption for pumping and stirring is relatively unimportant for the overall contributions to global warming from slurry. Accordingly, a sensitivity analysis on the marginal electricity production will not change the results.
- The choice of marginal N and P fertiliser also influences the results – the “avoided contribution” to global warming. However, the uncertainty on the N2O emissions from field dominates the overall uncertainty for the avoided contribution from mineral fertilisers.
- The difference between soil types JB3 and soil type JB6 is very small and it has no significance for the overall contributions to global warming (see figure 3.3. and 3.4).
- After a period of 100 years, the CO2 emissions from the applied slurry to the soil are higher than after a period of 10 years, which is visible at figure 3.3 and 3.4. The increase is only partly counterbalanced by the differences in CO2 emissions from the replaced mineral N fertiliser. As explained in table A.18 in Annex A, application of mineral N fertiliser gives rise to extra storage of C in the soil during the 10 years time horizon due to more residues from a larger crop. This means that application of extra mineral N fertiliser reduces the emissions of CO2, and opposite: Application of less mineral N fertiliser means that less C is stored in the soil, accordingly it corresponds to a positive net contribution to global warming. The positive CO2 contribution is higher on a 100 year horizon than on a 10 year horizon.
The incorporation of C in soil for the reference scenario is shown in table 4.1 and 4.2 in the end of section 4. For pig slurry, a net amount of 3.6 kg of C (per 1000 kg pig slurry “ex animal”) is still remaining in the soil after a period of 10 years, corresponding to 13.2 kg CO2. After 100 years, the net amount of C remaining in the soil is in the magnitude of 1 kg C, corresponding to 3.8 kg CO2. For dairy cow slurry, the net amount of stored C is in the soil is approximately 7 kg after 10 years, and 2 kg C after 100 years.
According to the uncertainty on the data, the relative contributions from CH4 emissions from the housing units, CH4 emissions from the outdoor storage and the CO2 and N2O emissions from the fields could be different than shown in figure 3.3 (for pig slurry) and figure 3.4 (for dairy cow slurry). However, in spite of these uncertainties, there is no doubt, that these are the important emissions contribution to the global warming for both pig slurry and dairy cow slurry.
3.4.4 Acidification
The main contributions to acidification come from the in-house storage of slurry. Furthermore, significant contributions come from the field processes and the outdoor storage also contributes some. As can be seen when comparing figure 3.3 and 3.4, the relative contribution from the housing units is higher for pig slurry than for dairy cow slurry.
As can be seen from table 3.5 and 3.6, the contribution to acidification is totally dominated by NH3 emissions. The unit for the characterised results in table 3.5 are expressed as the area of ecosystem within the full deposition area which is brought to exceed the critical load of acidification as a consequence of the emission (area of unprotected ecosystem, i.e.m² UES), as defined by the EDIP 2003 method (Hauschild et al, 2005).
Table 3.5. Contributions to acidification from pig slurry in the reference scenario.
Per 1000 kg of slurry “ex animal”. The % should be taken with care due to the high uncertainties – it is rather rough estimates! The number of digits is not an expression of the uncertainty.
Contributions to Acidification |
m² UES |
Uncertainty
Range
m² UES |
% of total positive contribution |
Comments and reference on uncertainty |
NH3 |
|
|
|
|
NH3 from in-house storage |
29.8 |
[ 15 – 60] |
59.2% |
Uncertainty not stated. It is estimated that the uncertainty for this is a factor 2. |
NH3 from “Storage” (in pre-tank and outdoor storage) |
3.1 |
[ 1.5 – 6 ] |
6.1% |
Uncertainty not stated. It is estimated that the uncertainty for this is a factor 2. |
NH3 from field |
15.3 |
[ 7.6 – 31] |
30.4% |
Uncertainty not stated. It is estimated that the uncertainty for this is a factor 2. |
Avoided NH3 from avoided mineral fertilisers |
-2.9 |
[ -1.4 – -6] |
-5.7% |
Uncertainty not stated. It is estimated that the uncertainty for this is a factor 2. |
NOX |
|
|
|
|
NOX from slurry (in-house, storage, and field) |
1.6 |
[ 0.8 – 3] |
3.3% |
Uncertainty not stated. It is estimated that the uncertainty for this is a factor 2. |
NOX from electricity for pumping and stirring |
0.03 |
[0.02-0.07] |
0.1% |
Uncertainty not stated. It is estimated that the uncertainty for this is a factor 2. |
NOX from transport |
0.2 |
[0.1-0.4] |
0.4% |
Uncertainty not stated. It is estimated that the uncertainty for this is a factor 2. |
NOX from avoided production of fertilisers |
-0.9 |
[-0.43- - 1.7] |
-1.7% |
Uncertainty not stated. It is estimated that the uncertainty for this is a factor 2. |
SO2 |
|
|
|
|
SO2from transport |
0.1 |
[0.05 –0.2] |
0.2% |
Uncertainty not stated. It is estimated that the uncertainty for this is a factor 2. |
Avoided SO2 due to avoided production of mineral fertilisers |
-1.7 |
[ -0.8- -3.4] |
-3.3% |
Uncertainty not stated. It is estimated that the uncertainty for this is a factor 2. |
Residue |
|
|
|
|
Residue from other processes |
0.1 |
Not estimated |
0.2% |
|
TOTALS |
|
|
|
|
Total POSITIVE contributions |
50.3 |
[25-100] |
100% |
Rough estimate: A factor 2 |
Total AVOIDED contributions |
-5.5 |
[-2.7--11] |
-10.6% |
Rough estimate: A factor 2 |
Total NET contribution
(= positive - negative) |
44.8 |
[22-90] |
89.1% |
Rough estimate: A factor 2 |
Table 3.6. Contributions to acidification from dairy cow slurry in the reference scenario. Per 1000 kg of slurry “ex animal”. The % should be taken with care due to the high uncertainties – it is rather rough estimates! The number of digits is not an expression of the uncertainty.
