| Bottom | | Front page |
Environmental Project no. 995, 2005
Update on Impact Categories, Normalisation and Weighting in LCA
- Selected EDIP97-data
Contents
Preface
Authors preface
Summary and conclusions
Sammenfatning og konklusioner
1 Introduction to the project
2 Selection of impact categories
3 Development of normalisation references for different geographic areas
4 Global warming
5 Stratospheric ozone depletion
6 Photochemical ozone formation
7 Acidification
8 Nutrient enrichment
9 Human toxicity
10 Ecotoxicity
11 Calculation of weighting factors
Preface
This report was prepared within the Danish LCA methodology and consensus creation project during the period from 1997 to 2003.
The report is one out of five technical reports to be published by the Danish Environmental Protection Agency and dealing with key issues in LCA. The reports were prepared as background literature for a
number of guidelines on LCA, planned to be published by the Danish Environmental Protection Agency during the autumn of 2004 and spring 2005. The reports present the scientific discussions and
documentation for recommendations offered by the guidelines. The reports and guidelines developed within the project are presented in the overview figure below.
A primary objective of the guidelines has been to provide advice and recommendations on key issues in LCA at a more detailed level than offered by general literature, like the ISO-standards, the EDIP
reports, the Nordic LCA project and SETAC publications. The guidelines must be regarded as a supplement to and not a substitution for this general literature.
It is, however, important to note that the guidelines were developed during a consensus process involving in reality all major research institutions and consulting firms engaged in the LCA field in Denmark.
The advice given in the guidelines may thus be considered to represent what is generally accepted as best practice today in the field of LCA in Denmark.
The development of the guidelines and the technical reports was initiated and supervised by the Danish EPA Ad Hoc Committee on LCA Methodology Issues 1997-2001. The research institutions and
consulting firms engaged in the development and consensus process are:
COWI, Consulting Engineers and Planners (Project Management)
Institute for Product Development, the Technical University of Denmark
FORCE Technology
The Danish Technological Institute
Carl Bro
The Danish Building Research Institute
DHI - Water and Environment
Danish Toxicology Institute
Rambøll
ECONET
National Environmental Research Institute
Guidelines and technical reports prepared within the Danish LCA-methodology and consensusproject
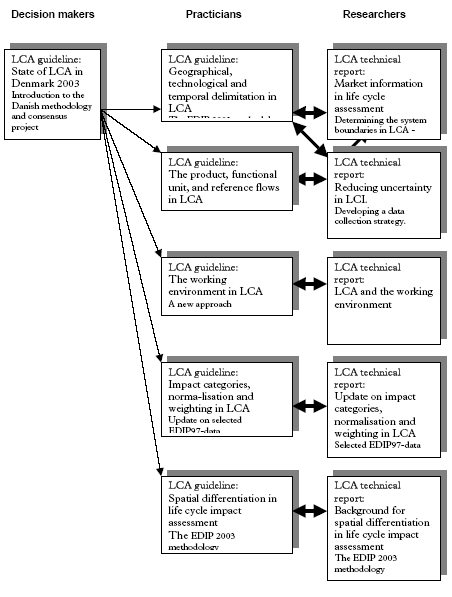
Authors preface
This technical report is a result of a LCA methodology and consensus-making project focusing on normalisation and weighing. The project has involved a large part of the LCA practitioners and interested
parties in Denmark. The technical report documents normalisation references with the reference year 1994 and weighting factors with 2004 as the target year. Along with the technical report a guideline
("LCA Guideline: Impact categories, normalisation and weighting in LCA") has been developed.
The project has been organised as a true consensus-process. First, the project leader in dialogue with the involved consultants and researchers discussed and agreed upon a general data collection process,
the idea being to receive consistent data from a wide range of national and international information sources. However, this approach appeared to be less effective than hoped for, and it was therefore
decided to proceed in a less uniform way within different impact categories in order to obtain the requested/necessary data and use them in development of normalisation references. The choices made were
presented at two workshops, each providing valuable input to the project group as well as securing a consensus among all interested parties.
The following persons have been responsible for the individual chapters:
Chapter 2+3: Heidi K. Stranddorf and Leif Hoffmann, dk-TEKNIK ENERGY & ENVIRONMENT
Chapter 4: Global warming: M.Sc (Chem. Eng) Leif Hoffmann, dk-TEKNIK ENERGY & ENVIRONMENT
Chapter 5: Ozone depletion: M.Sc (Chem. Eng) Leif Hoffmann, dk-TEKNIK ENERGY & ENVIRONMENT
Chapter 6: Photochemical ozone formation: B.Sc (Chem. Eng) Karsten Fuglsang, dk-TEKNIK ENERGY & ENVIRONMENT
Chapter 7: Acidification: M.Sc (Chem. Eng) Leif Hoffmann, dk-TEKNIK ENERGY & ENVIRONMENT
Chapter 8: Nutrient enrichment: Ph.D. (Biology) Jørgen Larsen, Danish Technological Institute
Chapter 9: Ecotoxicity: Ph.D. (Ecotoxicology) Jens Tørsløv, DHI Water and Environment
Chapter 10: Human toxicity: M.Sc (Eng) Frans Møller Christensen, Danish Toxicology Centre & M.Sc (Chem. Eng) Leif Hoffmann, dk-TEKNIK ENERGY & ENVIRONMENT
Chapter 11: Weighting: M.Sc (Eng) Niels Juul Busch, Rambøll
The main part of the data collection and calculations have been performed in the period 1998-2001, with small adjustments and re-calculations being made in 2003. Anders Schmidt, FORCE
Technology/dk-TEKNIK ENERGY & ENVIRONMENT was responsible for the final editing of the report in autumn, 2003. A special thank is given to Michael Hauschild, Danish Technical University, for
his guidance and reviews of the efforts in the project.
Summary and conclusions
The report addresses a number of elements that all are related to the impact assessment step in the EDIP methodology:
- It gives a short introduction to some of the key elements in EDIP, e.g. the person equivalent, and the normalisation and weighting steps
- It gives a short discussion regarding choice of impact categories in relation to the recommendations in the ISO 14040 standard series, the international consensus regarding the applicability of different
methods and the possibilities of performing calculations in the LCV programme that has been made especially in order to make EDIP calculations
- It describes a number of procedures that makes it possible to establish normalisation factors for the EU-15 and the World through extrapolation from relatively limited data sets
- It gives a detailed insight into the data base that has been used in the updating of the existing normalisation and weighting factors and to establish new factors for EU-15 and globally.
Regarding the choice of impact categories the report gives an overview of the categories that have been addressed in central LCA documents and method papers. A number of impact categories and the
methods used for their assessment is common to all methods, while methods suggested for assessment of impacts on the local scale like human toxicity, ecotoxicity, noise, land use, etc. show marked
differences in their approach. The report does not give a final recommendation whether a specific impact category shall be included in the LCA or whether one or the other approach is better. The
recommendation is to include a sufficiently wide range of impact categories to fulfil the goal and scope of the LCA. Omission of impact categories should be a conscious and motivated choice, for which the
lack of quantifiability for a given category is not necessarily a valid excuse. There are no requirements in the general LCA concept that all results should be quantified, and qualitative assessments can
therefore be used to describe areas and issues that would otherwise be neglected. In some cases it will be relevant to develop and apply new methods in order to assess other impact categories than those
traditionally addressed in LCA. This can be very demanding in terms of resources, and the final decision to do so should be determined by the commissioner and the LCA practician.
Another aim of the subproject was to develop new normalisation references for the current 15 member states of the EU (EU-15) and at the Global level – a "World Proxy". The background for this aim is
that in the original EDIP method, normalisation references have only been developed for "Denmark" which can make it difficult to interpret the results of a LCA with a broader geographic scope. With the
calculation of additional normalisation references, primarily for the EU-15, the LCA practitioner therefore gets better possibilities to assess the relative importance of different impact categories in an
international perspective.
For the EU-15 the new normalisation references for photochemical ozone formation, acidification, nutrient enrichment, human toxicity and ecotoxicity were calculated by using different approaches and
information from a broad range of literature sources. For some combinations of impact categories/compounds/activities, e.g. regarding many of the contributions to human toxicity and ecotoxicity, the
normalisation references were calculated by extrapolation from selected national inventories as no consistent database was available. The extrapolation was based on the assumption that there is a linear
relationship between the economic activity in a given EU-country and its emissions of a given substance or group of substances. Where possible, a cross reference was made with the few available data in
order to give an indication of the uncertainty introduced by extrapolation.
The extrapolation approach used for calculation of global normalisation references was to use the relation between the economic activity in a country/region (measured as the Gross Domestic Product, GDP)
and the emission of substances contributing to acidification. Both parameters have a well-founded statistical base for many countries and the correlation between them was considered to be adequate for
further calculations. Based on the relationship a general extrapolation was developed, simply scaling the European normalisation reference to the global level, the so-called World Proxy.
The report describes the uncertainties associated with the newly developed normalisation references and recommends that the European normalisation references be used in general to calculate the base case
scenarios. The recommendations are described in more detail in the Guidelines from the project, but are primarily based on the assumption that most LCA's include impact potentials that are found on a
broad geographical scale and therefore should be matched by relevant normalisation factors. A final recommendation, however, is to use Danish normalisations references and the World Proxies in a
sensitivity analysis in order to get a better indication of the robustness of the results.
The report gives a detailed insight into the data sources and calculation procedures that have been used in the calculation of each normalisation reference. For each impact category the database is presented
in tabular form along with the most important intermediate calculations. This content is probably most interesting for experts in different areas, giving the possibility for other types of calculation, but is of
course also a good starting point for future updates.
The last chapter in the report presents new weighting factors for Denmark, EU-15 and the World. This chapter also provides a detailed insight into the basic data, the way the weighting factors have been
calculated, and the associated uncertainties. It is emphasized that the Global weighting factors for impact categories on the regional scale can only be calculated with a relatively high uncertainty, and for the
impact categories on the local scale it has not been possible to establish reliable weighting factors. In order to make such calculations, information on current emissions as well as reduction targets must be
available for each country/region. It was far beyond the scope of the project to collect and manipulate this very large amount of information, and it is therefore recommended to use the weighting factors for
EU-15 in the base case calculations. It is, however, mentioned that especially the weighting factors for local impacts tend to be underestimated, compared to those for the regional impacts. The reason for
this is that even for the EU-15 is it difficult to establish the full overview of the single member states' reduction targets for emissions of single substances or groups of substances.
It is concluded that the results of the project increases the possibility of making a LCA with a broader perspective than the original EDIP method allows. The broader perspective is, however, achieved at the
cost of an increased uncertainty and the recommendations of the report and and the associated Guidelines are therefore focused on the use of sensitivity analysis to the extent relevant to fulfil the goal and
scope of the study.
Sammenfatning og konklusioner
Rapporten indeholder en række elementer der alle har relation til vurdering af miljøbelastninger i UMIP-metoden:
- Den giver en kortfattet introduktion til visse nøglebegreber i UMIP såsom personækvivalenter, normalisering og vægtning
- Den indeholder en kortfattet diskussion omkring valg af belastningskategorier, set i relation til anbefalingerne i ISO's standarder om livscyklusvurdering, den internationale konsensus om de enkelte
metoders anvendelighed samt mulighederne for at foretage beregninger i et EDB- værktøjet LCV, der er specielt udviklet til at håndtere UMIP-metoden
- Den beskriver en række procedurer, der gør det muligt at udarbejde normaliseringsfaktorer for EU-15 og Verden gennem ekstrapolation fra forholdsvis begrænsede datamængder
- Den giver et detaljeret indblik i det datagrundlag, der er anvendt for at opdatere UMIP's eksisterende vægtningsfaktorer og udarbejde nye vægtningsfaktorer for EU-15 og på globalt plan.
Omkring valg af belastningskategorier giver rapporten en oversigt over de belastningstyper, som er nævnt/behandlet i en række centrale LCA-dokumenter og metodebeskrivelser. Der er naturligvis en del
kategorier og metoder, der er fælles for alle metoder, men omkring vurdering af forskellige former for lokale belastninger som human toksicitet, økotoksicitet, brug af arealer, støj m.v. er der foreslået
metoder, der har betydelige forskelle. Rapporten tager ikke stilling til, om en bestemt kategori skal medtages i en LCA eller om den ene eller den anden fremgangsmåde er den bedste. Anbefalingen er, at
man inkluderer en så bred vifte af belastningstyper, at formålet med LCA'en kan opfyldes. Hvis man udelader visse belastningstyper, skal dette være et bevidst og begrundet valg, hvor manglende
kvantificerbarhed ikke uden videre bør accepteres som begrundelse. Der er i det overordnede LCA-koncept ikke krav om kvantificering, og kvalitative vurderinger kan derfor meget vel anvendes til at
beskrive vidensmæssige huller. Endelig peger rapporten på, at det i nogle tilfælde kan være relevant at udvikle og anvende metoder til vurdering af andre belastningskategorier end de traditionelle. Her skal
man dog være opmærksom på, at dette ofte er tidskrævende/dyrt, men den endelige beslutning om at inkludere en eller flere nye belastningskategorier bør afgøres i samråd mellem opgavestilleren og
LCA-praktikeren.
Et andet formål med delprojektet var at udvikle normaliseringsfaktorer for de 15 nuværende EU-lande og – hvor muligt og relevant – også på globalt niveau. I den originale UMIP-metode er der "kun"
udarbejdet normaliseringsreferencer for Danmark, hvilket på nogle områder kan give vanskeligheder med at tolke resultaterne. Ved at udarbejde nye normaliseringsreferencer, først og fremmest for EU-15,
får LCA-praktikeren og opgavestilleren bedre muligheder for at vurdere betydningen af de enkelte belastninger i et internationalt perspektiv.
For EU-15 blev de nye normaliseringsreferencer for fotokemisk ozondannelse, forsuring, næringssaltbelastning og human- og økotoksicitet beregnet på forskellige måde ved hjælp af tilgængelige data fra en
bred vifte af litteraturkilder. For visse stoffer/aktiviteters bidrag til belastninger som human- og økotoksicitet er der dog ekstrapoleret fra enkeltstående nationale opgørelser, idet der ikke findes et konsistent
datagrundlag. Ekstrapolationen er foretaget ud fra den basale antagelse, at der er en lineær sammenhæng mellem et EU-lands økonomiske aktivitet og dets udledninger af et givet stof eller stofgruppe. Hvor
muligt, er der i sådanne tilfælde krydsrefereret med de få tilgængelige data for at få en indikation af usikkerheden.
De nye globale normaliseringsreferencer er fremkommet ved at anvende relationen mellem et lands/regions (EU-15) økonomiske aktivitet (målt som bruttonationalproduktet (GDP)) og dets udledninger af
stoffer, der bidrager til forsuring. Begge parametre har et relativt velfunderet statistisk grundlag for mange lande og det var muligt at vise en god korrelation mellem dem. Baseret på den viste sammenhæng er
der udarbejdet en generel ekstrapolationsmodel, der simpelt skalerer fra den europæiske normaliseringsreference til globalt niveau, en såkaldt Verdensproxy.
Rapporten beskriver de – ofte betydelige – usikkerheder, der er ved de ny normaliseringsreferencer. På trods af usikkerheden anbefaler rapporten dog brug af de europæiske normaliseringsreferencer som
udgangspunkt i en vurdering. Anbefalingerne, der er mere detaljeret beskrevet i delprojektets vejledning, begrundes i at de fleste LCA'er inkluderer belastningspotentialer, der finder sted i et bredt udvalg af
lande og regioner, og at det derfor er mest relevant at bruge normaliseringsfaktorer, der matcher det geografiske spektrum. Det anbefales dog også at anvende de danske normaliseringsreferencer og
Verdensproxyer i en følsomhedsanalyse med henblik på at fastslå, hvor robuste konklusionerne er.
Rapporten giver et detaljeret indblik i de datakilder og procedurer, der er anvendt ved beregning af alle normaliseringsreferencer. For hver enkelt belastningskategori findes talmaterialet i tabelform, inklusive
en lang række mellemregninger. Disse kapitler har mest interesse for eksperter, der for eksempel kan bruge tallene til andre typer af beregninger, men de er naturligvis også et godt udgangspunkt for
eventuelle opdateringer.
Rapporten afsluttes med et kapitel, hvori der beregnes nye vægtningsfaktorer for Danmark, EU-15 og på globalt plan. Også dette kapitel giver et detaljeret indblik i de grundlæggende data og de
usikkerheder, der er forbundet med beregningerne. Globale vægtningsfaktorer for regionale belastningskategorier behæftet med relativ stor usikkerhed, og for de lokale belastningskategorier har det ikke
været muligt at beregne en troværdig vægtningsfaktor. Dette kræver i princippet at der findes oplysninger om både de nuværende udledninger og specifikke reduktionsmål for disse for et givet land eller
region. En konsistent indsamling og bearbejdning af sådanne informationer ligger langt udenfor rammerne det nærværende projekt, og det anbefales derfor, at man anvender vægtningsfaktorerne for EU-15
som udgangspunkt i sin vurdering, idet disse er beregnet med en acceptabel sikkerhed. Det skal dog bemærkes, at de europæiske vægtningsfaktorer for lokale belastninger med stor sandsynlighed er
underestimerede i forhold til faktorerne for regionale belastninger. Årsagen er, at det selv for "nærområdet" EU-15 er vanskeligt at skabe et overblik over de enkelte landes reduktionsmål for udledninger af
enkeltstoffer og stofgrupper.
Det konkluderes afslutningsvis, at rapportens resultater giver mulighed for en livscyklusvurdering med et bredere perspektiv end den originale UMIP-metode. Det bredere perspektiv kan imidlertid kun opnås
på bekostning af en større usikkerhed, og rapportens og den tilhørende vejlednings anbefalinger fokuserer derfor på, at der bør anvendes følsomhedsanalyser i det omfang, det er muligt og relevant for at leve
op til LCA'ens målsætninger.
1 Introduction to the project
This report updates and extends the LCA normalisation and weighing data and methods that are presented in EDIP (Environmental Design of Industrial Products) (Wenzel et al. 1997; Hauchild & Wenzel
1998) further. Normalisation is a methodology used to compare results (e.g. potential greenhouse effect) to well-known figures and weighting aims to weight or rank different impact categories by using
different principles i.e. political goals etc. The fundamental principles in normalisation and weighting are described in details in Wenzel et al. (1997).
This project has had four goals through a consensus process to:
- Establish a comprehensive list of impact categories and improve the basis for the LCA practitioners choice of impact categories
- Provide more options for normalisation and weighting of regional and local impact categories
- Update the existing normalisation reference for a number of impact categories in EDIP
- Update the weighting factors in EDIP.
1.1 Background
The background for the project is the international standards as well as the theoretical and practical considerations in Wenzel et al. (1997) and Haucshild and Wenzel (1998). The relevant sections from the
relevant standards will shortly be introduced below.
1.1.1 The ISO standards of LCA and LCIA
The ISO 14040 standard "Life Cycle Assessment – Principles and frameworks" presents, as a part of an interactive process, the following steps in a Life Cycle Assessment:
- The Goal and Scope Definition
The goal of an LCA study shall unambiguously state the intended application, the reasons for carrying out the study and the intended audience, i.e. to whom the results of the study are intended to be
communicated (for details see ISO 14041 (ISO 1998)).
- Life Cycle Inventory Analysis (LCI)
The phase of the life cycle inventory analysis involves the compilation and quantification of inputs and outputs, for a given product system throughout its life cycle (for details see ISO 14041 (ISO 1998)).
- Life Cycle Impact Assessment (LCIA)
The phase of life cycle impact assessment is aimed at understanding and evaluating the magnitude and significance of the potential environmental impacts of a product system (for details see ISO 14042 (ISO
2000a)).
- Life Cycle Interpretation
The phase of Life Cycle interpretation is where the findings of either the inventory analysis or the impact assessment, or both, are combined consistent with the defined goal and scope in order to reach
conclusions and recommendations (for details see ISO 14043 (ISO 2000b)).
The goal and scope definition, the inventory and the interpretation is mandatory while impact assessment is optional.
According to ISO 14042 (ISO 2000a), life cycle impact assessment includes several steps from the Inventory to the Interpretation:
- Life cycle inventory assignment of LCI-results ("classification"), where the impact categories are defined and the exchanges from the inventory are assigned to impact categories according to their
ability to contribute to different problem areas.
- Calculation of category indicator results ("characterisation"), where the LCI results are calculated and converted to common units and finally aggregated within each impact category. Together, this
results in a numerical indicator result, i.e. the LCIA profile for the product system.
- Normalisation or calculating the magnitude of the category indicator results relative to reference values where the different impact potentials and consumption of resources are expressed on a common
scale through relating them to a common reference, in order to facilitate comparisons across impact categories.
- Weighting where weights are assigned to the different impact categories and resources reflecting the relative importance they are assigned in the study in accordance with the goal of the study.
- Interpretation where sensitivity analysis and uncertainty analysis assist interpreting the results of the life cycle assessment according to the goal and scope of the study to reach conclusions and
recommendations.
In Life Cycle Impact Assessment, the first three steps; assignment of inventory results to impact categories, the characterisation, and the classification are mandatory, while normalisation, grouping and
weighting are optional elements.
1.2 The basis of normalisation and weighting in EDIP -methodology
In EDIP as well as in the ISO standards, the overall advantage of normalisation is an increased possibility of interpretation of indicator results compared to each other. Further, it also provides an option of
control by unveiling extreme indicator results. Finally, normalisation is a preparatory element in the optional element of weighting.
The goal of normalisation is to set a common reference enabling comparison of different environmental impacts.
It is an integral part of EDIP that the impacts are summarised for the area, which actually contributes to the current condition of the environment as it is experienced. For the global impacts it makes no
difference where the environmental exchanges occur. But for regional and local impacts, only the exchanges occurring within the regions or local areas in question contribute to the current and future condition
in the environment there. Due to this reasoning the normalisation and the subsequent weighting should ideally be carried out with normalisation references and weighting factors which, for each individual
emission, are representative of the region where the emissions occur. However in the EDIP 1997, the data of the global impacts are available while data for regional impacts, generally, are not available.
Instead, EDIP use Danish normalisation references as default values.
In contrast to EDIP 97 it has been chosen to regard human toxicity and ecotoxicity as being primarily regional impacts with the same normalisation reference being applied to all EU-15 countries. It is,
however, also possible to use the updated Danish normalisation reference, e.g. in an assessment of specific Danish products or product systems, or as an element in the sensitivity analysis. The same
possibility also exists for most of the other EU-15 countries.
Due to lack of data no regional normalisation references for other regions e.g. Central Africa and the Middle East have been developed. For acidification, normalisation references are provided for EU-15 as
well as for the individual countries within EU-15. The individual normalisation references can be found in the current report.
This project has provided a large number of normalisation references for EU-15 countries but some additional work still remains to be done before the ultimate goal, i.e. normalisation references for all
relevant regions in the World, can be reached.
A different approach has been taken in a parallel project within the LCA methodology and consensus project, site-characterisation (Hauschild & Potting, 2003). The present report does not discuss the pros
and cons of either method, but provides only an update of the original EDIP97 method with respect to normalisation and weighting.
1.2.1 The person-equivalent
The global impact will always be much greater than the impact from a particular region, irrespective of the type of effect to which the impact contributes. Use of global impacts as the normalisation reference
for the global impact categories and regional impacts for the regional and the local impact categories will thus give an imbalance in the normalisation, and it will result in global impacts from the product system
coming to appear much less than the other impacts, because they are compared with the activity of the population of the entire world, while the others are compared only with the activity of e.g. the Danish
population.
To correct this bias and ensure that the set of normalising references constitutes a common scale for all impact categories, irrespective of whether they are global or regional, the normalisation references are
calculated as the background impact over the course of one year per person in the area for which the impact is computed. This gives the normalisation references the unit "impact potential per person per
year" for each individual impact category.
1.2.2 Establishing of normalisation references
Normalisation references are established for Denmark, Europe (EU-15), and worldwide. For the relevant areas surveys of emissions contributing to the different impact categories have been established. The
normalisation references are calculated based on EDIP (Wenzel et al. 1997):
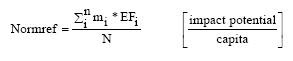
where: mi is emitted quantity of substance i
EFi is the equivalence factor for substance i
N is number of capita in the considered area
i.e. for each of the substances contributing to the impact category, the emitted quantity is multiplied with the equivalence factor for the specific substance giving the potential impact caused by the specific
substance. The potential impacts by all the contributing substances are summarised and finally divided with the number of capita in the considered area.
The figures used for normalisation in the EDIP methodology are the potential effects caused per capita in a defined geographical area e.g. potential greenhouse effect per capita per year. 1994 has been
chosen as reference year. The actual normalisation references are expressed as kg CO2-eq./capita/year. The potential environmental impact e.g. greenhouse effect is expressed as person equivalents (PE)
i.e. relative to the yearly contribution to the greenhouse effect by one person. In principle, the normalisation methodology allows different impact categories to be compared to each other. The present study
develops normalisation references for Denmark, Europe (EU-15) and worldwide.
1.2.3 Weighting
The figures used for weighting in the EDIP methodology are the political reduction goals for the individual substances contributing to the relevant impact category. 2004 has been chosen as the reference year.
The actual weighting factors are dimensionless. The weighting factors are linked to the above mentioned normalisation references with regard to the geographical area covered. The present study presents
weighting factors for Denmark, Europe (EU-15) and worldwide.
1.2.4 Reference year
In EDIP 1996, 1990 was used as reference year for normalisation, while 1990 and 2000 were chosen as reference years for weighting. In this update, 1994 and 2004 have been chosen as new reference
years for normalisation references and weighting factors, respectively. The reason for choosing 1994 as reference year has been easy availability of compilations of data for most of the countries included in
the EU-15 normalisation reference. In the recent years the availability of emission data has increased significantly e.g. by access to databases via Internet, and the delay caused by central compilation of data
can be expected to be reduced in the future. Therefore, future updating can be expected to be faster with reference years closer to the actual year.
1.3 Guide to the reader
The report is divided in three parts. The first part deals with choice of impact categories and of normalisation reference. The second part is focused on update and expansion of selected impact categories,
and the third part is dealing with update on weighting factors.
Part I includes chapter 2 and chapter 3.
Chapter 2 is an overview of five international lists regarding different impact categories. Each impact category is discussed and it is concluded whether the impact category is internationally recognised as well
as to which extend characterisation models are agreed upon.
Chapter 3 deals with a global approximation ("world proxies") of normalisation references. This includes the development of a simple extrapolation model based on emissions of acidifying substances. This
allows the calculation of world proxies of the regional impact e.g. photochemical ozone formation and acidification as well as local impact i.e. human toxicity.
Part II includes the update and expansion of impact categories. Each chapter describes shortly the impact category and includes a list of substances contributing to the impact. The methodology used for
calculation of the normalisation reference and the calculated normalisation references for the whole world, EU-15 and Denmark are presented in each chapter. For each chapter, one or more appendices
elaborates the content, e.g. by describing relevant data sources and providing detailed figures for emissions of substances in a number of countries.
Chapter 4 deals with global warming. Global warming is a global impact category.
Chapter 5 deals with stratospheric ozone depletion. Stratospheric ozone depletion is a global impact category.
Chapter 6 deals with photochemical ozone formation, Photochemical ozone formation is considered primarily as a regional impact category. Normalisation references for EU-15 as well as Denmark are
presented. Also a world proxy is presented.
Chapter 7 deals with acidification. Acidification is considered as a regional impact category. A normalisation reference for EU-15 is provided as well as normalisation references for the individual countries
within EU-15. A world proxy is presented.
Chapter 8 deals with nutrient enrichment (eutrophication). Nutrient enrichment is considered as a local as well as a regional impact category. Normalisation references for Denmark as well as for EU-15 are
provided. A world proxy is presented.
Chapter 9 deals with human toxicity. Human toxicity is a local impact category. The chapter offers a normalisation reference for Denmark together with normalisation references for each of the EU-15
countries as well. A world proxy is also presented.
