| Bottom | | Front page |
Environmental project no. 1003, 2005
The indoor and outdoor concentrations of particulate air-pollution and PAHs in different size fractions and assessment of exposure and health impacts in the Copenhagen population
Contents
Preface
Summary and conclusions
Sammenfatning og Konklusion
1 Introduction
2 Experimental methods
3 PM in indoor and outdoor air
4 PAHs in indoor and outdoor air
5 Conclusions
6 Reference list
Appendix A
Appendix B
Appendix C
Appendix D
Appendix E
Appendix F
Preface
The overall goal of this study was to update our present knowledge of the indoor and outdoor air-pollution in central Copenhagen (Denmark), to investigate the importance of the infiltration of traffic
generated air pollution to the indoor environment in a case-study apartment, as well as to assess the potential adverse health effects in the Copenhagen population. The measures in the study was fine (PM1
and PM2.5) and coarse (inhalable dust) particulate air-pollution and sixteen volatile and semivolatile polycyclic aromatic hydrocarbons associated with the particles.
The project was financed by the Danish Environmental Protection Agency (Danish EPA) and established as an extension of Work-Package C3.1 under the Centre for Traffic Research on environmental and
health Impacts and Policy, TRIP (http://www.akf.dk/trip/index.htm).
The project was followed by an observation group consisting of:
- Poul Bo Larsen, Danish Environmental Protection Agency (chairman)
- Christian Lange Fog, Danish Environmental Protection Agency
- Ole Hertel, National Environmental Research Institute
- Marianne Glasius, National Environmental Research Institute
- Steffen Loft, University of Copenhagen
FieLDwork was conducted between January and July of 2002 in close collaboration with the TRIP WP3.1C Working Group from the National Environmental Research Institute (Roskilde), The Danish
Building and Urban Research Institute (Helsingør), The Danish Environmental Protection Agency, University of Copenhagen and The National Institute of Occupational Health (Copenhagen).
The current project was conducted and reported by a working group at the National Institute of Occupational Health, Denmark, which consisted of Vivi Kofoed-Sørensen, Per Axel Clausen and Keld
Alstrup Jensen. Dorte Narv and Tina Trankjær Olsen additionally supported with technical assistance during the fieLD campaigns.
During the course of this study we have had fruitful discussions with the observation group who also reviewed the current report. Additionally, we are grateful for beneficial discussions on the HPLC method
with Åse-Marie Hansen and Dorrit Meincke.
Summary and conclusions
Fifteen one-week samples of PM1, PM2.5, inhalable dust (PMinh) and 16 polycyclic aromatic hydrocarbons (PAHs) were collected inside and outside of an uninhabited 4th floor apartment at the Jagtvej
street canyon in central Copenhagen during winter, spring and summer in 2002. Similar urban background samples were collected at a 2 km distant 4th floor high rooftop. PAHs in PM1 and PMinh were
collected on glass fibre filters only. PAHs in PM2.5 were collected on glass fibre filters followed by adsorbent sample and backup tubes containing Tenax. PM was determined by filter weighing. The PAHs
were analyzed by liquid extraction of filters and adsorbent tubes followed by high performance liquid chromatography with UV and fluorescence detection.
The Copenhagen particulate air-pollution was dominated by fine particles. App. 70 wt% of the PM2.5 consisted of PM1 at all sites. The average PM2.5 content in PMinh was 54 and 69 wt% at Jagtvej and
in the urban background, respectively. Indoors PMinh consisted almost entirely of PM2.5. Correlation analysis showed a strong relationship between PM1, PM2.5 and PMinh at Jagtvej and in the urban
background. However, PM at Jagtvej exceeded the urban background concentrations. The difference suggests that traffic on average contributed with 3.5±1.9 g/m3, 5.0±2.7 g/m3 and 14.6±4.0 g/m3 to PM1,
PM2.5 and PMinh, respectively.
Indoor PM correlated well with PM in both the street and the urban background. However, indoor-outdoor ratios below unity (0.77±0.21 for PM1 and 0.77±0.24 for PM2.5) were only achieved using
PM-concentrations measured in the street at Jagtvej. The average indoor PM2.5 concentration (15.20 μg/m3) exceeded the annual indoor PM2.5 concentration of 15 μg/m3, which is recommended in the US
based on the US-EPA air-quality guideline for PM2.5. At the best, the outdoor PM2.5 concentrations (19.80 μg/m3 at Jagtvej and 14.85 μg/m3 in the urban background) just complied with the target values
proposed by the EU CAFE Working Group to be within 12 to 20 μg/m3. Assessment of adverse health effects induced by PM2.5 at 95% CI suggested 780520 excess accumulated deaths per million in
Copenhagen in 2002. Additionally, 1556701 excess hospitalisations were predicted per million inhabitants for respiratory symptoms and all cardiovascular disease, combined.
In PM2.5 samples the total concentrations of the 16 US-EPA gas and particle phase priority PAHs (ΣPAH) were 15-284 ng/m3 indoors, 46-235 ng/m3 outdoors, and 2-105 ng/m3 in the urban background.
The concentrations were probably underestimated due to extraction recovery below 100%, breakthrough, and reaction with ozone and nitrogen oxides during sampling. The real concentrations may be up to
two times higher than observed. Urban background, traffic and indoor sources contributed to the overall concentration of PAHs in the uninhabited apartment. Traffic in the Jagtvej street canyon and indoor
sources appeared to be the most important sources for PAHs indoors. The WHO unit risk value of 8.7 10-5 per ng/m3 B(a)P for life-time cancer risk suggests that ~10 cancer cases per 106 inhabitants
may occur at a life-time exposure to the PAH-concentrations observed in the apartment. For comparison, 8 and 5 cases per 106 inhabitants are expected from the street and urban background
concentrations, respectively. B(a)P may be an inadequate marker for assessment of cancer induced by urban air-pollution. Differences between indoor and outdoor PAH profiles and presence of other
carcinogenic compounds may result in serious estimation errors.
Sammenfatning og Konklusion
Sundhedsskadelige partikler og tjærestoffer fra biltrafikken trænger ind i selv husenes øverste etager langs trafikerede gader i København. Forureningen med fine partikler er mindre end grænseværdien
foreslået af EU, men kan alligevel betyde ca. 780 for tidlige dødsfald om året i Storkøbenhavn. Koncentrationen af de målte kræftfremkaldende tjærestoffer er lav og ser ud til kun at have ringe betydning for
udvikling af lungekræft. Dette er nogle af resultaterne fra en undersøgelse af partikler og tjærestoffer i luften indenfor og udenfor en ubeboet 3. sals lejlighed på Jagtvej i København. I undersøgelsen blev der
fokuseret på indtrængning af udendørsforureningen ind i lejligheden og vurdering af helbredseffekter.
Baggrund og formål
Videnskabelige undersøgelser har vist, at jo højere luftforureningen med små partikler er i byerne, desto flere dødsfald og hospitalsindlæggelser er der pga. især hjertekarsygdomme, luftvejsproblemer og
kræft. Imidlertid ved man, at det ikke partiklerne alene der er årsag til de flere dødsfald. Nogle af indholdsstofferne er skadelige i sig selv. Blandt de mest omtalte indholdsstoffer er de kræftfremkaldende
tjærestoffer. Disse stoffer dannes ved forbrænding af organisk materiale. Forbrænding af fossile brændstoffer som kul, olie, diesel og benzin er formodentlig den vigtigste kilde til tjærestoffer i luften i
Danmark. Indendørs er rygning og madlavning vigtige kilder til luftforureningen med tjærestoffer.
Partiklernes evne til at trænge ind i bygninger og deres biologiske effekter varierer med partiklernes størrelse. Små partikler med en diameter på under én mikrometer kan let trænge ind i en bygning og kan
inhaleres langt ned i menneskers lunger. Tjærestofferne findes hovedsageligt i disse små partikler. Imidlertid kan partikler med tiden enten optage eller afgive gasser til omgivelserne og klumpe sammen,
hvorved deres masse og størrelse ændres. Derfor er det uklart hvor meget af udendørs partikelforureningen der faktisk trænger ind i bygninger. Formålet med projektet var at måle koncentrationen af
partikler og tjærestoffer i luften udenfor og indenfor i en lejlighed, for at kunne vurdere den faktiske indtrængning af disse trafikforureninger i bygninger.
Undersøgelsen
Undersøgelsen, der blev foretaget af Arbejdsmiljøinstituttet, blev baseret på luftprøver opsamlet indenfor og udenfor en ubeboet tredje-sals-lejlighed på en trafikeret gade (Jagtvej) i København i løbet vinter,
forår og sommer 2002. Der blev opsamlet i alt 15 én-uges luftprøver af tre forskellige partikelstørrelser i de tre kampagner af hver fem ugers varighed. Prøverne skulle bruges til bestemmelse af
luftforureningen med partikler og 16 forskellige tjærestoffer (Polycyclic Aromatic Hydrocarbons: PAHer). Tilsvarende prøver af bybaggrundsluften blev indsamlet i tredje sals højde på
Arbejdsmiljøinstituttets tag, ca. 2 km fra lejligheden. Meget fine partikler (PM1; partikler mindre end 1 mikrometer), fine (PM2.5; partikler mindre end 2,5 mikrometer) og inhalerbare partikler (PMinh;
partikler op til ca. 100 mikrometer) blev opsamlet på filtre. Gasformige PAHer blev desuden opsamlet på et specielt gasfilter monteret efter partikelfiltret til indsamling af PM2.5. Koncentrationen af de
opsamlede partikler blev målt ved vejning af filtrene. PAH'erne blev målt ved at udtrække dem fra partikel- og gas-filtre med et opløsningsmiddel og efterfølgende analysere udtrækket med
specialinstrumenter.
Hovedkonklusioner
Biltrafikken forurener luften med partikler og tjærestoffer på Jagtvej i København. Denne forurening trænger ind i den undersøgte tredje-sals-lejlighed på Jagtvej. I lejligheden var der også andre kilder til
luftforurening med partikler og tjærestoffer. Denne forurening kunne være indtrængning af udendørsforurening igennem nabolejligheder eller forurening fra nabo-lejlighederne, hvor der f.eks. blev lavet mad
eller røget. Den gennemsnitlige koncentration af fine partikler (PM2.5) på Jagtvej (19.80 mikrogram per m3) og i bybaggrunden (14.85 mikrogram per m3) lå i grænseområdet sammenlignet med de årlige
middelkoncentrationer på 12 til 20 mikrogram per kubikmeter, som diskuteres i den såkaldte CAFE arbejdsgruppe i EU. Baseret på PM2.5 ser den fine partikelforurening ud til at kunne betyde ca. 780
(95CI: 260 – 1300) for tidlige dødsfald om året i Storkøbenhavn. Alle målinger af tjærestoffet benzo(a)pyren var under den nyligt vedtagne EU-målværdi på 1 nanogram benzo(a)pyren per kubikmeter. De
målte koncentrationer af tjærestoffer ser da heller ikke ud til at kunne betyde mere end ti kræftdødsfald i hele Storkøbenhavn over en 70-årig periode. Benzo(a)pyren er måske ikke tilstrækkelig til at vurdere
kræftrisikoen ved den atmosfæriske luftforurening. Det kan skyldes at sammensætningen af tjærestoffer i de forskellige miljøer varierer for meget. Desuden kan partikler indeholde andre kræftfremkaldende
stoffer og evt. i sig selv forstærke den kræftfremkaldende effekt. Det tager den velaccepterede benzo(a)pyren model ikke højde for.
Projektresultater
Den københavnske luftforurening viste sig at være domineret af fine partikler. Cirka 70% af PM2.5 bestod af PM1. Det gennemsnitlige indhold af PM2.5 i PMinh var henholdsvis 54% og 69% udendørs på
Jagtvej og i bybaggrunden. PMinh i den ubeboede lejlighed bestod næsten udelukkende af PM2.5. Der var en stærk sammenhæng mellem PM1, PM2.5 og PMinh på Jagtvej og i bybaggrunden.
Partikelkoncentrationen på Jagtvej var dog højere end i bybaggrunden og forskellen tyder på at trafikken i gennemsnit bidrog med 3.5±1.9, 5.0±2.7 og 14.6±4.0 mikrogram per kubikmeter til henholdsvis PM1,
PM2.5 and PMinh.
Forholdet mellem partikler indendørs og udendørs
Indendørs partikelforureningen varierede klart med udendørs-koncentrationerne både på Jagtvej og i bybaggrundsluften. Imidlertid var de gennemsnitlige indendørs/udendørs-forhold for PM1 (0.77±0.21) og
PM2.5 (0.77±0.24) kun mindre end én, når indendørskoncentrationerne blev sammenlignet med partikel-koncentrationerne på Jagtvej. Inde-ude forholdet var derimod tæt på én for både PM1 (1.120.34) og
PM2.5 (1.050.35), når man sammenlignede med ders koncentrationen i bybaggrundsluften. Da mennesker mest opholder sig indendørs kan den sammenhæng måske forklare, hvorfor data for forureningen i
bybaggrunden kan anvendes i epidemiologiske studier af helbredseffekter forårsaget af luftforurening.
Indendørs og udendørs grænseværdier for partikler
I relation til grænseværdier og vejledninger for partikulære luftforureninger, så overskred den gennemsnitlige indendørskoncentration af PM2.5 (15.2±5.0 mikrogram per kubikmeter) amerikanske anbefalinger
for en årlig middelværdi for PM2.5 på 15.0 mikrogram per kubikmeter i indeklimaet. Det skal her bemærkes, at vi må antage at gennemsnittet af de 15 én-ugers målinger kan ekstrapoleres til årsgennemsnit.
Den gennemsnitlige udendørskoncentration i bybaggrunden (14.9±6.0 mikrogram per kubikmeter) og på Jagtvej (19.8±7.7 mikrogram per kubikmeter) var under den maksimale årlig gennemsnits målværdi på
20 mikrogram per kubikmeter, som er foreslået af CAFE arbejdsgruppen i EU. Koncentrationerne oversteg dog alle steder klart CAFE-gruppens laveste foreslåede årlige gennemsnits målværdi på 12
mikrogram per kubikmeter.
Vurdering af de fine partiklers helbredseffekter
Selvom luftforeningen i gennemsnit ligger på den rigtige side af den foreslåede grænseværdi, så viser en vurdering af helbredseffekterne som funktion af PM2.5, at luftforureningen stadig medfører adskillelige
tidlige dødsfald og indlæggelser. Beregninger vha. dosis-respons forhold fra Verdenssundhedsorganisationen (WHO) og førende amerikanske forskere viser, at der for hver million indbyggere årligt kan være
780 (95CI: 250 – 1300) for tidlige dødsfald i alt og ca. 1560 (95CI: 860 – 2260) ekstra indlæggelser på grund af hjertekarsygdomme og luftvejsproblemer baseret på koncentrationerne i bybaggrunden. De
fleste af indlæggelserne (1006; 95CI: 305-1707) er forventet at skyldes hjertekarsygdomme.
Koncentrationen af PAHer i luften
Den totale koncentration af de 16 undersøgte PAH'er (ΣPAH) i PM2.5-prøverne var 15-284 nanogram per kubikmeter indendørs, 46-235 nanogram per kubikmeter udendørs på Jagtvej, og 2-105
nanogram per kubikmeter i bybaggrunden. Koncentrationerne skal sandsynligvis betragtes som minimumsværdier, da man sjældent kan udtrække PAHerne 100% fra prøverne. Desuden kan der ske
nedbrydning af PAHerne ved reaktion med nitrogenoxider og ozon under prøvetagningen. De virkelige koncentrationer kan være op til 2 gange højere.
Hvor kommer PAHerne i indeklimaet fra?
Både bybaggrunden, trafik på Jagtvej og indendørs kilder bidrog til PAH forureningen i den ubeboede lejlighed. De to sidstnævnte kilder var de vigtigste. Specielt var der en klar lineær sammenhæng mellem
indendørskoncentrationen af tre partikelbundne PAHer (chrysene+benzo(b)fluoranthene+benzo(k)flouranthene), som er sporstoffer fra diesel, og udendørskoncentrationen på Jagtvej. Denne sammenhæng
var der ikke, når deres koncentration i bybaggrundsluften blev brugt og viser at trafikken på Jagtvej spiller en væsentlig rolle for PAH og partikelforureningen inde i den undersøgte lejlighed.
Indendørskilderne viste størst udslag for de gasformige PAHer, som evt. stammer fra rygning og madlavning i andre lejligheder i bygningen.
Kræftrisikoen fra PAH
Kræftrisikoen fra PAHer bliver normalt vurderet udfra koncentrationen af benzo(a)pyren. WHO's værdi på 8.7 10-5 per nanogram per kubikmeter benzo(a)pyren for risiko for udvikling af kræft antyder, at
der kan opstå ~10 kræfttilfælde for hver million indbyggere ved livstidseksponering for PAH-koncentrationerne målt i lejligheden. Tilsvarende kan ca. 8 og 5 kræfttilfælde forventes for hver million
indbyggere fra gade og bybaggrunds-koncentrationerne. Benzo(a)pyren er måske uegnet til at vurdere den samlede kræftrisiko ved udsættelse for den atmosfæriske luftforurening. Det kan skyldes
udsættelse for andre kræftfremkaldende stoffer og medhjælpende irritation fra partikler i lungen.
1 Introduction
1.1 Background
Epidemiological studies have shown a relationship between increased mass concentrations of urban air-pollution and increased hospitalisation for respiratory symptoms and mortality rates (e.g.,Künzli et al.
2000; Schwartz et al. 2001; Schwartz et al. 2002; Pope, III et al. 2002; Nafstad et al. 2004)). These adverse health effects are better correlated to fine particles (PM1 and PM2.5) [1] than coarser particle
measures (e.g., PM10).
However, adverse health effects from air-pollution are not related to particle mass-concentrations alone. Long-term exposure to specific Hazardeous Air-Pollution Substances - often referred to as HAPS or
air toxics - can also result in negative health effects of which cancer appears to be the most important outcome. In the USA, 188 HAPS' have been identified under the Clean Air Act Amendments of 1990
(http://www.epa.gov/ttn/atw/188polls.html). Among these air toxics, benzene and PAH's (Polycyclic Aromatic Hydrocarbon) are some of the most important carcinogenic compounds.
Combustion of fossil fuels (coal, oil, diesel and gasoline) is generally the most important source of PAHs in ambient air. Increased use of wood-burning stoves for domestic heating in Denmark has
additionally re-introduced wood burning as an important PAH source in local environments . PAHs also exist as constituents in emissions from typical indoor sources such as cooking, smoking and indoor
emissions from wood-burning stoves. Hence, human exposure to particulate matter and PAHs in indoor environments is a mixture of the contribution from outdoor air and that emitted from indoor sources.
The transport properties and biological effects from exposure to particulate air-pollution strongly depend on particle size distribution. Submicrometer particles can easily penetrate into the indoor environment,
especially if air-filtration does not occur.
PAH's mainly occur in the fine particle fraction (Schnelle et al., 1995). A recent study has shown that the size distribution of PAH's at the 20 m roof-top in Saitama (Japan) was unimodal with a peak
concentration at 480-680 nm . On the other hand, the maximum in relative PAH-contents was found in the particulate matter smaller than 200 nm. This is consistent with observations in exhaust from
combustion of fossil fuels, where it has been found that PAHs form individual particles or adsorb onto especially the ultra-fine combustion particles during cooling of the flue gas or exhaust. Hence, owing to
the size of combustion particles and production of volatile PAHs from e.g. traffic and other outdoor sources, PAHs may be an important constituent of the particulate air pollution indoors. Several studies
have already been completed to quantify the degree of particle infiltration. However, owing to particle phase transformations and potential influence of indoor sources, it is still unclear to what extent urban
air-pollution de facto penetrates into the indoor environment. Indoor-outdoor ratios of specific urban air-pollution compounds, such as PAHs, may yieLD better estimates.
In addition to the infiltration efficiency into Buildings, particle size also determines the deposition efficiency of particles in the respiratory system. In humans, coarse particles deposit in the upper respiratory
system, whereas the fine (PM1 and PM2.5) and ultrafine particles ( ≤ 100 nm) predominantly are retained in the deeper bronchioalveolar region of the lung. Some ultrafine particles also deposit in the nose and
throat owing to their high diffusion rates. Consequently, since indoor particulate air-pollution and vehicle exhaust including particle-bound PAHs mainly occur in the submicrometer range, they are predicted
to deposit mainly in the gas-exchange region in the deeper respiratory tract with lesser amounts in the nose and throat. Therefore, bias may occur in causal studies using coarser mass-dose estimates
1.2 Purpose of the study
The purpose of this study was to:
- Determine the concentrations and indoor-outdoor relationship for PM1, PM2.5 and inhalable dust indoors and outdoors of an uninhabited apartment at Jagtvej in the centre of Great Copenhagen
combined with roof-top measurements of urban background concentrations at the National Institute of Occupational Health (Copenhagen, Denmark).