Contributions to Acidification |
m² UES |
Uncertainty
Range
m² UES |
% of total positive contribution |
Comments and reference on uncertainty |
NH3 |
|
|
|
|
NH3 from in-house storage |
15.4 |
[ 8 – 31] |
35.7% |
Uncertainty not stated. It is estimated that the uncertainty for this is a factor 2. |
NH3 from “Storage” (in pre-tank and outdoor storage) |
3.7 |
[ 2 – 7 ] |
8.4% |
Uncertainty not stated. It is estimated that the uncertainty for this is a factor 2. |
NH3 from field |
22 |
[ 11 – 44] |
50.9% |
Uncertainty not stated. It is estimated that the uncertainty for this is a factor 2. |
Avoided NH3 from avoided mineral fertilisers |
-3.1 |
[ -1.6 – -6] |
-7.2% |
Uncertainty not stated. It is estimated that the uncertainty for this is a factor 2. |
NOX |
|
|
|
|
NOX from slurry (in-house, storage, and field) |
1.7 |
[ 0.9 – 3.4] |
3.9% |
Uncertainty not stated. It is estimated that the uncertainty for this is a factor 2. |
NOX from electricity for pumping and stirring |
0.02 |
[0.01-0.05] |
0.1% |
Uncertainty not stated. It is estimated that the uncertainty for this is a factor 2. |
NOX from transport |
0.2 |
[0.1-0.4] |
0.4% |
Uncertainty not stated. It is estimated that the uncertainty for this is a factor 2. |
NOX from avoided production of fertilisers |
-0.9 |
[-0.5- - 1.9] |
-2.2% |
Uncertainty not stated. It is estimated that the uncertainty for this is a factor 2. |
SO2 |
|
|
|
|
SO2from transport |
0.1 |
[0.05 – 0.2] |
0.2% |
Uncertainty not stated. It is estimated that the uncertainty for this is a factor 2. |
Avoided SO2 due to avoided production of mineral fertilisers |
- 1.8 |
[ -1- -4] |
-4.1% |
Uncertainty not stated. It is estimated that the uncertainty for this is a factor 2. |
Residue |
|
|
|
|
Residue from other processes |
0.05 |
Not estimated |
0.1% |
|
TOTALS |
|
|
|
|
Total POSITIVE contributions |
43.3 |
[22-87] |
100% |
Rough estimate: A factor 2 |
Total AVOIDED contributions |
-5.9 |
[-3--12] |
-13.7% |
Rough estimate: A factor 2 |
Total NET contribution
(= positive - negative) |
37.3 |
[19-75] |
86.3% |
Rough estimate: A factor 2 |
As can be seen from table 3.5 and 3.6, less than 4% of the contributions to acidification come from nitrogen oxides, and less than 0.5% from electricity production and transport. The avoided production of mineral fertilisers leads to a small amount of nitrogen oxides and sulphur dioxide emissions being avoided, however it corresponds to less than 6% of the total contribution to acidification. Again, the relative contributions of NH3 from the in-house storage of slurry, the field processes and the outdoor storage are very dependent on the uncertainties on the data.
The NH3 emissions are mainly based on the Danish Norm Data. The uncertainty on these data is not stated, and the ammonia emissions are very dependent on temperature, biological activity and slurry composition (e.g. pH), however, it is assumed that the NH3 emissions used in this report is a rather good estimate for the “average Danish conditions”.
The results of the Life Cycle assessment for the reference scenario show that:
- Even when acknowledging that there is uncertainty on the Danish Norm data for NH3 emissions, it is likely that the contributions to acidification mainly come from in-house emissions of NH3, field emissions of NH3 and outdoor storage NH3 emissions. It is likely, that the relative contributions are as shown in figure 3.3 for pig slurry and 3.4 for dairy cow slurry.
- Transport of slurry from outdoor storage to field is unimportant for the overall contributions to acidification.