Chapter 10 deals with ecotoxicity. Ecotoxicity is considered to be a local as well as regional impact category. Normalisation references for Denmark and EU are provided in the chapter. Further,
normalisation references for each of the individual EU-15 countries is presented along with a world proxy.
Part III includes chapter 11. It presents weighting factors for the seven impact categories
The actual normalisation references and weighting factors are presented in tables in the summary in each chapter. The description of data collection, data handling, data sources, and data quality is intended
for future update of the figures. The actual weighting factors are also presented in a summarising table.
1.4 References
Hauschild, M. & Wenzel, H. 1998, Environmental Assessment of Products. Volume 2 - Scientific background. First edition. Chapman & Hall, London.
ISO 1997, Environmental management – Life cycle assessment – Principles and Framework. ISO 14040.
ISO 1998, Environmental management – Life cycle assessment – Goal and scope definition and inventory analysis. ISO 14041.
ISO 2000a, Environmental management – Life cycle assessment – Life cycle impact assessment. ISO 14042.
ISO 2000b, Environmental management – Life cycle assessment – Life cycle interpretation. ISO 14043.
Wenzel, H., Hauschild, M. & Alting, L. 1997, Environmental Assessment of Products. Volume 1 - Methodology, Tools and Case Studies in Product Development. First edition. Chapman & Hall,
London.
2 Selection of impact categories
The purpose of this chapter is first of all to give an overview of impact categories that are defined in different LCA methodology frameworks. The overview is formed by going through the impact categories
defined in different LCA methodologies. Each impact category is described briefly and it is stated to which extend the impact category is included in the methodologies. Similarities and differences among the
methods are highlighted.
The overview of geographical scaling is presented in a table in which it is indicated if the international consensus also includes the characterisation model.
The chapter also includes an overview regarding recommendations of the selection of impact categories. The overview is based on the same internationally recognised framework as just mentioned. The
chapter is concluded by some general recommendations of when to apply which impact categories.
2.1 Comprehensive list of impact categories
During the recent years different national and international working groups have worked with development of characterisation methods to assign input and output data to different impact categories. The
research groups have to a large extent used the work of others as a point of reference, and some persons have participated in the work of two or more groups. The methods have been improved/modified
continuously e.g. by adaptation to restricted geographical areas or national conditions. As point of reference for the description and discussion of impact categories the present paper will use lists made by
five different groups:
- The "Leiden list" (SETAC-Europe 1992)
- The "Nordic list" (Lindfors et al. 1995)
- The SETAC "default list" (Udo de Haes 1996)
- The "EDIP list" (Wenzel et al. 1996)
- The ISO 14047 list (preliminary) (ISO 1999)
The ISO 14047 list is a draft (ISO 1999). It only includes few impact categories. The purpose of ISO 14047 is primarily to illustrate how to work with the ISO 14040 - 14043 standards and it does not
deal with which impact categorise that has to be included in LCA.
The lists of impact categories are compiled in Table 2-1.
Table 2-1 Compilation of different lists of impact categories.
List →
Impact category ↓
|
The "Leiden list "
SETAC-Europe (1992)
|
The "Nordic list"
Lindfors et al. (1995)
|
SETAC, "default list"
Udo de Haes (1996)
|
The "EDIP list"
(Wenzel et al. 1997)
|
ISO, preliminary list
(ISO 1999)
|
Global warming |
Global warming
CO2-eq.
|
Global warming
CO2-eq.
|
Global warming
CO2-eq.
|
Global warming
CO2-eq.
|
Global warming/ climate change |
Depletion of stratospheric ozone |
Depletion of stratospheric ozone
CFC-11-eq.
|
Depletion of stratospheric ozone
CFC-11-eq.
|
Depletion of stratospheric ozone
CFC-11-eq.
|
Stratospheric ozone depletion
CFC-11-eq.
|
Stratospheric ozone depletion |
Photo-oxidant formation |
Photo-oxidant formation
C2H4-eq.
|
Photo-oxidant formation
C2H4-eq.
|
Photo-oxidant formation
C2H4-eq.
|
Photochemical oxidant formation
C2H4-eq.
|
Photochemical oxidant formation (smog) |
Acidification |
Acidification
SO2-eq.
|
Acidification
SO2-eq.
|
Acidification
SO2-eq.
|
Acidification
SO2-eq.
|
Acidification |
Nutrient enrichment - Eutrophication |
Eutrophication
- PO43-eq.
- COD (chemical oxygen
demand) discharge
|
Eutrophication
- N-emissions to air
For aquatic systems:
- Aggregation of P to water
- Aggregation of N to water
- Aggregation of N to water plus N to
air
- Aggregation of N and P to water
and air
|
Eutrophication
BOD (biological oxygen
demand) discharge
- PO43-eq. is suggested
|
Nutrient enrichment
- NO3--equivalents
- N-equivalents
- P-equivalents
|
Nutrient enrichment |
Effects of waste heat on water |
|
|
included |
|
|
Ecotoxicity |
Ecotoxicity
- aquatic
- terrestrial
|
Ecotoxicological impacts
- acute
- chronic
- wastewater
|
Ecotoxicological impacts |
Ecotoxicity
- water, acute
- water, chronic
- soil
- wastewater-plants
|
Ecotoxicity |
Human toxicity |
Human toxicity
- air, water, soil
|
Human health, toxicological and non-toxicological |
Human toxicological impact |
Human toxicity
- air, water, soil
|
Human toxicity |
Occupational health and safety |
Occupational safety
- qualitatively
|
Human health impacts in work environment |
|
Work environment
- carcinogenicity
- teratogenicity
- allergy
- neurotoxicity
- hearing impairments
- repetitive work
- accidents
|
|
Odour |
Critical volume approach is suggested |
Included in "Habitat alteration..." |
Odour |
|
|
Noise |
Worst case scenario is suggested |
Included in "Habitat alteration..." |
Noise |
|
|
Radiation |
|
|
Radiation |
|
|
Waste |
Final solid waste (hazardous)
Final solid waste
(non-hazardous)
|
|
Final solid waste |
Waste
- volume waste
- hazardous waste
- slags and ashes
- radioactive waste
|
Waste |
Resource consumption
- water
- land use
|
Energy and material
- renewable and non-renewable
Space requirement
|
Energy and material
- scarcity
Water
Land, including wetland
|
Depletion of abiotic resources
Depletion of biotic resources
|
Resource consumption
- renewable
- non-renewable
|
|
Habitat alterations and impacts on biological diversity |
|
Habitat alterations and impacts on biological diversity |
|
|
|
2.2 Impact categories
The considered impact categories will be described briefly below with the impact categories included in the "EDIP"-method for the time being as point of reference (Wenzel et al., 1997). The description is
qualitative and it includes an overview of the inventory input or output data that contribute to the specific impact categories. In the description, emphasis is placed on the geographical scaling as well as impact
categories that are included.
Interrelationships between emissions, environmental impacts and their various impacts and their consequences differ from one emitted substance to another. To illustrate to examples; emission of CO2 and
CH4, is described below.
The potential impact of the emission of CO2 is global warming which is considered as a global effect. No matter where CO2 is emitted the potential impact will be the same; global warming. The
consequences of global warming can, among others, be; loss of human lives, loss of crops and loss habitats. The potential impact of CH4 could be global warming as well as photochemical ozone formation.
The effects of photochemical ozone formation could be local when smog is formed and it can be regional, when CH4 is transported, and ozone is formed.
In LCA geographical scaling is divided in
- global effects,
- regional effects,
- local effect,
- working environmental effects.
Global effects and working environmental effects are relatively well defined by name, while regional and local are not very well defined terms. "Local" could be defined as impacts caused by human activity
within a radius of 25 km. "Regional" could be defined as impacts cased by human activity outside a radius of 25 km but not effecting globally.
2.2.1 Global warming
Global warming - or the "greenhouse effect" - is the effect of increasing temperature in the lower atmosphere. The lower atmosphere is normally heated by incoming radiation from the outer atmosphere
(from the sun). A part of the radiation is normally reflected by the soil surface but the content of carbon dioxide (CO2) and other "greenhouse" gasses (e.g. methane (CH4), nitrogen dioxide (NO2),
chlorofluorocarbons etc.) in the atmosphere absorb the IR-radiation. This results in the greenhouse effect e.g. an increase of temperature in the lower atmosphere to a level above normal. The possible
consequences of the greenhouse effect include an increase of the temperature level leading to melting of the polar ice caps, resulting in elevated sea levels. The increasing temperature level may also result in
regional climate change. Wherever greenhouse gasses are emitted they contribute to the same effect and thus the impact category is considered to be global.
All five lists include global warming as an impact category. The understanding of global warming in the lists is the same and they are generally referring to the same substances like CO2, CH4 and
halocarbons as contributors to the impact. N2O is included in the youngest of the lists. Further, there is also an international agreement about the characterisation model.
The impact category is described in more detail in chapter 4 in part II of this report.
2.2.2 Stratospheric ozone depletion
Decomposition of the stratospheric ozone layer is causing increased incoming UV-radiation that leads to impacts on humans, natural organisms and ecosystems. On humans the impacts can be increased
incidence of i.e. skin cancer, cataracts and decreased immune defence. The primary impact on natural organisms and ecosystems is probably a decreased production of plankton in the South Pole region,
and this may affect the food chain dramatically. The stratospheric ozone layer occurs at an altitude from 10 - 40 km, with maximum concentration from 15 - 25 km. The maximal generation of stratospheric
ozone (O3) occurs in the top of the stratosphere at the altitude of 40 km as a result of a reaction of molecular oxygen (O2) and atomic oxygen (O). The reaction depends on the UV-radiation used in the
decomposition of oxygen and the availability of other molecules used in the absorption of excess energy from the decomposition process. No matter where the contributing substances are emitted they
contribute to the same effect, and the impact category is therefore considered to be global.
All five lists include stratospheric ozone depletion as an impact category. Further, they include the same type of substances as contributors. These are halocarbons (CFCs, HCFCs, halons, etc.). There is also
international agreement about the characterisation model. The impact category is described more detailed in chapter 5 in part II of the report.
2.2.3 Photochemical oxidant formation
Photochemical ozone formation is caused by degradation of volatile organic compounds (VOC) in the presence of light and nitrogen oxide (NOx) ("smog" as a local impact and "tropospheric ozone" as a
regional impact). The biological effects of photochemical ozone can be attributed to biochemical effects of reactive ozone compounds. Exposure of plants to ozone may result in damage of the leaf surface,
leading to damage of the photosynthetic function, discolouring of the leaves, dieback of leaves and finally the whole plant. Exposure of humans to ozone may result in eye irritation, respiratory problems, and
chronic damage of the respiratory system.
UK AEA (United Kingdom Atomic Energy Authority) Environment and Energy Modelling and Assessment Group has been leading in the development of a "Photochemical Trajectory Model" describing the
photochemical oxidant formation in the atmosphere. The model involves the following factors:
- degradation route for specific substance including information on intermediates and reactions involved in the degradation
- background level of VOCs
- background level of NOx
- residence time of specific substance in air
Depending on the actual location for the emission of the considered VOCs scenarios can be set up and a POCP-value can be calculated for the actual case.
All five lists include formation of ozone as an impact category to be considered. Substances mentioned in the lists as contributing to the impact are: Alkanes, halogenated hydrocarbons, alcohols, aldehydes,
ketones, esters, olefins, acetylenes, aromatics and other organic compounds. There is international consensus regarding the characterisation model used. The impact category is further described in chapter 6.
2.2.4 Acidification
Acidification is caused by release of protons in the terrestrial or aquatic ecosystems. In the terrestrial ecosystem the effects are seen in softwood forests (e.g. spruce) as inefficient growth and as a final
consequence dieback of the forest. These effects are mainly seen in Scandinavia and in the middle and eastern parts of Europe. In the aquatic ecosystem the effects are seen as (clear), acid lakes without any
wildlife. These effects are mainly seen in Scandinavia. Buildings, constructions, sculptures and other objects worthy of preservation are also damaged by e.g. acid rain. The substances contributing to
acidification can be transported across boundaries via air. Acidification is rarely or not at all seen in North America. The impact category is considered to regional.
All five lists include acidification as an impact category. SO2 and its potential for acid formation is suggested in all lists as the reference substance. Sulfur oxides, nitrogen oxides, inorganic acids (hydrochloric
acid, nitric acid, sulfuric acid, phosphoric acid, hydrofluoric acid, hydrogen sulfide), and ammonia are mentioned as substances contributing to acidification. Inclusion of the impact category as well as the
characterisation model is generally agreed upon internationally. The impact category is further described in chapter 7.
2.2.5 Nutrient enrichment
Nutrient enrichment (eutrophication) can be defined as: enrichment of aquatic ecosystems with nutrients leading to increased production of plankton, algae and higher aquatic plants leading to a deterioration
of the water quality and a reduction in the value of the utilisation of the aquatic ecosystem.
The primary effect of surplus nitrogen and phosphorus in aquatic ecosystems is growth of algae. The secondary effect is decomposition of dead organic material (e.g. algae) and anthropogenic organic
substances. The decomposition of organic material is an oxygen consuming process leading to decreasing oxygen saturation and - sometimes - anaerobic conditions. The anaerobic conditions in the sediment
at the bottom of lakes or other inland waters may furthermore result in production of hydrogen sulfide (H2S) which may lead to "bottom up" incidents and liberation of toxic hydrogen sulfide to the
surrounding water.
The effects of nutrient enrichment of terrestrial ecosystem are seen on changes in function and diversity of species in nutrient poor ecosystems as heaths, dune heaths, raised bogs etc. and they are caused by
atmospheric deposition of nitrogen compounds. Nutrient enrichment can be considered as a regional as well as local effect.
Nutrient enrichment is mentioned in all five lists. Nitrogen and phosphorous compounds are mentioned as the main origin of nutrient enrichment. However, nutrient enrichment is subject for strong
development in connection with LCA. Thus, there are plentiful suggestions on how to measure the potential for nutrient enrichment. The development has moved from measurement of COD, BOD and
PO43- towards NO3-. The impact category is further described in chapter 8.
2.2.6 Effects of waste heat on water
Only one of the lists suggests effects of waste heat on water as a potential impact category. However, discharge of heat defiantly has effect at the local environment. The single suggestion can not be
considered as a basis for international consensus. In all cases, the energy consumption will be part of an LCA but the possibility for better growth conditions will generally not be included. The exception is
cases where the growth condition of the close surroundings might be included in the goal and scope of an LCA.
2.2.7 Human toxicity
Human toxicity is a large group of different impacts on humans. Human toxicity includes in principle all substances that are toxic to humans. It is included in all lists. The handling differs from one list to
another. In different ways they include threshold values. While it can be concluded that there is some sort of international agreement about inclusion of human toxicity it is obvious that the perfect method is
still to be developed. Thus it is also still a challenge to develop one - or perhaps more characterisation models. The impact category is further described in chapter 9.
2.2.8 Ecotoxicity
Ecotoxicity includes in principle all substances that are toxic to the environment. Often ecotoxicity is divided in two parts: One for terrestrial and one for aquatic environment. The aquatic often has a
subcategory concerning wastewater and sludge thereof. Ecotoxicity is in all cases divided in acute and long-term effects. Depending on the environment, e.g. riverine, sea or terrestrial, the impacts can be
regional as well as local.
All five lists include ecotoxicity. The Leiden list suggests a method using maximum acceptable concentration values as the starting point. The Nordic list suggests - by referring to the complexity of the subject
- that different approaches are used and that all substances that have an effect on the environment are included in LCA studies. As a minimum requirement the Nordic list suggests that all substances are
reported. The SETAC list includes ecotoxicity. In the list it is suggested that both possible effects and doses are included. The SETAC list refers to the EDIP as a good - for the time being - approach of
handling of ecotoxicity. The EDIP includes ecotoxicity as an integrated part of the tool. The EDIP is both fate and non-fate based. In principle, all substances with an ecotoxicological potential should be
included in the tool. For the time being it is mostly developed with respect to heavy metals and a number of organic substances. Tributyltin oxide, used as an ingredient in antifouling paint, is treated
separately.
As for human toxicity, the characterisation models for ecotoxicity is not developed yet. Many institutions work in this area. The impact category is discussed in more details in chapter 10.
2.2.9 Working environment
Working environment or occupational health and safety are in principle impacts on humans (occupants) while being at job. Impacts can be physical and psychological. It may be impacts due to long term
exposure or it may - as well - be impacts due to an accident.
Working environment is mentioned in three of the five lists. The Leiden list proposes to include number of victims by accidents. However, the definition of accidents and victims are not elaborated. The
Nordic list defines the working environment more broadly and includes both chemically bound impacts and accidents. The Nordic list suggests inclusion of both a qualitative and a quantitative method. The
original EDIP method is a mixture of specific assessment of processes and a general assessment on the sector level. In the suggestion for a revised method (Schmidt et al. 2001), only a sector assessment is
included. By using the developed database it is possible to create an overview of the working environmental impacts in a relatively quick way, but the method is not recommended for detailed working
environmental assessment of specific problems.
2.2.10 Waste
Waste can be defined as co-products without value for the producer. Depending on the nature of the waste - and the local/regional possibilities - the waste can be handled in different ways, e.g. by
incineration or landfilling.
The handling of waste differs from one LCA-methodology to the other. Several waste categories have been suggested in the Leiden list, the SETAC default list and EDIP, e.g. solid waste, volume waste,
hazardous and non-hazardous waste, slags and ashes and nuclear waste. The Nordic list does not include waste directly, but argues that waste handling is a part of the technical system of the LCA, requiring
inputs (e.g. land) and producing output, and therefore the contribution to other impact categories from the waste management processes should be included. A method for this is currently under development
in a Danish project.
Until a suitable method for treating the long-term impacts from waste management has been developed, it is suggested to characterise the waste according to the general methodology used.
2.2.11 Land use
One type of impact that relates to waste management as well as other activities is land use. The impact category is not fully operational in LCA-methodology so far, but the main suggestion is that land use is
treated by including both the amount of land used for a given activity and the decrease in aesthetic value that is caused by the activity. It is also possible to include land as just one form of abiotic resources,
potentially taking the quality of the land into account. Biodiversity can also be an assessment parameter.
Both the Nordic list and the Leiden list include land use as impact categories.
Heijungs (1997) gives an overview of the methods that have been proposed but concludes that none of the proposals are fully satisfying. It is therefore recommended in the present project that land use - if
included in a LCA - is treated in a qualitative way.
2.2.12 Resource consumption
The main concern relating to the impact category "Resource consumption" is that use of a given resource leads to a reduced availability of the same resource for future generations.
Resource consumption can be looked upon from different angles. The most common way is to divide resources into a number of sub-categories in order to give a balanced view of the potential impacts, but
the approach differs to some extent in the lists. The Leiden list and the Nordic list divides resources into material and energy resources, while the SETAC default list divide resources into biotic and abiotic
resources. The Nordic list and the Leiden list also include land as a separate resource. Further, the Nordic list includes water. A common feature of all methodologies developed so far is that they use a
scarcity index for the assessment, taking into account the supply adequacy of a given resource.
The methodologies focus on the use of abiotic resources. Biotic (renewable) resources does not receive the same attention, but the problem of a non-sustainable use of e.g. wood from rain forests is
acknowledged.
2.2.13 Habitat alterations and impacts on biological diversity
Habitat alterations and impacts on biological diversity is in the Nordic list used to describe a variety of potential impacts. In Lindfors et al. (1995), the heading also covers non-chemical outputs like noise,
smell, light, etc with a potential impact that should be considered. Finally, the heading also includes effects of oil spills, introduction of genetically modified organisms and emissions of waste heat to water.
However, only a limited number of characterisation methods are assumed to be available.
2.3 Methodological inclusion of impact categories
Based on the five lists above the impact categories have been identified and categorised in groups relating to their geographical scaling and the degree of international consensus that is reached. This is
illustrated in Table 2-2.
Table 2-3
Characterisation of impact categories.
Impact categories |
Geografic scale1 |
International consensus |
|
G |
R |
L |
W |
Classsification |
Characterisation |
Global warming |
x |
|
|
|
yes |
yes |
Stratospheric ozone depletion |
x |
|
|
|
yes |
yes |
Photochemical oxidant formation |
|
x |
x |
|
yes |
(yes) |
Acidification |
|
x |
x |
|
yes |
(yes) |
Nutrient enrichment |
|
x |
x |
|
yes |
(yes) |
Effects of waste heat water |
|
|
x |
|
no |
no |
Ecotoxicity |
|
x |
x |
|
(yes) |
no |
Human toxicity |
|
x |
x |
|
(yes) |
no |
Working environment |
|
|
|
x |
yes |
no |
Odour |
|
|
x |
|
yes |
no |
Noise |
|
|
x |
|
yes |
no |
Radiation |
|
|
|
|
yes |
no |
Resource consumption |
x |
x |
x |
|
yes |
(yes) |
Land use |
|
|
x |
|
no |
no |
Waste |
|
|
x |
|
(yes) |
no |
Effects on eco-systems |
|
|
|
|
no |
no |
G: global, R: regional, L: local, W: work environment.
1. Nielsen PH and Laursen J has recently June 2000 submitted with a substantial
input regarding classification and characterisation of noise, integration of external
noise nuisance from road and rail transportation in life cycle assessment.
Choices regarding which geographical area to choose for normalisation is dealt with in chapter 3.
2.4 Which impact categories to choose?
LCA is used for many purposes. The commissioner can be authorities, companies, consumer organisations and many other interested parties. The authorities can apply LCA in order to provide
environmentally conscious public purchase, community action plans e.g. priority of packaging for beers and soft drinks and for consumer information. Companies can apply LCA in product development,
environmental product information and product-oriented environmental policy. (Consumer) Organisations can apply LCA when providing environmental conscious consumption. Thus it is obviously not
possible to set up specific guidelines that apply to every purpose of LCA.
2.4.1 Inclusion of different impact categories and standardisation
In the work of standardisation, great efforts have been made in order not to develop different LCA methodologies for different application of LCA; e.g. product development, environmental labelling etc.
Thus, in ISO 14040 (ISO 1997) it is stated that "Definition of the scope of the study include (among others) types of impact and methodology of impact assessment, and subsequent interpretation to be
used." Further it is mentioned in connection to Life Cycle Impact Assessment that "the level of detail, choice of impacts evaluated and methodologies used depends on the goal and scope of the study."
ISO 14042 (ISO 2000) provides the following guidance regarding selection of impact categories, category indicator and characterisation models (5.3): "For most LCA studies, existing impact categories,
category indicators or characterisation models will be selected. Whenever impact categories, category indicators and characterisation models are selected in an LCA study, the related information shall be
referenced. .... However, in some cases existing impact categories, category indicators or characterisation models are not sufficient to fulfil the defined goal and scope of the LCA study, and new ones have
to be defined. When new impact categories, category indicators or characterisation models are defined, the requirements and recommendations in this subclause also apply".
The category indicator can be chosen anywhere along the environmental mechanism between the LCI results and the category endpoint(s).
Further, it is stated that "the selection of impact categories, indicators and models shall be consistent with the goal and scope of the LCA study" and "the selection of impact categories shall reflect a
comprehensive set of environmental issues related to the product system being studied taking the goal and scope into consideration"
In addition to these general recommendations it is recommended when impact categories are selected that these are environmentally relevant, internationally accepted and that double counting should be
avoided.
The ISO standard does not elaborate on which impact categories should be included in different applications of LCA.
2.4.2 Inclusion of different impact categories and different methodologies
All lists used in the section about forming a maximum list include considerations about which impact categories that should be included in LCA studies. Below the approaches recommended in the lists is
presented.
2.4.2.1 The Leiden methodology
The Leiden list or methodology (SETAC-Europe 1992) does not recommend inclusion or exclusion of specific impact categories compared to other impact categories. In the Leiden "methodology" it is
recommended to consider a full LCA at first hand. When a full LCA and a detailed process tree is developed it might be possible to exclude parts of a full LCA. This depends, however, of the goal and
scope of the study. In the methodology it is recommended not to exclude anything before justification - due to results of an inventory - is provided. The methodology includes a framework for handling a
number of impact categories, see Table 2-1. These are only suggestions and are not to be regarded as exhaustive.
2.4.2.2 The Nordic methodology
The Nordic guideline (Lindfors et al. 1995) focus on "key issue identification" and therefore on studies with limited resources available. The consequence of this restriction may be that selection of a full set of
impact categories is not possible due to the data requirement. The guideline conclude in general terms that:
"A "key issue identification" type LCA will never quantify all types of potential environmental impacts ..... Some impact categories will be addressed by qualitative assessments and some may even be
omitted. The choice of impact categories is a subjective and free choice that depend on the goal of the study and the access to quantitative or qualitative inventory data. There is thus no strict requirement to
include all potential impact types in LCA as long as: i) the choice is justified in relation to the goal of the study, ii) omitted impact categories are clearly reported, and iii) conclusions are limited to the studied
impacts."
In more detailed terms the guideline presents a number of issues that should be considered when deciding which impact categories to respectively include or exclude. These considerations are i.e.
completeness and practicality. Thus, it has to be ensured that all environmental problems of relevance i.e. problems which are generally regarded as major environmental problems and also problems which
may be of specific significance for the systems under study, are included. On the other hand it is recommended that inclusion of too many categories should be avoided.
The Nordic methodology includes a list of potential relevant impact categories. However, the list is not claimed to be exhaustive, see Table 2-1.
2.4.2.3 The SETAC methodology of 1996
The main task of the SETAC 1996 document (Udo de Haes 1996) is impact assessment. This means that only little attention is paid to goal and scope definition and its impacts on choice of impact
categories. However, it is mentioned that the default list, which is presented in Table 2-1 in this chapter, is non-exhaustive.
2.4.2.4 EDIP method
Selection of impact categories is also discussed briefly in the EDIP method, and in the light of LCA as a holistic approach, all significant resource consumption, environmental impacts and impacts on the
working environment must be covered (Wenzel et al., 1998). The procedure for selection of impact categories can be expressed as:
- "Include all resource types, environmental impact categories and categories on the working environment implemented in the EDIP method.
- Consider whether some of the resource consumption and impact categories, which the EDIP method has not implemented, are significant.
- If so, include such resource consumption and impact categories. Describe and assess them quantitatively, and include them in the data on which the decision will be based together with the quantitative
assessment of the impact categories implemented in the EDIP method."
In the EDIP method the procedure for selection of impact categories is followed by a list of impact categories that are ready available; see Table 2-1.
2.4.3 Summary of the advice from the methodologies
The guidance regarding choice of impact categories from all four methodologies are more or less the same, i.e. to include the impact categories that are necessary to provide a robust result. In all of the
mentioned methodologies it is stressed that the default lists probably are relevant in most cases. However, the lists are not to be considered as exhaustive and other relevant impact categories can equally well
be included. In conclusion, the lists do not give guidelines for when an impact category should be included or can be omitted, other than it should be determined in the goal and scope definition. Furthermore,
it is stressed that LCA is an iterative process and the need for inclusion of relevant impact categories may emerge at all times during the process.
The missing guidelines apply to all applications of LCA. It is acknowledged in all lists that LCA is used for many purposes and function, but it has been an important element in the development of
LCA-standards that different methods should not be used for different applications.
It is obvious that choosing to omit certain impact categories can be a way to simplify the LCA. Again, no general rules can be given and the most important aspect is that it is done in close collaboration
between the practitioner and the commissioner and that the omissions are clearly stated and discussed in the report.
2.5 Consideration regarding choice of impact category
The ISO standards regarding LCA and the default lists recommend that the choice of impact categories are based on thorough considerations.
As demonstrated in Table 2-2 there is a large degree of correlation of suggested impact categories from one list to another. The lists and their underlying methodological approach do not guide the
practitioner in the discussion or exclusion of impact categories for different applications of LCA. This is considered to be a never-ending task. The application of LCA is so varying that it is not meaningful to
provide guidance in general term on the one hand and on the other hand it is meaningless and perhaps not possible - at least compared to the effort it would take - to prepare guidelines of the choice of
impact categories per application, e.g. product comparison, eco-labelling etc.