- Determine the concentrations and indoor-outdoor relationships for specific volatile and semivolatile PAH's in PM2.5 at the three sample sites with focus on the influence from traffic air-pollution on indoor
air.
- Evaluate the potential adverse health effects induced by the particulate air-pollution and the carcinogenic risk induced by the observed PAH-concentrations in the indoor and outdoor environments in
Copenhagen.
The project was funded by the Danish Environmental Protection Agency and conducted in synergy with the TRIP-project (Centre for Traffic Research on environmental and health Impacts and Policy)
funded by the Strategic Environmental Research Program (http://www.akf.dk/trip/index.htm) and a parallel study of the characterization, inflammatory potential and genotoxic effect of the particulate
air-pollution in Copenhagen funded by the Danish Ministry of the Interior and Health, Research Centre for Environmental Health (J.nr. 383-29-2001).
Footnotes
[1] PM is short for Particulate Matter. PM1 is the mass concentration of particles up to 1 m-size.
2 Experimental methods
2.1 Sampling conditions
One-week samples of PM1, PM2.5, PMinh (inhalable dust) and PAH's were collected from three locations at two fieLD sites (Fig. 2.1): Indoors and immediately outside the living room window of a 4th
floor uninhabited apartment at Jagtvej, 2200 Nørrebro, Copenhagen, Denmark and at the 4th floor-high roof-top of the National Institute of Occupational Health (NIOH), Lersø Parkallé, DK-2100
Copenhagen, Denmark.
Click here to see the Figure
Figure 2.1: Map showing the location of the fieLD sites in the study and placement of city train tracks and crematories, which are potential local particle and/or PAH near the fieLD sites.
All samples were collected during three fieLD campaigns in 2002 covering winter (Jan 9 – Febr 13), spring (March 27 – May 8) and early summer (May 29 – June 26). The first two fieLD campaigns were
conducted contemporaneously with the last two multi-instrumental fieLD campaigns in the national research project called TRIP (http://www.akf.dk/trip/index.htm). It was not possible to obtain samples during
fall as the sublet contract of the apartment ended June 2002.
2.2 Description of fieLD sites
2.2.1 The Jagtvej apartment (Jagtvej_IN)
Indoor particulate and PAH air-pollution were sampled in a furnished uninhabited 35.3 net m2 apartment. The apartment was located at the fourth floor (3. sal, tv.) in a residential Building at Jagtvej 83, in the
central part of Copenhagen, Denmark (Fig. 2.1).
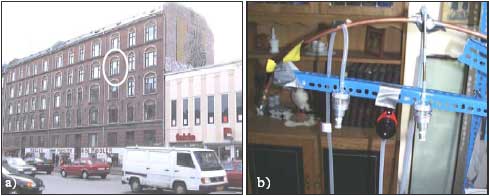
Figure 2.1: a) Picture of the apartment façade at Jagtvej. The encircled living-room window indicates the apartment used for the study. b) Image of the PM samplers mounted 1.5 m above the floor in the
living-room, ~1.5 m from the living-room window. The CIS sampler is seen in the middle between the two Triplex cyclones for collection of PM1 and PM2.5. Tenax tubes are mounted in line after the
PM2.5 sampler on the right hand side.
The Building was erected around 1900 and has five stories with dwellings. The basement contains shops and storage rooms. The outer walls were masonry and ~32 cm thick story-partitions were made of
wood with a layer of clay in between floor planks and underlying basal floor planks. In 1984 the Building was renovated with mechanical exhaust-only ventilation connected to kitchens and bathrooms. The
centralized heating system connected to municipality district heating is from the same period. Heating was maintained using radiators with thermostats placed under the windows. New double glazed windows
had been installed in well-sealed new frames around 1995.
During the fieLD campaigns, the apartment and mechanical ventilation was modified by inserting a false door, sealing of the kitchen area, where all pumps and computer equipment were stored (Fig. 2.2).
Tubes and wires were led through drilled and sealed holes in the false door structure. Taping door-cracks, keyholes and the mail slit in the entrance hall with gaffa tape minimized ventilation through the
entrance door to the hallway.
Owing to the construction type with wooden floors and the recent replacement of pipes for the central heating system, story-partitions could not be expected to be airtight. The new windows, on the hand
other were very airtight. Therefore ventilation slits were inserted in the street-ward window to allow higher fresh-air ventilation rates to levels closer to of at least 0.5 h1 satisfying the Danish Building
regulations. Despite this modification, results from the TRIP-project suggest that a significant amount of replacement air may enter the studied apartment through neighboring dwellings. Less than 20% of the
replacement air entered directly from the street through the designated ventilation slits). Therefore, there is a high risk of contamination of especially volatile compounds from neighbour activities.
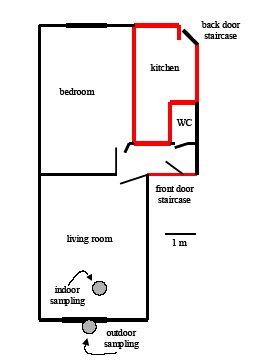
Figure 2.2: Sketch of the Jagtvej apartment with location of the indoor and outdoor sampling locations. The kitchen was sealed of by inserting an air-tight false door between the hallway and the kitchen.
Air-exchange through the front door was sought eliminated by taping of the mail-slit, key-hole and the edges around the door.
2.2.2 The Jagtvej street canyon (Jagtvej_EXT)
Outdoor particulate and PAH air-pollution were sampled in the Jagtvej street canyon at 4th floor height immediately outside the living room window of the uninhabited apartment (Fig. 2.2). Jagtvej is a
NW-SE oriented two-way street with a traffic load on the order of 26,000 vehicles per working day of which approximately 6 % of the traffic is heavy traffic. In the monitoring area, the street canyon is
partially open owing to the presence of a small street perpendicular to Jagtvej and a lower Building opposite of the apartment complex.
The samplers were mounted outside the living room window, placed next to each other appr. 10-15 cm apart shielded from direct sunlight at rain by a white hard-plastic bucket. Great care was taken to
ensure that the sample inlet was well free of the shelter.
There was no apparent risk of sample contamination from proximate outdoor particles or PAH point sources at the 4th floor sampling height. However, at ground level, a bus stop was located on both sides
of the street in front of the monitored Building complex. Additionally, the near-by intersection between Jagtvej and Nørreallé (Fig. 2.1), which is another densely trafficked city road in Copenhagen, also may
play a role on the local air-pollution levels. At 4th floor height, however, these local effects are thought to be highly diluted and be of negligible importance as compared to the general traffic-load on Jagtvej
senso stricto.
2.2.3 The National Institute of Occupational Health, Denmark (NIOH)
Urban background particulate and PAH air-pollution were sampled at the 4th floor roof-top height of the National Institute of Occupational Health (NIOH) lecture hall (Fig. 2.3). The NIOH is located at the
NW-SE oriented Lersø Parkallé 105, DK-2100 Copenhagen (Fig. 2.1).
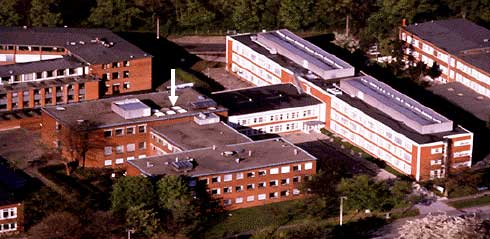
Figure 2.3: Photo of the National Institute of Occupational Health, Denmark showing where the roof-top was selected for urban background measurements. The samplers were placed at the arrow.
The NIOH Buildings are placed in a relatively open-Build area of Copenhagen dominated by two-to-three story office-Buildings and a park to the E and SE. The lecture hall, where measurements were
conducted, lays withdrawn from the Lersø Parkallé with no taller Buildings around it. Towards the E, behind the NIOH Buildings, an open area also occurs through which a electrically driven city-trains pass
twelve times per hour from 5 am to 7 pm and six times per hour from 7 pm to 1 am and on Sundays (Fig. 2.1).
On the lecture hall rooftop, the samplers were located approximately 5 m's from the inlet of an encased rooftop ventilation system (Fig. 2.3). The samplers were mounted next to each other app. 10-15 cm
apart 1.5 m above the rooftop shielded from direct sunlight at rain by a white hard-plastic bucket, similar to that at Jagtvej street canyon (section 2.2.1). The location of the samplers was chosen to minimize
the potential contamination from the exhaust duct, placed on the opposite site of the encasement. However, during SE wind directions, the ventilation shed may to a minor extent act as a shield. SE-winds
mainly occurred during the spring and summer time and varied between 5 and 23% of the time on a weekly basis (http://www.dmi.dk/dmi/index/viden/dmi-publikationer/tekniskerapporter.htm).
As the samplers were placed away from exhaust ducts, Building ventilation exhausts were not expected to affect the measurements significantly. No additional point sources to particles and PAH's were
identified in the near proximity of the samplers.
Further away, two major point sources; a fossil fuel energy plant (Vestforbrænding, Bispebjerg) and the local hospital crematorium (Bispebjerg Crematorium) occur to the E. Meteorological data showed
that eastern winds were frequent for the spring (0-36% of the time) and summer campaigns (0-70% of the time). However, owing to the strict environmental legislation for point sources in Denmark and the
relatively distant location, we expect the contamination to be short-time episodic and insignificant over the course of a sampling time of one week.
2.3 Indoor and outdoor meteorology
Wind direction and wind speed for Great Copenhagen are listed in Appendix A and were obtained from the Danish Meteorological Institute (DMI; http://www.dmi.dk/dmi/index/viden/dmi-publikationer/tekniskerapporter.htm) based on the Copenhagen Airport monitoring station. These data were used to assess potential effects from point sources as discussed in the
section above.
Indoor air-exchange rates were obtained from the TRIP-project until it ended May 8, 2002. During the TRIP study, the air-exchange rate was fixed at appr. 0.5 and 1 h-1 every alternating week during the
measurement campaigns and monitored using three-point trace gas measurements of SF6 . After May 8, 2002, the air-exchange was uncontrolled and not monitored.
2.4 Collection of particulate matter and volatile compounds
2.4.1 Collection of PM1, PM2.5 and Inhalable dust
Particles and PAH's were collected on GFC glass fibre filters (Millipore AP 4003705) using Triplex cyclones (BGI Inc.) for PM1 and PM2.5 and CIS's (Conical Inhalable Samplers) for inhalable dust
(PMinh). See Appendix B for a detailed description of sampling conditions and collection efficiencies of the samplers.
Mass-concentrations were determined by weighing using a high-precision Sartorius Micro Scale (Sartorius AG, Göttingen, Germany). Filters were weighed after 24 hours of equilibration in a climate
controlled weighing room (20°C; 50% RH) before and after exposure. Each filter series included three un-exposed filters used as internal controls for passive mass changes and chemical analysis of sorbed
volatiles in the PAH measurements. Weighing data were also corrected for mass loss during mounting and dismounting filters in the samplers (see Appendix B).
Prior to weighing and air-sampling, the GFC filters were cleaned in an EMITECH K1050X plasma asher (Emitech Ltd. Kent, U.K.) operated at 85 W using 15 mL O2 per minute for 15 minutes at an
air-pressure of 6 10-1 mbar. This was completed to prevent potential contamination of PAH's on the filters for the subsequent HPLC analysis.
After weighing, the filters were stored in a freezer (–20°C) placed in individual glass petri dishes and wrapped in aluminum foil until extraction for chemical analysis of organic compounds.
2.4.2 Collection of volatile compounds
SVOCs in the gas phase, not trapped by the filter, or evaporated from particles on the filter during sampling were collected using cleaned Tenax TA sample and backup tubes (see Appendix D) mounted
down stream the PM2.5 filter sampler. The main purpose was to collect volatile PAHs as well as check for the potential break-through of semi-volatile PAHs during sampling. Prior to sampling, the tubes
were cleaned by heating up to 275 °C in a stream of nitrogen. The tubes were checked for contamination by thermal desorption and GC with a FID detector.
The mass-concentrations of the volatile compounds were calculated based on the total volume flow for the respective PM2.5 samples.
2.5 Analysis of PAHs
2.5.1 Pressurized Liquid Extraction of glass fibre filters and Tenax TA
PAHs were extracted using a Dionex ASE 200 Accelerated Solvent Extractor for PLE equipped with 1-mL and 5-mL stainless steel extraction cells. PLE is a fully automated extraction process that uses
liquid solvents at high temperature (above the boiling point) and pressure (to keep the solvent liquid) for extraction of the sample. The solvent is pumped into the extraction cell containing the sample and after
some minutes with high temperature and pressure the extract is transferred from the heated cell to a collection vial. The sample was then ready for analysis. However, solvent volume reduction of glass fibre
filter extracts was required. The PLE parameters used for the PAH extraction are shown in Appendix D.
2.5.2 High Performance Liquid Chromatography (HPLC) analysis
Sixteen PAH compounds were analyzed using HPLC with UV and fluorescence detection (FD) using multiple wavelength shifts for simultaneous quantification of sixteen different PAH compounds . The
PAH compounds were separated by reversed-phase HPLC and detected by UV absorbance at 254 nm with a diode array detector (DAD) for quantification of acenaphtylene, fluoranthene and
indeno(1,2,3-cd)pyrene and a FD to quantify the other 13 PAH compounds using multiple wavelength shifts. The quality of the PAH analyses was controlled and documented with several different methods.
Analytical details and the HPLC parameters used for the PAH analysis are shown in Appendix D.
3 PM in indoor and outdoor air
In this chapter we discuss the levels of particulate air-pollution indoors and outdoors at Jagtvej and in the urban background. The relationships between PM1, PM2.5 and PMinh were investigated at the
three measuring points to create a regression line model for estimating missing data. Statistical analyses were conducted to suggest a yearly average concentration of the three PM fractions. Linear regression
analysis are performed to investigate the contribution for all three size fractions in the urban background on the particulate air-pollution in the street as well as the influence from both Jagtvej and urban
background to the particulate air-pollution indoors in the apartment studied.
3.1 PM1, PM2.5 and PMinh
Tables 3.1-3.3 list the weekly measured and estimated air-pollution mass concentrations indoors (IN) and outdoors (EXT) at Jagtvej and in the urban background air (NIOH). The tables include PM1 2001
fall data from Sharma (2002) and predicted mass-concentrations for weeks with missing data (see section 3.1.1).
Table 3.1: Weekly indoor mass-concentration at Jagtvej
Sampling period |
PM1_JGTVJ_IN |
PM2.5_JGTVJ_IN |
PMinh_JGTVJ_IN |
dd/mm-dd/mm 'yy |
[μg/m3] |
[μg/m3] |
[μg/m3] |
03/10-10/10 '01§ |
10.90 |
|
15.04 |
eq. 1 |
17.16 |
eq. 2 |
10/10-17/10 '01§ |
13.40 |
|
17.42 |
eq. 1 |
19.07 |
eq. 2 |
17/10-24/10 '01§ |
9.20 |
|
13.42 |
eq. 1 |
15.87 |
eq. 2 |
24/10-31/10 '01§ |
17.50 |
|
21.32 |
eq. 1 |
22.20 |
eq. 2 |
09/01-16/01 '02 |
14.73 |
±1.56 |
24.06 |
±3.23 |
24.39 |
eq. 3 |
16/01-23/01 '02 |
11.07 |
±3.12 |
13.53 |
±3.12 |
17.35 |
±1.23 |
23/01-30/01 '02 |
9.27 |
±1.37 |
15.83 |
±3.20 |
12.46 |
±0.87 |
30/01-06/02 '02$ |
7.73 |
±1.36 |
8.11 |
±1.36 |
10.44 |
±0.87 |
06/02-13/02 '02£,$ |
5.83 |
±1.32 |
13.61 |
±3.08 |
10.74 |
±0.85 |
03/04-10/04 '02 |
22.52 |
±1.61 |
26.14 |
±3.76 |
23.70 |
±1.04 |
10/04-17/04 '02 |
18.93 |
±1.38 |
19.91 |
±3.22 |
20.28 |
±0.89 |
17/04-24/04 '02 |
12.60 |
±1.35 |
16.66 |
eq. 1 |
13.95 |
±0.88 |
24/04-01/05 '02 |
7.82 |
eq. 1 |
12.11 |
eq. 1 |
11.38 |
±0.87 |
01/05-08/05 '02 |
6.20 |
±1.36 |
7.36 |
±3.22 |
12.44 |
±0.89 |
23/05-29/05 '02 |
- |
- |
- |
- |
- |
- |
29/05-05/06 '02 |
8.01 |
±1.36 |
12.54 |
±3.58 |
8.58 |
±0.89 |
05/06-12/06 '02 |
9.16 |
±1.39 |
13.63 |
±3.19 |
9.71 |
±0.87 |
12/06-19/06 '02 |
5.48 |
±1.37 |
11.02 |
±3.08 |
10.62 |
±0.88 |
19/06-26/06 '02 |
8.94 |
±1.93 |
11.94 |
±4.52 |
11.84 |
±1.24 |
Average |
11.07 |
±4.75 |
15.20 |
±5.04 |
15.12 |
±5.06 |
§ Measured PM1 data from Sharma (2002).
$ Ventilation slits moved from the living room window to the bedroom window facing the backyard.
£ The artificial door to the sealed off kitchen was open the last 24 h.
Shaded cells: modelled data based on linear regression analysis
eq. # in shaded cells refers to which regression equation in section 3.1.1. that was used.
Tabel 3.2: Weekly street canyon mass-concentrations at Jagtvej
Sampling period |
PM1_JGTVJ_EXT |
PM2.5_JGTVJ_EXT |
PMinh_JGTVJ_EXT |
dd/mm-dd/mm 'yy |
[μg/m3] |
[μg/m3] |
[μg/m3] |
03/10-10/10 '01§ |
13.10 |
|
18.70 |
eq. 4 |
32.99 |
eq. 5 |
10/10-17/10 '01§ |
10.70 |
|
15.67 |
eq. 4 |
29.82 |
eq. 5 |
17/10-24/10 '01§ |
16.50 |
|
22.98 |
eq. 4 |
37.48 |
eq. 5 |
24/10-31/10 '01§ |
11.60 |
|
16.81 |
eq. 4 |
31.01 |
eq. 5 |
09/01-16/01 '02 |
26.00 |
±1.56 |
34.95 |
eq. 4 |
58.86 |
eq. 16 |
16/01-23/01 '02 |
15.40 |
±1.39 |
24.59 |
±3.18 |
54.54 |
eq. 16 |
23/01-30/01 '02 |
8.15 |
±1.37 |
12.43 |
±3.20 |
29.65 |
±1.07 |
30/01-06/02 '02 |
12.91 |
±1.36 |
15.70 |
±3.16 |
32.47 |
±0.87 |
06/02-13/02 '02 |
7.40 |
±1.32 |
12.14 |
±3.08 |
27.90 |
±0.85 |
03/04-10/04 '02 |
19.94 |
±1.38 |
25.54 |
±3.23 |
37.38 |
±0.87 |
10/04-17/04 '02 |
25.60 |
±1.44 |
36.92 |
±3.45 |
51.28 |
±0.89 |
17/04-24/04 '02 |
22.99 |
±1.41 |
30.03 |
±3.19 |
49.45 |
±0.88 |
24/04-01/05 '02 |
12.17 |
±1.56 |
16.31 |
±3.15 |
33.58 |
±0.73 |
01/05-08/05 '02 |
11.03 |
±1.38 |
13.68 |
±3.22 |
22.79 |
±0.89 |
23/05-29/05 '02 |
- |
- |
- |
- |
- |
- |
29/05-05/06 '02 |
10.94 |
±1.34 |
14.53 |
±3.17 |
27.39 |
±0.87 |
05/06-12/06 '02 |
8.71 |
±1.35 |
14.34 |
±2.99 |
30.69 |
±0.89 |
12/06-19/06 '02 |
8.62 |
±1.33 |
14.12 |
±3.19 |
25.99 |
±1.64 |
19/06-26/06 '02 |
9.48 |
±1.93 |
16.90 |
±4.51 |
28.99 |
±1.74 |
Average |
13.96 |
±5.93 |
19.80 |
±7.66 |
35.68 |
±10.59 |
§ Measured PM1 data from Sharma (2002).
Shaded cells: modelled data based on linear regression analysis
eq. # in shaded cells refers to which regression equation in section 3.1.1. that was used.