- The electricity consumption for pumping and stirring is relatively unimportant for the overall contributions to acidification.
3.4.5 Aquatic eutrophication (N)
In table 3.7 and 3.8 the contributions to aquatic N-eutrophication are shown in N-equivalents. The EDIP 2003 method includes modelling of the fate of the substances, and the EDIP 2003 impact potential thus represents the fraction of the emission which can actually be expected to reach different aquatic systems (Hauschild et al., 2005) (i.e. not all of 1 kg N leached actually reach aquatic systems). The contributions to aquatic eutrophication (N) are dominated by nitrogen leaching from the field. NH3 emissions from the slurry also contribute to some extent (contributions from the indoor storage is due to NH3 emissions in figure 3.3. and 3.4). The application of slurry leads to nitrogen leaching, however, this is partly counterbalanced by the avoided N leaching due to the replaced amount of mineral N fertiliser.
As can be seen from figure 3.3 and 3.4, the N leaching from the slurry is significantly lower from soil type JB6 than from soil type JB3. However, as the N leaching from the mineral N fertiliser is also lower, the net leaching is within the same order of magnitude (only slightly lower for JB6, a maximum of 10% difference for the net contributions from the overall system).
As can be seen from figure 3.3 and 3.4, there are significant differences between the 10 years time horizon and the 100 years time horizon. The nitrogen leaching from the slurry is significant higher during the 100 years, however, this is to some extent counterbalanced because the avoided nitrogen leaching from the replaced mineral N fertiliser is also higher. In fact, the “net contribution” is less than 4% higher on a 100 year horizon than for the 10 year horizon (pig slurry, JB3 and JB6). For dairy cow slurry the difference is less than 3% for both soil type JB3 and JB6. The reason for the higher nitrogen leaching at the long time-scale is the mineralisation of organic matter, of which a substantial part goes to leaching.
Table 3.7. Contributions to aquatic N-eutrophication from pig slurry in the reference scenario. Per 1000 kg of slurry “ex animal”. The % should be taken with care due to the high uncertainties – it is rather rough estimates! The number of digits is not an expression of the uncertainty.
Contributions to Aquatic
N-eutrophication |
N-equivalents |
Uncertainty
Range
N-eq. |
% of total positive contribution |
Comments and reference on uncertainty |
NH3 |
|
|
|
|
NH3 from in-house storage |
0.24 |
[ 0.12 – 0.48] |
16.1% |
Uncertainty not stated. It is estimated that the uncertainty for this is a factor 2. |
NH3 from “Storage” (in pre-tank and outdoor storage) |
0.025 |
[ 0.013 – 0.05 ] |
1.7% |
Uncertainty not stated. It is estimated that the uncertainty for this is a factor 2. |
NH3 from field |
0.13 |
[ 0.06 – 0.25] |
8.2% |
Uncertainty not stated. It is estimated that the uncertainty for this is a factor 2. |
Avoided NH3 from avoided mineral fertilisers |
-0.024 |
[-0.012– -0.05] |
-1.6% |
Uncertainty not stated. It is estimated that the uncertainty for this is a factor 2. |
N leaching from field |
|
|
|
|
N leaching from fields (from slurry) |
1.1 |
[ 0.6 – 2.2] |
72.7% |
Uncertainty not stated. It is estimated that the uncertainty for this is a factor 1.5. |
Avoided N leaching from fields (due to avoided mineral N fertilisers) |
-0.9 |
[ -0.5- -1.8 ] |
- 58.9% |
Uncertainty not stated. It is estimated that the uncertainty for this is a factor 1.5. |
NOX |
|
|
|
|
NOX total in system |
0.011 |
[0.006-0.02] |
0.7% |
Uncertainty not stated. It is estimated that the uncertainty for this is a factor 2. |
Residue |
|
|
|
|
Residue from other processes |
Almost 0 |
Not estimated |
<0.2% |
|
TOTALS |
|
|
|
|
Total POSITIVE contributions |
1.51 |
[ 0.8-3.0 ] |
100% |
Rough estimate: A factor 2 |
Total AVOIDED contributions |
-0.93 |
[ -0.5- -1.9] |
-61.3% |
Rough estimate: A factor 2 |
Total NET contribution
(= positive - negative) |
0.59 |
[0.3 – 1.2] |
38.7% |
Rough estimate: A factor 2 |
Table 3.8. Contributions to aquatic N-eutrophication from dairy cow slurry in the reference scenario. Per 1000 kg of slurry “ex animal”. The % should be taken with care due to the high uncertainties – it is rather rough estimates! The number of digits is not an expression of the uncertainty.