It is recommended that the proposed impact categories in the different lists should be included in LCA studies unless there are consciously omitted. If they are omitted this should be based on sound and
justified arguments. It is not an argument in itself for exclusion that a certain impact category is not quantifiable. However, this might very well in practise be an exclusion factor. If an impact category is not
quantifiable it could be included qualitatively. The relevance of this depends in the end of the application of the specific LCA.
2.5.1 PC Tools
The final choice of impact categories is in the end left to the practitioner and the commissioner. In practice, however, the choice is often made along with the choice of PC-tool. Several LCA software tools
exist at the market place. Best known in Denmark are undoubtedly SimaProTM, LCA Inventory Tool® and the PC-tool of EDIP (LCV). If the Danish LCV-tool is chosen for an assessment, all the impact
categories in EDIP are integrated in the assessment. As the calculation of the impacts is performed automatically, there is no reason to omit some impact categories a priori.
It should be noted in this context that many of the PC-tools deals with the same impact categories, although there are differences in methods for especially the local impacts like human toxicity, ecotoxicity
and waste. Most tools will therefore give a broad overview of the potential impacts, but the overview is of course not complete.
Some PC-tools only deals with the inventory analysis in LCA. In order to use this kind of tools, the practitioner has to develop his own way of handling the impact assessment phase, i.e. by establishing
classification and characterisation factors and – if needed – also normalisation and weighting factors. This is obviously also resource demanding and lack of time will often cause the number of impact
categories to be reduced, compared to the lists described in this project. In this case, the omission of certain impact categories should be thoroughly discussed in the goal and scope definition. The standards
do not require that specific impact categories must be included, and the commissioner, the practitioner and relevant stakeholders can therefore make the final decision on what to include.
2.5.2 Inclusion of "new" impact categories
If "new" impact categories are to be included in an assessment, it may be necessary to perform parallel calculations. This could e.g. be the case if noise or land use is mentioned in the goal and scope
definition as relevant impacts and the proposed methods are robust enough to handle the assessment. It is obvious that this is rather demanding in terms of time/resources, and the best solution on the longer
term is of course to add the impact categories to the PC-tool.
The development in LCA-methodology is rapid. New or refined methods are being developed for some impact categories, e.g. as a result of the present Danish consensus-project where changes in the
EDIP-method regarding acidification, human toxicity and the working environment have been suggested. Also, methods for new impact categories are emerging, e.g. regarding noise and land use, but none of
these developments have so far been integrated in a PC-tool. Whether or not to include these impact categories is - like for all other impact categories - primarily an issue between the commissioner and the
practitioner. The main criteria for inclusion is of course the relevance of the impact in the given context. A main criteria for exclusion of new or changed impact categories could be that it will be very time
consuming (read: costly), and the potential for added value should be related to this in the goal and scope definition.
Some have suggested general welfare parameters like prospect of women's involvement in the labour market, children labours, welfare for animals could as well as the listed in Weidema (1993). These
factors could be relevant in some cases. Inclusion of "new" impact categories as well as general welfare parameters is definitely a possibility. If a LCA practitioner choose to do so, he or she should be
attentive to the fact that it might cause difficulties when classification and characterisation models are not agreed upon internationally. However, if general welfare parameters are important for a
decision-maker the parameter should be included elsewhere in the assessment of the product or service in question.
2.6 References
Heijung, R., Guinée, J. & Huppes, G. 1997, Impact categories for natural resources and land use. CML report 138. Leiden University.
ISO 1999, Environmental management – Life cycle assessment – Examples of application of ISO 14042. ISO TR 14047. Working draft 1, September 1999.
ISO 1997, Environmental management - Life cycle assessment - Life cycle impact assessment. ISO/CD 14 042.1, 1997.01.15.
ISO 1997, Environmental management – Life cycle assessment – Principles and Framework. ISO 14040.
ISO 2000a, Environmental management – Life cycle assessment – Life cycle impact assessment. ISO 14042.
Lindfors, L.-G., Christiansen, K., Hoffmann, L., Virtanen, Y., Juntilla, V., Hanssen, O.J., Rønning, A., Ekvall, T. & Finnveden, G. 1995. Nordic Guidelines on Life-Cycle Assessment. Nord 1995:20.
Copenhagen: Nordic Council of Ministers.
Nielsen, P.H. & Laursen, J.E. 2000, Integration of external noise nuisance from road and rail transportation in lifecycle assessment.
SETAC-Europe 1992, Life-cycle assessment. LCA Workshop, Leiden 2-3 December 1991. Society of Environmental Toxicology and Chemistry (SETAC) - Europe. Brussels.
Schmidt, A., Rasmussen, P.B., Poulsen, K.E., Fløe, T. & Andreasen, J. 2003, A new approach for incorporating the working environment in LCA. EDIP 2000 methodology. dk-TEKNIK ENERGY
& ENVIRONMENT.
Udo de Haes (ed.) 1996, Towards a methodology for life cycle impact assessment. Society of Environmental Toxicology and Chemistry (SETAC) - Europe. Brussels.
Weidema, B.P. 1993, Udvikling af en metode til livscyklusvurdering af produkter, med særligt henblik på levnedsmidler (Development of a method for product life cycle assessment, with special
reference to food products).
Wenzel, H., Hauschild, M. & Alting, L. 1997, Environmental Assessment of Products. Volume 1 - Methodology, Tools and Case Studies in Product Development. First edition. Chapman & Hall,
London.
3 Development of normalisation references for different geographic areas
In chapter 1, Introduction to the project, the basic ideas and principles in normalisation of impact categories are outlined. One of the basic ideas is that global impacts are normalised globally, regional impacts
are normalised regionally and local impacts are normalised locally. In practise, however, it is not possible to obtain relevant data for specific regions all over the world. That is either because it is too big a job
to provide them or because they simply do not exist.
In this chapter a method used to predict normalisation factors from one geographical area to another is presented. The specific challenge has been to establish an approximation for a world value ("world
proxy") for the following local and regional normalisation factors: acidification, nutrient enrichment (eutrophication), photochemical ozone formation, ecotoxicity and human toxicity. The extrapolation is
relevant due to lack of worldwide emission data for the year 1994. The applicability of the outlined method is discussed for the local and regional impact categories.
Global warming and stratospheric ozone depletion are global effects with a large amount of available data that can be used in calculations. Therefore, the normalisation factors are based on available global
emission/estimates of consumption of ozone depleting substances and global emission of greenhouse gasses.
A general extrapolation method is described by using normalisation references for acidification covering approximately 40 European countries (section 3.2) due to easy availability of emission data for
substances contributing to the impact category (SO2, NOx, NH3). The present data have been used to identify relations between available acidification data and different technical and economical factors.
The application of the outlined methodology is based on the assumption that the substances contributing to the other local and regional impact categories shows the same correlation to the tested factors.
However, it is quite obvious that not all impact categories can be described equally qualified by the same parameters.
This report provides for specific impact categories relatively good data or estimates for the World, EU and Denmark. However, in many cases it is not known where the actual environmental impact takes
place. In these cases, a general proxy for the world might be just as good as the Danish and EU-15 normalisation references. At least such an option could be used as part of sensitivity analysis.
The chapter is concluded with recommendations regarding the choice of normalisation references and with considerations of the advantages and limitations of the results obtained thereby.
3.1 General considerations
Extrapolation from countries/groups of countries to a larger geographic scale (e.g. EU-15/the World) has been discussed in other LCA methodology projects, and the conclusion - like in the present study -
is that all extrapolation methods will be based on general assumptions and they are therefore never generally valid. The uncertainty introduced by using extrapolation is generally unknown and can only be
verified by worldwide emission data i.e. by comparing results of extrapolation with real data.
It was neither the aim nor practically possible within the economic frames of the project to develop a detailed or complex model for extrapolation. An extrapolation will always be uncertain regardless of the
applied methodology and the uncertainty is assumed to increase as the complexity increase. The expectation is that almost all collected data will represent some uncertainty and by adding, multiplying etc. the
uncertainty will be multiplied and thereby increased. Therefore the proposed methodology is based on as few factors as possible and the same method is used for all impact categories.
The extrapolation is based on easy available quantitative or qualitative data i.e. data that are available for the whole world in one source if possible (e.g. World Bank reports, United Nations reports,
OECD reports, EUROSTAT reports). Examples on available data are:
- GDP - gross domestic [1] product US dollars
- GNP - gross national [2] product US dollars
- Population
- Sector contribution to GDP (agriculture, industry, services) %
- Total energy consumption million tons of oil equivalent (Mtoe)
- Energy consumption (coal, oil, gas, nuclear energy, hydro power) Mtoe
- Carbon dioxide emissions million metric tons
- Energy efficiency 1987 [3] $ per kg oil equivalent
- Energy intensity total primary energy supply (Mtoe) divided by GDP (in constant prices; 1990 dollars)
Examples on advantages and disadvantages with the different data are presented in the Table 3-1.
Table 3-1
Advantages and disadvantages with different statistical data.
Parameter |
Advantages |
Disadvantages |
GDP (Gross Domestic product)
US dollars
|
GDP is available for approx. 200 countries and groups of countries; World
Bank/.United Nations statistics |
GDP depends on financial, industrial and agricultural activity; not all activities
included in GDP influence on the emissions |
GDP/capita |
Same as above |
Same as above |
GNP (Gross National product)
US dollars
|
GNP is available for approx. 200 countries and groups of countries; World
Bank/.United Nations statistics |
GNP depends on financial, industrial and agricultural activity; not all activities
included in GDP influence on the emissions |
GNP/capita |
Same as above |
Same as above |
Total energy consumption
(per capita)
million tons of oil equivalent (Mtoe)/(capita)
|
Information on total energy consumption is available in World Bank statistics |
Potential emissions depend on distribution on energy sources rather that total
energy consumption |
Commercial energy use (per capita) in oil equivalents
million tons of oil equivalent (Mtoe)
|
Information on commercial energy use available in World Bank statistics |
|
Energy consumption/distribution on sources
[%[
|
Emissions to air depend on distribution of energy sources |
Consumption of specific sources only known for the developed countries (OECD,
European) |
Energy efficiency
1987 $ per kg oil equivalent
|
Information on energy efficiency is available in World Bank statistics |
|
Energy intensity
total primary energy supply (Mtoe)/GDP
|
Emissions to air depend on distribution of energy sources |
Information on energy intensity is only known for the developed countries
(OECD, European) |
Sector contribution to GDP (agriculture, industry, services)
[%]
|
Information on sector contribution to GDP is available in World Bank statistics |
|
Agricultural activity
[qualitative]
|
Information on agricultural activity is available in World Bank statistics |
|
Technological level
[qualitative]
|
Information on technological level necessary for estimation of potential emissions |
No statistics available; qualitative estimates can be used |
Industrial activity
[qualitative]
|
Information on industrial activity can be used in estimation of emissions |
Information on industrial activity is only known for the developed countries
(OECD, Europe) |
Cleaning technology (overall)
[qualitative]
|
Information on cleaning technology can be used in estimation of emissions
using knowledge of industrialisation |
No quantitative data are available |
Cleaning technology (flue gas cleaning)
[qualitative]
|
Information on flue gas cleaning technology necessary for estimation of the
total emissions to air |
Information on flue gas cleaning is only known for the developed countries
(OECD, European) |
Wastewater treatment
[qualitative]
|
Information on wastewater treatment technology necessary for estimation of
the total emissions to water |
Information on wastewater treatment technology is only known for the developed
countries (OECD, European) |
Of the potential parameters GDP per capita, GNP per capita and energy efficiency have the best coverage, worldwide. These parameters were therefore the first choice in the efforts to establish an
extrapolation procedure, while the other parameters were investigated in less detail. The report focuses on GDP and GNP per capita in the discussion while for the other parameters, only the results of the
correlation calculations are presented. The possibility of combining several parameters was not tested. It is possible that this may give a better correlation, but this was not investigated.
3.2 General extrapolation method
Acidification depends to a large extend on the emissions of NH3, NOx and SO2; for a detailed description of the impact category see chapter 7, Acidification. According to the CORINAIR 94 summary
report (Ritter, 1997) the distribution between the above mentioned substances is 24%, 32% and 44%. The sectors responsible for the acidification in Europe are shown in Table 3-2.
Table 3-2
Distribution of emission acidifying substances from industrial sectors (Ritter, 1997).
Sector |
Distribution
%
|
Combustion in energy and transformation processes |
34 |
Agriculture and forestry, land use and wood stock change |
23 |
Road transport |
17 |
Combustion in manufacturing industry |
11 |
Other mobile sources and machinery |
6 |
Non-industrial combustion plants |
5 |
Production processes |
3 |
Waste treatment and disposal |
1 |
Extraction and distribution of fossil fuels/geothermal energy |
0.4 |
Solvent and other product use |
∼0 |
Mentioned in descending order of importance in relation to acidification, the most important sector is seen to be combustion in energy and transformation processes, agriculture and forestry, land use and
wood stock change, road transport and combustion in manufacturing industry. Information on the activity in these sectors is not available in statistical material covering the whole world but the three of the
four most important sectors are closely related to industrial and economic activity.
Economical activity and energy consumption are parameters that are often used as a measure for activity in the different sectors. This information is available in UN or World Bank statistics.
The relation between acidification and a number of selected parameters has been tested by linear regression analysis. The parameters tested are:
- GDP/capita US$/capita
- GNP/capita US$/capita
- fossil fuel/total energy
- energy efficiency (GDP/unit of energy use) US$ per kg oil equivalent)
Economical activity is measured as "gross domestic product" (GDP) and as "gross national product" (GNP). GDP measures the output of goods and services occurring within the domestic territory of a
given country whereas GNP also includes foreign income. GDP is therefore supposed to be the best indicator to describe the activity in the above mentioned industrial sectors in a specific well-defined
geographical area.
Figure 3-1 and Figure 3-2 show potential acidification potentials expressed as sulfur dioxide equivalents (i.e. kg SO2-eq./year/capita) versus GDP/capita for EU-15 respectively Europe including the
European part of Asia and Balkan (note: countries with zero values for acidification equivalents or GDP/capita are omitted in the plot and the regression line). Both parameters show very low correlation to
acidification. The correlation coefficient R2 is determined to 0.1767 and 0.0052 respectively. The very low correlation with GDP/capita for Europe may be explained by lack of industrial activity or at least
lack of reported activity in some of the middle and low-income countries.
Click here to see the figure.
Figure 3.1
Acidification (SO2-eq./year/capita) vs. GDP/capita (1994) for 15 European countries (EU-15).
Click here to see the figure.
Figure 3.2
Acidification (SO2-eq./year/capita) vs. GDP/capita (1994) for 38 European countries.
By this method the weighting of the single countries is set equal, i.e. the acidification potentials/capita for Luxembourg or Liechtenstein are equally important as the acidification potential for Germany,
regardless that Germany contributes with approximately 20% of the total acidification in EU-15.
An alternative method is to calculate the acidification potential for groups of countries where the grouping is based on income. This method weighs the acidification potential in relation to the population in the
group based on income and is described in the following sections.
The World Bank statistical material uses a grouping based on income expressed as GNP/capita resulting in four groups: high income, upper middle income, lower middle income and low income
economies; see Table 3-3 for a general description of the different income groups.
One argument for using GNP/capita for different income groups is that the level of economy somehow reflects the industrial activity (e.g. the consumption of fossil fuels). Another argument is that the World
Bank statistics include average values for a number of other parameters for the same income groups.
Figure 3.3 and Figure 3.4 show the acidification versus GDP/capita/income group and GNP/capita/income group respectively. The European countries are divided into groups according to the grouping
made by the World Bank i.e. based on GNP/capita in 1997. Average GDP/capita is also calculated for groups based on the above-mentioned grouping in order to maintain consistency in the grouping. The
average GDP/capita for the different groupings is based on GDP for the European countries actually assigned to the different income groups. The grouping is presented in Table 3-3
Table 3-3
Statistical data on income groups based on GNP/capita and GDP/capita (UN 1996; World Bank 1998),
Group |
Criteria GNP/capita [$/capita] |
Average GNP/capita (1997)
[$/capita]
|
Average GDP/capita (1994)
[$/capita]
|
Countries |
High income economies |
above 9,656 |
25,700 |
20,323 |
EU-15 + Iceland, Liechtenstein, Norway, Slovenia and Switzerland |
Upper middle economies |
3,126 - 9,655 |
4,520 |
2,588 |
Croatia, Czech Rep., Estonia, Hungary, Poland, Slovak Rep., Turkey |
Lower middle |
786 - 3,125 |
1,230 |
1,447 |
Belarus, Bulgaria, FYROM (Macadonia), Latvia, Lithuania, Romania, Russian
Fed., Ukraine |
Low income economies |
below 785 |
350 |
711 |
Albania, Armenia, Bosnia & Herzegovina |
Click here to see the figure.
Figure 3.3
Acidification (SO2-eq./year/capita) vs. GDP/capita for 38 European countries placed in four income groups (high income, upper middle income, lower middle income and low income). The income groups
are based on GNP/capita in 1997.
The relation between acidification versus GDP/capita/income group and GNP/capita/income group is tested by regression analysis. The results are presented in Figure 3.4 and Table 3-4 and show that there
is a relatively good correlation between acidification and the logarithmic value for both GNP and GDP per capita when the countries are placed in the four income groups. The best correlation coefficient
(R2=0,9092) is seen for ln(GNP/capita) and a slightly lower coefficient (R2=0,7626) is seen for ln(GDP/capita). Despite the lower correlation coefficient, the GNP/capita was used in the subsequent
calcaulations, the argument being that GNP is a better indicator of (economic) activity in a specific geographic area.
Table 3-4
Correlations between acidification (SO2-eq./year/capita) and GDP/capita/income group and GNP/capita/income group.
Acidification vs. |
Relation |
Correlation line |
Correlation coefficient (R2) |
GDP/capita |
Linear |
y = 0.0017x + 42,11 |
0.4848 |
Logarithmic |
y = 13.524ln(x) - 54.435 |
0.7626 |
GNP/capita |
Linear |
y = 0.0014x + 41.624 |
0.5351 |
Logarithmic |
y = 11.593ln(x) - 38.937 |
0.9092 |
Click here to see the figure.
Figure 3.4
Acidification (SO2-eq./year/capita) vs. GNP/capita (1997) for 38 European countries placed in four income groups (high income, upper middle income, lower middle income and low income).
The methodology using grouping of countries according to their GNP/capita has the disadvantage that the representation of countries in the different groups are unequal. The number of countries is 20, 7, 8
and 3 in the high income, upper middle income, lower middle income and low income countries, respectively. The average GNP/capita (worldwide) and GDP/capita (worldwide) are both slightly above the
average in upper middle income countries. These conditions have not been analysed further.
Statistical data regarding consumption of fossil fuels/total energy and energy efficiency were also available in sufficient detail to perform a regression analysis. However, as shown in Table 3-5, the correlation
obtained in this way was not as good when using either GNP or GDP/capita.
Table 3-5
Overview of relations between acidification and selected parameters.
|
Geographical area |
Correlation |
Corr. coef. R2 |
Comments |
GDP/capita |
EU-15 |
negative |
0.1767 |
Figure 3.1 |
GDP/capita |
EU-15 + 23 |
negative |
0.0052 |
Figure 3.2 |
GDP/capita
ln(GDP/capita)
|
38; income groups1 |
positive, linear
positive, logarithmic
|
0.4848
0.7626
|
Figure 3.3 |
GNP/capita2
ln(GNP/capita)
|
38; income groups1 |
positive, linear
positive, logarithmic
|
0.5351
0.9092
|
Figure 3.4 |
Fossil/total energy |
EU-15 |
positive |
0.1895 |
Not presented |
Energy efficiency |
EU-133 |
negative |
0.1029 |
Not presented |
Energy efficiency |
EU-13 + 6 |
negative |
0.4574 |
Not presented |
1 For definition of income groups se World Bank statistics
2 GNP data for 1997.
3 EU-15 except Germany and Luxembourg.
Based on the calculations outlined in the previous sections it was determined to use GDP per capita, divided into four income groups, for the extrapolation calculations. In order to estimate the difference
between use of GNP and GDP, the normalisation reference based on both papameters was calculated.
Based on world population and average income in GDP/capita [4] at 4,515 US$/capita the world normalisation reference for acidification can be calculated to:
59 kg SO2-eq./year/capita
Based on average GNP/capita at 5,130 US$/capita (1997) (World Bank, 1998) for the world the similar value can be calculated to:
60 kg SO2-eq./year/capita
Both methods results in a world normalisation factor at approximately 60 kg SO2-eq./.year/capita but despite a lower correlation coefficient, the method using GDP/capita is preferred as GDP is more
related to a specific geographical area than GNP.
The presented method result in the normalisation factors for the different areas and the world as shown in Table 3-6.
Table 3-6
Summary of the calculated normalisation factors areas.
Geographical area1 |
Average income
GDP/capita
|
Acidification potential
kg SO2-eq./year/.capita
|
Notes |
Denmark |
28,245 |
1014 |
Calculated; see section 7.5.1 |
EU-15 |
19,992 |
745 |
Calculated as weighted average; see section 7.5.1.1 |
High income economies |
20,323 |
74 |
Weighted average; 20 countries |
Upper middle income economies |
2,588 |
64 |
Weighted average; 7 countries |
Lower middle income economies |
1,447 |
50 |
Weighted average; 8 countries |
Low income economies |
711 |
22 |
Weighted average; 3 countries |
World |
4,5152
5,1303
|
59
60
|
GDP/capita, logarithmic
GNP/capita, logarithmic
|
1 See Table 3-3 for description of the countries representing the income groups.
2 GDP/capita.
3 GNP/capita.
4 Based on the equation shown in Table 3-4 the normalisation reference for Denmark can be calculated to 84 kg SO2-eq./year/capita.
5 Based on the equation shown in Table 3-4 the normalisation reference for EU-15 can be calculated to 79 kg SO2-eq./year/capita.
3.2.1 Proposed method and assumptions
Based on the results of the acidification scenario a general extrapolation methodology is outlined based on the following assumptions:
- GDP/capita is the best indicator for for economic activity in relation to acidification as this value is based on the activities within a specific area of consideration
- There is a linear relationship between normalisation factor and ln(GDP/capita)
- The normalisation factor is zero when the average income expressed as GDP/capita is zero
- The relation between normalisation factor for EU-15 and the world is the same for the local and regional impact categories
- The relation of GDP/capita and acidification is supposed to be the same in other regions than Europe.
Based on the assumption that the relation between the normalisation reference for acidification for EU-15 and the world is the same as the relation for other local and regional impact categories, the
relationship between the normalisation reference for acidification and for other local or regional normalisation references can be expressed mathematically as follows:

where Norm.refImpact.cat.,world is the worldwide normalisation reference for a local or regional impact category
Norm.refAcid.,world is the worldwide normalisation reference for acidification
Norm.refImpact.cat.,EU-15 is the EU-15 normalisation reference for a local or regional impact category
Norm.refAcid,EU-15 is the EU-15 normalisation reference for acidification
and if Norm.refAcid,EU-15 = 74 kg SO2-eq./year/capita and Norm.refAcid,World = 59 kg SO2-eq./year/capita then the worldwide normalisation references for local and regional impact categories can
be expressed as:

The proposed extrapolation methodology can be expressed as:


3.3 Extrapolation applied to different impact categories
This section describes advantages and disadvantages of the presented extrapolation method in relation to the effect categories photochemical ozone formation, nutrient enrichment, ecotoxicity and human
toxicity.
3.3.1 Photochemical ozone formation
The substances contributing to photochemical ozone formation (anthropogenic emissions of NMVOC, methane and CO) have been compiled worldwide and reported in the EDGAR database. The
collection, processing and publishing of 1994 data are not available and therefore only data for 1990 were used in calculating of normalisation factors. Anyway, the worldwide emission data for 1990 were
expected to give a more reliable result than using the extrapolation at the European data for 1994. The worldwide normalisation factor based on 1990 data has been calculated to:
22 kg C2H2/capita/year
This value can be compared with the normalisation factor calculated by extrapolation, which is 20 kg C2H2/capita/year. Thus, for this impact category, there seem to be a good correlation between the
calculated value and the value derived by the extrapolation methodology.
3.3.2 Nutrient enrichment
The worldwide normalisation factor for nutrient enrichment has been calculated by using the presented extrapolation methodology. Nutrient enrichment differs from a number of the other effect categories as
wastewater treatment, agricultural areas, agricultural praxis etc. influence the emission of substances contributing to nutrient enrichment. These conditions are not reflected in the GDP/capita and therefore a
systematic error may be introduced by using the presented extrapolation methodology. The worldwide normalisation reference for nutrient enrichment has been calculated to:
19 kg N-equivalents/capita/year
0.3 kg P-equivalents/capita/year
or aggregated as
95 NO3- - equivalents/capita/year.
3.3.3 Human toxicity
The proposed extrapolation method is very uncertain for the human toxicity impact category. The emission of toxic substances will be highly influenced by a large number of factors, which are not or poorly
reflected in the GDP. For instance, it is not uncommon, that `heavy' and very polluting production facilities are placed in low income (low GDP) regions and the facilities are usually equipped with less
pollution abatement devices compared to facilities in high-income countries. Further, the fuel types used for traffic and energy production may differ substantially (for instance in heavy metal content) as may
the need for transportation and energy production. Altogether, GDP is a very rough extrapolation parameter, which ideally should be supplemented with a number of infrastructure parameters. The
worldwide normalisation reference for human toxicity has been calculated to:
2.45*109 m3 air/capita/year (exposure via air)
4.18*104 m3 water/capita/year (exposure via water)
1.02*102 m3 soil/capita/year (exposure via soil)
3.3.4 Ecotoxicity
The proposed extrapolation method is very uncertain for the ecotoxicity impact category. The emission of ecotoxic substances will be highly influenced by a large number of factors, which are not or poorly
reflected in the GDP. For instance, it is not uncommon, that `heavy' and very polluting production facilities are placed in low income (low GDP) regions and the facilities are usually equipped with less
pollution abatement devices compared to facilities in high-income countries. Altogether, GDP is a very rough extrapolation parameter, which ideally should be supplied with a number of infrastructure
parameters. The worldwide normalisation reference for ecotoxicity has been calculated to:
2.82*105 m3/capita/year (etwc; chronic ecotoxicity via water)
2.33*104 m3/capita/year (etwa; acute ecotoxicity via water)
7.71*105 m3/capita/year (etsc; chronic ecotoxicity via soil)
3.3.5 Uncertainties
The uncertainties have not been quantified for the worldwide normalisation reference for the individual impact categories but are expected to be considerable. There are several reasons for this.
The extrapolation methodology has been developed for an impact category depending on three emissions (SO2, NOx and NH3), all of which are determined with a relatively high degree of precision. For
the other impact categories, the information regarding actual emissions in the primary area, e.g. Denmark, are not of the same quality, and this introduces a general uncertainty. For the impact categories
human toxicity and ecotoxicity, the number of substances potentially contributing to the impacts is very high and the actual emissions are only known in very little detail.
At the same time, it is very difficult to relate the emissions to specific economic activities. It is therefore an open question to what extent the GNP and GDP actually can be used reflect environmental impacts
and – accordingly – as an extrapolation parameter.
The use of both calculated and extrapolated normalisation references should therefore be performed with great caution. As a minimum it should be investigated whether use of other normalisation references
changes the overall conclusions of a LCA, e.g. by integrating these calculations in the sensitivity analysis.
3.4 Recommendations on selection of normalisation references
The strategy so far has been to normalise global impacts globally as well as regional and local impacts regionally, based on Danish conditions. In the present project, new normalisation references have been
developed for global, regional and local effects. For global warming and stratospheric ozone depletion global normalisation references have been calculated. For photochemical ozone formation, acidification,
nutrient enrichment, human toxicity and ecotoxicity Danish and European (EU-15) normalisation references have been calculated and worldwide normalisation references have been extrapolated.