Tabel 3.3: Weekly urban background mass-concentrations at NIOH
Sampling period |
PM1_NIOH |
PM2.5_NIOH |
PMinh_NIOH |
dd/mm-dd/mm 'yy |
[μg/m3] |
[μg/m3] |
[μg/m3] |
03/10-10/10 '01§ |
9.57 |
eq. 10 |
13.90 |
eq. 12 |
19.19 |
eq. 13 |
10/10-17/10 '01§ |
7.18 |
eq. 10 |
11.71 |
eq. 12 |
17.07 |
eq. 13 |
17/10-24/10 '01§ |
12.97 |
eq. 10 |
17.01 |
eq. 12 |
22.18 |
eq. 13 |
24/10-31/10 '01§ |
8.08 |
eq. 10 |
12.53 |
eq. 12 |
17.87 |
eq. 13 |
09/01-16/01 '02 |
22.45 |
eq. 10 |
25.70 |
eq. 11 |
39.27 |
±1.05 |
16/01-23/01 '02 |
8.89 |
±1.57 |
17.96 |
±3.49 |
34.96 |
±0.94 |
23/01-30/01 '02 |
6.15 |
±2.91 |
7.94 |
±3.21 |
16.96 |
eq. 13 |
30/01-06/02 '02 |
9.03 |
±1.39 |
13.29 |
±3.12 |
18.84 |
eq. 13 |
06/02-13/02 '02 |
4.05 |
±1.84 |
12.07 |
±2.85 |
18.75 |
±1.21 |
03/04-10/04 '02 |
19.18 |
±1.35 |
22.41 |
±3.14 |
25.63 |
±0.87 |
10/04-17/04 '02 |
23.30 |
±1.38 |
28.17 |
eq. 12 |
32.09 |
±0.89 |
17/04-24/04 '02 |
18.26 |
±1.35 |
21.38 |
±3.09 |
27.35 |
±0.85 |
24/04-01/05 '02 |
8.18 |
±1.35 |
13.52 |
±3.15 |
16.67 |
±0.87 |
01/05-08/05 '02 |
5.15 |
±1.38 |
9.12 |
±3.23 |
8.02 |
±0.88 |
23/05-29/05 '02 |
10.86 |
±1.41 |
11.11 |
±3.29 |
21.68 |
eq. 9 |
29/05-05/06 '02 |
4.27 |
±1.48 |
9.56 |
±3.58 |
16.23 |
±0.95 |
05/06-12/06 '02 |
7.71 |
±1.39 |
11.42 |
±3.37 |
19.96 |
±0.89 |
12/06-19/06 '02 |
6.18 |
±1.38 |
8.74 |
±3.21 |
14.45 |
±0.89 |
19/06-26/06 '02 |
7.11 |
±1.33 |
10.91 |
±3.10 |
16.52 |
eq. 13 |
Average |
10.43 |
±6.15 |
14.85 |
±5.99 |
21.27 |
±7.93 |
§ Data modelled based on data in Sharma (2002).
Shaded cells: modelled data based on linear regression analysis
eq. # in shaded cells refers to which regression equation in section 3.1.1. that was used.
The results show a wide range in the weekly particulate air-pollution at all sites. High levels occur during January 9 – 23 and March 27 – April 24, 2002 with PM1, PM2.5 and PMinh reaching up to
26.00±1.56, 36.92±3.45 and 58±(95% CI) g/m3 at Jagtvej, respectively (Tables 3.1-3.3; Fig. 3.1.1a). Lower air-pollution concentrations were observed at the NIOH with measured concentrations reaching
23.30±1.38 g/m3 and 39.27±1.05 g/m3 for PM1 and PMinh, respectively. The lowest air-pollution level was observed February 6 – 13 with a PM1 concentration of 7.40±1.32 g/m3 and 4.05±1.84 g/m3 at
Jagtvej and NIOH, respectively.
Independent of measuring site, PM1 on average makes appr. 70 wt% of PM2.5. The average content of PM2.5 in inhalable dust was 54 and 69 wt% at Jagtvej_EXT and NIOH, respectively. In the
uninhabited apartment PMinh consisted almost entirely of PM2.5.
3.1.1 Intrasite relationships and prediction of missing PM data
Scatter plots show that there generally is a strong correlation between the PM1, PM2.5 and PMinh from the same measuring locality. Fig. 3.1 shows an example of this co-variation for the PM
measurements at Jagtvej_EXT.
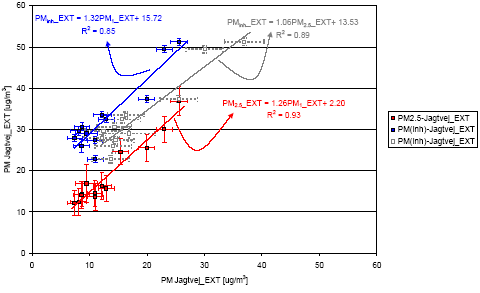
Figure 3.1: Scatter plot showing the high correlation for PM2.5 vs. PM1 (R2=0.93), PMinh vs. PM1 (R2=0.85) and PMinh vs. PM2.5 (R2=0.89) between the individual PM size fractions at Jagtvej_EXT.
The strong relationship between the fine and coarse size-fractions may be caused by the fact that the bulk of the particulate air-pollution is dominated by PM1. Another explanation may be that the dominant
particle sources emit particles in both fine and coarse particles. Regression analysis showed that the relationships between the size fractions measured at the same site enable prediction of missing
PM-concentration data with p-values of at least 0.035:
1) PM2.5_IN = 4.66 + 0.952 · PM1_IN (p=0.000; R2=77.3%)
2) PMinh_IN = 5.12 + 0.801 · PM1_IN (p=0.003; R2=83.7%)
3) PMinh_IN= 3.16 + 0.783 · PM2.5_IN (p=0.003; R2=65.4%)
4) PM2.5_EXT = 2.19 + 1.26 · PM1_EXT (p=0.000; R2=93.5%)
5) PMinh_EXT = 15.7 + 1.32 · PM1_EXT (p=0.000; R2=84.7%)
6) PMinh_EXT = 13.5 + 1.06 · PM2.5_EXT (p=0.000; R2=88.6%)
7) PM2.5_NIOH = 5.44 + 0.859 · PM1_NIOH (p=0.003; R2=76.7%)
8) PMinh_NIOH = 12.9 + 0.818 · PM1_NIOH (p=0.035; R2=44.7%)
9) PMinh_NIOH = 3.14 + 1.23 · PM2.5_NIOH (p=0.012; R2=62.1%)
The regression equation for prediction of PMinh_NIOH from PM1_NIOH had a relatively poor, but still significant p-value (p=0.035). The probability was slightly improved (p=0.012) using the PM2.5
data, but R2 values were relatively low for all analysis intrasite NIOH regressions. Much better statistics were obtained using the regression equations between the data from JGTVJ_EXT and NIOH (see
below). Consequently equations a to f were used to predict missing values at JGTVJ_IN and JGTVJ_EXT in Tables 3.1 and 3.2, respectively.
3.1.2 Outdoor-outdoor relationships and prediction of missing data
Similar to the intrasite co-variation, regression analysis showed a strong relationship between the levels of particulate air-pollution in the Jagtvej street canyon (Jagtvej_EXT) and the PM levels in the urban
background at NIOH (Fig. 3.2):
10) PM1_NIOH = - 3.50 + 0.998PM1_EXT (p=0.000; R2=90.1%)
11) PM2.5_NIOH = 1.93 + 0.914PM1_EXT (p=0.000; R2=83.1%)
12) PM2.5_NIOH = - 0.36 + 0.773PM2.5_ EXT (p=0.000; R2=86.0%)
13) PMinh_NIOH = - 2.82 + 0.667PMinh_EXT (p=0.000; R2=85.5%)
14) PM1_EXT = 4.48 + 0.903PM1_NIOH (p=0.000; R2=90.1%)
15) PM2.5_EXT = 2.85 + 1.112PM2.5_NIOH (p=0.000; R2=86.0%)
16) PMinh_EXT = 8.56 + 1.281PMinh_NIOH (p=0.000; R2=85.5%)
Owing to better statistics than observed for intrasite regression statistics (equations 7 to 9), the equations numbered 10 to 16 were used to generate PM concentrations for the missing data at NIOH and
Jagtvej_EXT (Table 3.3) when applicable.
Figure 3.1.2.1 shows a scatter plot with both measured and predicted PM data and associated linear regression equations. It is evident that the estimated values for the missing data generally fit very well
with the trend of the measured data. Hence, the estimated data were included in the further data analysis presented hereafter.
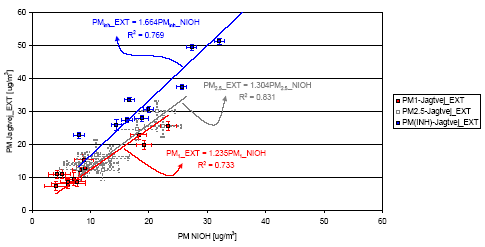
Figure 3.2: Scatter plot showing the correlation between PM in the Jagtvej street canyon and PM in the urban background at NIOH. The plot includes both measured and predicted data from the 2002
campaign.
3.1.3 The contribution from traffic to the particulate air-pollution
Based on the ultrafine to fine particle size of car exhaust, one may expect that traffic mainly would contribute significantly to the concentration of PM1 and have less pronounced effects on PM2.5 and PMinh.
However, coarse particles such as suspended dust as well as tire and brake wear particles also play an important role. Previous analysis by ) has shown that the contribution from "traffic" to PM10 was on
the order of 11.4 g/m3. In fact chemical analysis and particle characterization suggest that fine to coarse brake wear particles are quite significant constituents of this size fraction in the streets in Copenhagen
(pers. comm. P. Wåhlin, NERI;.
The current study can provide an assessment of the contribution from traffic to both PM1, PM2.5 and PMinh, assuming that the traffic contribution (dPM) can be estimated from the equation:
17) dPM = PM_EXT – PM_NIOH
Including estimated PM concentrations, equation 17 suggests that traffic on average contributes with 3.5±1.9 μg/m3 PM1; 5.0±2.7 μg/m3 PM2.5 and 14.6±4.0 μg/m3 PMinh, respectively at Jagtvej (Fig. 3.3).
Omitting estimated PM concentrations, did not change the amount of traffic dPM emissions significantly (Fig. 3.3). Noteworthy, the estimate of dPMinh (14.6±4.0 μg/m3) is within the same range as
determined for PM10 (11.4 μg/m3) by Palmgren et al. (2002). Despite PM10 and PMinh are not directly comparable measures the results support each other. However, the estimates of dPM from this
calculation should still be considered minimum values, because resuspension of dust and especially traffic emissions also contribute to the PM concentrations in the urban background.
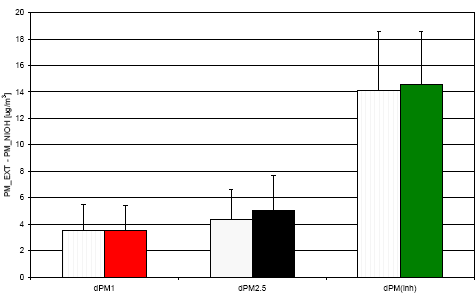
Figure 3.3: Average contribution from traffic (dPM) to the particulate air-pollution at Jagtvej estimated from equation 17. Filled bars are PM contribution from traffic based on both modeled and measured
data, whereas dotted bars are estimates based on measured data alone. Note there is only a minor difference between the estimates based on measured data alone and estimates including modeled data.
3.1.4 Indoor-outdoor relationships
Indoor-outdoor (I/O) mass-concentration ratios is often used to assess the penetration of outdoor air into the indoor environment. However, I/O-ratios, of-course cannot tell the origin of the particulate
air-pollution. Because the measurements were conducted in an uninhabited apartment, the influence from indoor sources was reduced to a minimum in this study. Hence, the indoor PM concentrations should
be a good measure of particles that have penetrated from the outdoor environment. Further assessment of the particle infiltration from outdoors is made by statistical analysis of the relationships between the
indoor and outdoor air-pollution levels.
3.1.4.1 I/O ratios
Table 3.4 lists the I/O (indoor/outdoor) ratios for the particulate air-pollution in the apartment compared to the air-pollution levels in the Jagtvej street canyon and in the urban background (NIOH). Table 3.4
also lists the measured average total air-exchange rates in the apartment.
The average I/O-ratios for PM1 (0.770.21) and PM2.5 (0.770.24) were almost the same using the outdoor PM concentrations from Jagtvej. The average I/O-ratio was notably higher: 1.120.34 and
1.050.35 for PM1, and PM2.5, respectively when indoor concentrations were compared to the city background data from NIOH. The I/O-ratio for PMinh was lower than observed for PM1 and PM2.5,
averaging 0.390.10 and 0.480.17 using street and urban background data, respectively. This was expected due to the poorer penetration efficiency for coarse particles.
It is important to note that the average PM1 and PM2.5 I/O ratios were higher than or close to unity when the I/O-ratios was calculated based on urban background data. This may be of relevance for
epidemiological studies that normally use urban background concentrations as a reference for estimating the frequency of adverse health effects. Since, the average I/O-ratios for PM1 and PM2.5 exceed
unity, when calculated based on urban background PM concentrations and not when calculated based on street concentrations, the street PM appears to have significant influence on the indoor particle
concentration in this fourth floor apartment. From the total mass concentrations, the bulk, however, appears still to be controlled by the urban background concentrations.
Table 3.4: I/O-ratios for indoor PM1, PM2.5 and PMinh versus the particulate air-pollution in the Jagtvej street canyon (IN/EXT) and in the urban background (IN/NIOH).
Sampling period
dd/mm-dd/mm `yy
|
χ h-1 |
PM1
IN/EXT
|
PM2.5
IN/EXT
|
IPMinh
IN/EXT
|
PM1
IN/NIOH
|
PM2.5
IN/NIOH
|
PMinh IN/NIOH |
09/01-16/01 '02 |
1.13 |
0.57 |
0.69 |
0.41 |
0.66 |
0.94 |
0.57 |
16/01-23/01 '02 |
0.68 |
0.72 |
0.55 |
0.32 |
1.25 |
0.75 |
0.25 |
23/01-30/01 '02 |
1.20 |
1.14 |
1.27 |
0.42 |
1.51 |
1.99 |
0.36 |
30/01-06/02 '02$ |
0.63 |
0.60 |
0.52 |
0.32 |
0.86 |
0.61 |
0.48 |
06/02-13/02 '02£,$ |
0.64 |
0.79 |
1.12 |
0.38 |
1.44 |
1.13 |
0.22 |
03/04-10/04 '02 |
0.70 |
1.13 |
1.02 |
0.63 |
1.17 |
1.17 |
0.75 |
10/04-17/04 '02 |
1.40 |
0.74 |
0.54 |
0.40 |
0.81 |
0.71 |
0.73 |
17/04-24/04 '02 |
0.75 |
0.55 |
0.55 |
0.28 |
0.69 |
0.78 |
0.67 |
24/04-01/05 '02 |
1.35 |
0.64 |
0.74 |
0.34 |
0.96 |
0.90 |
0.49 |
01/05-08/05 '02 |
0.61 |
0.56 |
0.54 |
0.55 |
1.20 |
0.81 |
0.64 |
23/05-29/05 '02 |
|
|
|
|
|
|
0.50 |
29/05-05/06 '02 |
§ |
0.73 |
0.86 |
0.31 |
1.87 |
1.31 |
0.26 |
05/06-12/06 '02 |
§ |
1.05 |
0.95 |
0.32 |
1.19 |
1.19 |
0.39 |
12/06-19/06 '02 |
§ |
0.64 |
0.78 |
0.41 |
0.89 |
1.26 |
0.43 |
19/06-26/06 '02 |
§ |
0.94 |
0.71 |
0.41 |
1.26 |
1.09 |
0.43 |
Average ± σ |
|
0.77±0.21 |
0.77±0.24 |
0.39±0.10 |
1.12±0.34 |
1.05±0.35 |
0.48±0.17 |
£ The artificial door to the sealed off kitchen was open the last 24 h.
$ Ventilation slits moved from the living room window to the bedroom window facing the backyard.
§ The air-exchange rate was uncontrolled and not monitored.
σ Standard deviation
Compared to a previous study, the average I/O-ratio for PM1 was slightly lower than that observed in the same apartment during May 15 – June 13 and October 10 – November 11, 2001 (0.89±0.33
μg/m3;. However, in Sharma's study the street data for PM1 were collected at first-floor level at the National Environmental Research Institute's Jagtvej monitoring station located on the other side of the
street ~500 m NNE of the apartment outside Jagtvej 108. Owing to predominant western winds in Copenhagen, the PM-levels at this monitoring station are generally lower than at the other side of the street
where the measurements in this study were conducted. During the current measurement campaign, the wind direction was in the western and eastern quadrant 42% and 29% of the time, respectively.
Additionally, the ventilation slits were not installed in the apartment before October 2001. Before, during the 2001 summer campaign, the outdoor air entered the apartment through a large tube placed in the
living room window. Both of these circumstances are expected to be of significant importance for the I/O relationships determined.
3.1.4.2 Variation in I/O ratio with air-exchange rate
It was anticipated that the infiltration of outdoor air-pollution would vary as function of the air-exchange rate in the apartment. - Increased air-exchange rates were expected to result in higher I/O ratios.
However, no clear relationship was observed between I/O-ratios, or indoor particle concentrations for that matter, with air-exchange rate. The indoor particle mass concentrations only varied as function of
the particle mass concentrations in outdoor air. Hence, variations in the ventilation rates appear to have insignificant effect on the indoor particle mass concentrations in the apartment. This is further supported
by the modeling results in Schneider et al. (2004), who showed that the penetration of outdoor particles in the size-range 0.5 to 4.0 m was controlled mainly by the outdoor particle concentration and the
indoor to street façade pressure difference.
3.1.4.3 Variation in indoor PM with outdoor PM
Scatter plots suggest a strong relationship between the levels of indoor particulate air-pollution with particulate air-pollution outdoors (Fig. 3.4). Based on the discussion above regression statistics was
employed for further analysis of the indoor-outdoor relationships. The variation in indoor PM mass concentration was compared against PM measurements in the street canyon (Jagtvej_EXT) and in the
urban background (NIOH) for each size fraction:
18) PM1_IN = 1.98+0.604PM1_EXT (p = 0.001; R2=63.5%)
19) PM1_IN = 3.78+0.636PM1_NIOH (p = 0.001; R2=75.7%)
20) PM2.5_IN = 5.54+0.457PM2.5_EXT (p = 0.004; R2=52.1%)
21) PM2.5_IN = 5.32+0.622PM2.5_NIOH (p = 0.001; R2=59.2%)
22) PMinh_IN = 2.14+0.329PMinh_EXT (p = 0.002; R2=51.1%)
23) PMinh_IN = 4.19+0.454PMinh_NIOH (p = 0.001; R2=60.4%)
It is clear that the indoor PM-levels can be predicted from outdoor particle concentrations with a relatively high probability for all three size-fractions measured. However, due to the high degree of mixing
between the particulate air-pollution at Jagtvej and in the urban background (Fig. 3.2), it is unclear, whether the particulate air-pollution in the street or in the urban background has the greater influence on
the indoor air from the direct regression analysis:
There appears to be a slightly better statistical relationship between the particulate air-pollution indoors and in the urban background. However, the I/O relationships using urban background data are
unexpectedly high for PM1 and PM2.5 exceeding unity eight times. For comparison, the I/O ratio only exceeded unity three times when street data were used (Table 3.4). The high I/O ratios may be
explained by episodic influence from indoor sources or indirect outdoor to indoor pathways. Indoor sources and indirect pathways have previously been suggested to play an important role on the fine and
ultrafine particle concentrations in the apartment . However, it is not likely that the indoor sources would contribute with so much particulate air-pollution that the indoor PM1 and PM2.5 would exceed the
outdoor air-pollution. Therefore, the apparent conclusion is that street air does have a significant influence on the indoor air pollution.
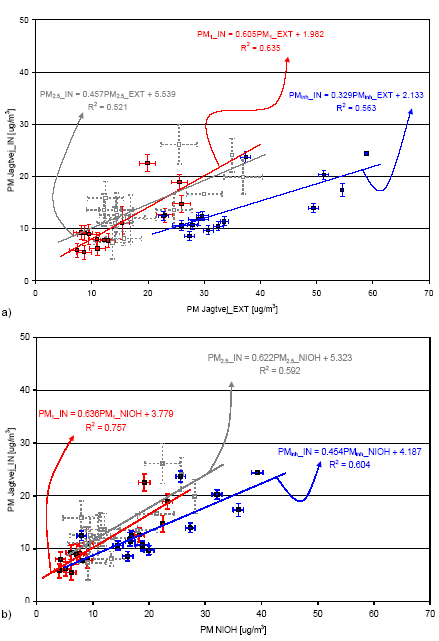
Figure 3.4: Scatter plots showing the relationship between the particulate air-polltion indoors and outdoors. a) PM1, PM2.5 and PMinh indoors vs. corresponding PM1, PM2.5 and PMinh at Jagtvej. b)
PM1, PM2.5 and PMinh indoors vs. corresponding PM1, PM2.5 and PMinh at NIOH.
Analyzing the relationship between I/O values with the levels of particulate air-pollution and meteorological conditions show that high I/O ratios mainly occur when the air-pollution levels are low (Fig. 3.5).