Contributions to Aquatic
N-eutrophication |
N-equivalents |
Uncertainty
Range
N-eq. |
% of total positive contribution |
Comments and reference on uncertainty |
NH3 |
|
|
|
|
NH3 from in-house storage |
0.13 |
[ 0.06 – 0.25] |
8.1% |
Uncertainty not stated. It is estimated that the uncertainty for this is a factor 2. |
NH3 from “Storage” (in pre-tank and outdoor storage) |
0.03 |
[ 0.015 – 0.06 ] |
1.9% |
Uncertainty not stated. It is estimated that the uncertainty for this is a factor 2. |
NH3 from field |
0.18 |
[ 0.09 – 0.36] |
11.6% |
Uncertainty not stated. It is estimated that the uncertainty for this is a factor 2. |
Avoided NH3 from avoided mineral fertilisers |
-0.025 |
[-0.013– -0.05] |
-1.6% |
Uncertainty not stated. It is estimated that the uncertainty for this is a factor 2. |
N leaching from field |
|
|
|
|
N leaching from fields (from slurry) |
1.2 |
[ 0.6 – 2.4] |
77.0% |
Uncertainty not stated. It is estimated that the uncertainty for this is a factor 2. |
Avoided N leaching from fields (due to avoided mineral N fertilisers) |
-0.96 |
[ -0.5- -1.9 ] |
- 62.0% |
Uncertainty not stated. It is estimated that the uncertainty for this is a factor 1.5. |
NOX |
|
|
|
|
NOX total in system |
0.01 |
[0.005-0.02] |
0.7% |
Uncertainty not stated. It is estimated that the uncertainty for this is a factor 2. |
Residue |
|
|
|
|
Residue from other processes |
Almost 0 |
Not estimated |
<0.2% |
|
TOTALS |
|
|
|
|
Total POSITIVE contributions |
1.55 |
[ 0.8-3.1 ] |
100% |
Rough estimate: A factor 2 |
Total AVOIDED contributions |
-1.00 |
[ -0.5- -2.0] |
-64.5% |
Rough estimate: A factor 2 |
Total NET contribution
(= positive - negative) |
0.55 |
[0.28 – 1.1] |
35.5% |
Rough estimate: A factor 2 |
3.4.6 Aquatic eutrophication (P)
The results for the aquatic phosphorous eutrophication (P) have a very high uncertainty due to lack of data and due to that it has not been possible to apply advanced modelling within the frames of the study. The results are mainly affected by two rough assumptions: The assumption that 0.6% of the applied phosphorus is leaching and reach the aquatic environment (both for animal slurry and for mineral fertilisers) and the assumptions related to the data for the leaching of P from production of mineral fertilisers (see Annex A, section A.5.6). Furthermore, there is significant uncertainty on the Life Cycle Inventory data for the production of mineral P fertiliser.
First of all, there is a high uncertainty on the fraction of phosphorus that is actually leaching. However, as it is assumed to be the same for P leaching from slurry and P leaching from the replaced mineral fertiliser, the uncertainty to some degree “outbalance” each other. The assumption that 0.6% of the P are leaching and reach the aquatic environment have a high uncertainty and depends to a high degree on the soil type and the content of phosphorus in the soil. Soils in areas with a high density of animals (pigs or cattle) generally have a higher content of P than soils in areas with a low density of animals. In figure 3.3 and 3.4 it seems like that the amount of P leaching from the slurry is more than counterbalanced by the same amount of P leaching from the avoided mineral P fertiliser. However, this is only correct if the leaching from 1 kg P in slurry is identical to the leaching from 1 kg P in mineral fertilisers, which might not always be the case. The discussion of P leaching from slurry vs. mineral fertilisers is beyond the scope of this study.
As discussed in Annex A, sensitivity analysis has been carried out for different kind of mineral P fertilisers. Changing mineral P fertiliser might increase the P leaching by a factor 8.6, when calculating with phosphoric acid plants in Morocco dispose the phosphogypsum directly to the sea (as described in section A.6.4), which has huge significance for the overall conclusions.
In this study, the uncertainty related to phosphorous eutrophication to aquatic environment is rather insignificant, as all the alternative new technologies has the same contribution as the reference system. However, in future life cycle assessments based on this report, it is strongly recommended to perform sensitivity analysis as described in Annex A.
3.4.7 Photochemical Ozone Formation (“smog”)
In the EDIP 2003 method, the photochemical ozone formation potentials (for human exposure) are expressed in the unit pers·ppm·hours. This corresponds to the accumulated exposure above the threshold of 60 ppb times the number of persons which are exposed as a consequence of the emission. No threshold critical for chronic exposure of humans to ozone has been established. Instead, the threshold of 60 ppb is chosen as the long-term environmental objective for the EU ozone strategy proposed by the World Health Organisation, WHO.
For vegetation, the impact is expressed as the accumulated exposure (duration times exceedance of threshold) above the threshold of 40 ppb times the area that is exposed as a consequence of the emission. The threshold of 40 ppb is chosen as an exposure level below which no or only small effects occur. The unit for vegetation exposure is m²·ppm·hours (Hauschild et al., 2005).
In this study, only the human exposure is included, as the results calculated by SimaPro shows very similar patterns for the two impacts (same sources, same relative distributions etc).