The intentions with updating and extension of the normalisation references are that:
- Global effects are (still) normalised globally based on global figures
- Regional effect are normalised regionally based on reliable European figures
- Worldwide normalisation references are available for the local and regional effects if found appropriate; the worldwide normalisation references are based on extrapolation
Furthermore, the new set of normalisation references allows the user to choose a normalisation reference adjusted to a specific purpose. These new possibilities give occasion for choosing the main question
being when and how to apply specific normalisation references.
3.4.1 When and how to apply specific normalisation
For use of the updated EDIP97 normalisation references, the following recommendations are given for normalisation of the LCA results:
- For global impacts (global warming and stratospheric ozone depletion) always use the worldwide normalisation reference in the base case analyses
- EU-15 or Danish normalisation references can be used in a sensitivity analyses to mirror the relative importance in highly developed industrial countries with a large contribution per capita
- For regional impacts (acidification, photochemical ozone formation and nutrient enrichment) and local impacts (ecotoxicity, human toxicity) use the EU-15 normalisation reference as the base reference
- If the main impacts are known to take place in a given region, for which a more appropriate normalisation reference is available, this may be used, clearly reporting this deviation from the general
recommendation. As an example, for energy consuming devices used in Denmark, the main impacts can be assumed (or verified) to arise in Denmark and accordingly, the applied normalisation reference for
the energy-related impact categories could equally well be Denmark. For energy consuming products produced in Denmark and used (primarily) outside of Europe, the worldwide normalisation references
could be applied. It should however be noted that the European reference probably gives the most precise results if the area of use predominantly is industrialised countries and this is therefore also an option.
- Where relevant, use normalisation references for other geographical regions as an element in the sensitivity analyses, acknowledging the inherent uncertainties. The case used in the report is an example of
this, where the generally recommended normalisation reference for EU-15 could be supplemented with the Danish normalisation references in a sensitivity analysis. This is especially beneficial in relation to
nutrient enrichment, human toxicity and ecotoxicity, all of which impacts have a local as well as a regional element.
The recommendations above reflect the inherent uncertainties and lack of knowledge in normalisation, especially if the step has a broad scope. Obviously, the more is known about the product (system)
investigated as regards the geographical extent of its potential impacts, the more precisely the normalisation step will mirror the relative importance of different impacts.
The recommendations are a modification of earlier recommendations for EDIP97, where Danish normalisation references were recommended for regional and local impacts. The suggested shift to the EU
normalisation reference is justified by the better scope for many industrial products, well knowing that the absolute precision for specific products decreases in doing so.
3.5 References
Olivier J.G.J., Bouwman A.F., van der Maas C.W.M., Berdewski J.J.M., Veldt C., Bloos J.P.J., Visschedijk A.J.H., Zandveld P.Y.J., Haverlag J.L. 1996, Description of EDGAR version 2.0: A set of
global emission inventories of greenhouse gasses and ozone-depleting substances for all anthropogenic and most natural sources on a per country basis and on 1ox1o grid. RIVM report nr.
771060 002/TNO-MEP report nr. R96/119.
Ritter, M. 1997, CORINAIR 94 - Summary Report - European Emission Inventory for Air Pollutants. Copenhagen: European Environment Agency.
USBC 1996, Midyear world population 1950 - 1995. United States Bureau of the Census, International Data base. (Available at http://uastr1.math.umass.edu)
UN 1996, Statistical Yearbook 1994. Data available as of 31 March 1996. Forty-first issue. United Nations, Department for Economic and Social Information and Policy Analysis Statistics Division. New
York.
World Bank 1998, World development indicators 1998. (Available at http://www.worldbank.org.)
Appendix A: Data sources
Databases (paper)
UN 1996, Statistical Yearbook 1994. Data available as of 31 March 1996. Forty-first issue. United Nations, Department for Economic and Social Information and Policy Analysis Statistics Division. New
York.
Databases (electronic)
OECD
World Bank
World Development Indicators 1998 (available at CD-rom; extracts are available at http://www.worldbank.org)
Organisations
4 Global warming
Leif Hoffmann, dk-TEKNIK ENERGY & ENVIRONMENT
4.1 Summary
This chapter summarises the presently available data of 1994 on worldwide consumption/.emission of substances contribution to global warming. Global warming is a global effect. The substances or
emissions included are:
- Carbon dioxide (CO2)
- Methane (CH4)
- Nitrous oxides (N2O)
- CFCs (CFC-11, -12, -113, -114, -115)
- HCFCs (HCFC-22, -123, -124, -141b, -142b)
- HFCs (HFC-125, -134a, -152a)
- Halons
- Tetrachloromethane (CCl4)
- 1,1,1-Trichloroethane (CCl3CH3)
- Carbon moNOxide (CO)
The CFCs, HCFCs, HFCs, tetrachloromethane, and 1,1,1-trichloromethane are also considered as ozone depleting substances and their contribution to the stratospheric ozone depletion is described in
chapter 5, Stratopheric ozone depletion. Consumption data/emission data are generally available worldwide as the substances are regulated according to the Kyoto Protocol and the Montreal Protocol.
The normalisation reference for global warming has been calculated to:
8.7 ton CO2-eq./capita/year
Carbon dioxide (CO2) and methane (CH4) are accounting for more than 80% of the effect potential contributing to the normalisation reference for global warming. The normalisation reference is relatively
certain as almost complete data are easy available due to the regulation of consumption/.emission according to the Kyoto Protocol and the Montreal Protocol. The ozone depleting substances are
measured as consumption whereas the other greenhouse gasses are measured as emissions.
4.2 Description of the impact category
Global warming is considered as a global effect. Global warming - or the "greenhouse effect" - is the effect of increasing temperature in the lower atmosphere. The lower atmosphere is normally heated by
incoming radiation from the outer atmosphere (from the sun). A part of the radiation is normally reflected from the surface of the earth (land or oceans). The content of carbon dioxide (CO2) and other
"greenhouse" gasses (e.g. methane (CH4), nitrogen dioxide (NO2), chlorofluorocarbons etc.) in the atmosphere reflect the infrared (IR)-radiation, resulting in the greenhouse effect i.e. an increase of
temperature in the lower atmosphere to a level above normal. The energy balance is illustrated in Figure 4.1.
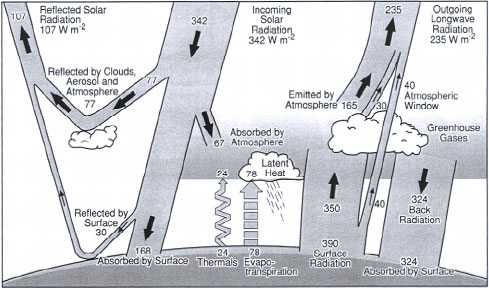
Figure 4.1
Global warming - energy balance (Trenberth et al. 1996).
The possible consequences of the greenhouse effect include an increase of the temperature level leading to increased temparatures in the oceans and melting of the polar ice caps and glaciers in mountain
areas, resulting in elevated sea levels. The increasing temperature level may also result in regional climate changes.
See also Hauschild and Wenzel (1998) for further description of the impact category.
4.3 Substances contributing to the impact category
The substances contributing to global warming are defined as substances which at normal temperature and pressure are gases and:
- absorb infrared radiation or are degraded to CO2
- have an atmospheric lifetime sufficient to allow a significant contribution to global warming
The substances considered as primary contributors to global warming are:
- Carbon dioxide (CO2)
- Methane (CH4)
- Nitrous oxides (N2O)
- CFCs (CFC-11, -12, -113, -114, -115)
- HCFCs (HCFC-22, -123, -124, -141b, -142b)
- HFCs (HFC-125, -134a, -152a)
- Halons
- Tetrachloromethane (CCl4)
- 1,1,1-Trichloroethane (CCl3CH3)
- Carbon moNOxide (CO)
For CO2 it is a special requirement that the emission must represent a net contribution, i.e. it must increase the content of CO2 in the atmosphere beyond that would be observed if the emission did not take
place. Examples of this are burning of fossil fuels and changes in land use, e.g. as a cause of deforestation. Use of biomass (wood, straw, etc.) for energy production does not give a net contribution, because
it can be assumed that the materials would degrade under all circumstances.
The CFCs, HCFCs, HFCs, tetrachloromethane, and 1,1,1-trichloromethane are also considered as ozone depleting substances and their contribution to the stratospheric ozone depletion is described in
chapter 5, Stratopheric ozone depletion. GWPs are normally based on modelling and are quantified for time horizons of 20, 100 or 500 years for a number of known greenhouse gasses (e.g. CO2, CH4,
N2O, CFCs, HCFCs, HFCs and several halogenated hydrocarbons etc.). The modelling is done by a working group under the Intergovernmental Panel on Climate Change (IPCC) and is described in a
number of reports from IPCC e.g. Houghton et al. (1996) and recently by Albritton and Meira Filho (2001). The emission of greenhouse gasses are regulated by the Kyoto Protocol (UN 1997) under the
Climate Convention (UN 1992).
The equivalency factors for the greenhouse gasses are expressed relatively to the effect of carbon dioxide i.e. as CO2-equivalents. The equivalency factors are compiled in Table 4-1. Please note that not all
greenhouse gasses mentioned in Table 4-1 are included in the calculation of the normalisation reference due to lack of knowledge about the consumption or emissions; see Appendix B for details about the
included substances. This may cause the normalisation reference to be underestimated by a few percent.
Table 4-1
Characterisation factors for global warming (in g CO2-equivalents/g). Taken from Albritton and Meira Filho, 2001.
Gas |
Lifetime (years) |
Global Warming Potential
Time horizon |
20 years |
100 years |
500 years |
Carbon dioxide |
CO2 |
|
1 |
1 |
1 |
Methane |
CH4 |
12.0 |
62 |
23 |
7 |
Nitrous oxide |
N2O |
114 |
275 |
296 |
156 |
Carbon moNOxide |
CO |
Months |
2* |
2* |
2* |
Hydrocarbons (NMHC) of fossil origin |
CxHy |
Days-months |
3* |
3* |
3* |
Partly oxidised hydrocarbons of fossil origin |
CxHyOz |
Days-months |
2* |
2* |
2* |
Partly halogenated hydrocarbons of fossil origin (not listed
below) |
CxHyXz |
Days-months |
1* |
1* |
1* |
Chlorofluorocarbons |
CFC-11 |
CCl3F |
45 |
6300 |
4600 |
1600 |
CFC-12 |
CCl2F2 |
100 |
10200 |
10600 |
5200 |
CFC-13 |
CClF3 |
640 |
10000 |
14000 |
16300 |
CFC-113 |
CCl2FCClF2 |
85 |
6100 |
6000 |
2700 |
CFC-114 |
CClF2CClF2 |
300 |
7500 |
9800 |
8700 |
CFC-115 |
CF3CClF2 |
1700 |
4900 |
7200 |
9900 |
Hydrochlorofluorocarbons |
HCFC-21 |
CHCl2F |
2.0 |
700 |
210 |
65 |
HCFC-22 |
CHClF2 |
11.9 |
4800 |
1700 |
540 |
HCFC-123 |
CF3CHCl2 |
1.4 |
390 |
120 |
36 |
HCFC-124 |
CF3CHClF |
6.1 |
2000 |
620 |
190 |
HCFC-141b |
CH3CCl2F |
9.3 |
2100 |
700 |
220 |
HCFC-142b |
CH3CClF2 |
19 |
5200 |
2400 |
740 |
HCFC-225ca |
CF3CF2CHCl2 |
2.1 |
590 |
180 |
55 |
HCFC-225cb |
CClF2CF2CHClF |
6.2 |
2000 |
620 |
190 |
Hydrofluorocarbons |
HFC-23 |
CHF3 |
260 |
9400 |
12000 |
10000 |
HFC-32 |
CH2F2 |
5.0 |
1800 |
550 |
170 |
HFC-41 |
CH3F |
2.6 |
330 |
97 |
30 |
HFC-125 |
CHF2CF3 |
29 |
5900 |
3400 |
1100 |
HFC-134 |
CHF2CHF2 |
9.6 |
3200 |
1100 |
330 |
HFC-134a |
CH2FCF3 |
13.8 |
3300 |
1300 |
400 |
HFC-143 |
CHF2CH2F |
3.4 |
1100 |
330 |
100 |
HFC-143a |
CF3CH3 |
52 |
5500 |
4300 |
1600 |
HFC-152 |
CH2FCH2F |
0.5 |
140 |
43 |
13 |
HFC-152a |
CH3CHF2 |
1.4 |
410 |
120 |
37 |
HFC-161 |
CH3CH2F |
0.3 |
40 |
12 |
4 |
HFC-227ea |
CF3CHFCF3 |
33.0 |
5600 |
3500 |
1100 |
HFC-236cb |
CH2FCF2CF3 |
13.2 |
3300 |
1300 |
390 |
HFC-236ea |
CHF2CHFCF3 |
10.0 |
3600 |
1200 |
390 |
HFC-236fa |
CF3CH2CF3 |
220 |
7500 |
9400 |
7100 |
HFC-245ca |
CH2FCF2CHF2 |
5.9 |
2100 |
640 |
200 |
HFC-245fa |
CHF2CH2CF3 |
7.2 |
3000 |
950 |
300 |
HFC-365mfc |
CF3CH2CF2CH3 |
9.9 |
2600 |
890 |
280 |
HFC-43-10mee |
CF3CHFCHFCF2CF3 |
15 |
3700 |
1500 |
470 |
Chlorocarbons |
CH3CCl3 |
|
4.8 |
450 |
140 |
42 |
CCl4 |
|
35 |
2700 |
1800 |
580 |
CHCl3 |
|
0.51 |
100 |
30 |
9 |
CH3Cl |
|
1.3 |
55 |
16 |
5 |
CH2Cl2 |
|
0.46 |
35 |
10 |
3 |
Bromocarbons |
CH3Br |
|
0.7 |
16 |
5 |
1 |
CH2Br2 |
|
0.41 |
5 |
1 |
<<1 |
CHBrF2 |
|
7.0 |
1500 |
470 |
150 |
Halon-1211 |
CBrClF2 |
11 |
3600 |
1300 |
390 |
Halon-1301 |
CBrF3 |
65 |
7900 |
6900 |
2700 |
Iodocarbons |
CF3I |
|
0.005 |
1 |
1 |
<<1 |
Fully fluorinated species |
SF6 |
|
3200 |
15100 |
22200 |
32400 |
CF4 |
|
50000 |
3900 |
5700 |
8900 |
C2F6 |
|
10000 |
8000 |
11900 |
18000 |
C3F8 |
|
2600 |
5900 |
8600 |
12400 |
C4F10 |
|
2600 |
5900 |
8600 |
12400 |
c-C4F8 |
|
3200 |
6800 |
10000 |
14500 |
C5F12 |
|
4100 |
6000 |
8900 |
13200 |
C6F14 |
|
3200 |
6100 |
9000 |
13200 |
Ethers and Halogenated Ethers |
CH3OCH3 |
|
0.015 |
1 |
1 |
<<1 |
(CF3)2CFOCH3 |
|
3.4 |
1100 |
330 |
100 |
(CF3)CH2OH |
|
0.5 |
190 |
57 |
18 |
CF3CF2CH2OH |
|
0.4 |
140 |
40 |
13 |
(CF3)2CHOH |
|
1.8 |
640 |
190 |
59 |
HFE-125 |
CF3OCHF2 |
150 |
12900 |
14900 |
9200 |
HFE-134 |
CHF2OCHF2 |
26.2 |
10500 |
6100 |
2000 |
HFE-143a |
CH3OCF3 |
4.4 |
2500 |
750 |
230 |
HCFE-235da2 |
CF3CHClOCHF2 |
2.6 |
1100 |
340 |
110 |
HFE-245cb2 |
CF3CF2OCH3 |
4.3 |
1900 |
580 |
180 |
HFE-245fa2 |
CF3CH2OCHF2 |
4.4 |
1900 |
570 |
180 |
HFE-254cb2 |
CHF2CF2OCH3 |
0.22 |
99 |
30 |
9 |
HFE-347mcc3 |
CF3CF2CF2OCH3 |
4.5 |
1600 |
480 |
150 |
HFE-356pcF3 |
CHF2CF2CH2OCHF2 |
3.2 |
1500 |
430 |
130 |
HFE-374pc2 |
CHF2CF2OCH2CH3 |
5.0 |
1800 |
540 |
170 |
HFE-7100 |
C4F9OCH3 |
5.0 |
1300 |
390 |
120 |
HFE-7200 |
C4F9OC2H5 |
0.77 |
190 |
55 |
17 |
H-Galden 1040x |
CHF2OCF2OC2F4OCHF2 |
6.3 |
5900 |
1800 |
560 |
HG-10 |
CHF2CHF2OCF2OCHF2 |
12.1 |
7500 |
2700 |
850 |
HG-01 |
CHFOCFCFCHFOCFCFOCHF2 |
6.2 |
4700 |
1500 |
450 |
The potential global warming or greenhouse effect is normally quantified by using global warming potentials (GWP) for substances having the same effect as CO2 in reflection of heat radiation. The GWP
for greenhouse gases is expressed as CO2-equivalents, i.e. the effects are expressed relatively to the effect of CO2.
The potential greenhouse effect of a process can be estimated by calculating the product of the amount of emitted greenhouse gas per functional unit and the potential for greenhouse effect given in kg
CO2-equivalents per kg for each gas. Finally, the contribution to the potential greenhouse effect from each gas has to be summarised:

where GWPi is the equivalency factor for the substance i
mi is the emission of the substance i
4.4 Methodology
The calculation of the normalisation reference for global warming has been carried out according to the methodology described in Hauschild and Wenzel (1998). The normalisation factor is calculated as:

where Normref GWP is the normalisation reference for the greenhouse effect
mi is emitted quantity of substance i
GWPi is the equivalence factor for the substance i
N is the number of capita in the considered area
Included in the normalisation reference are the following substances:
- Carbon Dioxide (CO2)
- Methane (CH4)
- Nitrous Oxides (N2O)
- CFCs (CFC-11, -12, -113, -114, -115)
- HCFCs (HCFC-22, -123, -141b, -142b)
- HFCs (HFC-134a)
- Halons
- Tetrachloromethane (CCl4)
- 1,1,1-Trichloroethane (CCl3CH3)
- Carbon MoNOxide (CO)
These substances are included due to ready availability of data on emission or consumption of these substances.
4.5 Normalisation reference
Global warming is a global impact and therefore only a global normalisation reference is relevant. For comparison the Danish emission/consumption of greenhouse gasses is presented in Appendix C to this
chapter, showing that the consumption pattern has changed as the consumption of CFC is nearly phased out. This tendency has continued in the subsequent years.
The data used in calculation of the normalisation reference for potential global warming is presented in Appendix B. The emissions of CO2 and CH4 are based on estimates made by the Oak Ridge National
Laboratory in the U.S. Houghton & Hackler (2002), Marland, Boden & Andres (2002), Stern & Kaufman (1998), emissions of N2O and CO are estimated by RIVM/TNO in the Netherlands (Olivier et
al. (2002), and consumption of CFCs, HCFCs, HFCs and halogenated carbons (CCl4 and CH3CCl3) are based on estimates made by the "Intergovernmental Panel on Climate Change" (IPCC)
(Schimel et al. 1996). The information on consumption of halons is based on reports from countries that have ratified/accessed/accepted/approved the Montreal Protocol (UNEP 1987; 1999).
The normalisation reference for global warming potential can be calculated to:
8.7 ton CO2-eq./capita/year
The value is similar to that for 1990, i.e. app. 8.7 ton CO2-eq./capita/year. The total global warming potential has increased with about 5% (from 46.1*109 ton CO2-eq. in 1990 to 48.6*109 ton
CO2-eq. in 1994 and the world population has increased from 5.3*109 in 1990 to 5.6*109 in 1994. CO2 and CH4 are the main contributors to the total global warming potential for both years, as they
constitute approximately 80 % of the effect potential. N2O, CFC-12 and CO constitute 15% of the impact potential.
The quality of the data varies from substance to substance. None of the emissions are measured in countries all over the world and often they are not measured in the same year or by the same method.
The total emissions of energy related substances are based on emission factors for the relevant processes (e.g. energy production) and models developed by IPCC have been used for estimation of world
emissions.
Emissions of CFCs, HCFCs, HFCs, and halogenated carbons (CCl4 and CH3CCl3) are not measured regularly. The potential emissions are estimated by using the assumption that all the consumed
amount of the actual substances will be emitted sooner or later due to their chemical stability. This methodology is problematic as the lifetime of the substances in the products is different depending on the
purpose of the actual product (substances used as degreasing agents are supposed to be emitted immediately during the use phase and substances used as cooling agents are supposed to be emitted after the
use phase i.e. when the goods are disposed off). However, the last assumption is only valid for some countries. Collection of goods containing cooling agents has been established in some countries (e.g.
Denmark) in order to recycle the cooling agent or to ensure proper destruction of the cooling agent.
4.6 Recommendations for future update
The future updating of the normalisation reference for global warming is recommended to be based on the same methodology and data sources as used in the present update. The statistical information used
in this report is compiled by IPCC (greenhouse gasses) and for the greenhouse gasses with ozone depleting potential from the statistical information compiled by UNEP. All the data have to be reported
regularly according to the Kyoto Protocol as well as the Montreal Protocol.
4.7 References
Albritton, D.L. and Meira Filho, L.G. (eds.): Climate Change 2001: The scientific basis. ISBN: 0521014956, Earthprint Ltd. U.K., 2001.
Hansen, J.H. 1995, Ozonlagsnedbrydende stoffer og HFC - forbrug i 1994. Miljøprojekt nr. 302. København: Miljøstyrelsen.
Hauschild, M. & Wenzel, H. 1998, Global warming as a criterion in the environmental assessment of products. In Hauschild M, Wenzel H (eds.). Environmental assessment of products. Volume 2:
Scientific background. London: Chapman & Hall.
Houghton, J.T., Meira Filho, L.G., Callander, B.A., Harris, N., Kattenberg, A. & Maskell, K. (eds.) Climate change 1995 - The science of climate change. Cambridge: Cambridge University Press.
Houghton RA, Hackler JL (2002). Carbon flux to the atmosphere from land-use changes. In Trends: A compendium of data on global change. Carbon dioxide information analysis center, Oak Ridge
National Laboratory, U.S. Department of Energy, Oak Ridge, Tennessee, USA.
Marland G, Boden TA, Andres RJ (2002). Global, regional and national CO2 emissions. In Trends: A compendium of data on global change. Carbon dioxide information analysis center, Oak Ridge
National Laboratory, U.S. Department of Energy, Oak Ridge, Tennessee, USA.
Olivier JGJ, Berdowski JJM, Peters JAHW, Bakker J, Visschedijk AJH, Bloos JPJ. Applications of EDGAR. Including a description of EDGAR 3.2: reference database with trend data for 1970-1995.
RIVM report 773301001 / NRP 410 200 051.
Schimel, D., Alves, D., Enting, M., Heimann, M., Joos, F., Raynaud, D., Wigley, T., Prather, M., Derwent, R., Ehhalt, D., Fraser, P., Sanhueza, E., Zhou, X., Jonas, P., Charlson, R., Rodhe, H.,
Sadasivan, S., Shine, K.P., Fouquart, Y., Ramaswamy, V., Solomon, S., Srinivasan, J., Albritton, D., Isaksen, I., Lal, M. & Wuebbles, D. (eds.) 1996, Radiative forcing of climate change. Chapter 2 in
Houghton, J.T., Meira Filho, L.G., Callander, B.A., Harris, N., Kattenberg, A. & Maskell, K. (eds.) Climate change 1995 - The science of climate change. Cambridge: Cambridge University Press.
Stern DI, Kaufmann RK (1998). Annual estimations of global anthropogenic methane emissions. Trends Online: A compendium of data on global change. Carbon dioxide information analysis center, Oak
Ridge National Laboratory, U.S. Department of Energy, Oak Ridge, Tennessee, USA.
Trenberth, K.E., Houghton, J.T., Meira Filho, L.G. (1996). The climate system: an overview. Chapter 1 in Houghton, J.T., Meira Filho, L.G., Callander, B.A., Harris, N., Kattenberg, A. & Maskell, K.
(eds.) Climate change 1995 - The science of climate change. Cambridge: Cambridge University Press.
UN 1992, United Nations Framework Convention on Climate Change. May 1992.
UN 1997, Kyoto Ptotocol to the United Nations Framework Convention on Climate Change. December 1997.
UNEP 1987, The 1987 Montreal Protocol on Substances that Deplete the Ozone Layer as adjusted and amended by the second Meeting of the Parties (London, 27-29 June 1990) and by the fourth
Meeting of the Parties (Copenhagen, 23-25 November 1992) and further adjusted by the seventh Meeting of the Parties (Vienna, 5-7 December 1995) and further adjusted and amended by the ninth
Meeting of the Parties (Montreal, 15-17 September 1997). Available at: http://www.unep.org.
UNEP 1998, Data report on production and consumption of ODSs - 1986 - 1996. United Nations Environment Programme, Ozone Secretariat.
UNEP 1999, Status of Ratification/Accession/Acceptance/Approval of the agreements on the protection of the stratospheric ozone layer. The Vienna Convention for the Protection of the Ozone
Layer (1985); The Montreal Protocol on Substances that Deplete the Ozone Layer (1987); The London Amendment to the Montreal Protocol (1990); The Copenhagen Amendment to the Montreal
Protocol (1992); and the Montreal Amendment to the Montreal Protocol (1997). February 8 1999. UNEP: The Ozone Secretariat. (Available at: http://www.unep.ch) .
Appendix A: Data sources
Databases (paper)
- CORINAIR reports (until now 1990 and 1994 data).
- Databases (electronic)
- CORINAIR database - expected to contain data updated yearly (National as well as European databases can be expected to be public available in the future.);
http://www.aeat.co.uk/netcen/corinair
- UNEP 1998, Data report on production and consumption of ODSs - 1986 - 1996. United Nations Environment Programme, Ozone Secretariat. Available at: http://www.unep.org.
Organisations
The following institutions are relevant in relation to obtaining data on the consumption/emission of greenhouse gasses:
- The Intergovernmental Panel on Climate Change (IPCC); The IPCC Secretariat can be contacted at:
World Meteorological Organisation
1211 Geneva 2
Switzerland
Fax: 41 22 733 1270
http://www.ipcc.ch
- United Nations Environment programme UNEP), Ozone Secretariat
Switzerland, Geneva Executive Centre: http://www.unep.ch
Kenya, Nairobi: http://www.unep.org
Contact: ozoneinfo@unep.org
- World Meteorological Organisation (WMO)
Information and Public Affairs Office
1211 Geneva 2
Switzerland
Phone 41 22 730 8314/15
Fax 41 22 733 2829
e-post: ipa@www.wmo.ch
http://www.wmo.ch
- Alternative Fluorocarbons Environmental Acceptability Study (AFEAS)
Washington, DC 20005
USA
Phone: 1 202 296-5000
Fax: 1 202 296-7960
http://www.afeas.org
Appendix B: Data used in the calculation of normalisation references for global warming.