Low outdoor air-pollution levels generally occurred at high wind velocities and when the wind approached from the eastern and southern quadrants. In fact, the particle concentration showed a strong
relationship with an empirical wind-factor (Wf) based on percent of time the wind velocity was below 3 Beaufort times the percent of time the wind direction was between NE and SW (Fig. 3.6). A similar
trend was observed for PM in the urban background. This relationship may be explained by the fact that low wind velocities result in accumulation of traffic generated air-pollution in the street, whereas
long-range transported air-pollution from Eastern and Central Europe generally are transported along air masses from South and East.
The relatively strong relationship between the Wf and I/O ratios suggest that meteorological conditions may play an important role on the particle penetration efficiency and penetration routes in the studied
apartment complex. Especially elevated wind velocities could create complex pressure systems at Building facades and establishment of passage through alternative routes and/or enhancing transport of
air-masses vertically in the Building complex. Further studies are required to understand these relationships in detail.
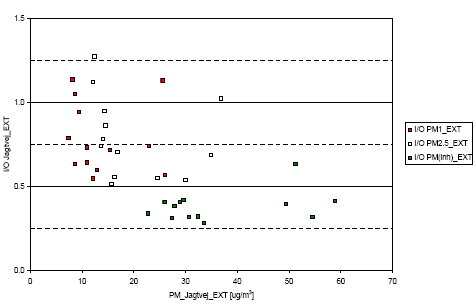
Figure 3.5: Scatter plot showing the relationship between the I/O ratio and the PM concentrations at Jagtvej_EXT.
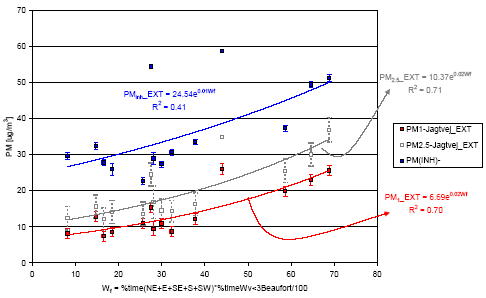
Figure 3.6: Variation in the particulate air-pollution in the Jagtvej street canyon with a combined wind direction and wind velocity (Wf) factor. The exponential regression equations were chosen based on
visual best fit. Atmospheric data are listed in Appendix A.
3.2 Seasonal variation and comparison with PM guidelines
3.2.1 Seasonal variation
The collected and modeled data allows an evaluation of the seasonal variation in the particulate air-pollution levels in Copenhagen (Tables 3.5-3.7). To ensure comparison between the size-fractions, for all
seasons, the summer season of was calculated based on four weeks only, omitting the NIOH data from the May 23-29, 2002 (Table 3.3). Despite predicted with high confidence (95%), the Fall '01
concentrations of PM2.5 and PMinh must be considered with caution as all data for that season are modeled.
Table 3.5: Seasonal and annual median and means for PM1, PM2.5 and PMinh at Jagtvej_EXT
Click here to see Table 3.5
Table 3.6: Seasonal and annual median and means for PM1, PM2.5 and PMinh at NIOH
Click here to see Table 3.6
The seasonal variation in the PM air-pollution is plotted in Figs. 3.7a to 3.7c representing each site. In the Jagtvej street canyon as well as in the urban background, all size fractions reached a maximum
median PM concentration during the spring 2002 (EXT: PM1 = 19.46, PM2.5 = 25.54 and PMinh = 37.38 μg/m3 and NIOH: PM1 = 18.26, PM2.5 = 21.38 and PMinh = 25.63 μg/m3). At both of these
outdoor locations, however, PMinh had higher maximum concentrations during the winter 2002 (Tables 3.5 and 3.6; Fig. 3.7). This may be caused by higher amounts of traffic-generated air-pollution such
as brake wear and resuspended dust (soil as well as sand and de-icing salts) from the road during wintertime. Increased effects of low wind velocities and long-range transport from eastern and
middle-eastern Europe may also have an effect as indicated by the relationship between the wind factor (Wf) and PM levels in Fig. 3.6. Indoors, the maximum medians were observed during fall 2001 (PM1
= 12.15, PM2.5=16.23 and PMinh=18.12 μg/m3) and spring 2002 (PM1 = 12.60, PM2.5=16.66 and PMinh=13.95 μg/m3).
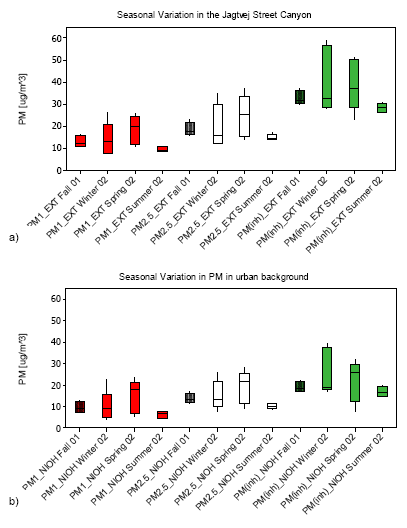
Figure 3.7: Seasonal variation of PM1, PM2.5 and PMinh using both measured and modelled data. a) PM in the Jagtvej street canyon (EXT); c) PM in the urban background (NIOH); and c) PM indoors at
Jagtvej (IN). Data from Fall '01 were obtained from Sharma (2002). The box plots show the median value, the upper and lower interquartile range and the 95% confidence interval for the interquartile range.
Table 3.7: Seasonal and annual median and means for PM1, PM2.5 and PMinh at Jagtvej_IN
Click here to see Table 3.7
3.2.2 Comparison with air-pollution guidelines
Table 3.8 summarizes selected air-pollution guidelines. Currently, the Danish Environmental Protection Agency (DK-EPA) operates based on a limit for 24-hour average TSP concentrations (Total
Suspended Dust) of 150 μg/m3. However, the EU has established a 2005 limit allowing a yearly average of 40 μg/m3 PM10 and maximum 24-hour average of 50 μg/m3 that may be exceeded up 35 days per
year. This limit will be further strengthened in 2010, where the annual average PM10 concentration must not exceed 20 μg/m3 or exceed a 24h-average of 50 μg/m3 more than 7 times.
No EU-regulation has been developed for PM1 and PM2.5. However, American Environmental Protection Agency (US-EPA) have currently a guideline for a yearly average PM2.5-concentration of 15
μg/m3, which the ASHRAE (American Society of Heating, Refrigerating and Air-conditioning Engineers) also suggest for indoor environments in the USA (Table 3.8). The EU, however, has formed a
working group for establishing revised European air-pollution guidelines, including a guideline for PM2.5 . This so-called CAFE working group has suggested that the future European guideline should not
exceed an annual average PM2.5 of 20 μg/m3 and a 90 percentile above 35 μg/m3 (Table 3.8).
Table 3.8: Selected National and International Air Quality Standards
Particle fraction |
Limit/target value |
Averaging time |
Statistics |
Period |
Effectuation date |
Organization |
TSP |
300
150
|
24 h
24 h
|
95 percentile
annual AM
|
Year
Year
|
In use
In use
|
EU£ |
PM10 |
50
40
50
20
|
24 h
24 h
24 h
24 h
|
≤35 times
annual AM
≤7 times
annual AM
|
Year
Year
Year
Year
|
2005
2005
2010
2010
|
EU£ |
PM2.5 |
15§
65
|
24 h
24 h
|
annual AM
98 percentile
|
Year Year |
In use |
US-EPA$ |
PM2.5 |
12-20
35
|
24 h
24 h
|
annual AM
90 percentile
|
Year Year |
in progress |
EU |
AM = Arithmic mean
£ Larssen et al. (2003) Air pollution in Europe 1990-2000, Topic report 4, EEA
$ www.epa.gov/airs/criteria.html
§ The guideline also accounts for indoor environments
The EU CAFE Working Group on Particulate Matter recommends that an annual limit value should not exceed 20 μg/m3 and considers 12 to 20 μg/m3 as input for further assessment. The 35 μg/m3 is
recommended as a reasonable starting point for a 24-hour limit.
Assuming that the average mass-concentrations represent the annual variation, the urban background concentration of PM2.5 (14.85 μg/m3) was within the region of the EU-CAFE limit value proposals of 12
to 20 μg/m3 and just below the USA-EPA limit of 15 μg/m3. For comparison the average PM2.5 concentration in the street was 19.80 μg/m3. Hence, the average street concentrations exceed the US-EPA
annual average and all, but the maximum, EU-CAFÉ limit value proposals.
Whether there is a risk of exceeding the 24-hour PM2.5 guideline was assessed by statistical analysis. The empirical distribution function analysis showed that the PM data could be described well with a
3-parameter lognormal distribution function. Fig. 3.8 shows the cumulative frequency plot and the distribution function for PM2.5 at all three sites. The 3-way distribution function was chosen above the
lognormal distribution function (P-value 0.026), because the 3-way function generally showed better fit and also defines a minimum threshold value based on the observed data. This prevents overestimation
of low-concentration data.
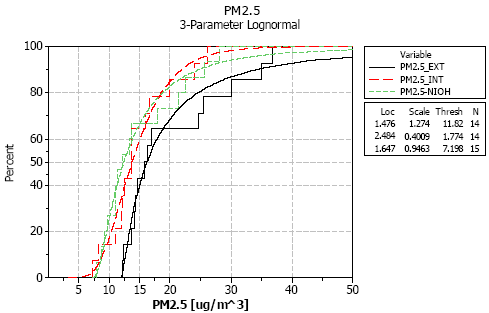
Figure 3.8: Cumulative histogram plot and the corresponding empirical 3-parameter lognormal distribution function for PM2.5_EXT, PM2.5_IN and PM2.5_NIOH.
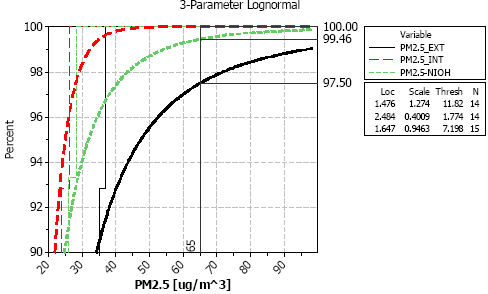
Figure 3.9: Percentiles for the 65 μg 24-hour limit on the empirical 3-parameter lognormal distribution function for PM2.5_EXT, PM2.5_IN and PM2.5_NIOH.
Currently, the US-EPA requires that the 98% percentile of the 24-hour PM2.5 concentrations is 65 μg/m3 or less. I.e., the average PM2.5 cannot exceed 65 μg/m3 more than seven days during a year. Based
on the distribution functions for PM2.5, more that 99% of the PM2.5 concentrations were below 65 μg/m3 in both the indoor and urban background air in this study (Fig. 3.9). In the street canyon, the
PM2.5 concentrations were statistically higher than 65 μg/m3 2.5% of the time (9 days) in 2002 and hence exceeded the seven-day limit (Fig. 3.9). Despite, the estimates at the maximum concentrations
drastically exceed reported values, a weekly average PM2.5 concentration of 60.23 μg/m3 was measured in the urban background at NIOH during March 27 and April 4, 2002 during sampling on Teflon
filters; outside of this project. This suggests that the statistical estimates presented are not unlikely.
Compared to the EU-CAFE proposal for PM2.5, the PM2.5 concentration at 4th floor height at Jagtvej would be below 35 μg/m3 90.47% of the time, hence just satisfying the potential EU 24-hour
guideline (Fig. 3.10). Similarly, the annual average PM2.5 EU-proposal of 20 μg/m3 was just satisfied in the street at Jagtvej (19.80±7.66 g/m3). In the urban background, the PM2.5 concentration was below
35 μg/m3 96.16% of the time and clearly complied with the potential future guideline.
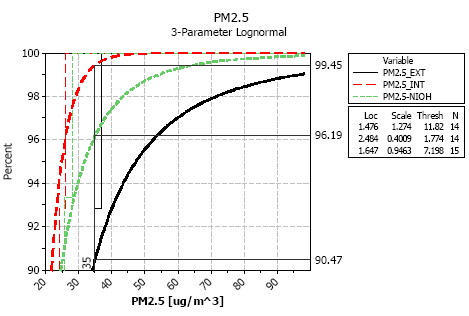
Figure 3.10: Percentiles for the 35 μg 24-hour limit on the empirical 3-parameter lognormal distribution function for PM2.5_EXT, PM2.5_IN and PM2.5_NIOH.
The average indoor PM2.5 air-pollution (15.20±5.04 μg/m3) in the uninhabited apartment slightly exceeded the ASHRAE recommendation of 15 μg/m3. Regarding regulation of the particulate air-pollution in
the indoor environment and considering that the apartment in this study was uninhabited, the results suggests great difficulties in compliance with the ASHRAE guideline for indoor environments and that
susceptible people living at Jagtvej frequently may suffer from respiratory symptoms.
3.3 Evaluation of particle induced health effects
Increased concentrations of atmospheric air-pollution can result in acute health effects, such as increased rates of hospitalization owing to respiratory symptoms and cardiovascular disease as well as
increased daily mortality. Long-term effects of air-pollution also occur and include increased risk of early mortality, respiratory morbidity and cancer related to long-term exposure to specific toxic
compounds in the air .
Several studies have reported strong relationships between the incidence of acute or accumulated chronic health effects and increased 24-hour concentrations of especially PM10 and PM2.5 (e.g., Künnzli et
al., 2001; Schwartz et al., 2001; 2002; Pope et al., 2002; Nafstad et al., 2004). The dose-response relationships are linear, at least up to several hundred μg/m3 of PM10 and the response, here exemplified
by acute daily mortality, is much stronger for PM2.5:
24) %IncrAcuteMort-WHO2.5 = (0.151±0.039)PM2.5)
than for PM10
25) %IncrAcuteMort -WHO10 = (0.070 ±0.012)PM10,
where %IncrAcuteMort-WHO is the percent increase in mortality per million as function of the 24-hour mass dose of the respective PM measure in μg/m3 given by the WHO (2000). The stronger effect
from PM2.5 is in accord with the higher deposition efficiency of PM2.5 in the lower human respiratory tract as compared to PM10. The higher dose-response relationship for PM2.5 also suggests that
assessments based on PM10 may underestimate the number of outcomes in regions if the atmospheric air-pollution is strongly dominated by fine particles.
Previously estimated the number of several health effects associated with atmospheric air-pollution in Denmark based on PM10. They concluded that 435 extra deaths would occur per million adults above
30 years age (30+) in Denmark, including long-term effects, per 10 μg/m3 increase in PM10. This mortality rate was determined at 7.5 μg PM10/m3 resulting in a baseline of 10,122 deaths per year/million
inhabitants. The corresponding number of excess hospitalization events for respiratory symptoms were 281 cases per million at a baseline of 14,670/year/million inhabitants.
3.3.1 Assessment of adverse health effects related to PM2.5
In relation to this study, Relative Risk (RR) estimates only exist for 24-hour PM2.5 measurements. Using the 7-day measurements, collected in this study directly, would result in underestimation of the
maximum dose-response effects as compared to estimates using the corresponding 24-hour measurements. Applying the statistical distribution in mass-concentration from the 3-parameter lognormal
distribution in Fig. 3.8 should minimize the risk of errors in the assessment of maximum and minimum acute dose-response effects. Moreover a statistical minimum value could be established, which is
required for baseline calculations (Rate-PM2.5base in equation 26). Consequently, the health effect assessment was made based on the statistical distribution of PM2.5 in the urban background (Fig. 3.8).
The variation was determined by converting the cumulative distribution frequency to number of days a year the air-pollution was below a specific PM2.5 level, assuming that the statistical range reflected the
24-hour variation (Table 3.9). The statistically determined lower 24-hour PM2.5 concentration of 7.57 μg/m3 was used to establish the baseline for the health effect assessment at which the contribution from
urban air-pollution was assumed negligible. The statistical upper 24-hour PM2.5 concentration of 79.12 μg/m3 was chosen as the maximum air-pollution level in the adverse health effect calculations (Table
3.9).
The effects on human health were assessed from published RR values for acute and accumulated (all cause) mortality, and for effects on acute hospitalization rates for cardiovascular and respiratory disease,
respectively. The accumulated mortality includes deaths in relation to long-term exposure. Results are given as the number of incidents per million inhabitants per 10 μg/m3 PM2.5. Health effect and population
data used in the assessments were retrieved from Statistics Denmark (http://www.statistikbanken.dk) for the years 1999, 2000 and 2002 and listed in Table 3.10. The only exception was the 2002 data for
mortality, which was estimated from the total number of deaths in 2002 using the average percentage of non-violent deaths in 1999 and 2000 (range 73.0±0.8% to 75.5±0.6% for the different population
groups). Disease and mortality data were collected for Denmark as a whole and the Copenhagen and Frederiksberg Counties in the central part of Greater Copenhagen. The national population data (total
population 5,313,577 to 5,368,354) were retrieved to test for abnormal variations in the Copenhagen data (total population 581,309 to 591,853). No sudden abnormalities were observed. However, higher
disease and mortality rates were generally observed in the Copenhagen population.
Table 3.9: Cumulative distribution of the statistical PM2.5 concentrations in the urban background (PM2.5_NIOH) and method for conversion from cumulative distribution to time (t) mid mass distribution
(PM2.5mid).
% of the Year |
PM2.5_NIOH [μg/m3] |
PM2.5mid [μg/m3] |
t [N days] |
0.0000001 |
7.22 |
- |
- |
0.000001 |
7.22 |
- |
- |
0.00001 |
7.24 |
- |
- |
0.0001 |
7.26 |
- |
- |
0.001 |
7.29 |
- |
- |
0.010 |
7.35 |
- |
- |
0.100 |
7.48 |
- |
- |
0.274 |
7.57 |
= 1 day minimum 7.57 |
= (0.274)*365/100 = 1.00 |
1 |
7.77 |
= (7.57+7.77)/2 = 7.67 |
= (1-0.274)*365/100 = 3.29 |
2 |
7.94 |
= (7.77+7.94)/2 = 7.86 |
= (2-1)*365/100 = 3.65 |
3 |
8.07 |
8.01 |
3.65 |
4 |
8.19 |
8.13 |
3.65 |
5 |
8.29 |
8.24 |
3.65 |
6 |
8.39 |
8.34 |
3.65 |
7 |
8.48 |
8.44 |
3.65 |
8 |
8.57 |
8.53 |
3.65 |
9 |
8.66 |
8.62 |
3.65 |
10 |
8.74 |
8.70 |
3.65 |
20 |
9.54 |
9.14 |
36.50 |
30 |
10.36 |
9.95 |
36.50 |
40 |
11.28 |
10.82 |
36.50 |
50 |
12.39 |
11.84 |
36.50 |
60 |
13.80 |
13.09 |
36.50 |
70 |
15.73 |
14.76 |
36.50 |
80 |
18.71 |
17.22 |
36.50 |
90 |
24.66 |
21.69 |
36.50 |
91 |
25.67 |
25.16 |
3.65 |
92 |
26.83 |
26.25 |
3.65 |
93 |
28.18 |
27.51 |
3.65 |
94 |
29.81 |
29.00 |
3.65 |
95 |
31.83 |
30.82 |
3.65 |
96 |
34.42 |
33.12 |
3.65 |
97 |
37.99 |
36.20 |
3.65 |
98 |
43.46 |
40.72 |
3.65 |
99 |
54.13 |
48.80 |
3.65 |
99.726 |
79.12 |
= (79.12-+54.13)/2 = 66.63 |
2.65 |
99.900 |
103.90 |
= 1 day maximum = 79.12 |
= (100-99.726)*365/100 = 1.00 |
99.990 |
182.83 |
- |
- |
99.999 |
301.11 |
- |
- |
In the first step of the assessment, the base level rates (Rate-PM2.5base) were determined from equation 26 where the number of incidents per million inhabitants at the lowest air-pollution level was
calculated:
26) Rate-PM2.5base = Nobs/(1+[(RR-1)((PMave- PM2.5base)/10 g/m3)]),
where Nobs is the observed number of incidents per million, RR is the relative risk estimate per million inhabitants per 10 μg/m3 increase in PM2.5, and PM2.5base (7.57 μg/m3) is the minimum PM2.5 level.
The number of cases per million per 10 μg/m3 (N-PM2.5) was subsequently estimated from equation 27:
27) N-PM2.5/10g/m3 = (RR-1)Rate-PM2.5base
3.3.2 Increase per million per 10 g/m3 increase in PM2.5
Table 3.11 lists the results from the assessment of acute mortality and cumulated mortality as well as the acute hospitalization rate for respiratory (Resp.) and cardiovascular diseases (CVD).
In all cases, the assessments for year 1999 and 2000 were conducted using PM2.5 levels for 2002. Only the 2002 assessments were based on corresponding PM and health data sets. The results for the
1999 and 2000 assessments can only be used for evaluation of disease and mortality variations within the population.
Table 3.10: Data materials used for assessment of adverse health effects from PM2.5.