As can be seen in table 3.10 and 3.11, the main contributor to photochemical ozone formation is the CH4 emissions from the in-house storage of slurry and the outdoor storage of slurry. Furthermore, emissions of nitrogen oxide (from in-house and outdoor storage) also contribute to the ozone formation. The high uncertainty in the CH4 emission is discussed above under global warming. Accordingly, conclusions on the relative contributions from respectively the in-house storage and the outdoor storage should be taken with care.
As can be seen in table 3.10 and 3.11, there are some contributions to photochemical ozone formation from NOX from the slurry in the housing units, during storage and after application to field. The uncertainty on these emissions is very high, as the NO and NO2 emissions are basically rough estimates rather than measurements. Accordingly, these values should be taken with care.
The contributions from electricity consumption and transport are not significant.
The choice of marginal N, P and K fertilisers are relatively unimportant for the overall contributions to the ozone formation.
Table 3.9. Contributions to Photochemical Ozone Formation from pig slurry in the reference scenario. Per 1000 kg of slurry “ex animal”. The % should be taken with care due to the high uncertainties – it is rather rough estimates! The number of digits is not an expression of the uncertainty.
Contributions to photochemical ozone formation |
pers·ppm·
hours |
Uncertainty
Range
pers·ppm·
hours |
% of total positive contribution |
Comments and reference on uncertainty |
CH4 |
|
|
|
|
CH4 from in-house storage |
0.095 |
[ 0.008 – 0.19] |
53.3% |
Based on IPCC (2006). If IPCC data for < 1 month storage had been chosen, the emissions would be a factor 5.67 times lower. In addition, an uncertainty on a factor 2 is assumed as on the other data from IPCC (2006), see below. |
CH4 from “Storage” (in pre-tank and outdoor storage) |
0.056 |
[ 0.02 – 0.17 ] |
31.4% |
Data based on IPCC (2006). IPCC (2006) does not estimate the uncertainty. The uncertainty must be high – the emissions depend on storage time which is not included in the IPCC model. Estimated uncertainty: A factor 3. |
NOX |
|
|
|
|
NOX from slurry (in-house, storage, and field) |
0.023 |
[0.008- 0.068] |
12.7% |
Uncertainty not stated. It is estimated that the uncertainty for this is a factor 3 (the values in the study are very rough estimates). |
NOX from electricity for pumping and stirring |
0.0005 |
[0.0002-
0.001] |
0.3% |
Uncertainty not stated. It is estimated that the uncertainty for this is a factor 2. |
NOX from transport |
0.003 |
[0.001-0.005] |
1.5% |
Uncertainty not stated. It is estimated that the uncertainty for this is a factor 2. |
NOX from avoided production of fertilisers |
-0.012 |
[-0.006 – 0.024] |
-6.6% |
Uncertainty not stated. It is estimated that the uncertainty for this is a factor 2. |
Residue |
|
|
|
|
Residue from other processes |
Approx. 0 |
Not estimated |
< 1% |
|
TOTALS |
|
|
|
|
Total POSITIVE contributions |
0.179 |
[0.09-0.36] |
100% |
Rough estimate: A factor 2 |
Total AVOIDED contributions |
-0.014 |
[-0.007-0.029] |
-7.7% |
Rough estimate: A factor 2 |
Total NET contribution
(= positive - negative) |
0.165 |
[0.08-0.33] |
92.3% |
Rough estimate: A factor 2 |
Table 3.10. Contributions to Photochemical Ozone Formation from dairy cow slurry in the reference scenario. Per 1000 kg of slurry “ex animal”. The % should be taken with care due to the high uncertainties – it is rather rough estimates! The number of digits is not an expression of the uncertainty.
Contributions to photochemical ozone formation |
pers·ppm·
hours |
Uncertainty
Range
pers·ppm·
hours |
% of total positive contribution |
Comments and reference on uncertainty |
CH4 |
|
|
|
|
CH4 from in-house storage |
0.083 |
[ 0.007 – 0.17] |
51.9% |
Based on IPCC (2006). If IPCC data for < 1 month storage had been chosen, the emissions would be a factor 5.67 times lower. In addition, an uncertainty on a factor 2 is assumed as on the other data from IPCC (2006), see below. |
CH4 from “Storage” (in pre-tank and outdoor storage) |
0.049 |
[ 0.02 – 0.15 ] |
30.6% |
Data based on IPCC (2006). IPCC (2006) does not estimate the uncertainty. The uncertainty must be high – the emissions depend on storage time which is not included in the IPCC model. Estimated uncertainty: A factor 3. |
NOX |
|
|
|
|
NOX from slurry (in-house, storage, and field) |
0.024 |
[0.012- 0.048] |
15% |
Uncertainty not stated. It is estimated that the uncertainty for this is a factor 3 (the values in the study are very rough estimates). |
NOX from electricity for pumping and stirring |
0.0003 |
[0.0002-
0.0007] |
0.2% |
Uncertainty not stated. It is estimated that the uncertainty for this is a factor 2. |
NOX from transport |
0.003 |
[0.001-0.005] |
1.6% |
Uncertainty not stated. It is estimated that the uncertainty for this is a factor 2. |
NOX from avoided production of fertilisers |
-0.013 |
[-0.007 – 0.026] |
-8.2% |
Uncertainty not stated. It is estimated that the uncertainty for this is a factor 2. |
Residue |
|
|
|
|
Residue from other processes |
Approx. 0 |
Not estimated |
< 1% |
|
TOTALS |
|
|
|
|
Total POSITIVE contributions |
0.159 |
[0.08-0.32] |
100% |
Rough estimate: A factor 2 |
Total AVOIDED contributions |
-0.015 |
[-0.008-0.030] |
-9.5% |
Rough estimate: A factor 2 |
Total NET contribution
(= positive - negative) |
0.14 |
[0.07-0.29] |
90.5% |
Rough estimate: A factor 2 |
3.4.8 Respiratory inorganics (small particles)
The contributions to respiratory inorganics are totally dominated by contributions from NH3. Nitrogen oxides from transport and production of electricity contribute with less than 7%. The uncertainty on the NH3 emissions is discussed above under Acidification.