Substances |
GWP100 |
Emis901 |
Emis94 |
Eq-emis901 |
% |
Eq-emis94 |
% |
|
|
kt |
kt |
kt CO2 |
|
kt CO2 |
|
CO2 |
1 |
2,71E+07 |
3,10E+072,3 |
2,71E+07 |
58,74 |
3,10E+07 |
63,75 |
CH4 |
23 |
3,51E+05 |
3,71E+054 |
8,07E+06 |
17,50 |
8,53E+06 |
17,55 |
N2O |
296 |
7,23E+03 |
1,12E+045 |
2,14E+06 |
4,64 |
3,31E+06 |
6,81 |
CFC-11 |
4600 |
2,98E+02 |
1,88E+026 |
1,37E+06 |
2,97 |
8,63E+05 |
1,77 |
CFC-12 |
10600 |
3,63E+02 |
2,48E+026 |
3,85E+06 |
8,34 |
2,62E+06 |
5,40 |
CFC-113 |
6000 |
1,47E+02 |
9,90E+016 |
8,82E+05 |
1,91 |
5,94E+05 |
1,22 |
CFC-114 |
9800 |
1,30E+01 |
6,00E+006 |
1,27E+05 |
0,28 |
5,88E+04 |
0,12 |
CFC-115 |
7200 |
7,00E+00 |
9,50E+006 |
5,04E+04 |
0,11 |
6,84E+04 |
0,14 |
HCFC-22 |
1700 |
1,38E+02 |
4,20E+026 |
2,35E+05 |
0,51 |
7,13E+05 |
1,47 |
HCFC-225 |
400 |
5,00E+00 |
|
2,00E+03 |
0,00 |
|
|
HCFC-123 |
120 |
|
9,10E+016 |
|
|
1,09E+04 |
0,02 |
HCFC-124 |
620 |
|
|
|
|
0,00E+00 |
0,00 |
HCFC-141b |
700 |
|
4,55E+016 |
|
|
3,19E+04 |
0,07 |
HCFC-142b |
2400 |
|
1,07E+016 |
|
|
2,57E+04 |
0,05 |
HFC-125 |
3400 |
|
|
|
|
|
|
HFC-134a |
1300 |
|
9,40E+006 |
|
|
1,22E+04 |
0,03 |
HFC-152a |
120 |
|
|
|
|
|
|
Halon 1301 |
6900 |
3,00E+00 |
|
2,07E+04 |
0.04 |
|
|
Halon 1211 |
1300 |
3,00E+00 |
|
3,90E+03 |
0.01 |
|
|
Halon (sum) |
6900 |
|
3,33E+006 |
|
|
2,30E+04 |
0,05 |
CCl4 |
1800 |
1,19E+02 |
4,50E+016 |
2,14E+05 |
0,46 |
8,10E+04 |
0,17 |
CH3CCl3 |
140 |
7,38E+02 |
3,20E+026 |
1,03E+05 |
0,22 |
4,48E+04 |
0,09 |
CO |
2 |
9,96E+05 |
3,14E+055 |
1,99E+06 |
4,32 |
6,28E+05 |
1,29 |
CH3Br |
5 |
6,30E+01 |
6,23E+016 |
|
|
3,12E+02 |
0,00 |
Total |
|
|
|
4,61E+07 |
|
4,86E+07 |
|
|
|
|
|
|
|
|
|
Population |
|
|
|
5,29E+09 |
|
5,61E+09 |
|
|
|
|
|
t CO2/p/y |
|
t CO2/p/y |
|
Normalisation reference |
|
|
|
8,72 |
|
8,67 |
|
1. Hauschild & Wenzel (1998).
2. Houghton & Hackler (2002)
3. Marland, Boden & Andres (2002)
4. Stern & Kaufman (1998)
5. Olivier et al (2002)
6. Schimel et al. (1996).
See chapter 5 for description of the procedure used for estimation of emission/consumption of halon (sum). In the calculation of global warming potential the halons are assumed to be 100% halon 1301.
Appendix C: Consumption/emission of greenhouse gasses
Consumption of greenhouse gasses/ozone depleting substances and emission of greenhouse gasses in Denmark in 1994.
Substances |
Consumption1 |
Emission |
|
1990 |
1994 |
19902 |
19943 |
|
kt |
kt |
kt |
kt |
CO2 |
|
|
56030 |
62846 |
CH4 |
|
|
761.49 |
430.64 |
N2O |
|
|
16.422 |
12.2 |
CFC-11 |
2.175 |
0.055 |
|
|
CFC-12 |
0.795 |
0.24 |
|
|
CFC-113 |
0.365 |
0.065 |
|
|
CFC-115 |
0.055 |
0.025 |
|
|
HCFC-22 |
0.84 |
0.75 |
|
|
HCFC-141b |
0 |
0.51 |
|
|
HCFC-142b |
0 |
0.145 |
|
|
Other HCFC |
0 |
0.005 |
|
|
HFC-134a |
0 |
0.52 |
|
|
HFC-152a |
0 |
0.05 |
|
|
Halon 1301 |
0.075 |
0.005 |
|
|
Halon 1211 |
0.005 |
0 |
|
|
CCl4 |
0.002 |
0.0007 |
|
|
CH3CCl3 |
0.81 |
0.57 |
|
|
CO |
|
|
789,3 |
715,4 |
CH3Br |
0.039 |
0.012 |
|
|
1 Hansen (1995).
2 Corinair 90
3 Ritter (1997).
5 Stratospheric ozone depletion
Leif Hoffmann, dk-TEKNIK ENERGY & ENVIRONMENT
5.1 Summary
This chapter summarises the presently available data on worldwide consumption of substances contributing to the stratospheric ozone depletion. Stratospheric ozone depletion is a global effect. The
substances or emissions included are:
- CFCs (CFC-11, -12, -113, -114, -115)
- HCFCs (HCFC-22, -123, -124, -141b, -142b)
- Tetrachloromethane (CCl4)
- 1,1,1-Trichloroethane (CCl3CH3)
- Halons
- Methyl bromide
Consumption data are generally available worldwide as the substances are regulated according to the Montreal Protocol. The normalisation reference for stratospheric ozone depletion has been calculated
to:
0.103 kg CFC-11-eq./capita/year
CFC-11, CFC-12 and CFC-113 accounts for two thirds of the effect potential contributing to the normalisation reference for stratospheric ozone depletion. The normalisation reference is relatively
certain as almost complete data are easy available due to the regulation of consumption according to the Montreal Protocol.
5.2 Description of the impact category
Stratospheric ozone depletion is considered as a global effect. Stratospheric ozone acts as a filter protecting the earth from incoming ultraviolet(UV)-radiation. The decomposition of ozone is enhanced by the
stratospheric input of anthropogenic halogenated compounds (e.g. CFCs, HCFCs, halons etc.). The halogenated organic compounds are relatively stable and therefore a considerable amount reaches the
stratosphere before they are decomposed. In the stratosphere they are decomposed to chlorine and bromine under the influence of UV-radiation. Nitrogen oxides, hydrogen oxides, methane, chlorine and
bromine act as catalysing agents in the decomposition of ozone.
The presence of the stratospheric ozone layer is fundamental for life on earth, as ozone absorb UV-radiation. The natural UV-radiation will make life as known today impossible. Decomposition of the
stratospheric ozone layer will cause increased incoming UV-radiation (especially UV-B-radiation) leading to impacts on humans such as increased levels of e.g. skin cancer, cataracts and decreased immune
defence. The increased UV-radiation has also an impact at natural organisms and ecosystems e.g. plankton in the South Pole region, where the decomposition of the ozone layer is already significant.
The stratospheric ozone layer occurs at an altitude from 10 - 40 km, with maximum concentration from 15 - 25 km. The maximum generation of stratospheric ozone (O3) occurs in the top of the
stratosphere at the altitude of 40 km as a result of a reaction of molecular oxygen (O2) and atomic oxygen (O). The decomposition of ozone is enhances by the stratospheric input of halogenated
compounds (e.g. CFCs, HCFCs, halons etc.).
See also Albritton et al. (1998) and Hauschild and Wenzel (1998) for further description of the impact category.
5.3 Substances contributing to the impact category
The substances contributing to stratospheric ozone depletion are defined (Solomon & Albritton 1992) as substances which:
- are sufficiently stable in the atmosphere to allow a substantial fraction to reach the stratosphere, and
- contain chlorine or bromine which, upon release into the atmosphere, will participate in a chemical decomposition of ozone
The substances or emissions normally considered as contributors to stratospheric ozone depletion are:
- CFCs (CFC-11, -12, -113, -114, -115)
- HCFCs (HCFC-22, -123, -124, -141b, -142b)
- Tetrachloromethane (CCl4)
- 1,1,1-Trichloroethane (CCl3CH3)
- Halons
- Methyl bromide
The ozone depletion potentials of the relevant substances are calculated relative to the potential of CFC-11, and therefore the ozone depletion potentials are given as CFC-11 equivalents. The regulation of
the production and consumption of ozone depleting substances by the Montreal Protocol (UNEP 1987) include the following substances mentioned in groups corresponding with the year for the
inclusion in the protocol:
- Annex A, Group I: chlorofluorocarbons (CFCs) and Group II: halons (Montreal Protocol 1987 [5]);
- Annex B, Group I: other fully halogenated CFCs, Group II: carbon tetrachloride and Group III: methyl chloroform (London Amendment 1990 [6]);
- Annex C, Group I: hydrochlorofluorocarbons (HCFCs) and Group II: hydrobromofluorocarbons (HBFCs); Annex E: methyl bromide (Copenhagen Amendment 1992 [7])
The status for ratification, accession, acceptance and approval of the different annexes varies and therefore the availability of data varies as the countries that have not ratified the annexes do not necessarily
give information on consumption of the specific substances. In other words, the information on annex A substances are assumed to be more certain than information on annex B and C substances.
A complete list of substances included in annex A (I and II) and annex B (I) is presented in Appendix B.2 "Calculation of normalisation references".
Ozone depletion potentials (ODP) have been presented by the World Meteorological Organisation (WMO) for a number of halogenated compounds (Solomon & Wuebbles 1995; Pyle et al. 1991).
A report containing information on production and consumption of ozone depleting substances (UNEP 1998) also contains ozone depleting potentials on the controlled substances. The equivalence factors
differ slightly from the factors used in the EDIP methodology. The UNEP-report also mentions that the ozone depleting potentials "are estimates based on existing knowledge and will be reviewed and
revised periodically". The equivalency factors are compared in Appendix C "Ozone depleting substances". The most recent ODP factors from UNEP (2002) have been used in the present calculation of
normalisation reference for stratospheric ozone depletion. The values are given in Table 5-1, where they also are compared to recent values from Monztka, Frazer et al. (2002).
Table 5-1
Factors for characterisation of stratospheric ozone depletion (in g CFC-11-equivalents/g). Taken from UNEP (2002) and Montzka, Frazer et al. (2002) with range representing spread of reported results
from models and semi-empirical.
|
UNEP (2002)1 |
Montzka, Frazer et al (2002) |
Substance |
Formula |
Life time, years |
Total ODP
g CFC-11 eq/g
|
Total ODP
g CFC-11 eq/g
|
ODP range
g CFC-11 eq/g
|
CFC-11
CFC-12
CFC-113
CFC-114
CFC-115
Tetrachloromethane
HCFC-22
HCFC-123
HCFC-124
HCFC-141b
HCFC-142b
HCFC-225ca
HCFC-225cb
1,1,1-Trichloroethane
Methyl chloride
Halon 1301
Halon 1211
Halon 1202
Halon 2402
Methyl bromide
|
CFCl3
CF2Cl2
CF2ClCFCl2
CF2ClCF2Cl
CF2ClCF3
CCl4
CHF2Cl
CF3CHCl2
CF3CHFCl
CFCl2CH3
CF2ClCH3
C3F5HCl2
C3F5HCl2
CH3CCl3
CH3Cl
CF3Br
CF2ClBr
CF2BR2
CF2BrCF2Br
CH3Br
|
45
100
85
300
1,700
26
12.0
1.3
5.8
9.3
17.9
1.9
5.8
5.0
1.3
65
16
2.9
20
0.7
|
1.0
1.0
0.8
1.0
0.6
1.1
0.055
0.02-0.06
0.02-0.04
0.11
0.065
0.025
0.033
0.1
10.0
3.0
6.0
0.6
|
1.0
1.0
1.0
0.94
0.44
0.73
0.05
0.02
0.02
0.12
0.07
0.02
0.03
0.12
0.02
12
6.0
1.3
<8.6
0.38
|
-
0.82-0.9
0.9
0.85-1.0
0.40-0.44
0.73-1.20
0.034-0.05
0.012-0.02
0.02-0.026
0.037-0.12
0.014-0.07
0.017-0.025
0.017-0.03
0.11-0.15
-
12-13
5-6
-
-
0.37-0.38
|
1) Where a range is given, the highest value is used in the calculations
The potential depletion of stratospheric ozone as an impact from a given process can be estimated by summarising the ODPs:

where ODPi is the equivalency factor for the substance i
mi is the emission of the substance i
5.4 Methodology
The calculation of the normalisation reference for ozone depletion has been carried out according to the methodology described in Hauschild and Wenzel (1998). The normalisation factor is calculated as:
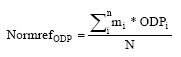
where NormrefODP is the normalisation reference for the ozone depletion
mi is emitted quantity of substance i
ODPi is the equivalence factor for substance i
N is the number of capita in the considered area (the World)
Included in the normalisation reference are the following substances:
- CFCs (CFC-11, -12, -113, -114, -115)
- HCFCs (HCFC-22, -123, -141b, -142b)
- Halons
- Tetrachloromethane (CCl4)
- 1,1,1-Trichloroethane (CCl3CH3)
- Methyl bromide
These substances are included due to ready availability of data on emission or consumption of these substances.
5.5 Normalisation reference
Ozone depletion is a global impact and therefore only a global normalisation reference is relevant. For comparison, the Danish consumption of ozone depleting substances is presented in appendix D
"Consumption of ozone depleting substances".
The nomalisation reference has been calculated by using information collected by UNEP (UNEP, 2002). Although the UNEP data are aggregated in classes of substances, they can be assumed to represent
the most precise and up-to-date information on ozone depleting substances. The consumption of ozone depleting substances has been used as an approximation for the emission as emission data does not
exist due to the character of the emissions. The emissions are in most cases diffuse and therefore not measured. The reason for using the above-mentioned approximation is that the relevant substances are
chemically very stable (an important quality in the use of the substances) and therefore the consumed amounts are expected to emit sooner or later. The actual delay will vary from substance to substance
depending on the actual use. The UNEP data are summarised in Table 5-2:
Table 5-2
Calculation of the normalization reference (based on UNEP, 2002)
|
ODP 1990 |
ODP 1994 |
Montreal Protocol |
Substance classes |
kt CFC-11-eq |
% |
kt CFC-11-eq |
% |
Annex A, Group1 |
CFC's |
732,1 |
61,2 |
358,1 |
61,9 |
Annex B, Group 1 |
Other fully halog. CFC's |
4,4 |
0,4 |
1,8 |
0,3 |
Annex C, Group1 |
HCFC |
14,4 |
1,2 |
25,1 |
4,3 |
Annex B, Group II |
Carbon tetrachloride |
181,5 |
15,2 |
123,4 |
21,3 |
Annex B, Group III |
1,1,1-trichloroethane |
62,7 |
5,2 |
14,2 |
2,5 |
Annex A, Group II |
Halons |
162,6 |
13,6 |
34,5 |
6,0 |
Annex E |
Methyl bromide |
38,6 |
3,2 |
21,7 |
3,7 |
|
Total ODP |
1196,3 |
|
578,8 |
|
|
|
|
|
|
|
|
Population |
5,29E+09 |
|
5,61E+09 |
|
|
|
|
|
|
|
|
Normalisation reference |
|
|
|
|
|
kg CFC-11-eq/capita/year |
0,226 |
|
0,103 |
|
|
|
|
|
|
|
|
EDIP97 Normalisation reference |
0,202 |
|
|
|
The normalisation reference for ozone depletion potential can thus be calculated as:
0.103 kg CFC-11-eq./capita/year
The similar value for 1990 can be calculated to 0.226 kg CFC-11-eq./capita/year. This means that the normalisation reference is reduced by about 55%. The decrease can be explained by a significant
reduction of the consumption of ozone depleting substances and an increase of the world population. At the same time the estimated ODP's for the CFC-12 and CFC-13 have been increased, adding to a
higher normalisation reference.
For 1994, the consumption of CFC-11, CFC-12 and CFC-113 constitutes approximately two thirds of the total consumption expressed as CFC-11 equivalents. With HCFCs as the exception, the
contribution in absolute figures has decreased for all relevant compounds although most markedly for the CFCs. The reason for these findings are obviously that CFCs to a large extent have been replaced
by HCFCs as refrigerants.
The normalisation reference is about 50% of that calculated in the original EDIP97. The difference is of course related to the actual decrease in thee total consumption of ozone depleting substances, but it is
interesting to note that there also is a large difference when examining the calculations based on the information that was available at the time of the calculations. Here, it can be seen that the calculation made
in EDIP97 underestimated the normalisation reference with about 20-25%. The reason for this is probably that the data collection was not fully implemented in all relevant countries. Another difference is that
some of the ODP's for specific substances have been re-evaluated, which in the total picture also causes an increase in the total ODP. The development in the assessment of ODP's for different substances is
illustrated in Appendix C.
5.5.1 Alternative calculations
Appendix B.1. shows an alternative calculation of the normalisation references for 1990 and 1994. The major difference from the selected database is that in this alternative calculation, a linear decrease in
the consumption of ozone depleting substances from 1990-1999 has been assumed, based on the fgures in Schiemel et al, 1996. Obviously, this causes a large overestimation –about 50% - of the ozone
depleting potential in the reference year 1994.
Appendix B.2. shows another alternative calculation of the normalisation reference. Here, the information collected by WMO/UNEP (Schiemel et al, 1996) and UNEP (1998) is used. A comparison
between Appendix B.2. and the updated figures in Table 5-2 shows that the figures for consumption of CFC's in 1994 were underestimated in the early report. The comparison, however, also shows that the
consumption of CFC's in 1990 was overestimated by about 7% in 1998, compared to the 2002 report.
The two alternative calculations thus demonstrate the scientific and regulatory development in the area of stratospheric ozone depletion. The figure chosen as the normalisation reference can be assumed to
represent the best possible knowledge, although there stille are some limitations in the data quality as discussed below.
5.5.2 Data quality
The general quality of the data used in the calculations can be considered as good. There are, however, some methodological problems that must be remembered.
Emissions of CFCs, HCFCs, HFCs, and halogenated carbons (CCl4 and CH3CCl3) are not measured regularly. The potential emissions are estimated by using the assumption that all the consumed
amount of the actual substances will be emitted sooner or later due to their chemical stability. This methodology is problematic as the lifetime of the substances in the products differs depending on the
purpose of the actual product. Substances used as degreasing agents are supposed to emit immediately during the use phase and substances used as cooling agents are supposed to emit after the use phase,
i.e. when the goods are disposed off. However, the last assumption is only valid for some countries as collection of goods containing cooling agents has been established in some countries (e.g. Denmark)
in order to recycle the cooling agent or to ensure proper destruction of the cooling agent.
Another inherent uncertainty in calculation of the normalisation reference is that the ODP of specific substances is based on models or semi-empirical knowledge. This causes frequent changes of the ODP's,
caused by the development of better models and collection of empirical data. The development is illustrated by the ODP's used for different calculations by different organisations, see Table 5-1 and
Appendix C for examples.
5.6 Recommendations for future update
The future updating of the normalisation reference for ozone depletion is recommended to be based on the same methodology and data source as used in the present update. The statistical information is
compiled by UNEP and this information is also expected to be available in the future i.e. yearly updates of data on production and consumption of ozone depleting substances.
5.7 References
Albritton, D.L., & Kuijpers, L. (eds.) 1999, Synthesis of the Reports of the Scientific, Environmental Effects, and Technology and Economic Assessment Panels of the Montreal Protocol. A
Decade of Assessment for Decision Makers Regarding the Protection of the Ozone Layer: 1988-1999. Nairobi: United Nations Environment Programme (UNEP). (Available at http://www.unep.ch or
http://www.unep.org).
Hansen, J.H. 1995, Ozonlagsnedbrydende stoffer og HFC - forbrug i 1994. Miljøprojekt nr. 302. København: Miljøstyrelsen.
Hauschild, M. & Wenzel, H. 1998, Stratospheric ozone depletion as a criterion in the environmental assessment of products, in Environmental assessment of products. Volume 2: Scientific
background, eds. Hauschild M, Wenzel H. London: Chapman & Hall.
Pyle, J.A., Wuebbles, D., Solomon, S. & Zvenigorodsky, S. 1991, Ozone depletion and chlorine loading potentials. World Meteorological Organisation: Scientific assessment of stratospheric ozone:
1991 Global ozone research and monitoring project. Report no. 25. Geneva.
Schimel, D., Alves, D., Enting, M., Heimann, M., Joos, F., Raynaud, D., Wigley, T., Prather, M., Derwent, R., Ehhalt, D., Fraser, P., Sanhueza, E., Zhou, X., Jonas, P., Charlson, R., Rodhe, H.,
Sadasivan, S., Shine, K.P., Fouquart, Y., Ramaswamy, V., Solomon, S., Srinivasan, J., Albritton, D., Isaksen, I., Lal, M. & Wuebbles, D. (eds.) 1996, Radiative forcing of climate change. Chapter 2 in
Houghton, J.T., Meira Filho, L.G., Callander, B.A., Harris, N., Kattenberg, A. & Maskell, K. (eds.) Climate change 1995 - The science of climate change. Cambridge: Cambridge University Press.
Solomon, S. & Albritton, D.L. 1992, Time-dependent ozone depletion potentials for short- and long-term forecasts. Nature, vol. 357, pp. 33-37.
Solomon, S. & Wuebbles, D. (lead authors) 1995, Ozone depletion potentials, global warming potentials and future chlorine/bromine loading in Scientific assessment of ozone depletion, 1994 eds.
Albritton, D.L., Watson, R.T. & Aucamp, P.J. World Meteorological Organisation, Global Ozone Research and Monitoring Project - Report No. 37. Geneva: WMO.
UNEP 1987, The 1987 Montreal Protocol on Substances that Deplete the Ozone Layer as adjusted and amended by the second Meeting of the Parties (London, 27-29 June 1990) and by the fourth
Meeting of the Parties (Copenhagen, 23-25 November 1992) and further adjusted by the seventh Meeting of the Parties (Vienna, 5-7 December 1995) and further adjusted and amended by the ninth
Meeting of the Parties (Montreal, 15-17 September 1997). Available at: http://www.unep.org.
UNEP 1998, Data report on production and consumption of ODSs - 1986 - 1996. United Nations Environment Programme, Ozone Secretariat.
UNEP 1999, Status of Ratification/Accession/Acceptance/Approval of the agreements on the protection of the stratospheric ozone layer. The Vienna Convention for the Protection of the Ozone
Layer (1985); The Montreal Protocol on Substances that Deplete the Ozone Layer (1987); The London Amendment to the Montreal Protocol (1990); The Copenhagen Amendment to the Montreal
Protocol (1992); and the Montreal Amendment to the Montreal Protocol (1997). February 8 1999. UNEP: The Ozone Secretariat. (Available at: http://www.unep.ch).
UNEP (2002). Production and consumption of ozone depleting substances under the Montreal Protocol 1986-2000. UNEP Ozone Secretariat, Apreil 2002. (http://www.unep.org/ozone).
Appendix A: Data sources
The production and consumption of substances contributing to stratospheric ozone depletion are well registered due to the Montreal Protocol. According to the protocol the parties that have ratified the
protocol has to report the consumption of ozone depleting substances every year. The data on the total production and consumption may improve in the future as more countries can be expected to ratify the
protocol. The quality of the data from the countries that have ratified the protocol may also be expected to improve because more knowledge and experience is obtained.
Databases (paper/electronic)
UNEP (2002). Production and consumption of ozone depleting substances under the Montreal Protocol 1986-2000. UNEP Ozone Secretariat, Apreil 2002. (http://www.unep.org/ozone)
Organisations
The following institutions are relevant in relation to obtain data on the world production and consumption of ozone depleting substances:
- United Nations Environment programme UNEP), Ozone Secretariat
Switzerland, Geneva Executive Centre: http://www.unep.ch
Kenya, Nairobi: http://www.unep.org
Contact: ozoneinfo@unep.org
- World Meteorological Organisation (WMO)
Information and Public Affairs Office
1211 Geneva 2
Switzerland
Phone 41 22 730 8314/15
Fax 41 22 733 2829
e-post: ipa@www.wmo.ch
http://www.wmo.ch
- UNEP IE OzonAction Programme
F-75739 Paris Cedex 15
France
Phone 33 1 44 37 14 50
Fax 33 1 44 37 14 74
http://www.unepie.org
- Alternative Fluorocarbons Environmental Acceptability Study (AFEAS)
Washington, DC 20005
USA
Phone: 1 202 296-5000
Fax: 1 202 296-7960
http://www.afeas.org
- The Intergovernmental Panel on Climate Change (IPCC); The IPCC Secretariat can be contacted at:
World Meteorological Organisation
1211 Geneva 2
Switzerland
Fax: 41 22 733 1270
http://www.ipcc.ch
Appendix B.1: Alternative calculation of normalisation references
Substances |
ODP |
Emis90 |
Emis941 |
Emis90 |
% |
Emis94 |
% |
|
|
kt |
kt |
kt CFC-11-eq |
|
kt CFC-11-eq |
|
CFC-11 |
1 |
2,98E+02 |
1,88E+02 |
2,98E+02 |
27,50 |
1,88E+02 |
26,75 |
CFC-12 |
1 |
3,63E+02 |
2,48E+02 |
3,63E+02 |
33,50 |
2,48E+02 |
35,30 |
CFC-113 |
1 |
1,47E+02 |
9,90E+01 |
1,47E+02 |
13,56 |
9,90E+01 |
14,12 |
CFC-114 |
0,94 |
1,30E+01 |
6,00E+00 |
1,22E+01 |
1,13 |
5,64E+00 |
0,80 |
CFC-115 |
0,44 |
7,00E+00 |
9,50E+00 |
3,08E+00 |
0,28 |
4,18E+00 |
0,60 |
HCFC-22 |
0,05 |
1,38E+02 |
4,20E+02 |
6,90E+00 |
0,64 |
2,10E+01 |
2,99 |
HCFC-225 |
0,025 |
5,00E+00 |
|
1,25E-01 |
0,01 |
|
|
HCFC-123 |
0,02 |
|
9,10E+01 |
|
|
1,82E+00 |
0,26 |
HCFC-124 |
0,02 |
|
|
|
|
|
|
HCFC-141b |
0,12 |
|
4,55E+01 |
|
|
5,46E+00 |
0,78 |
HCFC-142b |
0,07 |
|
1,07E+01 |
|
|
7,49E-01 |
0,11 |
Halon 1301 |
12 |
3,00E+00 |
|
3,60E+01 |
3,32 |
|
|
Halon 1211 |
6 |
3,00E+00 |
|
1,80E+01 |
1,66 |
|
|
Halon (sum) |
10 |
|
3,33E+00 |
|
|
3,33E+01 |
4,75 |
CCl4 |
0,73 |
1,19E+02 |
4,50E+01 |
8,69E+01 |
8,02 |
3,29E+01 |
4,69 |
CH3CCl3 |
0,12 |
7,38E+02 |
3,20E+02 |
8,86E+01 |
8,17 |
3,84E+01 |
5,48 |
CO |
|
9,96E+05 |
3,14E+05 |
|
|
|
|
CH3Br |
0,38 |
6,30E+01 |
6,23E+01 |
2,39E+01 |
2,21 |
2,37E+01 |
3,38 |
Total |
|
|
|
1,08E+03 |
|
7,01E+02 |
|
|
|
|
|
|
|
|
|
Population |
|
|
|
5,29E+09 |
|
5,61E+09 |
|
|
|
|
|
kg OPD/p/y |
|
kg OPD/p/y |
|
Normalisation reference |
|
|
|
0,20 |
|
0,125 |
|
1 Schimel et al. (1996) except consumption data for halons and methyl bromide which originates in UNEP (1998).
A detailed description of the data originating from UNEP (1998) is given in Appendix B.2. The consumption is reported in ODPs and the consumption of halons and methyl bromide (in ton) has been
calculated for the purpose of calculating the normalisation reference for ozone depletion as in Hauschild & Wenzel (1998).
The consumption of halons (as a group) is 33,300 ton CFC-11-eq./year and is assumed to be halon-1301. Based on this assumption and that the equivalency factor for halon 1301 was set at 10 ton
CFC-11-eq./ton halon 1301, the amount of consumed halons can be calculated to 3,300 ton/year. The contribution to the total ozone depleting potential in 1994 is based on the total amount of halons and
the revised ODP for halon 1301. Recently, the equivalency factor has been suggesteed to be increased to 12 ton CFC-11-eq./ton halon 1301 (Montzka, Frazer et al. (2002)), and this factor has been used
in the update calculations.