Copenhagen+Frederiksberg |
1999 |
2000 |
2002 |
Acute Mortality |
5721 |
5283 |
5075 |
Acute Mortality 30+ |
5708 |
5267 |
5061 |
Total Mortality |
5721 |
5283 |
5075 |
Total Mortality 30+ |
5708 |
5267 |
5061 |
CVD hosp |
12699 |
12675 |
12087 |
CVD hosp 30+ |
12458 |
12518 |
11952 |
Resp. Hosp |
5315 |
5304 |
4457 |
Resp. Hosp 30+ |
4254 |
4158 |
3151 |
|
|
|
|
Denmark |
1999 |
2000 |
2002 |
Acute Mortality |
44187 |
42801 |
42738 |
Acute Mortality 30+ |
44157 |
42759 |
42803 |
Total Mortality |
44187 |
42801 |
42738 |
Total Mortality 30+ |
44157 |
42759 |
42803 |
CVD hosp |
110988 |
114118 |
115752 |
CVD hosp 30+ |
109672 |
112789 |
114490 |
Resp. Hosp |
40381 |
39764 |
37723 |
Resp. Hosp 30+ |
30315 |
29588 |
27323 |
|
|
|
|
No. Inhabitants |
1999 |
2000 |
2002 |
Copenhagen+Frederiksberg |
581309 |
586026 |
591853 |
30+ |
342163 |
343492 |
346403 |
Greater Copenhagen |
1002894 |
1008642 |
1017271 |
30+ |
616176 |
617898 |
622168 |
Denmark |
5313577 |
5330020 |
5368354 |
30+ |
3325654 |
3344351 |
3387396 |
Source: http://www.statistikbanken.dk
Filled cells = modeled data from percent of total mortality during 1999 and 2000
30+ denotes group of population at age 30 and above
The acute and total (all cause) mortality was estimated based on the average RR-value given by the WorLD Health Organization, WHO (2000) and the 500,000 people metropolitan study from 1999-2000
by Pope et al. (2002), respectively. In the acute mortality, the RR value is based on the total age-range, whereas Pope et al (2002) estimated their RR-value for accumulated mortality in adults of 30 years
age or oLDer (30+).
The assessment of acute mortality suggest that the Copenhagen air-pollution may result in approximately 130 acute deaths per million Central Great Copenhagen inhabitants per 10 μg/m3 increase in PM2.5
above the base level. Slightly lower effects (~120 ) were observed using national data (Table 3.11). The accumulated mortality including long-term health effects was notably higher and reached 721±480 and
833±555 per million per 10 μg/m3 increase in PM2.5, using national and Central Great Copenhagen data, respectively. For comparison Palmgren et al. (2002) only predicted 435 deaths per million per 10 μg
increase in PM10. Assessment using PM10 may underpredict the adverse health effects from particulate air-pollution the modern city environment. However, careful epidemiological studies are needed to
verify this.
Table 3.11: Adverse health effect from PM2.5 based on statistical urban background concentrations.
Copenhagen +
Frederiksberg
|
Relative Risk |
Rate-PM2.5/base (7.57 μg/m3) |
N/million/10μg
increase in PM2.5
|
|
RR (SD 95%CI) |
1999 |
2000 |
2002 |
1999 |
2000 |
2002 |
Acute Mort |
1.015 (0.004) £ |
9714.7 |
8898.7 |
8464.0 |
146± 36 |
133± 36 |
127± 34 |
Accum Mort 30+ |
1.06 (0.04) $ |
15853.6 |
14572.1 |
13885.8 |
951 ±583 |
874± 583 |
833 ±555 |
CVD hosp |
1.033 (0.023) § |
21235.2 |
21024.4 |
19851.7 |
701± 484 |
694± 484 |
655± 457 |
Resp. hosp. |
1.05£ |
8761.6 |
8673.1 |
7216.3 |
438 |
434 |
361 |
Denmark |
RR (SD 95%CI) |
1999 |
2000 |
2002 |
1999 |
2000 |
2002 |
Acute Mort |
1.015 (0.004) £ |
8208.6 |
7926.6 |
7858.5 |
123± 32 |
119 ±32 |
118 ±31 |
Accum Mort 30+ |
1.06 (0.04) $ |
12618.3 |
12150.5 |
12008.5 |
757± 486 |
729 ±486 |
721 ±480 |
CVD hosp |
1.033 (0.023) § |
20304.0 |
20812.2 |
20959.5 |
670 ±479 |
687 ±479 |
692± 482 |
Resp. hosp. |
1.05£ |
7282.4 |
7149.0 |
6733.7 |
364 |
357 |
337 |
£ WHO (2000)
$ Pope et al. (2002)
§ Metzger et al. (2004)
Increased hospitalization rates for cardiovascular disease (CVD Hosp.) and respiratory symptoms (Resp. hosp.) are highly associated with increased levels of air-pollution. Metzger et al. (2004) determined
a relative risk (RR) of 1.033±0.004 per 10 μg/m3 increase in PM2.5 based on data from 4,407,535 all age emergency department visits in Atlanta (Georgia, USA) from 1993 to 2000. The average
RR-estimate for increased hospitalization rates for respiratory symptoms (WHO, 2000) is slightly higher with a RR-value of 1.05 at a CI (confidence interval) of 95%.
The emergency visits for all CVD and respiratory symptoms reach 655±457 and 361 per million inhabitants in central Copenhagen per 10 μg/m3 increase in PM2.5, respectively. The assessment of CVD and
respiratory effects using national data (Table 3.11) results in similar rates as observed for Copenhagen (CVD=692±482; Resp.= 337).
3.3.3 Health effect assessment based on the PM2.5 distribution function
In section 3.3.2. the adverse health effects were estimated as function of a 10 μg/m3 increase in PM2.5. However, the expected number of adverse health effects is related to the actual exposure. To enable,
an assessment of the number of cases related to specific air-pollution levels, the cumulative distribution function was converted to a time distribution function showing number of days with mid-point PM2.5
levels (PM2.5mid). The procedure to do this and the resulting time and PM2.5mid data are shown in the right side of Table 3.9.
Thereafter, the number of incidents per million could be estimated using equation 28:
28) Nyear = Σ(Rate-PM2.5base(RR-1)PM2.5midt/365) for PM2.5mid = 7.57 to 79.12
The 1-day upper and lower urban back-ground PM2.5 level varied between 92.12 μg/m3 and 7.57 μg/m3 in the distribution function, respectively (Table 3.9). Assessment of the corresponding number of
accumulated (all cause) daily deaths suggested an increase in up to app. 50% (= 117 people per million; Table 3.12). The corresponding maximum in excess hospitalizations for respiratory and
cardiovascular disease reached was app. 25 (14±10 cases permillion) and 40% (8 cases per million), respectively.
Table 3.12: Range and total number of PM2.5 dose-response effects in central Copenhagen.
Central Great Copenhagen |
% Range in increased response effects£ |
Range in
n cases/million/day£
|
Total
N cases/million/year£
|
Acute Mort |
1.1 – 11.9 |
0±0 – 3±1 |
196±50 |
Accum Mort 30+ |
4.5 – 47.5 |
1±1 – 11±7 |
780±520 |
CVD hosp |
2.5 – 26.1 |
1±1 – 14±10 |
1006±701 |
Resp. hosp. |
3.8 – 39.6 |
1 – 8 |
554 |
£ Response effects were calculated with 95% CI based on the RR values listed in Table 3.11
Summing up over the year, the annual excess acute mortality was estimated 196±50 per million inhabitants in central Copenhagen (Table 3.12). The number of accumulated deaths were estimated as 780±520
per million inhabitants in the same population. Using similar technique, the predicted number of excess hospitalization events for CVD and respiratory symptoms amounted to 1006±701 and 554 per million
Copenhagen inhabitants, respectively. Hence, in addition to approximately 800 early deaths, at least 1500 extra hospitalizations are expected to have occurred in Copenhagen per million inhabitants in 2002
owing to fine urban air-pollution.
4 PAHs in indoor and outdoor air
4.1 Evaluation and description of PAH-concentrations
The mean indoor, outdoor and urban background PM1, PM2.5 and PMinh concentrations of 16 EPA (Environmental Protection Agency, USA) priority PAHs are shown in Table 4.1 to Table 4.3. The
tables contain data on PAHs in both the gas and particle phase and individual results are listed in Appendix F. The air concentrations are based on the concentrations measured in the extracts of the filters
and Tenax TA above and below the defined instrumental limits of detection (LDs) except concentrations termed as "not detected" (n.d.) (see Appendix E). LDs are essentially a function of the uncertainty of
the analysis and in this report we consider values below the LDs as an indication of a larger uncertainty and not as "not detected".
4.1.1 Distribution of PAHs on the glass fibre filters and Tenax TA
PAHs in the air are distributed between the gas phase and the particulate phase depending on the physico/chemical properties of the particles and the individual PAHs, respectively (see e.g. ). When sampled
the particles are collected on the filter and the PAHs escaping as gasses from the filter are collected on the Tenax TA sample tube downstream the filter. PAHs that are not trapped by the Tenax TA sample
tube are colleted on the Tenax TA backup tube downstream the sample tube. During sampling, PAHs in the gas phase may be trapped by the filter and PAHs originally contained in the particles may be
volatilised and trapped by the Tenax TA. Thus concentrations estimated from analysis of the filter and the Tenax TA are not exact the concentrations in the particle and gas phases, respectively. However,
the lower the vapour pressure of the PAHs the larger are the fraction in the particle phase and the lower are the tendency to escape from the filter during sampling as illustrated in Figure 4.1. This effect is
usually observed when semi-volatile organic compounds (SVOCs) are sampled with filter/adsorbent samplers. For example linear alkanes with 18-20 carbon atoms were trapped on both filter and
adsorbent and alkanes with more than 20 carbon atoms were on the filter exclusively .
PM1 and PMinh PAH concentrations in Tables F 1 and F 3 (Appendix F) and Table 4.1 and Table 4.3 are based on analysis of filters only since no Tanax TA tubes were used for these samplings. The
PM2.5 concentrations for the 8 first PAHs (the most volatile) listed in Table F 2 and Table 4.2 are the sums of the concentrations estimated from analysis of the filter, the Tenax TA sample tube, and the
Tenax TA backup tube. The PM2.5 concentrations for the 8 last PAHs (the least volatile) listed in Table F 2 and Table 4.2 are estimated from analysis of the filters only owing to strong interference from
unknown compounds in the Tenax TA extracts. However, this introduces probably an insignificant error due to the high fraction of these PAHs in the particle phase and their low volatility from the filter. This
assumption is supported by the observation that the fraction of Flt, Pyr, B(a)A, and Chry in the particle phase in tobacco smoke has been estimated to 0.38, 0.18, 0.99, and 0.97, respectively, using denuder
technique . Thus, as an advantage this makes the concentrations of the last 8 PAHs listed in Tables F 1 – F 3 and Table 4.1 to Table 4.3 directly comparable. Acenaphtylene also interfered with unknown
compounds in the Tenax TA extracts during the HPLC analysis and were treated as described in Appendix B in the section "Data treatment". In spite of that, the acenaphtylene data probably still suffered
from positive bias and were omitted from the statistical analysis.
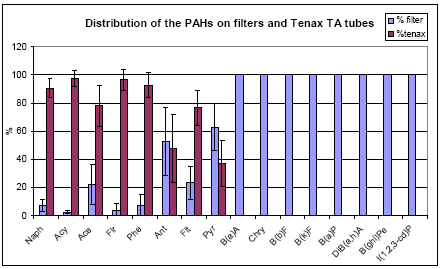
Figure 4.1: The observed average distribution of PAHs sampled on PM2.5 filters and Tenax TA tubes downstream the filters. The error bars are 95% confidence intervals. See Appendix C for abbreviations
of the PAH names. The fraction of the 8 least volatile PAHs on the filters have all been set to 100% since the Tenax TA data was rejected due to interference.
4.1.2 Artifacts influencing the measured concentrations of PAHs
In addition to the above-mentioned artifacts, sampling and analysis of PAHs is influenced by several effects that may lead to underestimation of the air concentrations . Among those are the extraction
recovery from filters and Tenax TA that were 60 – 100% for the PAHs (see Appendix D). During sampling PAHs broke through from the Tenax TA sample tubes to the backup tubes depending on the
volatility of the individual PAHs but were less than 15% on average for all PAHs (see Table D1 in Appendix D). When found on the backup tube an unknown amount of the PAH may have broken through
the backup tube, too. During sampling PAHs are also degraded by ozone and nitrogen oxides . Schauer et al. (2003) found that the PAHs collected on the filter may be underestimated by a factor of 2 or
more due to reaction with ozone and that the degradation was important for the variability of the results. During the sampling campaigns at Jagtvej ozone was present most of the time at concentrations up to
ca. 50 ppb outdoors and below ca. 5 ppb indoors .
4.1.3 Occurrence of the measured PAHs
In the PM2.5 samples the total concentrations of the 8 most volatile PAHs of the 16 EPA priority PAHs (ΣlowPAH) in the gas plus particle phases (shown in Table F 2) were 15-284 ng/m3 indoors, 46-232
ng/m3 outdoors, and 1-105 ng/m3 in the urban background air. The total concentrations in the PM2.5 samples of the 8 least volatile PAHs of the 16 EPA priority PAHs (ΣhighPAH shown in Table F 2) were
0.1-3.7 ng/m3 indoors, 0.3-3.9 ng/m3 outdoors, and 0.1-1.2 ng/m3 in the urban background air. The total concentrations in the PM1 samples of the 8 least volatile PAHs (ΣhighPAH shown in Table F 1)
were 0.1-2.3 ng/m3 indoors, 0.1-3.5 ng/m3 outdoors, and 0.2-1.6 ng/m3 in the urban background air. The total concentrations in the
PMinh samples of the 8 least volatile PAHs (ΣhighPAH shown in Table F 3) were 0.1-1.7 ng/m3 indoors, 0.3-3.1 ng/m3 outdoors, and 0.1-3.0 ng/m3 in the urban background air.
Table 4.1: Mean concentrations and standard deviations () based on all measurements of the 8 least volatile PAHs in PM1 (ng/m3).
PAHs |
Jagtvej indoor |
Jagtvej street |
City background |
|
Mean |
σ |
Mean |
σ |
Mean |
σ |
B(a)A |
0.03 |
0.04 |
0.12 |
0.18 |
0.03 |
0.04 |
Chry |
0.06 |
0.05 |
0.23 |
0.20 |
0.09 |
0.10 |
B(b)F |
0.15 |
0.10 |
0.24 |
0.22 |
0.19 |
0.19 |
B(k)F |
0.07 |
0.06 |
0.11 |
0.09 |
0.05 |
0.05 |
B(a)P |
0.11 |
0.12 |
0.08 |
0.08 |
0.04 |
0.06 |
DB(a,h)A |
0.05 |
0.05 |
0.04 |
0.03 |
0.04 |
0.02 |
B(ghi)P |
0.28 |
0.21 |
0.36 |
0.33 |
0.10 |
0.08 |
I(1,2,3-cd)P |
0.12 |
0.20 |
0.19 |
0.30 |
0.04 |
0.11 |
Table 4.2: Mean concentrations (gas + particle) and standard deviations () based on all measurements of the 16 PAHs in PM2.5 (ng/m3).
PAHs |
Jagtvej indoor |
Jagtvej street |
City background |
|
Mean |
σ |
Mean |
σ |
Mean |
σ |
Naph |
33.19 |
30.21 |
103.19 |
45.96 |
31.49 |
20.58 |
Acy£ |
19.27 |
5.76 |
21.40 |
4.51 |
17.19 |
5.66 |
Ace |
6.09 |
7.17 |
2.57 |
2.10 |
3.73 |
3.37 |
Flr |
3.38 |
2.32 |
4.74 |
2.67 |
2.06 |
1.10 |
Phe |
22.17 |
15.04 |
8.58 |
4.86 |
2.55 |
2.16 |
Ant |
0.28 |
0.56 |
0.43 |
1.31 |
0.18 |
0.34 |
Flt |
25.61 |
26.96 |
7.99 |
4.11 |
2.66 |
3.28 |
Pyr |
0.71 |
0.92 |
1.76 |
4.78 |
0.22 |
0.33 |
B(a)A |
0.04 |
0.07 |
0.09 |
0.09 |
0.04 |
0.05 |
Chry |
0.11 |
0.09 |
0.22 |
0.17 |
0.06 |
0.05 |
B(b)F |
0.25 |
0.20 |
0.35 |
0.23 |
0.21 |
0.20 |
B(k)F |
0.11 |
0.10 |
0.10 |
0.09 |
0.05 |
0.04 |
B(a)P |
0.12 |
0.15 |
0.09 |
0.09 |
0.05 |
0.05 |
DB(a,h)A |
0.09 |
0.12 |
0.21 |
0.53 |
0.08 |
0.06 |
B(ghi)P |
0.24 |
0.25 |
0.33 |
0.24 |
0.14 |
0.08 |
I(1,2,3-cd)P |
0.12 |
0.29 |
0.21 |
0.28 |
0.02 |
0.05 |
£ Given as minimum detection limit value if detected by GC-MS due to interference in HPLC analysis
Table 4.3: Mean concentrations standard deviations () based on all measurements of the 8 least volatile PAHs in PMinh (ng/m3).
PAHs |
Jagtvej indoor |
Jagtvej street |
City background |
|
Mean |
σ |
Mean |
σ |
Mean |
σ |
B(a)A |
0.02 |
0.02 |
0.14 |
0.12 |
0.03 |
0.04 |
Chry |
0.06 |
0.04 |
0.29 |
0.22 |
0.11 |
0.12 |
B(b)F |
0.18 |
0.13 |
0.22 |
0.31 |
0.15 |
0.11 |
B(k)F |
0.06 |
0.06 |
0.11 |
0.09 |
0.05 |
0.05 |
B(a)P |
0.09 |
0.09 |
0.09 |
0.11 |
0.08 |
0.14 |
DB(a,h)A |
0.04 |
0.04 |
0.06 |
0.06 |
0.02 |
0.03 |
B(ghi)P |
0.26 |
0.21 |
0.30 |
0.35 |
0.16 |
0.39 |
I(1,2,3-cd)P |
0.12 |
0.18 |
0.17 |
0.28 |
0.09 |
0.17 |
The average PM2.5 concentration profiles of the 16 PAHs for the indoor, outdoor, and urban background measurements are shown in Figure 4.2 and shows that the most volatile PAHs are the most
abundant in both the indoor, outdoor, and urban background environments. Figure 4.2 also shows the average PM1 and PMinh concentration profiles of the 8 least volatile PAHs for the indoor, outdoor,
and urban background measurements. These profiles indicate that largely the same levels of PAH concentrations were measured in the different particle fractions. This further indicates that the major part of
the least volatile PAHs is associated with the PM1 fraction, because the PM1 mass concentrations were much smaller than the mass concentrations of both PM2.5 and PMinh (see Tables 3.1 to 3.3).
Click here to see the Figure
Figure 4.2: Arithmetic mean concentrations and 95% confidence limits (at two scales) of all PM2.5 measurements of the 16 PAHs in the gas plus particle phase and the mean concentrations of the all PM1
and PMinh measurements of the 8 least volatile PAHs in the indoor, outdoor and background air samples. See Appendix C for abbreviations of the PAH names. Acenaphtylene (Acy) was somewhat
overestimated due to interference (see Appendix D, "Data treatment").
4.2 Indoor-outdoor relationships for PAHs associated with PM2.5
The relationships between specific PAHs associated with PM2.5 (gas + particle phases) were assessed using concentration ratios and regression analysis based
on the measurements of PAHs indoors in the 4th floor apartment at Jagtvej, outdoors in the Jagtvej street-canyon, and in the urban background.
4.2.1 Street to background relationships
Figure 4.3: Figure 4.3a shows that the PAH concentrations outdoors at Jagtvej often are notably higher than those found in the urban background. The major part of the street-to-background ratios are above 2.
However, the uncertainty of the ratios is very large in most cases due to the large variability of the data.
If it is assumed that the street and background air are well mixed in the street, that no local point sources influence the background measurements but that point sources may influence the street measurements
the following equation can be formed:
29) Street = street sources (traffic) + local point sources + background
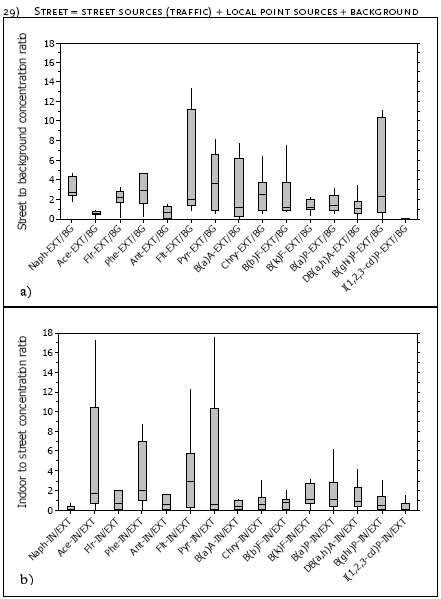
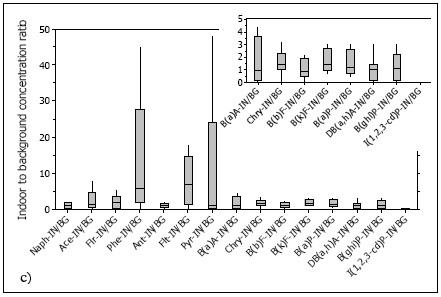
Figure 4.3: Boxplots of the concentration ratios of the PM2.5 PAHs in the gas plus particle phase between the a) street and urban background (EXT/BG), b) the indoor air and the street (IN/EXT), c) and
the indoor air and the urban background (IN/BG). The boxplots show the median, upper and lower interquartile range, and the 95% confidence limits.