The avoided contributions from the replaced mineral fertilisers are relatively unimportant. Contributions from transport are also unimportant (3%), as well as the contribution from electricity consumption (less than 0.5%).
The unit for respiratory inorganics is PM 2.5 which means that the impacts for this category in characterised in relation to the impact on the respiratory functions on humans from particulate matter with a size of 2.5µ.
Table 3.11. Contributions to respiratory inorganics from pig slurry in the reference scenario. Per 1000 kg of slurry “ex animal”. The % should be taken with care due to the high uncertainties – it is rather rough estimates! The number of digits is not an expression of the uncertainty.
Contributions to respiratory inorganics |
kg PM 2.5 eq. |
Uncertainty
Range
kg PM 2.5 eq. |
% of total positive contribution |
Comments and reference on uncertainty |
NH3 |
|
|
|
|
NH3 from in-house storage |
0.157 |
[ 0.08 – 0.31] |
54.3% |
Uncertainty not stated. It is estimated that the uncertainty for this is a factor 2. |
NH3 from “Storage” (in pre-tank and outdoor storage) |
0.016 |
[ 0.008 – 0.032 ] |
5.6% |
Uncertainty not stated. It is estimated that the uncertainty for this is a factor 2. |
NH3 from field |
0.08 |
[ 0.04 – 0.16] |
27.8% |
Uncertainty not stated. It is estimated that the uncertainty for this is a factor 2. |
Avoided NH3 from avoided mineral fertilisers |
-0.015 |
[ -0.008 –
-0.03] |
-5.3% |
Uncertainty not stated. It is estimated that the uncertainty for this is a factor 2. |
NOX |
|
|
|
|
NOX from slurry (in-house, storage, and field) |
0.024 |
[ 0.012 – 0.048] |
8.4% |
Uncertainty not stated. It is estimated that the uncertainty for this is a factor 2. |
NOX from electricity for pumping and stirring |
0.0005 |
[0.0003-0.001] |
0.2% |
Uncertainty not stated. It is estimated that the uncertainty for this is a factor 2. |
NOX from transport |
0.0029 |
[0.0014-0.0057] |
1.0% |
Uncertainty not stated. It is estimated that the uncertainty for this is a factor 2. |
NOX from avoided production of fertilisers |
-0.013 |
[-0.0063-
- 0.025] |
-4.4% |
Uncertainty not stated. It is estimated that the uncertainty for this is a factor 2. |
Particulates |
|
|
|
|
Particulates from transport |
0.0038 |
[0.0019 – 0.0076] |
1.3% |
Uncertainty not stated. It is estimated that the uncertainty for this is a factor 2. |
Particulates from electricity production |
0.00023 |
[0.0001 –
0.0005] |
0.1% |
Uncertainty not stated. It is estimated that the uncertainty for this is a factor 2. |
Avoided particulates due to avoided production of mineral fertilisers |
-0.015 |
[ -0.007-
-0.029] |
-5.1% |
Uncertainty not stated. It is estimated that the uncertainty for this is a factor 2. |
Residue |
|
|
|
|
Residue from other processes |
-0.004 |
Not estimated |
<2% |
|
TOTALS |
|
|
|
|
Total POSITIVE contributions |
0.288 |
[0.14-0.58] |
100% |
Rough estimate: A factor 2 |
Total AVOIDED contributions |
-0.050 |
[-0.02—0.10] |
-17.3% |
Rough estimate: A factor 2 |
Total NET contribution
(= positive - negative) |
0.238 |
[0.12-0.48] |
82.7% |
Rough estimate: A factor 2 |
Table 3.12. Contributions to respiratory inorganics from dairy cow slurry in the reference scenario. Per 1000 kg of slurry “ex animal”. The % should be taken with care due to the high uncertainties – it is rather rough estimates! The number of digits is not an expression of the uncertainty.