The consumption of methyl bromide is 37,400 ton CFC-11-eq./year. Based on the equivalence factor used in 1994 (1.3 ton CFC-11-eq./ton methyl bromide), the amount of methyl bromide can be
calculated to 62,300 ton/year. The new equivalence factor suggested by Montzka, Frazer et al. (2002) (i.e. 0.38 ton CFC-11-eq./ton methyl bromide) is used in the update calculations.
Appendix B.2: Calculation of normalisation references, based on 1998 information
Normalisation references calculated on basis of statistical information collected by UNEP/Ozone Secretariat from the countries that have ratified/.accessed/accepted/approved the Montreal Protocol with
the London and Copenhagen Amendments (UNEP 1998). The consumption data cover some countries that have ratified/accessed/accepted/approved the Montreal Protocol but not the London
Amendment (regulation of annex B substances: I: "other" fully halogenated CFCs, II: carbon tetrachloride and III: methyl chloroform) and Copenhagen Amendment (regulation of annex C substances: I
hydrochlorofluorocarbons, II: hydrobromofluorocarbons and annex E substance: methyl bromide). The consequence is that data are not complete for all substances.
The production and consumption are reported in ODPs according to equivalency factors presented in the same publication; the equivalency factors are presented in appendix C.
Annex |
Substances |
1990 |
|
1994 |
|
|
|
ton ODP/year |
% |
ton ODP/year |
% |
AI |
Chlorofluorocarbons (CFC-11, -12, -113, -114, -115) |
789,442 |
60.05 |
353,132 |
71.23 |
AII |
Halons (halon 1211, 1301, 2402) |
177,668 |
13.52 |
33,354 |
6.73 |
BI |
Other CFCs (CFC-13, -111, -112, -211, -212, -213, -214, -215, -216, -217) |
4,050 |
0.31 |
1,776 |
0.36 |
BII |
Carbon tetrachloride |
228,525 |
17.38 |
34,146 |
6.89 |
BIII |
1,1,1-Trichloroethane |
65,763 |
5.00 |
14,226 |
2.87 |
CI |
HCFCs |
13,760 |
1.05 |
21,742 |
4.39 |
CII |
HBFCs |
|
0.00 |
|
0.00 |
EI |
Methyl bromide |
35,340 |
2.69 |
37,393 |
7.54 |
Total |
|
1,314,548 |
|
495,769 |
|
Population |
|
5.29E+09 |
|
5.61E+09 |
|
Normalisation reference |
kg ODP/capita/year |
0.25 |
|
0.09 |
|
For some of the countries the consumption has not been required nor reported for the years of interest i.e. 1990 and 1994. A rough extrapolation has been made from the consumption before and after the
year of interest; a linear correlation has been assumed.
Appendix C: Ozone depleting potentials
The table below presents ozone depleting potentials for the most common ozone depleting substances. The calculation of ODP is based on an emission scenario, a calculation model and an atmospheric
scenario. Further details on different approaches can be found in Hauschild and Wenzel (1998).
Substance |
Formula |
Life time, years |
ODP1
EDIP97
|
ODP
UNEP 982 and 20004
|
ODP3
WMO
|
CFC-11
CFC-12
CFC-113
CFC-114
CFC-115
Tetrachloromethane
HCFC-22
HCFC-123
HCFC-124
HCFC-141b
HCFC-142b
HCFC-225ca
HCFC-225cb
1,1,1-Trichloroethane
Halon 1301
Halon 1211
Halon 1202
Halon 2402
Halon 1201
Halon 2401
Halon 2311
Methyl bromide
|
CFCl3
CF2Cl2
CF2ClCFCl2
CF2ClCF2Cl
CF2ClCF3
CCl4
CHF2Cl
CF3CHCl2
CF3CHFCl
CFCl2CH3
CF2ClCH3
C3F5HCl2
C3F5HCl2
CH3CCl3
CF3Br
CF2ClBr
CF2BR2
CF2BrCF2Br
CF2HBr
CF3CHFBr
CF3CHClBr
CH3Br
|
505
102
85
300
1,700
42
13.3
1.4
5.9
9.4
19.5
2.5
6.6
5.4±0.4
65
20
25
1.3
|
1.00
0.82
0.90
0.85
0.40
1.20
0.04
0.014
0.03
0.10
0.05
0.02
0.02
0.12
12
5.1
~1.25
~7
~1.4
~0.25
~0.14
0.64
|
1.00
1.00
0.80
1.00
0.60
1.10
0.055
0.02
0.022
0.11
0.065
0.025
0.033
0.10
10
3.0
-
6.0
0.74
0.7-1.2
0.60
|
1.00
1.00
1.00
0.94
0.44
0.73
0.05
0.02
0.02
0.12
0.07
0.02
0.03
0.12
12
6.0
1.3
<8.6
0.38
|
0. Solomon and Wuebbles (1995), Pyle et al. (1991) and Solomon and Albritton (1992) cited from Hauschild and Wenzel (1998).
1. UNEP (1998).
2. Montzka, Frazer et al. (2002)
3. UNEP (2002)
Appendix D.1: Consumption of ozone depleting substances
Information on the worldwide consumption of CFC, HCFC, HFC and halons are compared in the table below.
Substances |
Denmark |
EU-15 |
World |
World |
|
Consumption |
Production |
Emission |
|
AFEAS |
AFEAS |
kt |
|
kt |
kt |
kt |
CFC-11 |
|
0.055 |
|
125.0 |
60.2 |
137.4 |
CFC-12 |
|
0.24 |
|
165.0 |
133.6 |
211.5 |
CFC-113 |
|
0.065 |
|
66.0 |
29.5 |
40.1 |
CFC-114 |
|
|
|
6.0 |
3.2 |
4 |
CFC-115 |
|
0.025 |
|
6.0 |
6.8 |
11.9 |
HCFC-22 |
|
0.75 |
|
520.0 |
239.4 |
218.9 |
HCFC-225 |
|
|
|
|
na |
na |
HCFC-123 |
|
|
|
13.0 |
na |
na |
HCFC-124 |
|
|
|
|
0.9 |
0.4 |
HCFC-141b |
|
0.51 |
|
65.0 |
81.2 |
24.8 |
HCFC-142b |
|
0.145 |
|
10.7 |
38.4 |
10.7 |
Other HCFC |
|
0.005 |
|
|
na |
na |
HFC-125 |
|
|
|
|
na |
na |
HFC-134a |
|
|
|
9.4 |
50.4 |
9.4 |
HFC-152a |
|
|
|
|
na |
na |
Halon 1301 |
|
0.005 |
|
|
na |
na |
Halon 1211 |
|
0 |
|
|
na |
na |
Halon (sum) |
|
|
|
3.3 |
na |
na |
CCl4 |
|
0.0007 |
|
10.0 |
na |
na |
CH3CCl3 |
|
0.57 |
|
120.0 |
na |
na |
CO |
emission |
7.15E+02 |
4.51E+04 |
9.96E+05 |
na |
na |
CH3Br |
|
0.012 |
|
62.3 |
na |
na |
The table contains consumption and emission survey data from AFEAS (Alternative Fluorocarbons Environmental Acceptability Study). AFEAS is an organisation representing producers of CFC, HCFC
and HFC. They cover less than 35% of the worldwide CFC-production and 90% - 100% of HCFC/HFC as they primarily cover European, American (North and South) and Japanese producers whereas
producers from Eastern Europe and other Asiatic countries are outside AFEAS. The conclusion is that the world consumption of ozone depleting substances reported by AFEAS is below the actual
consumption and therefore not useable for the present project.
Appendix D.2: Consumption of ozone depleting substances
Consumption of greenhouse gasses/ozone depleting substances and emission of greenhouse gasses in Denmark in 1994.
Substances |
Consumption1 |
1990 |
1994 |
kt |
kt |
CFC-11 |
2.175 |
0.055 |
CFC-12 |
0.795 |
0.24 |
CFC-113 |
0.365 |
0.065 |
CFC-115 |
0.055 |
0.025 |
HCFC-22 |
0.84 |
0.75 |
HCFC-141b |
0 |
0.51 |
HCFC-142b |
0 |
0.145 |
Other HCFC |
0 |
0.005 |
HFC-134a |
0 |
0.52 |
HFC-152a |
0 |
0.05 |
Halon 1301 |
0.075 |
0.005 |
Halon 1211 |
0.005 |
0 |
CCl4 |
0.002 |
0.0007 |
CH3CCl3 |
0.81 |
0.57 |
CO |
|
|
CH3Br |
0.039 |
0.012 |
1 Hansen (1995).
6 Photochemical ozone formation
Karsten Fuglsang, dk-TEKNIK ENERGY & ENVIRONMENT
6.1 Summary
This chapter summarises the presently available data on emission of substances to the Danish and the European environment. The emissions include:
- nmVOC - non methane volatile organic carbons
- CO - carbon moNOxide
- CH4 - methane
Emission data for these substances are generally available for Denmark and EU-15 as well as for a number of other European countries. For the time being, worldwide emission data are available for 1990
and therefore the worldwide normalisation reference is based on 1990-data. This fact is exceptional for photochemical ozone formation. The normalisation references are presented in Table 6-1.
Table 6-1
Summary of normalisation references for photochemical ozone formation for Denmark, EU-15 and Worldwide in 1994.
|
|
Denmark |
EU-15 |
World |
1994 |
kg C2H4/capita/year |
20 |
25 |
221,2 |
1 1990.
2 Based on the extrapolation method outlined in section 3, 2, the normalisation reference can be calculated to 20 kg C2H2/capita/year.
The worldwide normalisation reference can be calculated to 20 kg C2H2/capita/year by the extrapolation method outlined in 3.2,General exstrapolation method. The extrapolated value is nearly the same as
the value calculated by using the worldwide emission data for 1990. nmVOC accounts for 70-80% of the effect potential contributing to the normalisation reference. The Danish and EU-15 normalisation
references are relatively certain, as the relevant emissions to air have been measured through many years according to the Convention on Long-range Transboundary Air Pollution and the following
Protocol on nmVOC (adopted in 1991).
6.2 Description of impact category
6.2.1 Tropospheric ozone formation
Ozone is formed in the troposphere under the influence of sunlight when nitrogen oxides are present. When VOCs are also present, peroxy radicals can be produced. Peroxy radicals are highly reactive and
toxic compounds, and the presence of peroxy radicals can result in an increase of the concentration of ozone through a complex reaction pattern. The primary reactions responsible for the formation of
ozone in the troposphere are described in 6.3. Ozone is a secondary pollutant, as there is practically no ozone present in source emissions derived from human activity.
Tropospheric ozone, or ground level ozone, has been recognised as an important environmental impact on the regional scale. At high concentrations it is hazardous to human health, but already at lower
concentrations it causes damage to the vegetation. Ozone is a trans-boundary pollutant, and it can be produced or consumed by other pollutants during transport over long ranges. The health problems
caused by ozone have generally been considered to be an effect of the very high peaks of ozone concentration, known as ozone episodes. Increased background levels of ozone causes damage to
vegetation, and thereby ozone also imposes an economic threat through a potential reduction of crop yield. It is assumed that anthropogenic emissions have resulted in a rise in the global background of
ozone concentration from around 10 ppb in the year 1900 to around 20 ppb in 1975 (Fenger 1995).
6.2.2 POCP - impact category
In the EDIP model, the photochemical ozone formation is described through POCP, the photochemical ozone creation potential, as an individual impact category (Hauschild & Wenzel 1998). POCP is used
in Europe for the ranking of VOCs according to their ability to produce ozone. In the US, a slightly different approach is used for the same purpose: Incremental Reactivity (Carter et al. 1995).
POCP describes the production of ozone from a VOC emission through computer modelling of a complex series of chemical reactions in the atmosphere over a given scenario. A large amount of input
data is required for the calculation of POCP by the model. The input data consist of the following principal components (Derwent et al. 1996):
- Emission inventories for VOCs and NOx
- Chemical and photochemical data
- Dry deposition rates for different surfaces
- Meteorological data
The model describes the chemical composition of primary pollutants during the transport away from their sources and of secondary pollutants during the transport towards the sensitive receptors where
environmental damage may occur. In the model, the chemical composition of parcels of air is followed as they travel trans-boundary across Europe. Emissions of NOx, CO, SO2 and VOCs are introduced
into the air parcels in a series of trajectory studies. The trajectories are meant to be illustrative of the general situation during photochemical episodes in Europe, and they illustrate the photochemical
production of ozone over 1-5 days (Derwent & Jenkins 1991). For a given VOC, POCP is calculated as the average of the results of the three scenarios. Most of the VOCs are oxidised more than 95%
after 4-5 days, so that the calculated POCP represents the total ozone creation potential.
6.2.3 Definition of POCP
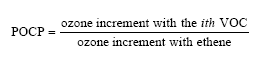
POCP is generally presented as a relative value where the amount of ozone produced from a certain VOC is divided by the amount of ozone produced from an equally large emission of ethene:
The unit of POCP is grams of ethene equivalents per gram of gas (g C2H4/g VOC). Ethene has been chosen as a reference gas as it is one of the most potent ozone precursors of all VOCs.
By definition, the calculated POCP values are not absolute values. POCP will be a function of the scenarios chosen, i.e. from one geographical area to another. As data for e.g. the chemical and
photochemical reactions are often not known in great detail, their representation in the model will often be a compromise. Therefore, even for the same scenario, the POCP values can be calculated with
higher precision when more accurate input data and more powerful computer tools are available.
The most commonly used POCP values have been calculated by Derwent and Jenkin (1991) and Derwent (1996), primarily from scenarios for the transport of ozone to the United Kingdom. IVL
(Instituttet för Vatten- och Luftvårdsforskning) in Sweden has calculated values for POCP under Scandinavian conditions using lower NOx concentrations and lower VOC emission rates. Because of the
importance of the NOx-level for the ozone formation, the low- NOx values for POCP under Scandinavian conditions are found to be slightly different, but generally a good agreement with the high- NOx
values (as calculated by Derwent for UK) is found.
6.3 Substances contributing to the impact category
The principal precursors of tropospheric ozone are
- NOx
- VOCs including CH4
- CO
Reactions (I)-(III) govern the background level of ozone in the troposphere:
NO2 + hn NO + O (I)
O + O2 O3 (II)
NO + O3 NO2 + O2 (III)
If VOCs are also present, they are oxidised to produce peroxy radicals. Peroxy radicals can either consume NO or convert it to NO2 and thus compete with ozone produced by reaction (II). Less ozone is
thereby destroyed through reaction (III), and the ozone concentration will then increase.
The ozone creation potential for several VOCs is dependent on the NOx concentration. In the calculation of a 1990 normalisation reference for POCP by Hauschild and Wenzel (1998), it was chosen not to
differentiate between high-NOx and low-NOx values in the calculation of a normalisation reference for Europe. Because of the dependency on NOx concentration, POCP values (and the calculated of the
normalisation references) will differ between areas of high NOx and low NOx concentrations. For this reason, high-NOx and low-NOx values for POCP were introduced. High NOx values are generally
used for densely populated and highly industrialised areas, where high NOx concentrations occur. The European Union was in this study defined as a high-NOx area except for the Scandinavian countries
and Ireland, as shown in Table 6-7.
6.4 Methodology
The calculation of normalisation references for 1994 has been carried out according to the methodology described in Wenzel et al. (1997). For photochemical ozone formation (POF), the normalisation
reference for 1994 is calculated as
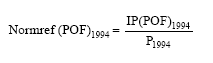
where Normref (POF)1994 is the normalisation reference for the area in question for the year 1994 [g C2H4-equivalents per person]
IP(POF)1994 is the impact potential for photochemical ozone formation for the area in question for the year 1994 [g C2H4-equivalents]
P1994 is the population of the area in question [persons]
The total impact potential IP (POF) from substances contributing to the impact category is calculated according to
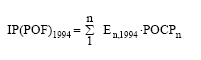
where En, 1994 is the total emission of substances from source category n to the atmosphere in 1994 for the area in question [kt per year]
POCPn is the photochemical ozone creation potential for source category n [kt C2H4-equivalents per kt]
Because of the influence of NOx on the formation of ozone from VOCs, the POCP values should be chosen according to the background concentration of NOx in the area in question.
6.4.1 Normalisation reference for 1990
For the calculation of a 1990-normalisation reference for Denmark, Hauschild and Wenzel (1998) chose low-NOx POCP values in the EDIP model. For the calculation of a 1985-normalisation reference
for EU, high-NOx POCP values was used. For practical reasons, the European Union was not separated into regions according to the background concentration of NOx. The POCP values used have been
calculated for each individual source category. These POCP values were calculated as weighted averages of individual POCPs for major nmVOC sources as shown in Table 6-2. A discussion of the
methodology is given in section 6.6.
Table 6-2
Average POCP values (g C2H4/g VOC) for nmVOC mixtures from source
categories as used in the EDIP model for calculation of normalisation
references for Denmark in 1990 and for EU in 1985 (Hauschild & Wenzel
1998).
Source of nmVOC |
POCP
(DK 1990)
low- NOx
|
POCP
(EU 1985)
high- NOx
|
Petrol-engine car, exhaust |
0.5 |
0.6 |
Petrol-engine car, vapour |
0.4 |
0.5 |
Diesel-engine car, exhaust |
0.5 |
0.6 |
Power plants |
0.4 |
0.5 |
Burning of woods or twigs |
0.6 |
0.6 |
Food industry |
0.4 |
0.4 |
Surface coating |
0.5 |
0.5 |
Dry cleaning |
0.3 |
0.3 |
Refining and distribution of oil |
0.4 |
0.5 |
Natural gas leakage |
0.2 |
0.2 |
Farming |
0.4 |
0.4 |
Other substances to be included |
CH4 |
0.007 |
0.007 |
CO |
0.04 |
0.03 |
6.4.2 Emission data: CORINAIR
For the purpose of updating normalisation references to 1994 for European countries, data for emissions of VOCs, CO and CH4 were taken from the CORINAIR 1994 database (Ritter 1997). In the
CORINAIR 1994 database, national emissions are summarised in main source sectors according to main source sectors as shown in Table 6-3. These main source sectors differ slightly from the source
categories used in the EDIP 1985 study for EU and 1990 study for Denmark.
The main source sectors of CORINAIR 1994 was defined as the source categories to be used in updating normalisation references for European countries. Because these source categories are not identical
with the source categories used in the calculations of normalisation references for Denmark and EU, this means that the weighted averages for POCP should be carefully transferred from the previously
calculated values. If transfer is not justifiable, new POCP values should be calculated from an analysis of the composition of the emissions of the individual subsectors. Table 6-3 shows the main source
sectors of the CORINAIR 1994 database and the equivalent sources of nmVOC applied for use of POCP values.
Table 6-3 Main source sectors as defined in CORINAIR 1994 and source categories
applied for calculation of POCP from nmVOC.
# |
Main source sector CORINAIR 1994 (Ritter 1997) |
Suggested use of POCP values from source category as used in EDIP (Hauschild
& Wenzel 1998): |
1 |
Combustion in energy and transformation industries |
Stationary combustion/power plants |
2 |
Non-industrial combustion plants |
Stationary combustion/power plants |
3 |
Combustion in manufacturing industry |
Stationary combustion/power plants |
4 |
Production processes |
Industrial processes (non specific) |
5 |
Extraction and distribution of fossil fuels/geothermal energy |
Oil refining and distribution |
6 |
Solvent and other product use |
Surface coating |
7 |
Road transport |
Petrol-engine cars |
8 |
Other mobile sources and machinery |
Diesel-engine cars |
9 |
Waste treatment and disposal |
Farming2 |
10 |
Agriculture and Forestry, luwc3 |
Farming |
1 Road transport is included as emissions from petrol-engine cars (assumption in EDIP
1985/1990 inventory).
2 Best estimate for non-methane VOC from waste treatment and disposal.
3 luwc: land use and wood stock change
Table 6-4
Main source sectors used in the CORINAIR 1994 (Ritter 1997) emission inventory and the POCP values for nmVOC assigned to these source categories (Hauschild &Wenzel 1998).
|
nmVOC
Low-NOx
POCP |
nmVOC
High-NOx
POCP |
Main source sector (source category) |
kt C2H4/kt |
kt C2H4/kt |
1. Combustion in Energy and transformation industries |
0,4 |
0,5 |
2. Non-industrial combustion plants (nmVOC) |
0,4 |
0,5 |
3. Combustion in manufacturing industry (nmVOC) |
0,4 |
0,5 |
4. Production processes (nmVOC) |
0,3 |
0,3 |
5. Extraction and distribution of fossil fuels/geothermal energy (nmVOC) |
0,4 |
0,5 |
6. Solvent and other product use (nmVOC) |
0,5 |
0,5 |
7. Road transport (nmVOC)1 |
0,4 |
0,6 |
8. Other mobile sources and machinery (nmVOC) |
0,5 |
0,6 |
9. Waste treatment and disposal (nmVOC) |
0,4 |
0,4 |
10. Agriculture and Forestry, luwc2 (nmVOC) |
0,4 |
0,4 |
1 Low value: road transport, Denmark; high value from petrol/diesel engine car, exhaust.
2 luwc: land use and wood stock change
6.5 Normalisation references
6.5.1 Denmark 1994
The calculation of a normalisation reference for Denmark is shown in detail in Appendix A. The calculated impact potential for nmVOC, CO and CH4 is shown in Table 6-5.
Table 6-5
Impact potentials calculated for 1994 for nmVOC, CO and CH4 for Denmark.
Substance |
Total emission
1994
(kt/year)
|
Impact potential 1994
(kt C2H4/year)
|
% distribution |
nmVOC |
166.1 |
72.1 |
69 |
CO |
715.4 |
28.6 |
28 |
CH4 |
430.6 |
3.0 |
3 |
Total |
|
103.7 |
100 |
The normalisation reference for Denmark in 1994 is calculated in Table 6-6.
Table 6-6
Impact potentials calculated for 1990 and 1994 for nmVOC, CO and CH4 for Denmark.
|
EDIP 19901 |
This study
1994
|
Total impact potential (POCP)
(kt C2H4/year)
|
100.9 |
103.7 |
Population (mio.) |
5.14 |
5.20 |
Normalisation reference Denmark
(kg C2H4/capita/year)
|
20.0 |
19.9 |
1 Hauschild and Wenzel (1998).
6.5.2 European Union 1994
The total impact potential IP(POF) from substances contributing to the impact category for a region like the EU having both areas of low-NOx and high-NOx background concentrations is calculated
according to the formula

where En, low-NOx, 1994 is the total emission of substances from source category n to the atmosphere in 1994 for the given area of low-NOx background concentration [kt per year]
En, high-NOx, 1994 is the total emission of substances from source category n to the atmosphere in 1994 for the given area having high-NOx background concentration [kt per year]
POCPn, low-NOx is the photochemical ozone creation potential for source category n in low-NOx areas [kt C2H4-equivalents per kt]
POCPn, high-NOx is the photochemical ozone creation potential for source category n in high-NOx areas [kt C2H4-equivalents per kt]
For the area covered by EU-15, areas of high- and low-NOx have been defined as shown in Table 6-7.
Table 6-7
Areas defined as low NOx and high NOx background concentration for calculation
of the impact potential in EU-15.
Low-NOx POCP factors used |
Sweden, Denmark, Finland and Ireland |
High-NOx POCP factors used |
Germany, United Kingdom, France, Italy, Spain, Netherlands, Greece, Belgium,
Portugal, Austria, Luxembourg |
The calculation of a normalisation reference for EU-15 is shown in detail in Appendix B. The calculated impact potential for nmVOC, CO and CH4 is shown in Table 6-8.
Table 6-8
Impact potentials calculated for 1994 for nmVOC, CO and CH4 for EU-15.
Substance |
Total emission
low NOx
(kt/year)
|
Total emission
high NOx
(kt/year)
|
Impact potential 1994
(kt C2H4/year)
|
%
distribution
|
nmVOC |
1,288.4 |
14,272.1 |
7,769 |
83 |
CO |
2,804.9 |
42,278.1 |
1,381 |
15 |
CH4 |
1,749.0 |
20,775.7 |
158 |
2 |
Total |
|
|
9,307 |
100 |
The normalisation reference is calculated for EU-15 in 1994 as shown in Table 6-9.
Table 6-9
Impact potential calculated for nmVOC, CO and CH4 for EU-15. Comparison
with 1985 values.
|
EDIP 19851 |
This study
1994
|
Total impact potential (POCP)
(kt C2H4/year)
|
5,050 |
9,307 |
Population (mio.) |
321.7 |
370 |
Normalisation reference EU-15, 1994 (kg C2H4/capita/year) |
18.0 |
25.2 |
1 Hauschild and Wenzel (1998). Based on high-NOx POCP values for the entire EU.
The low-NOx countries contribute to the EU-15 normalisation with a higher POCP-emission per capita, as shown in Table 6-10.
There are three major source categories contributing to the total impact of nmVOC: Road transport, solvent and other product use, and agriculture and forestry. From Table 6-10, it is clear that the
difference between the POCP emission per capita in the low-NOx countries compared to the high-NOx countries is due to differences in the CORINAIR 1994 emission inventory for nmVOC for the
source category "Agriculture and Forestry". This is probably due the difficulties in estimating nmVOC emissions from this category.
Table 6-10
Comparison between normalisation references for low-NOx and high NOx countries in EU-15, with and without nmVOC emissions from source category "Agriculture and forestry".
|
Normalisation reference for
EU-15, 1994
(kg C2H4/capita/year)
|
Normalisation reference for EU-15, 1994
excluding nmVOC emissions from agriculture and forestry
(kg C2H4/ capita /year)
|
Low-NOx countries |
29.5 |
21.3 |
High-NOx countries |
24.9 |
22.1 |
Total, EU-15 |
25.2 |
22.1 |
6.5.3 World 1990
One of the aims of this project has been to calculate a global normalisation reference for 1994. A methodology for the calculation of such a "world proxy" is proposed in chapter 3, Development of
normalisation references for different geographic areas. This methodology applies to the most frequent situation for impact categories: global emission data cannot be found in an acceptable form or quality.
In the case of POCP, anthropogenic emissions of the key substances (nmVOC, methane and CO) can be found for 1990 in the EDGAR database (Olivier et al. 1996). RIVM has not yet any published
global emission data for 1994. Given the data from 1990, it was decided to calculate a normalisation factor for 1990 instead of 1994. Because of the undoubtedly larger uncertainties associated with the
calculation of the global emissions from GDP, it is suggested that a 1994 normalisation reference for POCP is calculated when an update of the EDGAR database is at hand.
Appendix C shows the calculation of POCP emissions for the geographical areas as defined in EDGAR. For nmVOC, a division between high-NOx and low-NOx areas is done. High-NOx areas are in
Appendix C marked as "HN" (USA, Western Europe, Eastern Europe, Japan) and low-NOx are marked as "LN" (Canada, Latin America, Africa, Soviet Union (CIS), Middle East, India, China, East
Asia, Oceanic areas, Int. shipping). Table 6-11 shows the result of the calculation for POCP in kt C2H4 total and the calculated normalisation reference for 1990.
Table 6-11
Calculated normalisation reference for POCP for the world, 1990.
|
World proxy 1990 |
Global POCP, NMVOC (kt C2H4) |
77,000 |
Global POCP, methane (kt C2H4) |
2,000 |
Global POCP, CO (kt C2H4) |
37,000 |
TOTAL global POCP (kt C2H4) |
116,000 |
Population |
5.29*109 |
Normalisation reference World, 1990
(kg C2H4/capita/year)
|
22 |
Table 6-11 shows a very large contribution to the worldwide effect potential from CO emissions. The global CO emission per person calculated from the emissions in the EDGAR database is
relatively large compared to the CO emission per person in the CORINAIR database. As low temperature combustion is assumed to be the major source of CO emissions, the reason for this deviation
could be the widespread use of uncontrolled combustion in households etc. in the third world. A further assessment of the emission factors for CO used in the EDGAR database has not been pursued.