The experimental data shown in Figure 4.3a support this model since the street concentrations of all PAHs are generally higher than that observed in the urban background, except for Ace and Ant with
median EXT/BG (street-to background) ratios of 0.460 and 0.625, respectively. If it is assumed that local point sources have no influence this suggests that traffic contributes significantly to the concentration
of most PAHs in the street canyon. Median street to background ratios above 2 were observed for Naph, Flr, Phe, Flt, Pyr, Chry, and B(ghi)P. Additionally, the large interquartile ranges observed for
B(a)A and B(b)F suggests that these PAHs at least episodically are also influenced from high emissions in the Jagtvej street canyon.
Indoor-outdoor relationships in Figure 4.3b and c show boxplots of the I/O concentration ratios for each of the 16 PAHs and Figure 4.4 and Figure 4.5 show scatter plots of the indoor PM2.5
concentrations versus the outdoor concentrations at Jagtvej of the most and least volatile PAHs, respectively. The percentage of I/O ratios above one for Jagtvej outdoors and urban background were 56%
and 75%, respectively. The I/O ratios typically show the same trend for Naph, Flr, Ant and the series Pyr to I(1,2,3,cd)P independent of whether the street or urban background concentrations were used
for comparison. However, for Pyr to I(1,2,3,cd)P, median IN/BG ratios were generally higher (1.03 -1.42) than the median IN/EXT ratios (0.29-1.03). Except for Pyr, regression analysis showed
significant (p 0.05) to near significant (p ≤0.15) relationships between indoor and street or background concentrations for these compounds (see Table 4.4 and Table 4.5).
Depending on the wind direction more or less background air may be infiltrated to the apartment from the backside of the house. If it is assumed that the street and/or background and indoor air are well
mixed indoors the following equation can be formed:
30) Indoor = indoor sources + P(Wstreet·street + Wbackside·background)
Where Wstreet and Wbackground are wind dependent factors and P is a penetration factor. Penetration factors are in the range 0 < P < 1 and are usually approximately 0.5. When the street to background
concentration ratios are close to one and have small variation the wind factor is not important and the equation can be simplified to:
31) Indoor = indoor sources + P street, when EXT/BG ~ 1
Indoor sources may vary a lot independent of the street concentrations and thus correlations of indoor and street air may result in large positive or negative intercepts and negative slopes. However, for
PAHs with indoor to street ratios close to one and a small variation, indoor sources are probably not important and the equation can be further simplified:
32) Indoor = P street, when EXT/BG ~ 1 and IN/EXT ~ 1
Thus, a correlation of indoor and street PAHs fulfilling these criteria based on the box plots in Figure 4.3b and c should have a positive slope smaller than 1 and an intercept close to zero if the PAHs from
the street and/or background air infiltrate to the indoor air.
Table 4.4 shows that for PAHs approximately fulfilling the criteria B(b)F, B(k)F, B(a)P, and DB(a,h)A have significant (p < 0.05) or near significant (p < 0.15)
Click here to see the Figure
Figure 4.4: Scatter plots, regression lines, and the 1:1 lines showing the relationship between the PM2.5 concentrations of the most volatile PAHs indoors versus outdoors in the Jagtvej street canyon.
indoor to street correlations. B(a)P and DB(a,h)A have slopes greater than one indicating that these PAHs also have important indoor sources. The same tendency
is shown in Table 4.5 for the indoor to background correlations. The significant correlations indicate that these PAHs are infiltrated from the outdoor air.
Considering indoor sources, the median I/O ratios for Ace, Phe, Flt and Pyr are notably above 1 (1.36-6.72) compared to both street and urban background concentrations. This suggests strong episodical
influence of these compounds from indoor sources.
Click here to see the Figure
Figure 4.5: Scatter plots, regression lines, and 1:1 lines showing the relationship between the PM2.5 concentrations of the least volatile PAHs indoors versus outdoors in the Jagtvej Street Canyon.
4.2.3 The contribution from traffic to PAHs in the indoor air
The influence of traffic on the indoor air-pollution of specific PAHs associated with PM2.5 (gas + particle phases) was assessed by regression analysis based on the measurements of PAHs indoors in the 4th
floor apartment at Jagtvej and outdoors in the Jagtvej street-canyon.
Harrison et al. state that B(ghi)P and coronene in diesel emissions and gasoline emissions are similar, but diesel emissions are probably enriched in B(b)F and B(k)F relative to gasoline emissions. In the
present study
Table 4.4: Linear regression statistics of PAHs in the indoor air versus in the Jagtvej street canyon. P-values in boLD are significant at 5% and p-values in boLD italics are significant at 15%.
Jagtvej_IN |
|
Intercept |
Slope |
p-value for slope = 0 |
R2 (%) |
n* |
Naph |
= |
- 11 |
0.43 |
0.016 |
46 |
12 |
Acy£ |
|
|
Not applicable |
- |
- |
- |
Ace |
= |
4.8 |
0.51 |
0.63 |
2 |
12 |
Flr |
= |
6.1 |
- 0.59 |
0.014 |
47 |
12 |
Phe |
= |
20 |
0.23 |
0.81 |
1 |
12 |
Ant |
= |
0.24 |
0.10 |
0.43 |
6 |
12 |
Flt |
= |
12 |
1.7 |
0.40 |
7 |
12 |
Pyr |
= |
0.79 |
- 0.041 |
0.49 |
5 |
12 |
B(a)A |
= |
0.026 |
0.23 |
0.28 |
11 |
13 |
Chry |
= |
0.092 |
0.11 |
0.52 |
4 |
13 |
B(b)F |
= |
0.13 |
0.37 |
0.14 |
19 |
13 |
B(k)F |
= |
0.050 |
0.65 |
0.032 |
35 |
13 |
B(a)P |
= |
0.015 |
1.2 |
0.012 |
45 |
13 |
DB(a,h)A |
= |
0.011 |
1.2 |
0.017 |
42 |
13 |
B(ghi)P |
= |
0.16 |
0.26 |
0.43 |
6 |
13 |
I(1,2,3-cd)P |
= |
0.0036 |
0.63 |
0.043 |
32 |
13 |
£ Not applicable, because only "not detected" data exist
*Number of observations including measurements with zero concentrations
Table 4.5: Linear regression statistics of PAHs in the indoor air versus in the urban background. P-values in boLD are significant at 5% and p-values in boLD italics are significant at 15%.
Jagtvej_IN |
|
Intercept |
Slope |
p-value for slope = 0 |
R2 (%) |
n* |
Naph |
= |
- 7.1 |
1.2 |
0.008 |
56 |
11 |
Acy£ |
|
|
Not applicable |
- |
- |
- |
Ace |
= |
2.0 |
1.0 |
0.154 |
21 |
11 |
Flr |
= |
6.4 |
1.4 |
0.091 |
29 |
11 |
Phe |
= |
27 |
1.7 |
0.474 |
6 |
11 |
Ant |
= |
- 0.017 |
1.3 |
0.002 |
66 |
11 |
Flt |
= |
13 |
4.8 |
0.064 |
33 |
11 |
Pyr |
= |
0.96 |
- 0.96 |
0.290 |
12 |
11 |
B(a)A |
= |
0.002 |
1.1 |
0.000 |
75 |
12 |
Chry |
= |
0.035 |
1.0 |
0.015 |
46 |
12 |
B(b)F |
= |
0.21 |
0.099 |
0.733 |
1 |
12 |
B(k)F |
= |
0.079 |
0.34 |
0.633 |
2 |
12 |
B(a)P |
= |
0.039 |
1.1 |
0.063 |
30 |
12 |
DB(a,h)A |
= |
0.027 |
0.97 |
0.068 |
30 |
12 |
B(ghi)P |
= |
- 0.038 |
1.7 |
0.007 |
53 |
12 |
I(1,2,3-cd)P |
= |
0.048 |
- 0.33 |
0.461 |
6 |
12 |
£ Not applicable, because only "not detected" data exist
*Number of observations including measurements with zero concentrations
another traffic marker was established using Chry + B(b)F + B(k)F (called Diesel2). Figure 4.6 shows that the concentration of this marker was highest in the street and lowest in the background air.
Regression analysis using the traffic marker (B(b)F + B(k)F + B(ghi)P) showed a strong I/O relationship using street data (traffic IN = 0.56·traffic_EXT + 0.17 [ng/m3]; p = 0.056), but not when compared
to urban background data (p=0.703). The Diesel2 marker shows also a good relationship between outdoor and indoor air at Jagtvej: Diesel2_IN = 0.47·Diesel2_EXT + 0.17 (p = 0.052; n=13). The
relationship between the Diesel2 marker in the urban background air and the street air at Jagtvej show poor regression statistics with p-values above 0.467. This suggests that traffic emissions in the street air
have greater direct influence on the PAHs in the apartment than the similar PAHs in the urban background air.
4.3 Seasonal variation of the PAH concentrations
The collected data allows an evaluation of the seasonal variation of the ΣPAH concentrations indoors and outdoors at Jagtvej and in the urban background air. To ensure comparison between the
size-fractions, for all seasons, the summer season was calculated based on four weeks only, omitting the urban background data from the May 23-29, 2002 (see Tables F1 – F3, Appendix F).
The seasonal variation in ΣhighPAH concentrations associated with the PM air-pollution is plotted for each site in Figure 4.7 to Figure 4.9. At all three sites and size fractions, the main trend was highest
concentrations in the winter followed by spring and the lowest concentrations in the summer. This indicates that domestic heating during winter and to some extent during spring is an important source of
PAHs indoors and outdoors in the street and urban background air.
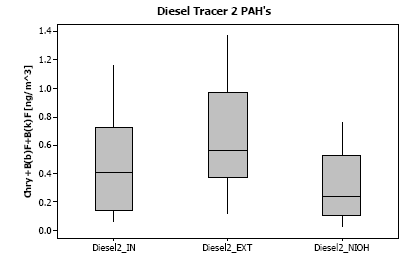
Figure 4.6: Box plot showing the upper and lower quartile of the indoor, outdoor and urban background concentrations of marker Diesel2 (Chry+B(b)F+B(k)F) measured during winter, spring and summer
2002.
The seasonal variation of the ΣlowPAH concentrations associated with the PM2.5 air-pollution is plotted in Figure 4.10 for all sites. There appear not to be a clear trend in these data except for a larger
variation during summer at all three sites.
4.4 Health effect evaluation based on the PAH measurements
The EU-EPA has recently enforced a yearly average target value of 1 ng B(a)P per m3 in PM10 starting in 2004 as proposed earlier this year . The average B(a)P concentrations for indoor, outdoor and
urban background found in this study (≤0.12 ng/m3) were well below that target value at all sites (see Table 4.1 to Table 4.3 and Figure 4.11).
The WorLD Health Organization (WHO) has established a unit risk value of 8.7 10-5 per ng/m3 B(a)P for life-time cancer risk . The WHO unit risk value suggests that ~10 cancer cases per 106 inhabitants
may occur at a lifetime exposure to the PAH-concentrations observed in the apartment. For comparison, 8 and 5 cases per 106 inhabitants are expected from the street and urban background
concentrations, respectively. The higher indoor B(a)P cancer risk reflects the potential influence of indoor sources on cancer risk. – Despite the apartment was uninhabited and the influence of indoor sources
was reduced to the inevitable minimum effects from neighboring apartments. However, because all PAHs contribute to carcinogenic risk, the indoor cancer risk indoors may be even higher than indicated by
the B(a)P unit risk value. This is mainly due to the relatively high
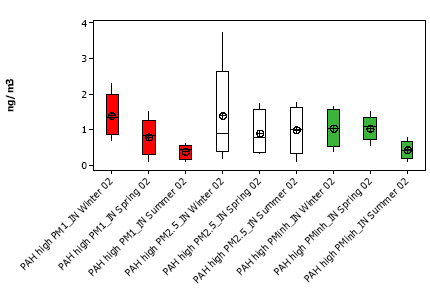
Figure 4.7: Seasonal variation of ΣhighPAH associated with indoor PM at Jagtvej
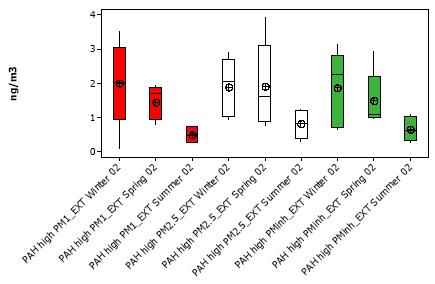
Figure 4.8: Seasonal variation of ΣhighPAH associated with outdoor PM at Jagtvej
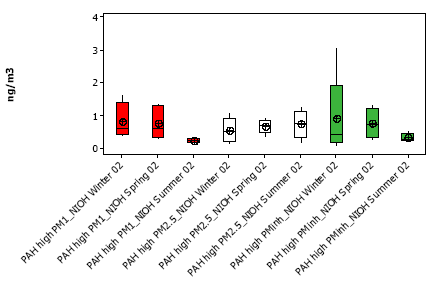
Figure 4.9: Seasonal variation of ΣhighPAH associated with PM in the urban background air
indoor concentration volatile PAHs, especially the relatively potent Flt (see Figure 4.2). Hence, in true environments, the cancer risk in indoor environments may be notably higher than estimated from both
street and urban background concentrations. However, it should be noted, that the study was conducted at 4th floor height. Street level PAH-exposures are expected to be higher still.
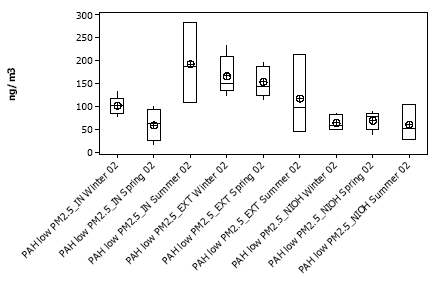
Figure 4.10: Seasonal variation of ΣlowPAH associated with PM2.5 in the indoor, outdoor, and urban background air.
Click here to see the Figure
Figure 4.11: Benzo[a]pyrene concentrations for each sampling week in the indoor, outdoor, and urban background air.
Compared to a previous B(a)P life-time cancer risk assessment conducted by Nielsen et al. (1995), this study found much lower cancer risk estimates of 5-10 cases per 106 Copenhagen inhabitants.
Nielsen et al. (1995) estimated 126 and 396 excess cancer cases per 106 Copenhagen inhabitants at a lifetime exposure to 1992-1994 background and street concentrations, respectively. The lower values
in the current study is a result of a dramatic decrease in PAH air-pollution since the early nineties.
The cancer risk estimate reported here is also relatively low as compared to the Relative Risk estimate (RR = 1.130.09) established for lung-cancer associated with PM2.5 by Pope et al. (2002). This on
one hand suggests that the WHO B(a)P unit risk alone cannot not explain the number of cancer incidents observed in epidemiological studies. Even bearing in mind, that sampling artefacts imply that the
actual concentrations may be a factor of two times higher. High concentrations of volatile PAHs and the presence of other carcinogenic compounds such as nitro-PAHs, benzene, inorganic compounds and
effects induced by particles alone also play an important role in the development of cancer. Similar conclusion was reached by e.g., Larsen and Larsen (1998). Naturally, these circumstances is not covered
by the B(a)P unit risk factor. On the other hand, it may be difficult to make direct comparison between current air-pollution levels and observed cancer incidents as cancer is the result of long-term exposure.
The current low PAH concentrations compared to the PAH concentrations a decade ago does therefore not reflect the current frequency of air pollution induced lung cancer.
5 Conclusions
PM in Copenhagen and in the urban background was dominated by fine particles. Approximately 70 wt% of PM2.5 consisted of PM1. The average content of PM2.5 in PMinh was 54 and 69 wt% at
Jagtvej and in the urban background, respectively, whereas PMinh in the uninhabited apartment consisted almost entirely of PM2.5.
There was a strong correlation (p = 0.000) between PM1, PM2.5 and PMinh in the street and city background. However, the difference between PM concentrations at Jagtvej and in the urban background
suggested that traffic contributed with 3.5±1.9 μg/m3, 5.0±2.7 μg/m3 and 14.6±4.0 μg/m3 to PM1, PM2.5 and PMinh, respectively.
The indoor PM correlated well with the outdoor PM concentrations at both Jagtvej and in the urban background (p < 0.004). However, the most reasonable I/O ratios were achieved using the
PM-concentrations measured in the street at Jagtvej.
The average indoor (15.20±5.04 μg/m3) and outdoor Jagtvej PM2.5 concentrations (19.80±7.66 μg/m3), exceeded the ASHRAE and US-EPA annual average air-quality guideline of 15 μg/m3, respectively. The
average PM2.5 concentration in the urban background (14.85±5.99 μg/m3) was at the limit of the US-EPA guideline. However, at the best, the outdoor concentrations just complied with the target values
proposed by the EU CAFE Working Group to be within 12 to 20 μg/m3.
Assessment of the adverse health effects induced by PM2.5 suggests 780±520 excess deaths occurred per million inhabitants in Copenhagen in 2002. Additionally, 1006±701 and approximately 550 excess
hospitalisations were predicted for cardiovascular disease and respiratory symptoms, respectively.
In the PM2.5 samples the total concentrations of the 16 US-EPA priority PAHs (ΣPAH) in the gas plus particle phases were 15-284 ng/m3 indoors, 46-235 ng/m3 outdoors, and 2-105 ng/m3 in the urban
background. The concentrations were underestimated due to extraction recovery below 100%, breakthrough, and reaction with ozone and nitrogenoxides during sampling. The real concentrations may be a
factor of two times higher than the observed concentrations.
Urban background, traffic at Jagtvej and indoor sources contributed to the PAH-concentrations in the uninhabited apartment. Traffic in the Jagtvej street canyon and indoor sources appeared to be the most
important sources for PAHs indoors.
The average B(a)P concentration exceeded the 0.0125 ng/m3 limit for 10-6 life-time cancer risk established by WHO at all sites. Based on the B(a)P unit risk value (8.7 10-5 per ng/m3) app. 10 cancer
cases per 106 inhabitants may occur at a life-time exposure to the PAH-concentrations observed in the apartment. For comparison, 8 and 5 cases per 106 inhabitants may be expected from the street and
urban background concentrations, respectively.
B(a)P may be inadequate for assessment of cancer risk from atmospheric air-pollution. Differences between e.g., indoor and outdoor PAH profiles and presence of other important carcinogenic compounds
may result in serious estimation errors. Future studies of cancer risks from air-pollution should include analysis of other carcinogenic compounds as well.
6 Reference list
Afshari A., Clausen P.A., Fogh C.L., Glasius M., Gunnarsen L., Jensen K.A.,
Jensen F.P., Kildesø J., Nielsen O.J., Sharma A.K. and Wåhlin P. (2004)
Indoor and outdoor particle measurements at Jagtvej in Copenhagen
(Denmark). (in preparation), (edited by Jensen,K.A. and Holm,G.)
Centre for Transport Research on the Environment, Health and Policy.
Copenhagen.
ASHRAE (2003) Ventilation for Acceptable Indoor Air Quality. ASHRAE
Addendum ad to ANSI/ASHRAE Standard 62-2001 edn, Atlanta, GA
30329: American Society of Heating, Refrigerating and Air-conditioning
Engineers, Inc.
Björklund E., Nilsson T. and Bøwadt S. (2000) Pressurised liquid extraction
of persistent organic pollutants in environmental analysis. trends in
analytical chemistry 19, 434-445.
Christensen S.L., Anglov J.T.B., Christensen J.M., Olsen E. and Poulsen
O.M. (1993) Application of a new AMIQAS computer program for
integrated quality control, method evaluation and proficiency testing.
Fresenius J Anal Chem 345, 343-350.
Clausen P.A., Bille R.L.L., Nilsson T., Hansen V., Svensmark B. and Bøwadt
S. (2003) Simultaneous extraction of di(2-ethylhexyl)phthalate and nonionic
surfactants from house dust. Concentrations in floor dust from 15
Danish schools. J.Chromatogr.A 986, 179-190.