Contributions to respiratory inorganics |
kg PM 2.5 eq. |
Uncertainty
Range
kg PM 2.5 eq. |
% of total positive contribution |
Comments and reference on uncertainty |
NH3 |
|
|
|
|
NH3 from in-house storage |
0.081 |
[ 0.04 – 0.16] |
32.3% |
Uncertainty not stated. It is estimated that the uncertainty for this is a factor 2. |
NH3 from “Storage” (in pre-tank and outdoor storage) |
0.019 |
[ 0.01 – 0.04 ] |
7.6% |
Uncertainty not stated. It is estimated that the uncertainty for this is a factor 2. |
NH3 from field |
0.12 |
[ 0.06 – 0.23] |
45.9% |
Uncertainty not stated. It is estimated that the uncertainty for this is a factor 2. |
Avoided NH3 from avoided mineral fertilisers |
-0.016 |
[ -0.008 –
-0.03] |
-6.5% |
Uncertainty not stated. It is estimated that the uncertainty for this is a factor 2. |
NOX |
|
|
|
|
NOX from slurry (in-house, storage, and field) |
0.025 |
[ 0.013 – 0.051] |
10% |
Uncertainty not stated. It is estimated that the uncertainty for this is a factor 2. |
NOX from electricity for pumping and stirring |
0.0004 |
[0.0002-0.0007 |
0.1% |
Uncertainty not stated. It is estimated that the uncertainty for this is a factor 2. |
NOX from transport |
0.0028 |
[0.0014-0.0055] |
1.1% |
Uncertainty not stated. It is estimated that the uncertainty for this is a factor 2. |
NOX from avoided production of fertilisers |
-0.014 |
[-0.0069-
- 0.028] |
-5.5% |
Uncertainty not stated. It is estimated that the uncertainty for this is a factor 2. |
Particulates |
|
|
|
|
Particulates from transport |
0.0037 |
[0.0018 – 0.0073] |
1.5% |
Uncertainty not stated. It is estimated that the uncertainty for this is a factor 2. |
Particulates from electricity production |
0.00016 |
[0.0001 –
0.0003] |
0.1% |
Uncertainty not stated. It is estimated that the uncertainty for this is a factor 2. |
Avoided particulates due to avoided production of mineral fertilisers |
-0.016 |
[ -0.008-
-0.032] |
-6.4% |
Uncertainty not stated. It is estimated that the uncertainty for this is a factor 2. |
Residue |
|
|
|
|
Residue from other processes |
-0.004 |
Not estimated |
<2% |
|
TOTALS |
|
|
|
|
Total POSITIVE contributions |
0.252 |
[0.13-0.50] |
100% |
Rough estimate: A factor 2 |
Total AVOIDED contributions |
-0.054 |
[-0.03—0.11] |
-21.5% |
Rough estimate: A factor 2 |
Total NET contribution
(= positive - negative) |
0.198 |
[0.10-0.40] |
78.5% |
Rough estimate: A factor 2 |
3.4.9 Non-renewable energy resources
The non-renewable energy resources are calculated by use of the LCA method Impact 2002+(Humbert et al. 2005). The unit is “MJ Primary Energy”, using the upper heating value.
The choice of replaced fertilisers has significance for the overall consumption of non-renewable resources. Changing the replaced fertilisers can reduce the net consumption of non-renewable resources by 30% or increase the net consumption by 50%.
Table 3.13. Consumption of non-renewable energy resources from management of pig slurry in the reference scenario. Per 1000 kg of slurry “ex animal”. The % should be taken with care due to the high uncertainties – it is rather rough estimates! The number of digits is not an expression of the uncertainty.
Consumption of non-renewable energy resources |
MJ primary energy |
Uncertainty
Range
MJ primary energy |
% of total positive contribution |
Comments and reference on uncertainty |
MJ Primary Energy |
|
|
|
|
Electricity for pumping and stirring |
56 |
[ 28-112] |
37% |
Uncertainty not stated. It is estimated that the uncertainty for this is a factor 2. |
Transport |
56 |
[ 28-112 ] |
37% |
Uncertainty not stated. It is estimated that the uncertainty for this is a factor 2. |
Field processes (tractor for application of slurry) |
40 |
[ 20-80] |
26% |
Uncertainty not stated. It is estimated that the uncertainty for this is a factor 2. |
Avoided production of mineral fertilisers |
-369 |
[ -184-737] |
-244% |
Uncertainty not stated. It is estimated that the uncertainty for this is a factor 2. |
Residue |
|
|
|
|
Residue from other processes |
0 |
Not estimated |
0% |
|
TOTALS |
|
|
|
|
Total POSITIVE contributions |
151 |
[75-300] |
100% |
Rough estimate: A factor 2 |
Total AVOIDED contributions |
-369 |
[-180-
-740] |
-244% |
Rough estimate: A factor 2 |
Total NET contribution
(= positive - negative) |
-217 |
[-110-440] |
-144% |
Rough estimate: A factor 2 |
Table 3.14. Consumption of non-renewable energy resources from management of dairy cow slurry in the reference scenario. Per 1000 kg of slurry “ex animal”. The % should be taken with care due to the high uncertainties – it is rather rough estimates! The number of digits is not an expression of the uncertainty.