By using the extrapolation method proposed in chapter 3 the worldwide normalisation reference for photochemical ozone formation is estimated to 20 kg C2H4-eq./capita/year. The normalisation reference
found by using the extrapolation method is slightly lower than the value found by using the 1990 emission inventory.
6.5.4 Comparison of updated normalisation references with previously used normalisation references
In Table 6-12 the normalisation references for photochemical ozone formation for 1994 estimated in this study are compared with the previously estimated normalisation references (Hauschild & Wenzel
1998).
Table 6-12
Summary of normalisation references for photochemical ozone formation estimated in the present study compared with previously normalisation references.
|
|
Denmark |
EU-15 |
World |
19901 |
kg C2H4/capita/year |
20 |
182 |
- |
1994 |
kg C2H4/capita/year |
20 |
25 |
223 |
1 Hauschild and Wenzel (1998).
2 1985.
3 1990.
The normalisation reference for Denmark is approximately the same as the normalisation reference for 1990 whereas the normalisation reference for EU-15 has increased by 25% from 1985 to 1994. The
worldwide normalisation reference has been estimated to 22 kg C2H4-eq./capita/year based on a worldwide inventory for nmVOC, CO and CH4.
6.6 Recommendations for future updating
6.6.1 Quality of emission data
Any updating of normalisation references should focus on uncertainties of the data used from emission inventories. The CORINAIR database used in this study is prepared by the European Environment
Agency and should as such be considered as the most validated database for European Union. The UN-ECE database is also an option as a considerable number of countries have ratified the Convention
on Long-range Transboundary Air Pollution (UN-ECE, 1979) and the protocol on VOC (UN-ECE, 1991). As apparent from the differences found in section 6.5.2 between POCP (nmVOC)
emissions per capita for Scandinavian countries compared to the rest of Europe, the countries reporting nmVOC data to CORINAIR have encountered difficulties in assessing the emissions from source
category no. 10 (agriculture and forestry). A future updating should generally focus on the quality of the emission data used, and a special attention should be paid to data from the source category
"agriculture and forestry".
Considerable discrepancy was found between emission data from the European countries in the CORINAIR 1994 database and in the UN-ECE database [8] for 1994. This is especially the case for
methane. The reason for these discrepancies has not been found.
6.6.2 NOx inclusion/exclusion?
POCP is a parameter developed to express the potential of VOCs to create ground level ozone, and emissions of NOx will thus not be included in the impact assessment for photochemical ozone formation
in the EDIP model. The impact of NOx is in EDIP present through the impact of acidification, nutrient enrichment and human toxicology. However, model calculations have shown that reductions of
emissions of NOx will in many cases have a larger effect than VOC reductions on the reduction of the ozone concentration levels (Fenger 1995). Hauschild and Wenzel (1998) has chosen POCP as the
best existing and the most internationally accepted model for the calculation of photochemical ozone potential for emissions of individual substances, and NOx can not be assigned as a separate factor
within this model. The use of POCP as a characterisation factor is still considered as the best internationally accepted method available. The error introduced hereby should however be evaluated.
To give the highest possible ozone reduction, it is necessary not only to reduce VOC and CO emissions, but also NOx emissions. As the impact of NOx on ozone production is not included in EDIP, a false
impact from this characterisation factor could be expressed in the assessment of a product system by EDIP. The magnitude of this error will be site dependent.
6.6.3 Focus on most potent individual VOCs?
McBride et al. (1997) has suggested that a more efficient ozone reduction could be achieved if the emission reductions focused on the most potent ozone producers instead of reducing all VOCs regardless
of the species. In EDIP, the VOCs are partly divided, not according to their ozone creation potential, but according to source categories. For a more efficient abatement strategy, it might be useful to
subdivide the VOCs according to their individual ozone creation potential. For practical reasons, this could be done for a reduced number of highly potential VOCs. E.g., at present solvent using industries
will have the same POCP factor (0.4-0.5 as shown in Table 6-2) regardless of the potency of the individual compound in the present EDIP model. During the assessment of a specific product, individual
factors applied to individual solvents will give a more realistic impact assessment of the specific tropospheric ozone formation.
6.6.4 Simplified POCP factor for nmVOC?
IVL has in a recent study concluded that POCP ranges might be more useful to introduce in stead of site-specific POCP values (i.e. high-NOx vs. low NOx) (Altensted & Pleijel 1998). Except for
production processes, all POCP factors in low and high NOx areas vary between 0,4-0,6 kg C2H4/kg for nmVOC. nmVOC from production processes is allocated a POCP factor of 0,3 kg C2H4/kg. In
the light of the uncertainties involved in the calculation of the POCP factors and the uncertainties of the emission data, it should be considered whether one weighted factor for POCP can be used for both
high and low-NOx areas. This would simplify calculations considerably and save resources in the elaboration of normalisation references for the use in LCA.
6.6.5 Exclusion of methane in future POCP calculations?
In the light of the relatively small contribution from methane to the total impact from POCP, it should be considered whether methane should be excluded in future POCP calculations.
6.6.6 Worldwide
The calculated worldwide normalisation reference for 1990 is relatively high as compared to the normalisation references for EU and Denmark. This is due to the relatively high global CO emissions per
capita derived from the EDGAR database. The uncertainties of the emission data in the EDGAR database should be compared to the uncertainties of the CORINAIR database.
The POCP factors used to calculate the worldwide normalisation reference are derived from European POCP model calculations and divided into low-NOx and high-NOx areas. POCP factors in tropical
areas might deviate considerably from the European POCP values meteorological factors (i.e. sunlight) influence the formation of ozone. This potential source of error should be investigated in the future
when calculating a worldwide normalisation reference for POPC.
6.7 References
Altenstedt, J. & Pleijel, K. 1998, POCP for individual VOC under European conditions. IVL Report B-1305, Swedish Environmental Research Institute, Stockholm.
Carter, W.P.L., Pierce, J.A., Luo, D. & Malkina, I.L. 1995, Environmental Chamber Study of maximum incremental reactivities of volatile organic compounds. Atmospheric Environment, 29 (18) pp.
2499-2511.
Derwent, R.G. 1996, Photochemical ozone creation potentials for a large number of reactive hydrocarbons under European conditions. Atmospheric Environment, 30 (2) pp. 181-199.
Derwent, R.G. & Jenkin, M.E. 1991, Hydrocarbons and the long-range transport of ozone and PAN across Europe. Atmospheric Environment, 25 (8) pp. 1661-1678.
Fenger, J. 1995, Ozon som luftforurening. DMU Tema-rapport 1995/3.
Hauschild, M. & Wenzel, H. 1998, Photochemical ozone formation as a criterion in the environmental assessment of products in Environmental assessment of products. Volume 2 Scientific background
eds. Hauschild. M. & Wenzel. H. London: Chapman & Hall.
McBride, S.J., Oravetz, M.A. & Russel, A.G. 1997, Cost-benefit and uncer.tainty issues in using organic reactivity to regulate urban ozone. Environm. Sci. Technol. 31 (5), pp. 138A-244A.
Olivier, J.G.J, Bouwman, A.F., van der Maas, C.W.M., Berdewski, J.J.M., Veldt, C., Bloos, J.P.J., Visschedijk, A.J.H., Zandveld, P.Y.J. & Haverlag, J.L. 1996, Description of EDGAR version 2.0: A
set of global emission inventories of greenhouse gasses and ozone-depleting substances for all anthropogenic and most natural sources on a per country basis and on 1ox1o grid. RIVM report
nr. 771060 002/TNO-MEP report nr. R96/119.
Ritter, M. 1997, CORINAIR 94 - Summary Report - European Emission Inventory for Air Pollutants. Copenhagen: European Environment Agency.
UN-ECE 1979, Convention on Long-range Transboundary Air Pollution. United Nations, Economic Commission for Europe. Available: http//:www.unece.org.
UN-ECE 1991, Protocol to the 1979 Convention on Long-range Transboundary Air Pollution concerning the control of emissions of volatile organic compounds or their transboundary fluxes.
United Nations, Economic Commission for Europe. Available: http//:www.unece.org.
Wenzel, H., Hauschild, M. & Alting, L. 1997, Environmental Assessment of Products, Vol. 1: Methodology, tools and case studies in product develop.ment. London: Chapman & Hall.
Appendix A.1: Calculation of normalisation reference for Denmark.
Total POCP calculated from anthropogenic NMVOC-, CO and CH4-emissions from Denmark.
|
Low-NOx factor |
DK 1994
VOC-
emission
|
Photochemical ozone-formation potential |
% distribu-
tion
|
Source category |
POCP |
kt/year |
kg C2H4/year |
POCP |
1. Combustion in Energy and transformation industries (nmVOC) |
0.4 |
1.4 |
0.5 |
0.5 |
2. Non-industrial combustion plants (nmVOC) |
0.4 |
8.2 |
3.3 |
3.2 |
3. Combustion in manufacturing industry (nmVOC) |
0.4 |
0.8 |
0.3 |
0.3 |
4. Production processes (nmVOC) |
0.3 |
3.4 |
1.0 |
1.0 |
5. Extraction and distribution of fossil fuels/geothermal energy (nmVOC) |
0.4 |
6.9 |
2.7 |
2.6 |
6. Solvent and other product use (nmVOC) |
0.5 |
40.6 |
20.3 |
19.6 |
7. Road transport (nmVOC) |
0.4 |
74.1 |
29.6 |
28.6 |
8. Other mobile sources and machinery (nmVOC) |
0.5 |
19.1 |
9.6 |
9.2 |
9. Waste treatment and disposal (nmVOC) |
0.4 |
0.0 |
0.0 |
0.0 |
10. Agriculture and Forestry, luwc (nmVOC) |
0.4 |
11.7 |
4.7 |
4.5 |
|
|
|
|
|
Total nmVOC |
|
166.1 |
72.1 |
69.5 |
|
|
|
|
|
CO (total) |
0.04 |
715.4 |
28.6 |
27.6 |
CH4 (total excluding "nature")* |
0.007 |
430.6 |
3.0 |
2.9 |
|
|
|
|
|
Total POCP, Denmark 1994 |
|
|
103.72 |
100.00 |
Population 1994 (Mio.) |
5,2 |
|
kg/capita/year |
kg C2H4/capita/year |
Emissions per capita (total NMVOC) |
31,9 |
13,9 |
Emissions per capita (CO) |
137,6 |
5,5 |
Emissions per capita (CH4 man made) |
82,8 |
0,6 |
|
|
|
Normalisation reference (1994) |
19,9 |
Appendix A.2: Calculation of normalisation reference for EU-15
Click here to see the Table.
|
Low-NOx countries |
High-NOx countries |
|
|
Low-NOx
countries
|
High-NOx
countries
|
Total EU-15 |
Population 1994 (Mio.) |
22.6 |
347.4 |
|
|
22.6 |
347.4 |
370.0 |
Emissions per capita (total nmVOC) (kg/capita/year) |
57.0 |
41.1 |
|
|
|
|
|
Emissions per capita (CO) (kg/capita/year) |
124.1 |
121.7 |
|
|
|
|
|
Emissions per capita (CH4 man made) (kg/capita/year) |
77.4 |
59.8 |
|
|
|
|
|
|
|
|
|
|
Low-NOx EU countries |
High-NOx EU countries |
Total EU-15 |
Normalisation reference (1994) (kg C2H4/capita/year) |
|
|
|
|
29.5 |
24.9 |
25.2 |
Normalisation reference (1994) (kg C2H4/capita/year)
excluding luwc |
|
|
21.3 |
22.1 |
22.1 |
1 CORINAIR 94 Summary report, Report from the European Topic Centre on Air Pollution (1997)
2 Low NOx countries include Denmark, Sweden, Finland and Ireland
3 75% of the CH4 emissions listed arise from agriculture and forestry
4 nmVOC contribution from luwc is 21 kg nmVOC/person/year in low-NOx countries, and in high-NOx countries only 6,8 kg nmVOC/person/year.
Appendix A.3: Calculation of a worldwide normalisation reference
Click here to see the Table.
7 Acidification
Leif Hoffmann, dk-TEKNIK ENERGY & ENVIRONMENT
7.1 Summary
This chapter summarises the presently available data on emissions of acidifying substances to the Danish and the European environment. The contributing emissions include:
- SOx - oxides of sulfur
- NOx - nitrogen oxides
- NH3 - ammonia
Emission data for these substances are generally available for Denmark and the European countries (EU-15) as well as number of other European countries. The normalisation references for acidification
are presented in Table 7-1. The worldwide normalisation references is calculated by extrapolation from the normalisation factor for EU-15.
Table 7-1
Summary of normalisation references for acidification for Denmark, EU-15 and Worldwide in 1994.
|
|
Denmark |
EU-15 |
Worldwide |
Acidification |
kg SO2-eq./capita/year |
101 |
74 |
59 |
SOx accounts for 30-45%, NOx for 30-35% and NH3 for 25-40% of the effect potential contributing to the normalisation reference. The normalisation reference is relatively certain as the relevant
emissions to air have been measured through many years according to the Convention on Long-range Transboundary Air Pollution and the following Protocols.
7.2 Description of the impact category
Acidification is a local and regional effect. Acidification is caused by releases of protons in the terrestrial or aquatic ecosystems. The acidifying substances are only contributing to acidification if the anion is
leached out from the system. If the anion stays in the system, natural processes usually neutralise the acid. In certain areas acidification leads to increased mobility of heavy metals and aluminium.
In the terrestrial ecosystem the effects are seen in softwood forests (e.g. spruce) but also in hardwood forests (e.g. beech) as inefficient growth and as a final consequence dieback of the forest. These
effects are mainly seen in Scandinavia and in the middle/eastern part of Europe. In the aquatic ecosystem the effects are seen as clear acid lakes without any wildlife. These effects are mainly seen in
Scandinavia. Buildings, constructions, sculptures and other objects worthy of preservation are also damaged by e.g. acid rain.
The potential effects are dependent on the acidification potential of the specific substance as well as the nature of the receiving ecosystem e.g. nitrogen oxides (NOx) can be fixed in the ecosystem due to
uptake in plants. This effect depends on the actual geographical area.
Acidifying substances can be divided in different groups according to their chemical nature: strong acids, acidic anhydrides, ammonia, and organic acids. Examples on strong acids are hydrochloric acid
(HCl), nitric acid (HNO3), and sulfuric acid (H2SO4) and they react with water as shown in the following example:
H2SO4 → 2H+ + SO42-
i.e. one mole of sulfuric acid release 2 mole of protons (H+).
Examples on acidic anhydrides are SO2, SO3, and NOx and they react as e.g.:
SO2 + H2O → H2SO3 → 2H+ + SO32-
i.e. one mole of sulfur dioxide release 2 mole of protons (H+).
Ammonia is normally considered as a base but in the presence of certain bacteria, ammonia is mineralised (nitrification) to nitrite and nitrate releasing protons:
NH3 + 2O2 H+ + NO3- + H2O
Organic acids are assumed to be mineralised rather than dissociated and leached. They are therefore not classified as contributors to acidification.
See also Hauschild and Wenzel (1998) for further description of the impact category.
7.3 Substances contributing to the impact category
Substances are considered to have an acidification effect if they result in (Hauschild & Wenzel 1998):
- Supply or release of hydrogen ions (H+) in the environment
- Leaching of the corresponding anions from the concerned system
The substances normally considered as contributors to acidification are:
- Sulfur Dioxide (SO2)
- Sulfur Trioxide (SO3)
- Nitrogen Oxides (NOx)
- Hydrogen Chloride (HCl)
- Nitric Acid (HNO3)
- Sulfuric Acid (H2SO4)
- Phosphoric Acid (H3PO4) (note: the anion does not leach and the contribution to acidification is in practice equal to zero)
- Hydrogen Fluoride (HF)
- Hydrogen Sulfide (H2S)
- Ammonia (NH3)
SO2 is used as basis for determination of the acidification potential or the equivalence factor. The method of establishing effect factors for acidifying substances is based on stoichiometric considerations
and it is internationally accepted. The equivalency factors are determined as:
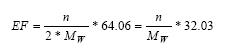
where MW is the molecular weight of the substance emitted [g/mole]
n is the number of hydrogen ions released in the recipient as a result of conversion of the substance
64.06 g/mole is the molecular weight of SO2
The equivalency factors for the acidifying substances are compiled in Table 7-2.
Table 7-2
Equivalency factors (EF) for acidifying substances [kg SO2-eq./substance] (Hauschild & Wenzel, 1998).
Substance |
Formula |
Reaction |
Molar weight
g/mole
|
n |
EF
kg SO2/kg
|
Sulfur dioxide |
SO2 |
SO2+H2OH2SO3→2H++SO32- |
64.06 |
2 |
1 |
Sulfur trioxide |
SO3 |
SO3+H2OH2SO4→2H++SO42- |
80.06 |
2 |
0.80 |
Nitrogen dioxide |
NO2 |
NO2+½H2O+¼O2→H++NO3H- |
46.01 |
1 |
0.70 |
Nitrogen oxide |
NOx1 |
NO2+½H2O+¼O2→H++NO3H- |
46.01 |
1 |
0.70 |
Nitrogen oxide |
NO |
NO+O3+½H2OH++NO3-+¾O2 |
30.01 |
1 |
1.07 |
Hydrogen chloride |
HCl |
HCl→H++Cl- |
36.46 |
1 |
0.88 |
Hydrogen nitrate |
HNO3 |
HNO3→H++NO3- |
63.01 |
1 |
0.51 |
Hydrogen sulfate |
H2SO4 |
H2SO4→2H++SO42+ |
98.07 |
2 |
0.65 |
Hydrogen phosphate |
H3PO4 |
H3PO4→3H++PO43- |
98.00 |
3 |
0.98 |
Hydrogen fluoride |
HF |
HF→H++F- |
20.01 |
1 |
1.60 |
Hydrogen sulfide |
H2S |
H2S+3/2O2+H2O→2H++SO32- |
34.03 |
2 |
1.88 |
Ammonium |
NH3 |
NH3+2O2→H++NO3-+H2O |
17.03 |
1 |
1.88 |
1 x is assumed to have an average value of 2.
The acidification potential (AP) can be estimated as SO2-equivalents:
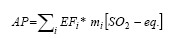
where EFi is the equivalency factor for the substance i
mi is the emission of the substance i
7.4 Methodology
The calculation of the normalisation reference for acidification has been carried out according to the methodology described in (Hauschild & Wenzel, 1998). The normalisation reference is calculated as:

where: Normrefacid is the normalisation reference for acidification
mi is emitted quantity of the substance i
EFi is the equivalence factor for the substance i
N is number of capita in the considered area
Included in the normalisation reference are the following substances:
- Sulfur Dioxide (SO2)
- Nitrogen Oxides (NOx)
- Ammonia (NH3)
as the substances are the only substances considered in the international regulations of acidifying substances.
7.5 Normalisation reference
7.5.1 Denmark
The emission data used in the calculation of the normalisation reference is presented in Table 7-3.
Table 7-3
Danish emission of acidifying substances in 1990 and 1994.
Substance |
Formula |
EF |
Emission |
|
Impact potential |
|
|
|
g SO2/g |
19901
kt/year
|
19942
kt/year
|
1990
kt SO2/year
|
1994
kt SO2/year
|
Oxides of sulfur |
SOx |
1 |
180 |
158 |
180 |
158 |
Nitrogen oxides |
NOx |
0.7 |
269 |
276 |
188 |
193 |
Ammonia |
NH3 |
1.88 |
140 |
122 |
263 |
176 |
Hydrogen chloride |
HCl |
0.88 |
3.8 |
- |
3.3 |
- |
Total |
|
|
|
|
634 |
527 |
1 Hauschild and Wenzel (1998).
2 Ritter (1997).
The Danish normalisation reference for acidification (1994) can be calculated as:
101 kg SO2-equivalents/year/capita
The similar value for 1990 was 124 kg SO2-equivalents/year/capita. This means that the normalisation factor is reduced by 20%.
The contribution of acidifying impact from the four substances included in the 1990 normalisation factor is 28%, 30%, 41%, and <1% for SOx, NOx, NH3, and HCl, respectively. In 1994 the distribution
can be calculated to 30%, 37%, and 33% for SOx, NOx, and NH3 respectively.
The quality of the data used for calculation of the Danish normalisation reference is expectedly of high quality, as the registration of the actual emissions has been performed in a number of years. According
to Ritter (1997) the emission estimates are made on highest level e.g. level A (the emission estimates are fully detailed).
7.5.1.1 EU-15
The emission data used in the calculation of the normalisation reference is presented in Table 7-4. Emission data for approximately forty European countries is presented in Appendix B.
Table 7-4
European emission of acidifying substances in 1990 and 1994.
Substance |
Formula |
EF |
Emission |
|
Impact potential |
|
|
|
g SO2/g |
19901
kt/year
|
19942
kt/year
|
1990
EU-12
kt SO2/year
|
1994
EU-15
kt SO2/year
|
Oxides of sulfur |
SOx |
1 |
12,450 |
12,000 |
12,450 |
12,047 |
Nitrogen oxides |
NOx |
0.7 |
11,960 |
12,500 |
8,372 |
8,781 |
Ammonia |
NH3 |
1.88 |
3,188 |
3,530 |
5,993 |
6,645 |
Total |
|
|
|
|
26,815 |
27,473 |
1 Hauschild and Wenzel (1998).
2 Ritter (1997).
The European (EU-15) normalisation reference for acidification (1994) can be calculated as:
74 kg SO2-equivalents/year/capita
The similar value for 1990 was 83 kg SO2-equivalents/year/capita. This means that the normalisation factor is reduced by 10%.
The contribution of acidifying impact from the three substances included in the 1994 normalisation factor is 44%, 32%, and 24% for SOx, NOx, and NH3 respectively. Normalisation references for the
fifteen European countries included in EU-15 are presented in Appendix C.
The quality of the data used in the calculation of the European normalisation reference depends on the actual countries as the emission estimates are provided by the national authorities/national reference
centres. Similar data can be found in a number of sources all based on the same national information. The CORINAIR report inform about the status of the presented emission estimates, and the 1994
emission are assessed as follows:
- A (the emission estimates are fully detailed): Austria, Belgium, Denmark, Greece, Ireland, Luxembourg, Sweden, United Kingdom
- B (the emission estimates are detailed): Germany, Netherlands
- C (the emission data cover the main source sectors): Finland, France, Italy, Portugal, Spain
According to the update report including 1995 emissions (Koch 1998), the assessment of the status of the estimates are decreased for two countries and the data are missing for four countries at the time of
publication. The process of making and publishing the emission estimates is continuous i.e. the national estimates are improved when better emission factors become available.
7.5.2 World
Acidification has been used as case for investigation and illustration of relations between normalisation factors and different general available data as economical data, population, and production data etc.
(see chapter 3, Development of normalisation references for different geographic areas, for details). By testing different relations between kg SO2-eq./capita/year and the considered factors the best relation
is shown to be kg SO2-eq./capita/.year vs. ln(GDP/capita). This extrapolation method results in the following worldwide normalisation reference for acidification:
59 kg SO2-eq./capita/year
The quality of the data used as basis for the extrapolation method is described in chapter 3.
7.5.3 Summary
The normalisation references for acidification covering different geographical areas compared with the previous normalisation references; see Table 7-5.
Table 7-5
Summary of normalisation references for acidification for different areas in 1990 and 1994.
|
|
Denmark |
EU-15 |
World |
19901 |
kg SO2-eq./capita/year |
124 |
83 |
- |
1994 |
kg SO2-eq./capita/year |
101 |
74 |
59 |
1 Hauschild and Wenzel (1998).
The normalisation references for Denmark and EU-15 are decreased by 10 - 20% in the years 1990 to 1994.
7.6 Recommendations for future update
As mentioned above the information on and quality of emissions depend on the national estimates. The limit for improvement of the normalisation references is the speed of national collection/estimation of
emissions. The national reporting to e.g. European Topic Centre on Air Emissions (ETC Air Emissions) or the Co-operative programme for monitoring and evaluation of the long range
transmission of air pollutants in Europe (EMEP) is also a limiting factor. These centres collect and evaluate national information on air pollutants according to the Convention on Long-range
Transboundary Air Pollution (UN-ECE 1979). Potential data sources to be used in future updating of the normalisation reference for acidification is compiled in Appendix A.
7.7 References
Hauschild, M. & Wenzel, H. 1998, Acidification as a criterion in the environmental assessment of products in Environmental assessment of products. Volume 2 Scientific background eds. Hauschild,
M. & Wenzel, H. London: Chapman & Hall.
Koch, D. 1998, Air emissions - Annual topic update 1997. Topic Report no.4. European Environment Agency.
Ritter, M. 1997, CORINAIR 94 - Summary Report - European Emission Inventory for Air Pollutants. Copenhagen: European Environment Agency.
UN-ECE 1979, Convention on Long-range Transboundary Air Pollution. United Nations, Economic Commission for Europe. Available: http//:www.unece.org.
Appendix A: Data sources
The substances contribution to acidification (SO2, NOx, and NH3) are all systematically registered due to different conventions on air pollution (e.g. "Convention on Long-range Transboundary Air
Pollution" (UN-ECE 1979)). Therefore, these emissions can be expected to be updated regularly e.g. yearly for most of the countries. The data sources can be divided in databases (paper) databases
(electronic), and organisations.
Databases (paper)
- CORINAIR reports (until now 1990 and 1994 data).
- Topic reports. Air emissions - Annual Topic Update. Reports made by European Topic Centre on Air Emissions (ETC Air Emissions) and published by European Environment Agency.
Databases (electronic)
- CORINAIR database - expected to contain data updated yearly (National as well as European databases can be expected to be public available in the future.); http://www.aeat.co.uk/netcen.
- EMEP - Tables of anthropogenic emissions in the ECE region (SO2, NOx, NH3. http://www.emep.int.
Organisations
- EMEP - co-operative programme for monitoring and evaluation of the long range transmission of air pollutants in Europe. Contact:
Det norske meteorologiske institutt (DNMI)
Postboks 43, Blindern,
0313 Oslo
Phone 47 22 96 30 00
Fax 47 22 96 30 50
e-post: met.inst@dnmi.no
Homepage: http:/www.dnmi.no
- ETC Air Emissions
Umweltbundesamt UBA
Contact person: Dietmar Koch
Bismarckplatz 1
D-14193 Berlin
Germany
Phone: [49] 30 8903 2392
Fax: [49] 30 8903 2178
E-mail: dietmar.koch@uba.de
Homepage: http://www.aeat.co.uk/netcen.