Clausen P.A. and Wolkoff P. (1997) Evaluation of automatic thermal
desorption - capillary GC for determination of semivolatile organic
compounds (SVOCs) in indoor air. J.High Resol.Chromatogr. 20, 99-108.
Clean Air For Europe Working Group on PM (2004) Second Position Paper
on Particulate Matter - draft for discussion. In Second Position Paper, pp.
1-214. EU, Brussel.
Council of the Eropean Union (2004) Proposal for a Directive of the
European Parliament and the Council relating to arsenic, cadmium,
mercury, nickel and polycyclic aromatic hydrocarbons in ambient air and
deposition. 11645/03 ENV 414 CODEC 1023 - COM(2003) 423 final.
Glasius,M., Wåhlin,P. and Palmgren,F. (2004) Brændeovne forurener luften.
DMUNyt, 8(6).
Gundel L.A., Lee V.C., Mahanama K.R.R., Stevens R.K. and Daisey J.M.
(1995) Direct determination of the phase distributions of semi-volatile
polycyclic aromatic hydrocarbons using annular denuders. Atmos.Environ.
29, 1719-1733.
Hansen Å.M., Olsen I.L.B., Holst E. and Poulsen O.M. (1991) Validation of
a high-performance liquid chromatography/fluorescenes detection method
for the simultaneous quantification of fifteen polycyclic aromatic
hydrocarbons. Ann.Occup.Hyg. 35, 603-611.
Harrison R.M., Smith D.J.T. and Luhana L. (1996) Source apportionment
of atmospheric ploycyclic aromatic hydrocarbons collected from an urban
location in Birmingham, UK. Environ Sci Technol. 30, 825-832.
Jakobsen H.B., Norrelykke M.R., Christensen L.P. and Edelenbos M. (2003)
Comparison of methods used for pre-concentrating small volumes of
organic volatile solutions. J.Chromatogr.A 1003, 1-10.
58
Jensen K.A., Glasius M., Wåhlin P., Palmgren F., Olesen N.Ø. and Grundvig
S. (2003) Analytical electron microscopy and pixe analysis of the urban
airpollution in a high-traffic street, Copenhagen (Denmark), Journal of
Aerosol Science Abstracts of the European Aerosol Conference 2003, S487-
S488
Jensen K.A., Utsunomiya S., Glasius M., Wåhlin P., Palmgren F., Olesen
N.Ø. and Ewing R.C. (2004) Nano- and micro-mineralogy of airborne
trace metals in the city background and a high-traffic street in Copenhagen
(Denmark), GFF, Vol. 126/1, p. 70
Kawanaka Y., Matsumoto E., Sakamoto K., Wang N. and Yun S.J. (2004)
Size distributions of mutagenic compounds and mutagenicity in
atmospheric particulate matter collected with a low-pressure cascade
impactor. Atmospheric Environment 38, 2125-2132.
Künzli N., Kaiser R., Medina S., Studnicka M., Chanel O., Filliger P., Herry
M., Horak F., Jr., Puybonnieux-Texier V., Quenel P., Schneider J.,
Seethaler R., Vergnaud J.C. and Sommer H. (2000) Public-health impact
of outdoor and traffic-related air pollution: a European assessment. Lancet
356, 795-801.
Larsen, J.C. and Larsen, P.B. (1998) Chemical carcinogens. In R.E. Hester
and R.M. Harrison (Eds.) Air Pollution and Health. Issues in Environmental
Science and Technology 10, 33-56.
Li S.-N., Lundgren D.A. and Rovell-Rixx D. (2000) Evaluation of six
inhalabla aerosol samplers. American Industrial Hygiene Association Journal
61 506-216.
Metzger K.B., Tolbert P.E., Klein M., Peel J.L., Flanders W.D., Todd K.,
Mulholland J.A., Ryan P.B. and Frumkin H. (2004) Ambient air pollution
and cardiovascular emergency department visits. Epidemiology 15, 46-56.
Miguel A.H., De Andrade J.B. and Hering S.V. (1986) Desorptivity versus
chemical reactivity of polycyclic aromatic hydrocarbons (PAHs) in
atmospheric aerosols collected on quartz fiber filters.
Intern.J.Environ.Anal.Chem. 26, 265-278.
Nafstad P., Haheim L.L., Wisloff T., Gram F., Oftedal B., Holme I.,
Hjermann I. and Leren P. (2004) Urban air pollution and mortality in a
cohort of norwegian men. Environmental Health Perspectives 112, 610-615.
Naumova Y.Y., Offenberg J.H., Eisenreich S.J., Meng Q., Polidori A., Turpin
B.J., Weisel C.P., Morandi M.T., Colome S.D. and Stock T.H. (2003)
Gas/particle distribution of polycyclic aromatic hydrocarbons in coupled
outdoor/indoor atmospheres. Atmos.Environ. 37, 703-719.
Nielsen, T., Poulsen, M., Larsen, J.C., Jensen, A.B., Schramm, J. and
Tønnesen, J. (1995) Traffic PAH and other mutagens in air in Denmark.
In Miljøprojekt, nr. 285, pp. 1-140. Danish Environmetal Protection
Agency.
Palmgren F., Wåhlin P., Berkowitcz R., Hertel O., Solvang S.S., Loft S. and
Raaschou-Nielsen O. (2001) Partikelfiltre på tunge køretøjer i Danmark -
Luftkvalitets- og sundhedsvurdering. In Faglig Rapport, nr. 358, pp. 1-81.
Danmarks Miljøundersøgelser.
Pope C.A., III, Burnett R.T., Thun M.J., Calle E.E., Krewski D., Ito K. and
Thurston G.D. (2002) Lung cancer, cardiopulmonary mortality, and
long-term exposure to fine particulate air pollution. JAMA 287, 1132-
1141.
Schauer C., Niessner R. and Pöshl U. (2003) Polycyclic aromatic
hydrocarbons in urban air particulate matter: decadal and seasonal trends,
chemical degradation, and sampling artifacts. Environ Sci Technol. 37,
2861-2868.
59
Schneider T., Jensen K.A., Clausen P.A., Afshari A., Gunnarsen L., Wåhlin
P., Glasius M., Palmgren F., Nielsen O.J. and Fogh C.L. (2004)
Prediction of indoor concentration of 0.5-4 um particles of outdoor origin
in an uninhabited apartment. Atmospheric Environment 38 6349-6359.
Schwartz J., Ballester F., Saez M., Perez-Hoyos S., Bellido J., Cambra K.,
Arribas F., Canada A., Perez-Boillos M.J. and Sunyer J. (2001) The
concentration-response relation between air pollution and daily deaths.
Environmental Health Perspectives 109, 1001-1006.
Schwartz J., Laden F. and Zanobetti A. (2002) The concentration-response
relation between PM(2.5) and daily deaths. Environmental Health
Perspectives 110, 1025-1029.
Sharma,A.K. (2002) Trafikrelaterede partikler i indeklimaet. Roskilde
Universitetscenter. pp.1-102.
Swartz E., Stockburger L. and Gundel L.A. (2003) Recovery of semivolatile
organic compounds during sample preparation: Implications for
characterization of airborne particulate matter. Environ Sci Technol. 37,
597-605.
Wåhlin P., Palmgren F., Afshari A., Gunnarsen L., Nielsen O.J., Bilde M. and
Kildesø J. (2002) Indoor and outdoor particle measurements in a street
canyon in Copenhagen, Vol. 1, pp. 182-187.
World Health Organization (2000) Guidelines for Air Quality. pp. 1-190.
World Health Organization. Geneva.
Zielinska B., Arey J., Ramdahl T., Atkinson R. and Winer A.M. (1986)
Potential for artifact formation during Tenax sampling of polycyclic
aromatic hydrocarbons. J.Chromatogr. 363, 382-386.
Appendix A
Meteorological wind data
Meteorological wind data during the measurement campaigns retrieved from http://www.dmi.dk/dmi/index/viden/dmi-publikationer/tekniskerapporter.htm.
Table A.1: Wind direction presented as percent of time the wind was in the eight quadrants or the wind velocity was zero
Period |
Quiet |
N |
NE |
E |
SE |
S |
SW |
W |
NW |
07/01-14/01 '02 |
0 |
2 |
0 |
0 |
0 |
16 |
34 |
46 |
2 |
14/01-21/01 '02 |
0 |
0 |
0 |
0 |
0 |
36 |
50 |
14 |
0 |
21/01-28/01 '02 |
0 |
0 |
0 |
0 |
0 |
29 |
34 |
25 |
13 |
28/01-04/02 '02 |
0 |
0 |
0 |
0 |
0 |
34 |
30 |
34 |
2 |
04/02-11/02 '02 |
0 |
0 |
0 |
0 |
0 |
34 |
38 |
29 |
0 |
01/04-08/04 '02 |
0 |
11 |
4 |
34 |
20 |
23 |
4 |
4 |
2 |
08/04-15/04 '02 |
0 |
20 |
32 |
36 |
4 |
7 |
2 |
0 |
0 |
15/04-22/04 '02 |
2 |
16 |
31 |
25 |
5 |
11 |
4 |
4 |
2 |
22/04-29/04 '02 |
0 |
0 |
7 |
0 |
11 |
38 |
14 |
18 |
13 |
29/04-06/05 '02 |
0 |
36 |
11 |
2 |
11 |
20 |
13 |
5 |
2 |
20/05-27/05 '02 |
2 |
5 |
4 |
27 |
23 |
14 |
14 |
4 |
7 |
27/05-03/06 '02 |
0 |
29 |
14 |
4 |
5 |
5 |
9 |
29 |
5 |
03/06-10/06 '02 |
0 |
0 |
4 |
70 |
18 |
9 |
0 |
0 |
0 |
10/06-17/06 '02 |
0 |
0 |
0 |
0 |
5 |
20 |
18 |
52 |
5 |
17/06-24/06 '02 |
2 |
7 |
4 |
5 |
13 |
14 |
18 |
34 |
4 |
Average |
0.4 |
8.4 |
7.4 |
13.5 |
7.7 |
20.7 |
18.8 |
19.9 |
3.8 |
Table A.2: Wind velocity presented as percent of time the wind velocity was in the listed intervals.
| Beaufort |
Period |
0-1 |
2-3 |
4-5 |
>=6 |
>=8 |
>=10 |
07/01-14/01 '02 |
2 |
86 |
13 |
0 |
0 |
0 |
14/01-21/01 '02 |
0 |
32 |
64 |
4 |
0 |
0 |
21/01-28/01 '02 |
0 |
13 |
64 |
23 |
0 |
0 |
28/01-04/02 '02 |
0 |
23 |
55 |
20 |
2 |
0 |
04/02-11/02 '02 |
0 |
23 |
68 |
9 |
0 |
0 |
01/04-08/04 '02 |
5 |
64 |
30 |
0 |
0 |
0 |
08/04-15/04 '02 |
5 |
80 |
14 |
0 |
0 |
0 |
15/04-22/04 '02 |
20 |
65 |
15 |
0 |
0 |
0 |
22/04-29/04 '02 |
11 |
43 |
46 |
0 |
0 |
0 |
29/04-06/05 '02 |
5 |
40 |
55 |
0 |
0 |
0 |
20/05-27/05 '02 |
4 |
64 |
32 |
0 |
0 |
0 |
27/05-03/06 '02 |
4 |
77 |
20 |
0 |
0 |
0 |
03/06-10/06 '02 |
0 |
32 |
61 |
7 |
0 |
0 |
10/06-17/06 '02 |
4 |
39 |
57 |
0 |
0 |
0 |
17/06-24/06 '02 |
2 |
50 |
46 |
2 |
0 |
0 |
Average |
4.1 |
48.7 |
42.7 |
4.3 |
0.1 |
0.0 |
Appendix B
Description of collection methods and filter weighing procedure
PM measurements
PM1, PM2.5, PMinh and PAH's were collected on GFC glass fibre filters (Millipore AP 4003705) using stationary mounted personal samplers. PM1 and PM2.5 were collected using Triplex SCC 1.062
cyclones (BGI Inc.). Inhalable dust (PMinh) was collected using CIS's (Conical Inhalable Samplers). Figure B.1 show the performance of the Triplex cyclones at the three cut-points possible. Figure B.2
shows the convention for inhalable dust sampling. The inhalable dust fraction is defined as the "mass fraction of total airborne particulates, which may be inhaled through the nose and mouth". The inhalable
fraction is defined as:
EI = 50(1+exp[-0.06Da];
Where EI is the percentage of airborne particles and Da is the aerodynamic particle size in m. A study of the performance of the CIS sampler has shown that the collection efficiency of the CIS may deviate
from the sampling convention for particles coarser than ~ 10 m at wind velocities above 0.5 m/sec. Hence, the outdoor PMinh mass concentration in this study should be considered minimum values.
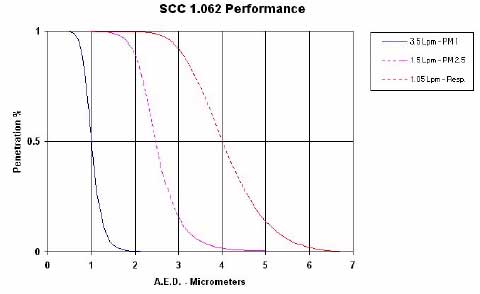
Figure B.1: Performance of the Triplex SCC 1.062 sampler set for collection of PM1, PM2.5 and respirable dust (www.bgiusa.com/ihi/triplex.htm)
The appropriate flows (1.5 L/min for PM1; 3.5 L/min for PM2.5 and PMinh) were achieved using single (1.5 L/min) or parallel-coupled (3.5 L/min) Dupont pumps. The only exception was the outdoor
measurement of inhalable dust at NIOH, where the CIS was connected to an AirCon2 High Volume Air Sampler (Struers, DK). The flow at the sampler inlets was checked at the beginning and at the end of
the sampling periods with random checks during the experiments. The volume of sampled air was calculated based on these measurements.
The PM samplers used for outdoor sampling were mounted next to each other appr. 10-15 cm apart at the top of a white plastic bucket turned upside-down to protect the samplers from direct sunlight and
rain. At Jagtvej, tubes were led through drilled and sealed holes in a wooden plate replacing one of the window sections in the living room window to the pumps, which were placed in the kitchen as
described in section 2.2.1.
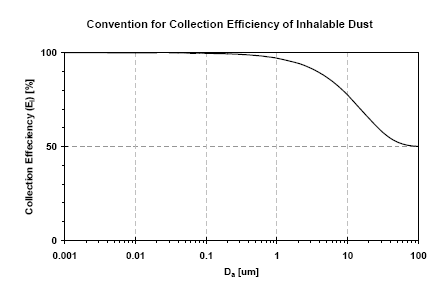
Figure B.2: Convention for the collection efficiency (EI) in sampling inhalable dust (PMinh). The CIS sampler comply with this CEN 1993 standard.
Filter cleaning and weighing procedure
Prior to weighing and sampling, the glass fibre filters were cleaned in an EMITECH K1050X plasma asher (Emitech Ltd. Kent, U.K.) operated at 85 W using 15 mL O2 per minute for 15 minutes at an
air-pressure of 6 10-1 mbar. This was completed to prevent potential contamination of PAH's on the filters for the subsequent HPLC analysis.
Filters were weighed using a Sartorius Micro Scale (Sartorius AG, Göttingen, Germany) after 24 hours of equilibration in a climate controlled weighing room (20°C; 50% RH). Each filter series included
three blind filters used as internal controls for passive mass changes and chemical analysis of sorbed volatiles. A 20 mg standard was used to establish accuracy and the weighing uncertainty (±0,003 mg)
based on 10 measurements before each weighing. Weight-loss during mounting and dismounting of glass fiber filters were corrected using empirical correction factors according to the type of sampler used
(see below). After weighing, the filters were stored in a freezer (–20°C) placed in individual glass petri dishes and wrapped in aluminum foil until extraction for chemical analysis of organic compounds.
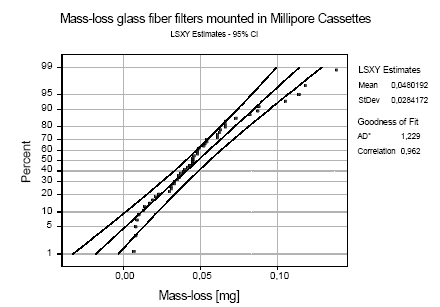
Figure B.3: Probability plot based on least squares estimates (LSXY) for mass-loss (in mg) from glasfiber filters during mounting and demounting in Millipore cassettes. AD*: Result from Anderson-Darling
normality test.
Mass-Loss Correction for Mass Concentration Measurements
Glass filters may be slightly damaged during mounting and dismounting in the filter cassettes. This is particularly true for glass fibre filters. Figure B.3 shows a probability plot for the mass-loss mounting and
demounting glass fibre filters in Millipore Cassettes. The average mass-loss was 48.019±28.42 g and there is less than 5% risk of a mass-loss larger than 90 μg at a 95% confidence interval. A similar test was
conducted for mounting and demounting glass-fibre filters in the CIS cassettes (Fig. B.4). The average mass-loss using CIS cassettes was 30.90±16.85 μg and there is less than 5% risk of a mass-loss larger
than 55 g at a 95% confidence interval. The measured particle concentrations were corrected by the appropriate average mass-loss values depending on sampling devise. The standard deviation of the
mass-loss data were included in the uncertainty for each mass concentration measurement according to the error propagation method: ΔF = √(Σxn2) for n = 1 – n.
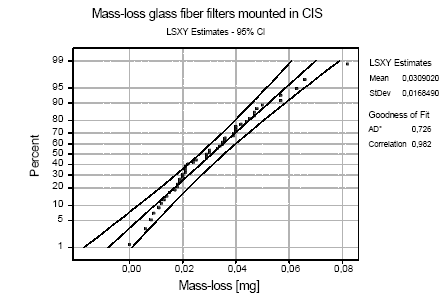
Figure B.4: Probability plot based on least squares estimates (LSXY) for mass-loss (in mg) from glasfiber filters during mounting and demounting in CIS cassettes. AD*: Result from Anderson-Darling
normality test.
Appendix C
List of PAH names, abbreviations, structures, etc.
* HPLC detectors: FD = Fluorescens Detector, DAD = Diode Array Detector
Name
Abbreviation
|
Structure |
Molecular
Weight
|
Boiling
Point [°C]
|
HPLC
Detector*
|
Detector wavelength |
CAS-nr |
|
|
|
|
|
EX(nm) |
EM(nm) |
|
Naphthalene
Naph
|
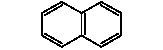 |
128 |
218 |
FD |
280 |
320 |
91-20-3 |
Acenaphthylene
Acy
|
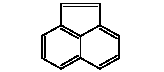 |
152 |
265-275 |
DAD
254nm
|
|
|
208-96-8 |
Acenaphthene
Ace
|
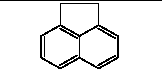 |
154 |
279 |
FD |
260 |
320 |
83-32-9 |
Fluorene
Flr
|
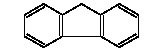 |
166 |
293-295 |
FD |
260 |
320 |
86-73-7 |
Phenanthrene
Phe
|
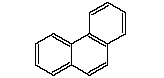 |
178 |
340 |
FD |
245 |
360 |
85-01-8 |
Anthracene
Ant
|
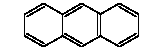 |
178 |
340 |
FD |
255 |
405 |
120-12-7 |
Fluoranthene
Flt
|
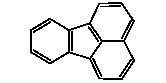 |
202 |
- |
DAD
254nm
|
|
|
206-44-0 |
Pyrene
Pyr
|
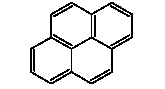 |
202 |
399 |
FD |
240 |
370 |
129-00-0 |
Benzo[a]anthracene
B(a)A
|
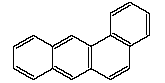 |
228 |
- |
FD |
265 |
400 |
56-55-3 |
Chrysene
Chry
|
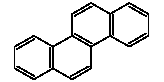 |
228 |
- |
FD. |
265 |
400 |
218-01-9 |
Benzo[b]fluoranthene
B(b)F
|
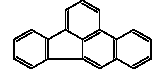 |
252 |
- |
FD |
290 |
450 |
205-99-2 |
Benzo[k]fluoranthene
B(k)F
|
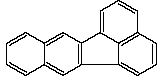 |
252 |
480 |
FD |
280 |
400 |
207-08-9 |
Benzo[a]pyrene
B(a)P
|
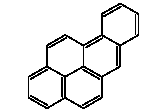 |
252 |
- |
FD |
280 |
400 |
50-32-8 |
Dibenzo[a,h]anthracene
DiB(a,h)A
|
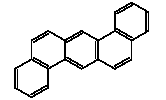 |
278 |
524 |
FD |
290 |
410 |
53-70-3 |
Benzo[ghi]perylene
B(ghi)Pe
|
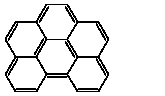 |
276 |
500 |
FD |
290 |
410 |
193-39-5 |
Indeno[1,2,3-cd]pyrene
I(1,2,3-cd)P
|
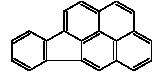 |
276 |
- |
DAD
254nm
|
|
|
191-24-2 |
Appendix D
Analysis of PAHs
Solvents and standards
Solvents
Acetonitrile and methanol was from Merck (pro analysi, >99.5%) and water from a Milli-Q water purification system (Millipore Waters). Acetone was from Rathburn (HPLC grade) or Merck (pro
analysis).