Consumption of non-renewable energy resources |
MJ primary energy |
Uncertainty
Range
MJ primary energy |
% of total positive contribution |
Comments and reference on uncertainty |
MJ Primary Energy |
|
|
|
|
Electricity for pumping and stirring |
40 |
[ 20-80] |
30% |
Uncertainty not stated. It is estimated that the uncertainty for this is a factor 2. |
Transport |
54 |
[ 27-107 ] |
41% |
Uncertainty not stated. It is estimated that the uncertainty for this is a factor 2. |
Field processes (tractor for application of slurry) |
38 |
[ 19-76] |
29% |
Uncertainty not stated. It is estimated that the uncertainty for this is a factor 2. |
Avoided production of mineral fertilisers |
-410 |
[ -205-820] |
-312% |
Uncertainty not stated. It is estimated that the uncertainty for this is a factor 2. |
Residue |
|
|
|
|
Residue from other processes |
0 |
Not estimated |
0% |
|
TOTALS |
|
|
|
|
Total POSITIVE contributions |
131 |
[66-260] |
100% |
Rough estimate: A factor 2 |
Total AVOIDED contributions |
-410 |
[-205-
-820] |
-312% |
Rough estimate: A factor 2 |
Total NET contribution
(= positive - negative) |
-279 |
[-140--560] |
-212% |
Rough estimate: A factor 2 |
3.5 Conclusion
It should be emphasised that essential assumptions and data in this report are chosen to represent Danish conditions only. Results cannot be immediately transferred to other countries due to differences in housing systems, retention time for the slurry in the housing units and in the outdoor storage, differences in how the slurry is stored (covered / uncovered), differences in temperatures, slurry composition (due to differences in the feeding of the animals), temperature and other weather conditions (during and after application), soil types and many other factors.
The conclusions are only valid for the preconditions described in this report! For example, differences in application method to the field, uncovered outdoor storage or differences in the slurry composition will significantly affect the results.
Apparently, the Danish scientific research in the “slurry management area” so far has to a high degree focussed on NH3 emissions, whereas emissions related to global warming has a higher uncertainty and needs more research. However, it can be concluded that CH4 emissions from the indoor storage of slurry, the CH4 emissions from the outdoor storage and the N2O emissions from the field has great significance for the overall contributions to global warming, and effort to reduce these are important.
The relative contributions to the global warming in table 3.3 and 3.4 should be interpreted with care due to high uncertainties on the data. Contributions to global warming mainly come from CH4 from in-housing storage, outdoor storage and from CO2 and N2O emissions after application of the slurry to the field. Scientific research is needed in the area.
The contribution to acidification is totally dominated by NH3 emissions in the housing units, during outdoor storage and after application of the slurry to the field.
Aquatic eutrophication (N) is dominated by N leaching. NH3 emissions also contribute to some extent (contributions from the indoor storage are due to NH3 emissions).
The uncertainty on aquatic phosphorous eutrophication (P) is high due to lack of data and it cannot be concluded that there is a “net saving on P leaching” by applying pig slurry or dairy cow slurry! The discussion of P leaching from slurry vs. mineral fertilisers is beyond the scope of this study.
The main contributor to ozone formation is the CH4 emissions from the in-house storage of slurry and the outdoor storage of slurry.
The contributions to respiratory inorganics are totally dominated by contributions from NH3.
The electricity consumption (for pumps and stirring) is rather insignificant (but for resource consumption).
Transport has a small contribution to the category “Resource consumption” due to the fuel consumption. The contributions to “Ozone formation” are rather small, and so are the contributions to the category “Respiratory inorganics” (caused by small particles emitted during driving). Transport is totally insignificant for the rest of the impact categories.
The difference between soil type JB3 and JB6 is only noteworthy for aquatic eutrophication (N) (nitrate leaching).
[4] I.e the 42% pigs in the category “Weaned pigs less than 50 kg” plus the 28% pigs in the category “Weaned pigs at 50 kg and above”.
[5] JB3 has a clay content of 5 – 10 %, a silt content < 25 % and a fine sand content <40 %. JB6 has a clay content of 10 – 15 %, a silt content < 30 % and a fine sand content of 40 – 90 %
[6] In Danish: Sengestald med spaltegulv (1.2 m kanal)
[7] In Danish: Tørstof (TS).
[8] In Danish: Askefrit tørstof eller glødetab.
| Front page | | Contents | | Previous | | Next | | Top |
Version 1.0 July 2009, © Danish Environmental Protection Agency
|