Standards
The PAH standard solution was from Supelco (610 Polynuclear Aromatic Hydrocabons Mix (610 PAH Mix)) and contained 16 PAHs naphthalene, acenaphthylene, acenaphthene, fluorene, phenanthrene,
anthracene, fluoranthene, pyrene, benzo[a]anthracene, chrysene, benzo[b]fluoranthene, benzo[k]fluoranthene, benz[a]pyrene, dibenzo[a,h]anthrancene, benzo[ghi]perylene, and indeno[1,2,3-cd]pyrene.
Certified Standard Reference Materials (SRMs) were from NIST (National Institute of Standards and Technology) and was Standard Reference Material 1647d, (in acetonitrile) and Standard Reference
Material 1649a, Urban Dust.
Preparation of bias control samples
For routinely evaluation of the bias of the HPLC analysis a batch of control samples were prepared from the SRM 1647d containing certified concentrations of the 16 PAHs. The PAH mixture was diluted
with acetonitrile to obtain the concentrations within the method range, transferred to micro vials containing ca. 200 μl and stored in a freezer until analysis. The resulting peak area count and the concentrations
of the selected compounds were plotted in an X-R control chart using the AMIQAS PC-Program .
Preparation of instrument control samples and calibration solutions
Retention time and stability of the response from the system and the detectors were monitored with instrument control samples produced by diluting 610 PAH Mix 125 times in acetonitrile. The resulting
peak area counts were plotted in an X-R chart. The standard solutions for the calibration curves (six points) were also made from 610 PAH Mix by different dilutions in actonitrile. The instrument controls
and the calibration solutions, respectively, were transferred to clean micro vials and stored in a freezer.
Samples for recovery experiments
Different studies were made to determine the recovery of the PLE extraction followed by HPLC or GC-MS analysis. All the recovery samples were prepared by loading filters or Tenax TA into clean PLE
extraction cells as described below. The samples were then spiked with 0.5 mL to 1 mL of a diluted 610 PAH Mix with a volumetric pipette or SRM 1649a dust weighed onto the filters in the cell.
Afterwards the cells were closed and extracted. A potion of the extracts was concentrated to ca. 400 μl by gentle evaporation in a stream of charcoal filtered N2. The concentrated and non-concentrated
extracts were stored in a refrigerator until analysis.
Sample preparation
Glass fibre filter cleaning
The glass fibre filters were cleaned in an EMITECH K1050X plasma asher (Emitech Ltd. Kent, U.K.) operated at 85 W using 15 mL O2 per minute for 15 minutes at an air-pressure of 6 10-1 mbar.
Tenax Ta tube cleaning
The sample tubes contained 40 mg 35-60 mesh Tenax TA (Chrompack) and the backup tubes 20 mg Tenax TA that was kept in place by small steel grids. The tubes were cleaned in a stream of ca. 60 ml
nitrogen per min at 275 °C for at least 2 h. The tubes were checked for contaminations by thermal desorption and GC with FID detection (TD-GC-FID).
Glassware cleaning
Glassware was washed by the normal procedure in the central dishwasher and afterwards cleaned by Oxygen Plasma Ashing for 60 minutes at 100 W and stored in cleaned aluminium-foil (rinsed with
acetone). After use, the glassware was placed in a saturated solution of KMnO4 overnight and afterwards placed in a saturated oxalic acid solution for a minimum of 4 hours. Subsequently the glassware
was rinsed in water and returned to central dishwasher. Before use, disposable glassware (micro vials) was cleaned by Oxygen Plasma Ashing for 60 minutes at 100 W and stored in cleaned aluminium-foil.
PLE cell cleaning
The PLE extraction cells were cleaned by sonication of the body and end caps in acetone for at least 5 minutes. Then the cells were assembled and filter papers (supplied by Dionex) were placed in the
bottom to prevent clogging of the metal frit. Ottawa sand (Fisher S23-3) was filled into the cells to reduce the amount of solvent. Subsequently a second filter was placed on the top of the sand before the
cells were closed. The cells, filters and sand were cleaned by a treatment identical to the one used for the samples by running the PLE extraction method.
Pressurized Liquid Extraction (PLE) of filters and Tenax TA
PLE extractions were carried out using an automated ASE 200 Accelerated Solvent Extractor (ASE) (Dionex, Sunnyvale, CA, USA) equipped with 1-mL or 5-mL stainless steel extraction cells as
previously described for phthalates in house dust . After cleaning of the extraction cells the top filter and the Ottawa sand was removed. Then the samples (glass fibre filter or Tenax TA) were carefully
loaded into the cells and the Ottawa sand and the top filter replaced. The glass fibre filters and the Tenax TA samples were treated in similar ways. The glass fibre filter samples were placed in 5-mL cells and
the Tenax TA samples were placed in 1-mL cells to avoid a large extraction volume. The filter samples were extracted two times consecutively and the Tenax TA samples extracted once. The extraction
procedure started with a 7-min thermal equilibration time during which the cells were heated to 150°C, filled with acetonitrile and pressurized to 2000 psi. The extraction was continued under static
conditions for 10 min. After the static extraction the samples were flushed with ca. 2.8 ml fresh acetonitrile. Finally, the cells were purged with gaseous nitrogen for 60 s at 150 psi.
After the extraction the samples were stored in brown vials protected from light, and refrigerated prior to the solvent volume reduction of only the filter samples. The filter samples were concentrated from ca.
5 mL to ca. 0.3 mL by evaporation in a gentle stream of nitrogen. The Tenax TA extracts were not concentrated in order to avoid evaporation losses of low boiling PAHs . The exact amount of the
extraction solvent was found by weighing of the vials before and after the concentration by evaporation. All the extracts were transferred to micro vials containing ca. 200 μl and stored in freezer until analysis
by HPLC. Beside the filter and Tenax TA blanks, also PLE system blanks were extracted. The PLE system blanks were cells only filled with Ottawa sand and filter papers.
High Performance Liquid Chromatography (HPLC) analysis of extracts
The PAHs were separated by reversed-phase HPLC using the following equipment : Pumps model 625 LC systems, a Waters 717 autosampler, a model 600E system controller and a SAT/IN data module
(all from Waters Associates Inc., Milford U.S.A). The column (250x 4.6 mm inner diameter) was a Supelcosil LC-PAH column (5μm packing) protected with a Waters Guard column Bondapak
C18/Corasil (37-50μm) and a pre-column Lichrocart 4-4, Lichrospher 100 RP-18, 5μm. Two different detectors were used: a Waters model 996 Photodiode Array Detector (DAD) (254nm); and a
Perkin-Elmer fluorescence detector model LS-5 (FD) (Perkin-Elmer Corp.,Noewalk, U.S.A.). The mobile phase was acetonitrile-water (40:60) held for 5 min, then increasing linearly to 90% acetoniltril
during 20 min, and held for 15 min. The flow rate was 1.0 ml/min and the injection volume was 25 μl. All injections and runs were performed at room temperature (20-22°C). The DAD was used for
quantification of acenaphtylene, fluoranthene and indeno[1,2,3-cd]pyrene and the FD for quantification of the other 13 PAHs using multiple wavelength shifts (see Appendix C).
Analysis sequence
The construction of the sequence was as follows: 6 calibrations standards, 4-6 bias control samples, 1 instrument control, 10-25 samples including blanks (filters/Tenax Ta tubes) and system blanks. Only
one injection per sample was used.
Calibration
Six points calibration curves were made for each analysis series. No calibration curves had intercepts significant different from zero and r² was between 0.9 and 0.999.
Blanks
From every one-week fieLD sampling 2-3 blank filters and one Tenax Ta blank was included. The blank samples had generally a low content of PAHs (see Appendix E). In most of the filter blanks no peaks
or only small peaks (under the detection limits) could be detected. In few cases larger amounts on filters was measured. This may be attributed to contaminations from handling or insufficient cleaning. Also
the Tenax TA blank tubes had generally low content of PAHs, but for acenapthylene 6 out of 14 blank tubes had amounts at or above the limit of detection. The explanation is probably interference in the
non-specific PDA detector, see more details in the section "Data treatment".
Gas chromatography combined with mass spectrometry (GC-MS)
GC-MS (Perkin Elmer Autosystem XL / TurboMass) was used for the recovery study and for verification of some of the HPLC result. The GC-MS had a constant flow of He (carrier gas) of
about 1 ml min-1 and was equipped with 30-m x 0.25 mm-i.d. Chrompack CP Sil 8 CB Low Bleed/MS (0.25 μm film thickness) column. The temperature program was 60 °C, held for 2 min, increased to
320 °C at 10 °C min-1 and held for 2 min. The splitless injector temperature was 250 °C (standard liner). The MS transfer line was 275 °C. The mass spectrometer was operated in the electron impact
ionization mode (EI+, 70 eV) with a source temperature of 175 °C using full scan mode (m/z 50 – 300) and single ion monitoring (SIM). No internal standards were used and the molecular ions were used
for SIM and quantification (see Appendix C).
Validation of the method
Recovery
The PLE extraction recovery of PAHs from filters and Tenax TA was studied. The samples was prepared and analysed with GC-MS or HPLC as described above. For the filters spiked with the SRM
1649a dust 10 out of 13 PAHs had recoveries better than 80%±20% (see Figure D 1). Fluorenthene had a mean recovery at 142%±23%, but two out of the six samples gave recoveries within 100±10%.
Benzo[a]pyrene and dibenzo[a,h]anthracene had a large variation with mean recoveries lower than 70%. However, for both compounds the confidence limits included 100% recovery.
For the Tenax TA tubes spiked with 610 PAH Mix and no concentration of the extracts, 11 out of 16 compounds had recoveries better than 75±25% (see Figure D 2). The other 5 PAHs were mostly high
boiling compounds and had recoveries better than 60%. For the Tenax TA tubes spiked with 610 PAH Mix and evaporation of the extracts to nearly dryness, the lowest boiling compounds from naphthalen
to pyrene had recoveries lower than 50%.
Limit of detection (LD)
The LD of HPLC-DAD/FD was estimated as three times the standard deviation of 18 low standards. The LDs varied between 0.002 to 0.028 ng/μl depending on the PAH (see Table B1).
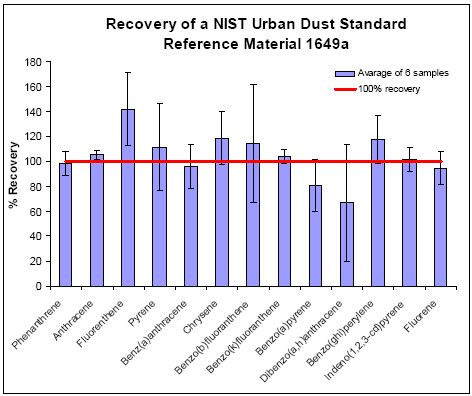
Figure D 1: Recovery of PAHs from the filters
Table D 1: Validation and quality assurance parameters.
PAH |
Limit of detektion (LD) |
Break through |
Filter blanks |
Tenax TA blanks |
Accuracy |
|
ng/μl |
% |
SD |
% n.d. |
% below or equally LD |
% above LD |
% n.d. |
% below or equally LD |
% above LD |
% |
Naph |
0,018 |
15 |
18 |
36 |
19 |
44 |
75 |
8 |
17 |
3,0 |
Acy |
0,028 |
n.a. |
|
72 |
14 |
14 |
33 |
50 |
17 |
7,2 |
Ace |
0,013 |
7 |
20 |
61 |
33 |
6 |
92 |
8 |
0 |
2,2 |
Flr |
0,003 |
11 |
26 |
72 |
28 |
0 |
75 |
17 |
8 |
3,9 |
Phe |
0,005 |
14 |
26 |
39 |
58 |
3 |
92 |
8 |
0 |
5,3 |
Ant |
0,002 |
1 |
8 |
67 |
19 |
14 |
92 |
8 |
0 |
9,6 |
Flt |
0,004 |
6 |
18 |
72 |
0 |
28 |
92 |
0 |
8 |
8,3 |
Pyr |
0,003 |
2 |
10 |
28 |
47 |
25 |
75 |
25 |
0 |
5,1 |
B(a)A |
0,003 |
n.a. |
|
69 |
25 |
6 |
92 |
8 |
0 |
1,6 |
Chry |
0,002 |
n.a. |
|
50 |
25 |
42 |
75 |
17 |
8 |
1,2 |
B(b)F |
0,009 |
n.a. |
|
28 |
53 |
19 |
83 |
17 |
0 |
1,1 |
B(k)F |
0,002 |
n.a. |
|
36 |
33 |
31 |
75 |
25 |
0 |
1,1 |
B(a)P |
0,005 |
n.a. |
|
44 |
50 |
6 |
75 |
25 |
0 |
5,6 |
DiB(a,h)A |
0,005 |
n.a. |
|
33 |
47 |
19 |
50 |
33 |
17 |
14 |
B(ghi)Pe |
0,003 |
n.a. |
|
33 |
42 |
33 |
50 |
33 |
17 |
0,1 |
I(1,2,3-cd)P |
0,004 |
n.a. |
|
100 |
0 |
0 |
0 |
100 |
0 |
7,5 |
n.a..= not available
Accuracy = the difference between the "true" and the measured PAH concentration divided with the "true" PAH value in the standard reference solution based on SRM 1647d.
Evaluation of breakthrough
The breakthrough for the PM2.5 Tenax TA tubes shown in Table B1 was calculated as the PAH amount on the backup tube divided by the amount on the sample tube and the backup tube in total. Break
through could not be calculated for high boiling PAHs due to interference. However, the breakthrough is probably insignificant (see discussion in section 4.1.1).
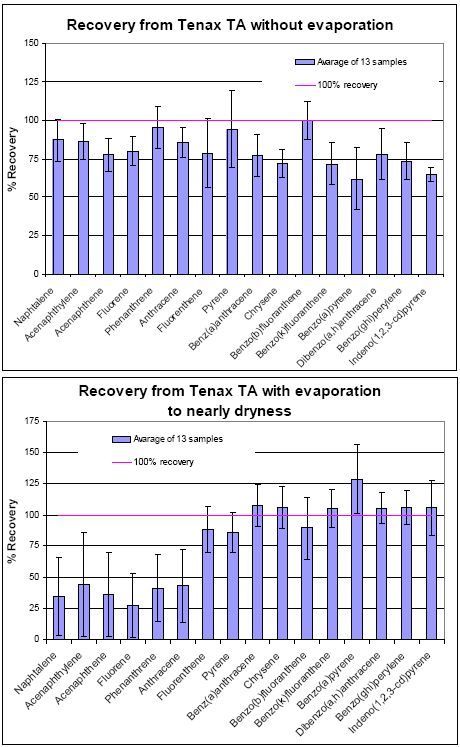
Figure D 2: Recovery af PAHs from the Tenax TA with and without concentration of the extract by evaporation
Evaluation of the blanks
The filter and Tenax TA blanks were evaluated as the fractions of all blanks that were n.d., below or equal to LD, and above LD (see Table B1).
Evaluation of the accuracy
The accuracy of the analysis was estimated from analysis of the bias control samples (se Table B1).
Quality assurance (QA)
X-R Control charts were established and were the basic tools to test the statistical control of the analytical method. They were used to document that the random and the systematic errors of the method
stayed within acceptable limits during the period of the analyses. These limits were estimated from the standard deviation x numbers of analysis of the control samples. The control charts were generated with
the statistical QA program AMIQAS .
To follow the stability of the response. five X-R control charts were set up. The peak area counts from the instrument control samples in each run were plotted in the charts. For the DAD detector the area
counts of acenaptylene and fluorenthene were plotted and for the FD detector the area counts of napthalene. pyrene and phenanthrene were plotted.
To evaluate the precision and statistical control of the analysis method. X-R control charts for five PAHs were established. In each sequence 4-6 bias control samples were run and from the peak area
counts the concentrations of the samples were calculated using the calibration data. X-R charts were made for the DAD and the FD detector for the following PAHs: naphthalene. acenaptylene.
phenanthrene. fluoranthene. and pyrene.
The results of the control samples were > 3 standard deviations (STDs) from the mean values in the following cases:
Area counts control charts (instrument control samples)
detector |
Acenaptylene |
Fluorenthene |
Pyrene |
comments |
PDA |
040204
040301
|
030507
040204
040301
|
|
|
LS-5 |
|
|
030527
040204*
|
*New lamp (respond high) |
The response of the detectors will decrease with the age of the lamps. The start and the end of a lamps lifetime will often provoke a rejection.
Concentration control charts (bias control samples)
detector |
Naphthalene |
Fluorenthene |
Phenanthrene |
comments |
PDA |
|
040109
040204
|
|
|
LS-5 |
030829**
040109
|
|
031030
040128
|
**Repair of autosampler |
When a control sample > 3 STDs from the mean value it indicates that the run was not in statistical control at the shown dates. The samples analysed in the sequence with the rejected control component.
was not automatically rejected since ca. 0.5% of the bias controls will statistically be out of range. A summery of all the controls and all the control component analysed on the same day indicates whether the
analyses was out of statistical control or not. In these cases it was found that the analysis method was in statistical control.
Data treatment
A spreadsheet (Excel) was used for most data treatment, calculation and plots. For some statistical tests and plots MiniTab was used. In the raw data table (Appendix E) concentrations within the calibration
limits are in normal font, concentrations below the instrumental LD are reported in italics, and when no HPLC peak in the chromatogram was found the results are reported as n.d. (not detected). In the
calculations all data (including data below LD) was used except data reported as n.d. that was treated as missing values (empty section/cell). Acenaphtylene concentrations measured by the non-specific
DAD detector were very high in all Tenax samples compared to the other PAHs. Therefore all the Tenax TA samples were analysed (semi quantitative) with GC/MS to check the acnaphtylene response. It
appeared that there was an interfering compound in the HPLC system, since acenaphtylene could not be detected with GC-MS. The lowest amounts that could be detected on the GC-MS were ca. 0.3
ng/0.5μl. Because of the much higher detection level on the GC-MS, quantification on Tenax tubes could not be performed. If an acenaptylene peak for Tenax TA extracts was reported by HPLC-DAD but
not by GC-MS, it was set equal to the detection limit of HPLC method and used as such in the calculations. When no acenaphtylene peak in the HPLC chromatogram of Tenax TA extracts was found the
results are reported as n.d. The filter concentrations of acenaphtylene were treated as the other PAHs.
Appendix E
HPLC raw data tables – PAH concentrations in extracts from filters. Tenax TA sample and backup tubes and blanks
The tables show the concentrations in the extracts (ng/μl) measured by the method described in Appendix D. Concentrations within the calibration limits are in normal font, concentrations below the
instrumental detection limits (LDs) are reported in italics, and when no HPLC peak in the chromatogram was found the results are reported as n.d. (not detected). Row 1: Sampling period and instrumental
LDs. Row 2. from right: Abbreviations of PAH names (see Appendix C) and other terms described in the following. Column 1 (left. external): F = filter. T = Tenax TA sample tube or backup tube (2nd
T of 2 consecutive Ts). Column 2: Sampled particle fraction. Column 3: Sample IDs. IN = PMxxxJagtvej_IN. EXT = PMxxxJagtvej_EXT. Background = PMxxxNIOH_EXT. Column 4: Sampled air
volumes. Column 5: Volume of extracts.
Click here to see the Tables
Appendix F
Tables of measured concentrations of PAHs in indoor, outdoor and urban background air
Indoor, outdoor and urban background concentrations (ng/m3) of PAHs collected on PM1-filters, (gas + particle) concentrations (ng/m3) of PAHs collected on PM2.5-filters, and Tenax TA sample and
backup tubes, and PAHs collected on PMinh-filters. The measurements termed "n.d." in Appendix E has been set equal to zero in Tables F 1-F 3 and used for calculation of the avaerage values. The
outdoor PM2.5 measurement of B(ghi)Pe 17/4-24/4 is considered an outlier and has been omitted from the calculations.
Table F 1: Indoor, outdoor and urban background concentrations (ng/m3) of PAHs collected on PM1-filters.
Click here to see the Table
Table F 2: Indoor, outdoor and urban background (gas + particle) concentrations (ng/m3) of PAHs collected on PM2.5-filters and Tenax TA sample and backup tubes.
Click here to see the Table
Table F 3: Indoor, outdoor and urban background concentrations (ng/m3) of PAHs collected on PMinh-filters.
Click here to see the Table
| Top | | Front page |
Version 1.0 May 2005, © Danish Environmental Protection Agency
|