- National Reference Centres for Air Emissions
Appendix B: Emission data
The date presented in the table is based on Ritter (1997)/Eurostat (1998) except where other references are given.
|
Population |
SO2
ton/year
|
NOx
ton/year
|
NH3
ton/year
|
Denmark, 19901 |
5,14E+06 |
1,80E+05 |
2,69E+05 |
1,40E+05 |
Denmark, 1994 |
5,20E+06 |
1,58E+05 |
2,76E+05 |
9,36E+04 |
Austria |
8,00E+06 |
5,49E+04 |
1,71E+05 |
8,62E+04 |
Belgium |
1,01E+07 |
2,79E+05 |
3,74E+05 |
7,89E+04 |
Finland |
5,10E+06 |
1,11E+05 |
3,88E+05 |
4,10E+04 |
France |
5,77E+07 |
1,01E+06 |
1,68E+06 |
6,67E+05 |
Germany |
8,11E+07 |
3,00E+06 |
2,27E+06 |
6,23E+05 |
Greece |
1,04E+07 |
5,56E+05 |
3,57E+05 |
4,45E+05 |
Ireland |
3,60E+06 |
1,77E+05 |
1,17E+05 |
1,25E+05 |
Italy |
5,70E+07 |
1,44E+06 |
2,16E+06 |
3,89E+05 |
Luxembourg |
4,00E+05 |
1,28E+04 |
2,26E+04 |
7,12E+03 |
Netherlands |
1,53E+07 |
1,46E+05 |
5,29E+05 |
1,72E+05 |
Portugal |
9,90E+06 |
2,73E+05 |
2,49E+05 |
9,26E+04 |
Spain |
3,91E+07 |
2,06E+06 |
1,22E+06 |
3,44E+05 |
Sweden |
8,70E+06 |
7,42E+04 |
4,44E+05 |
5,06E+04 |
UK |
5,82E+07 |
2,70E+06 |
2,39E+06 |
3,20E+05 |
EU15 |
3,70E+08 |
1,20E+07 |
1,25E+07 |
3,53E+06 |
Albania |
3,38E+06 |
5,50E+04 |
2,40E+04 |
3,10E+04 |
Armenia |
3,63E+06 |
4,00E+03 |
4,00E+03 |
0,00E+00 |
Azerbaijan |
7,53E+06 |
0,00E+00 |
0,00E+00 |
0,00E+00 |
Belarus |
1,04E+07 |
3,24E+05 |
2,03E+05 |
4,00E+03 |
Boz&Herz |
3,57E+06 |
3,20E+04 |
1,30E+04 |
3,10E+04 |
Bulgaria |
8,51E+06 |
1,48E+06 |
2,30E+05 |
1,46E+05 |
Croatia |
4,51E+06 |
8,90E+04 |
5,90E+04 |
3,30E+04 |
Cypres |
0,00E+00 |
4,60E+04 |
2,10E+04 |
0,00E+00 |
Czech Rep. |
1,03E+07 |
1,27E+06 |
4,35E+05 |
9,20E+04 |
Estonia |
1,49E+06 |
1,41E+05 |
4,30E+04 |
1,40E+04 |
FYROM |
2,16E+06 |
1,06E+05 |
3,90E+04 |
1,70E+04 |
Georgia |
5,45E+06 |
0,00E+00 |
0,00E+00 |
0,00E+00 |
Hungary |
1,01E+07 |
7,41E+05 |
1,87E+05 |
1,60E+05 |
Iceland |
2,69E+05 |
2,40E+04 |
2,90E+04 |
3,00E+03 |
Latvia |
2,54E+06 |
5,10E+04 |
4,50E+04 |
1,60E+04 |
Lichtenstein |
3,10E+04 |
0,00E+00 |
1,00E+03 |
0,00E+00 |
Lithaunia |
3,74E+06 |
1,17E+05 |
7,70E+04 |
8,00E+04 |
Malta |
3,67E+05 |
0,00E+00 |
0,00E+00 |
0,00E+00 |
Moldova |
4,44E+06 |
9,90E+04 |
1,80E+04 |
0,00E+00 |
Norway |
4,33E+06 |
3,40E+04 |
2,22E+05 |
2,50E+04 |
Poland |
3,86E+07 |
2,61E+06 |
1,11E+06 |
3,84E+05 |
Romania |
2,27E+07 |
9,12E+05 |
3,49E+05 |
2,21E+05 |
Russian Fed. |
1,48E+08 |
2,98E+06 |
2,00E+06 |
7,72E+05 |
Slovak Rep. |
5,34E+06 |
2,40E+05 |
3,10E+05 |
0,00E+00 |
Slovenia |
1,93E+06 |
1,77E+05 |
6,60E+04 |
2,20E+04 |
Switzerland |
7,17E+06 |
3,10E+04 |
1,39E+05 |
6,00E+04 |
Turkey |
6,08E+07 |
3,54E+05 |
1,75E+05 |
0,00E+00 |
Ukraine |
5,18E+07 |
1,72E+06 |
5,68E+05 |
1,50E+04 |
1 Hauschild and Wenzel (1998).
Appendix C: Normalisation references for EU-15 countries
Country |
Normalisation reference
kg SO2-eq./person/year
|
Reference |
Austria |
42 |
1 |
Belgium |
68 |
1 |
Denmark 1994 |
101 |
1 |
Finland |
90 |
1 |
France |
60 |
1 |
Germany |
71 |
1 |
Greece |
158 |
1 |
Ireland |
137 |
1 |
Luxembourg |
105 |
1 |
Netherlands |
55 |
1 |
Portugal |
63 |
1 |
Spain |
91 |
1 |
Sweden |
55 |
1 |
UK |
85 |
1 |
EU-15 | 74 | 1 |
1 Ritter (1997).
8 Nutrient enrichment
Jørgen Larsen, Danish Technological Institute
8.1 Summary
The actual emissions for the same reference year, 1994, are inventoried for all environmental impact categories.
In addition to point source loading, nutrients are also input to inland and marine waters by diffuse loading and atmospheric deposition. The normalisation references are shown in Table 8-1.
Table 8-1
Normalisation references for nutrient enrichment for Denmark, EU-15, and worldwide.
|
Denmark |
EU-15 |
Europe-24 |
Worldwide |
kg N-equivalents/capita/year |
53 |
24 |
24 |
19 |
kg P-equivalents/capita/year |
0.8 |
0.4 |
0.4 |
0.3 |
kg NO3--equevalents/capita/year |
260 |
119 |
119 |
95 |
The most significant diffuse sources of nutrient input to the aquatic environment are leaching of nitrogen from cultivated land and the deposition from the atmosphere of gaseous nitrogen compounds.
Because of the ongoing reduction in direct point source discharges, diffuse loading and atmospheric deposition will eventually come to account for a relatively greater share of inputs to inland and marine
waters, and hence be of correspondingly greater importance for the state of the environment.
8.2 Description of the impact category
The injurious effects of extensive quantities of nutrient salts have been observed regularly in lakes for many years. However, the discovery that the bottom of large bodies of water was practically oxygen-free
and lifeless in some part of the year came as an unpleasant surprise to many people and triggered serious discussion of nutrient salt emissions and their potential impact on the environment. In connection with
the 1987 Action Plan on the Aquatic Environment, a Danish nation-wide programme was established to monitor nutrient loading of aquatic areas from point sources, agriculture and the atmosphere to enable
the expected reduction in loading to be followed. By point sources are understood discharges from sewage works, separate industrial discharges, storm water outfalls, freshwater, marine and terrestrial
saltwater fish farms, and sparsely built-up areas.
8.2.1 Cause of oxygen depletion
The cause of oxygen depletion found in the bottom layers of lakes and coastal waters is nutrient enrichment. It can thus be defined as "an enrichment of the aquatic environment with nutrient salts leading to an
increased production of planktonic algae and higher aquatic plants, which in time leads to a reduction in the water quality and in the value of the exploitation which occurs in the area" (Christensen et al.
1993). The algae sink to the bottom and are broken down consuming oxygen in the bottom layer. If fresh oxygen-rich water from the surface does not reach the bottom layers, the oxygen concentration near
the bottom will gradually be reduced until the bottom-dwelling organisms move away or die.
The classification step defines nutrient enrichment as the man-made impact on aquatic or terrestrial systems of nitrogen, N, or phosphorus, P.
The total nutrient enrichment potential expresses the emissions as an equivalent emission of the reference substance NO3-.
The total impact potentials of the Danish emissions of nutrient salts in 1994 are used as reference for the normalisation of the potentials for nutrient enrichment. The normalisation references are presented as
the average nutrient enrichment potential per Dane.
In a tripartite division of environmental impact categories into global, regional and local, nutrient enrichment is designated a local and a regional impact. For local and regional impact categories, the Danish
or European emissions and populations are used to calculate normalisation references. To apply a comparable scale to impact potentials for the global impact categories (based on estimated global
emissions) and the regional impact categories (based on Danish or European emissions), the environmental impact potentials found as the background impact are divided by the population of the region for
which they were calculated. The environmental impact potential is thus expressed as the person-equivalent, i.e. the average contribution from each individual person in the area concerned.
8.3 Substances contributing to the impact category
8.3.1 Macro-nutrients
One of the two macro-nutrients nitrogen and phosphorus is usually the limiting element for the growth of primary producers, and it is therefore reasonable to consider only the elements nitrogen and
phosphorus as contributors to nutrient enrichment. In Danish lakes, phosphorus deficiency, or a combination of nitrogen and phosphorus deficiencies, is typically limiting for growth, and their addition
promotes algae growth. In Danish coastal waters and seas, nitrogen is often the limiting nutrient. Substances containing nitrogen or phosphorus in a biologically available form are therefore classified as
potential contributors to nutrient enrichment.
Three equivalence factors are defined for use in the calculation of the potential contributions from a given substance:
- the N potential, which expresses the substance's nitrogen content,
- the P potential, which expresses the substance's phosphorus content, and
- the equivalence factor for the total nutrient enrichment potential, where the nitrogen and phosphorus contents are aggregated in a figure based on the assumption of an average ratio of 16:1 between the
nitrogen and phosphorus contents in aquatic organisms.
8.3.2 Reference substance
The total nutrient enrichment potential expresses the emissions as an equivalent emission of the reference substance NO3-.
As it is evident from the formula on the average composition of aquatic organisms, the ratio of nitrogen to phosphorus is of the order of 16. If the concentration of bio-available nitrogen is significantly more
than 16 times the concentration of bio-available phosphorus in an ecosystem, it is thus reasonable to assume that phosphorus is the limiting nutrient and vice versa. Since most of the atmosphere consists of
free nitrogen, N2, further addition of N2 will not have any effect. N2 is therefore not classified as contributor to nutrient enrichment. The most important airborne emissions include oxides of nitrogen, NOx,
which are predominantly emitted from incineration processes, and ammonia, NH3, which is especially emitted from agricultural activities. Airborne emissions of phosphate are quite small and are therefore
not included in the calculations.
Most of the nitrogen loading to the aquatic environment is mainly attributable to leaching from the root zone of agricultural land. An important part of the nitrogen loading is related to the use of nitrogen
fertilisers and the number of livestock. Apart from the man-made emissions from agriculture an important loading of nitrogen comes from various point sources such as waste water treatment plants,
industry, fish farming and from sparsely built-up areas.
Most of the phosphorus loading of surface water is attributable to discharges from point sources, especially municipal sewage and industrial effluent. Only a small part of the industrial sector is responsible for
the majority of wastewater containing phosphorus. To name just a few important industries the fertiliser industry and other related chemical industries manufacturing products containing phosphorus (e.g.
pesticides and detergents), as well as the pulp and paper industry and fish processing industry should be mentioned. However, leaching from agricultural land also plays a significant part.
8.4 Updating of the normalisation reference for Denmark
For nutrient enrichment, the Danish emissions of N and P are used as normalisation references. These are summarised, and their environmental impact potentials calculated in Table 8-2.
8.4.1 Nitrogen loading to the aquatic environment
The total loading of the Danish aquatic environment with nitrogen and phosphorus in 1994 is summarised by the Danish National Environmental Research Institute (Larsen et al. 1995).
Part of this loading is emitted via the fresh waters and some of the nitrogen is removed on the way to the marine systems by denitrification in watercourses and lakes. Loading of the fresh waters with
nitrogen is thus greater than the quantity conveyed to the marine areas via the watercourses.
It has not been possible to find comprehensive accounts for losses on its way towards the sea and thus for the loading of fresh waters. However, it could easily be a third greater than the quantity of nitrogen
added to the marine areas via watercourses (Kirkegaard 1995).
With this reservation, the normalisation reference for nitrogen loading is based on the figures for loading of the marine areas in 1994:
- 119.100 kt N/year from watercourses and
- 9.300 kt N/year from direct emissions (Larsen et al. 1995; Miljøstyrelsen 1995)
The direct emissions are man-made, but apart from the man-made emissions from agriculture and various point sources such as waste water treatment plants, industry and fish farming, the loading from
watercourses also includes some natural leaching of nitrogen.
It is necessary for normalisation to know the anthropogenic loading. The natural background loading with nitrogen is estimated to be about 12% (Larsen et al. 1995). Thus, 88% of the waterborne
emissions of nitrogen are man-made.
This gives a total man-made waterborne loading with nitrogen in 1994 of:
- 0.88 x 119.100 kt N/year + 9.300 kt N/year = 114.108 kt N/year
8.4.2 Diffuse nitrogen loading from cultivated land
Nitrogen loading of groundwater and surface water is mainly attributable to leaching from the root zone of agricultural land. An important step towards reducing nitrogen leaching is that storage capacity for
animal fertiliser has been expanded considerably. Measurements and calculations of actual nitrogen leaching from the root zone have revealed considerable inter-annual variation. Measurements of nitrogen
loss to watercourses reveal correspondingly large variation that is also attributable to climatic variation. Diffuse nitrogen loading is closely tied to the amount of precipitation that falls in the winter half year.
As a consequence, the general decline in point source loading is blurred by the climatic variation in diffuse loading, especially that from cultivated land. It should be noted that the mean precipitation in
Denmark was 880 mm in 1994, which is more than normal.
It is estimated that changes in agricultural practice (that the storage capacity for animal fertiliser has been expanded considerably) have led to a small reduction in nitrogen leaching from the root zone.
However, it has not been possible to demonstrate this directly by means of measurements. In this connection, it should be noted that the majority of the measures decided in the "Action Plan on the Aquatic
Environment" and the "Action Plan for Sustainable Agricultural Development" have now been implemented. Any further reduction in nitrogen loss as a result of these changes in agricultural practice is
therefore likely to be limited (Miljøstyrelsen 1997).
8.4.3 Air emissions of nitrogen
A large part of nutrient input to Danish marine waters takes place via the atmosphere.
In the present study, the airborne emissions of nitrogen compounds are used for the normalisation references because it is assumed that the emissions have the potential to contribute to the nutrient
enrichment. The airborne emissions of nitrogen compounds inventoried for Denmark in Table 8-2 include oxides of nitrogen, NOx, which is emitted predominantly from incineration processes, and ammonia,
NH3, which is emitted especially from agricultural activities.
The goal, as stipulated in the EU declaration on the NOx Protocol, is to reduce Danish emissions from the 1986 level of 312,000 tonnes to 218,000 in 1998, corresponding to a reduction of approx. 30%.
International agreements to reduce NOx emissions and national endeavours to reduce ammonia volatilisation will, in the long term, help reduce atmospheric deposition on Danish marine waters.
Danish emissions of nitrogen compounds to air in 1994 are estimated to 159.9 kt N/year. Airborne emissions of nitrogen and phosphate from "nature" are small and in the calculations we assume that 100%
are man-made.
8.4.4 Phosphorus loading to the aquatic environment
The Aquatic Environment Plan's monitoring programme 1994 gives the total loading with phosphorus as
- 2.960 kt P/year from watercourses and
- 1.530 kt P/year from direct emissions (Larsen et al. 1995)
In contrast to nitrogen, there are no natural removal mechanisms for phosphorus. However, the emission of phosphorus in watercourses typically occurs in pulses, as phosphorus accumulates in the
watercourses sediment during drier periods and is then completely washed out into marine environment when the water flow increases, e.g. after a thunderstorm. This type of pulse emission is
difficult to include in a monitoring, and the emission of phosphorus in watercourses is therefore believed to have been underestimated (Kirkegaard 1995). It has not been possible to obtain any
estimate of how large the emission of phosphorus to marine areas actually is. However, the composition of raw sewage has changed during the last 5 years. The change concerns the phosphorus
content of the sewage, which has decreased significantly due to greater use of phosphate-free detergents.
It is estimated that 84% (Larsen et al. 1995) of the waterborne emissions of phosphorus are man-made. This gives a total man-made waterborne phosphorus loading in 1994 of:
- 0.84 x 2.960 kt P/year + 1.530 kt P/year = 4.016 kt P/year.
8.4.5 Airborne emissions of phosphate
Airborne emissions of phosphate are quite small and are therefore not included in the calculations.
8.4.6 Danish emissions of N and P
Total man-made riverine nutrient loading of marine waters in 1994 is estimated at approx. 104.808 kt nitrogen and 2.486 kt phosphorus. The overall trend in loading of inland waters is a decrease in both
nitrogen and phosphorus loading from point sources.
Direct discharges to marine waters from point sources amounted in 1994 to 9.300 kt nitrogen and 1.530 kt/phosphorus. A decrease has also been seen in both nitrogen and phosphorus loading from point
sources. Point source loading has decreased considerably since 1990. This is primarily attributable to the requirements stipulated in the Action Plan on the Aquatic Environment with regards to reducing
discharges from municipal sewage works and industrial outfalls (Miljøstyrelsen 1994).
Nearly all emissions of nitrogen to the air are assumed to be man-made and are estimated to consist of 159.9 kt N/year.
Total loading of marine waters in 1994 from watercourses, direct point-source discharges and atmospheric deposition is calculated to be approx. 274 kt nitrogen and approx. 4 kt phosphorus.
Signs of reduced primary production of plankton and algae are seen in many areas, together with improved oxygen conditions. In general, however, no major changes have been detected in the level of
nutrient enrichment in Danish marine waters relative to 1990 (Hauschild & Wenzel 1998).
Table 8-2
Danish emissions of nutrients in the form of nitrogen and phosphorus compounds to air and water. The unit is kt/year. Emissions to air from Ritter (1997); waterborne emissions from Larsen et al. (1995).
Substance |
Formula |
1994 |
EF(N) |
EF(P) |
Impact potential
1994
|
|
|
kt/year |
g N-eq./g |
g P-eq./g |
kt N-eq./
year
|
kt P-eq./
year
|
Airborne emissions |
Nitrogen oxides |
NOx |
276 |
0.30 |
0 |
82.8 |
0 |
Ammonia |
NH3 |
94 |
0.82 |
0 |
77.1 |
0 |
Waterborne emissions |
Total P |
PO43- -P |
4.016 |
0 |
1 |
0.0 |
4.0 |
Total N |
N |
114.108 |
1 |
0 |
114.1 |
0 |
Total |
|
|
|
|
274.0 |
4.0 |
NOx as NO2
8.4.7 Normalisation references for 1994
Based on the total Danish emission (1994) of 274 kt N-equivalents, and 4 kt P-equivalents and a population (1994) of 5.2 million (Ritter 1997), the Danish normalisation references for nutrient enrichments
are:
Person equivalents:
53 kg N-equivalents/capita/year
0.8 kg P-equivalents/capita/year
or aggregated as
260 kg NO3- -equivalents/capita/year
8.5 The normalisation reference for EU-15
For the normalisation reference for EU-15, the emissions of nutrients are estimated from the EU-15 countries. These are summarised, and their environmental impact potentials calculated, in Table 8-9. In
the following sections, the basic data are presented in some detail.
8.5.1 Air emissions of nitrogen and phosphorus compounds
A large part of the nutrient input to marine waters takes place via the atmosphere. Nutrient enrichment via the atmosphere primary depends on the emissions of NOx and NH3. The total emissions for the
EU-15 countries in 1994 are shown in Table 8-3.
Table 8-3
National total1 emissions 1994 (Ritter 1997).
Country |
Population
(Mio)
|
NOx
(kt/year)
|
NH3
(kt/year)
|
Germany |
81.1 |
2,266 |
623 |
United Kingdom |
58.2 |
2,387 |
320 |
France |
57.7 |
1,682 |
667 |
Italy |
57.0 |
2,157 |
389 |
Spain |
39.1 |
1,223 |
344 |
Netherlands |
15.3 |
530 |
172 |
Greece |
10.4 |
357 |
445 |
Belgium |
10.1 |
374 |
79 |
Portugal |
9.9 |
249 |
93 |
Sweden |
8.7 |
444 |
51 |
Austria |
8.0 |
171 |
86 |
Denmark |
5.2 |
276 |
94 |
Finland |
5.1 |
288 |
41 |
Ireland |
3.6 |
117 |
125 |
Luxembourg |
0.4 |
23 |
7 |
EU-15 |
370 |
12,544 |
3,535 |
1 National total emissions follow the UN-ECE/EMEP guidelines.
However, emissions from sector "nature" are excluded to ensure
inter country comparison.
According to UN-ECE/EMEP requirements, sources of emissions to air have to be reported according to 11 main source sectors. The emissions in the EU-15 countries from these 11 main source sectors
are given in Table 8-4.
Table 8-4
EU-15 main source sectors 1994 (Ritter 1997).
Sector |
|
NOx
(kt/year)
|
NH3
(kt/year)
|
1 |
Combustion in energy and transformation industries |
2,400.3 |
3.3 |
2 |
Non-industrial combustion plants |
552.9 |
1.3 |
3 |
Combustion in manufacturing industry |
1,184.7 |
1.7 |
4 |
Production processes |
215.2 |
100.9 |
5 |
Extraction and distr. of fossil fuels/geothermal energy |
113.5 |
- |
6 |
Solvent and other product use |
0.6 |
2.0 |
7 |
Road transport |
6,052.0 |
32.1 |
8 |
Other mobile sources and machinery |
1,892.8 |
0.1 |
9 |
Waste treatment and disposal |
96.9 |
36.0 |
10 |
Agriculture and forestry, land use and wood stock change |
34.5 |
3,357.7 |
11 |
Nature |
19.7 |
0.5 |
|
EU-15 total1 |
12,544.4 |
3,534.6 |
1 The EU-15 totally excludes emissions from sector "nature" (SNAP 11).
As shown in Table 8-4 the emission of nitrogen to the air from the sector "nature" constitutes less than 1% of the total emissions, therefore the exclusion of the emissions from "nature" is not assumed to
course significant errors.
Airborne emissions of phosphate are quite small and are therefore not included in the calculations.
8.5.2 Direct discharges and riverine inputs of total nitrogen and phosphorus compounds
The direct discharges and riverine inputs of total nitrogen and phosphorus into the different sea areas surrounding Europe are depicted in the Table 8-5 and Table 8-6. All data are finally aggregated in
Table 8-8, which illustrate the total direct discharges and riverine inputs to the sea of total nitrogen and phosphorus in the 15-EU countries.
Table 8-5
Direct discharges and riverine inputs of total nitrogen and total
phosphorus into the OSPAR area (kt/year)1 in 1994, Estimated from
EEA (1998a) and Excessive anthropogenic nutrients in European
ecosystems, EEA (in press).
Country |
Total Nitrogen
(kt/year)
|
Total Phosphorus
(kt/year)
|
Germany |
355.0 |
12.5 |
United Kingdom |
376 |
36 |
France |
67 |
- |
Netherlands |
490.0 |
27.5 |
Belgium |
47 |
2.0 |
Portugal |
15.7 |
14.2 |
Sweden |
6.9 |
0.3 |
Denmark |
74.1 |
2.2 |
Ireland |
179.1 |
10.5 |
EU-15 |
1610.8 |
105.2 |
1 High estimates used; some countries have given low and high estimates.
Table 8-6
Direct discharges and riverine inputs of total nitrogen and total
phosphorus into the Baltic Sea area (kt/year)1 in 1995, Estimated
from EEA (1998a) and Excessive anthropogenic nutrients in European
ecosystems, EEA (in press). These data are estimates from 1995 and
not from 1994; however, the error caused by this factor is expected to
be of minor importance.
Country |
Total Nitrogen
(kt/year)
|
Total Phosphorus
(kt/year)
|
Germany |
21.4 |
0.6 |
Sweden |
130.9 |
4.7 |
Denmark |
66.5 |
2.3 |
Finland |
66.1 |
3.6 |
EU-15 |
284.9 |
11.2 |
1 High estimates used; some countries have given low and high estimates.
Table 8-7
Estimated direct discharges and riverine inputs of total nitrogen
and total phosphorus into the Mediterranean Sea from the
EU-15 countries (kt/year) (Kristensen 1999).
Country |
Total Nitrogen
(kt/year)
|
Total Phosphorus
(kt/year)
|
Spain |
185 |
11 |
France |
118 |
8 |
Italy |
346 |
29 |
Greece |
117 |
7 |
EU-15 |
766 |
55 |
The Mediterranean Sea atmospheric inputs of nitrogen have been estimated to be about 1249 k tonnes/year, which are greater than those from direct discharges and riverine inputs.
Table 8-8
Direct discharges and riverine inputs to the sea of total nitrogen
and phosphorus in 15-EU countries (kt/year)1 in 1994. Estimated
from EEA (1998a) and Excessive anthropogenic nutrients in
European ecosystems, EEA (in press).
Country |
Total Nitrogen
(kt/year)
|
Total Phosphorus
(kt/year)
|
Germany |
376.4 |
13.1 |
United Kingdom |
376 |
36 |
France |
185 |
8 |
Italy |
346 |
29 |
Spain |
185 |
11 |
Netherlands |
490.0 |
27.5 |
Greece |
117 |
7 |
Belgium |
47 |
2.0 |
Portugal |
15.7 |
14.2 |
Sweden |
137.8 |
5 |
Austria |
|
|
Denmark |
140.6 |
4.5 |
Finland |
66.1 |
3.6 |
Ireland |
179.1 |
10.5 |
Luxembourg |
|
|
EU-15 |
2661.7 |
171.4 |
1 High estimates used; some countries have given low and high estimates.
8.5.3 EU-15 emissions of nutrients to air and water
It is necessary for normalisation to know the man-made loading, but the inventory published in "Excessive anthropogenic nutrients in European ecosystems" from the EEA (in press) does not admit the
possibility of assessing the natural contribution. However based on Danish calculations, it is estimated that 85-90% (Tygesen 1998) of the waterborne emissions of nitrogen and phosphorus are man-made.
In this calculation for the EU-15 emissions we assume that 88% are man-made as found for Denmark. However, it should be stressed that this is a rough estimate and that the actual amount differs
significantly from country to country. This gives total man-made waterborne nitrogen and phosphorus loading in 1994 of:
- 0.88 x 2661.7 kt N/year = 2342.3 kt N-equivalents/year
- 0.88 x 171.4 kt P/year = 150.8 kt P-equivalents/year.
Airborne emissions of nitrogen and phosphate from "nature" are small and in the calculations we assume that 100% are man-made. Furthermore, airborne emissions of phosphate are quite small and are
therefore not included in the calculations.
Table 8-9
EU-15 emissions of nutrients in the form of nitrogen and phosphorus compounds to air and water. The unit is kt/year. Emissions to air from Ritter (1997). Waterborne emissions from EEA (1998a).
Substance |
Formula |
1994 |
EF(N) |
EF(P) |
Impact potential
1994 |
|
Kt/year |
g N-eq./g |
g P-eq./g |
kt N-eq./
year |
kt P-eq./
year |
Airborne emissions |
Nitrogen oxides |
NOx1 |
12544 |
0.30 |
0 |
3763.2 |
0 |
Ammonia |
NH3 |
3535 |
0.82 |
0 |
2898.7 |
0 |
Waterborne emissions |
Total P |
PO4 3- -P |
150.8 |
0 |
1 |
0.0 |
150.8 |
Total N |
N |
2342.3 |
1 |
0 |
2342.3 |
0.0 |
Total |
|
|
|
|
9004.2 |
150.8 |
1 NOx as NO2
8.5.4 Normalisation references for EU-15 in 1994
Based on a total EU-15 emission (1994) of 9004 kt N-equivalents and 151 kt P-equivalents and a population (1994) of 370 million (Ritter 1997), the EU-15 normalisation references for nutrient enrichment
are:
Person equivalents:
24 kg N-equivalents/capita/year
0.4 kg P-equivalents/capita/year
or aggregated as
119 kg NO3- - equivalents /capita/year
8.6 The normalisation reference for selected European countries
For the purpose of calculating a normalisation reference for selected European countries, the emissions of nutrient are estimated from these countries. These are summarised, and their environmental impact
potentials calculated in Table 8-14. The calculation has been made to establish whether a widening of the EU-15 area would change the normalisation reference.
8.6.1 Air emissions of nitrogen and phosphorus compounds
Table 8-10
National total 1 emissions 1994 (Ritter 1997; EEA 1998b).
Country |
Population
(Mio)
|
NOx
(kt/year)
|
NH3
(kt/year)
|
Germany |
81.1 |
2,266 |
623 |
United Kingdom |
58.2 |
2,387 |
320 |
France |
57.7 |
1,682 |
667 |
Italy |
57.0 |
2,157 |
389 |
Spain |
39.1 |
1,223 |
344 |
Netherlands |
15.3 |
530 |
172 |
Greece |
10.4 |
357 |
445 |
Belgium |
10.1 |
374 |
79 |
Portugal |
9.9 |
249 |
93 |
Sweden |
8.7 |
444 |
51 |
Austria |
8.0 |
171 |
86 |
Denmark |
5.2 |
276 |
94 |
Finland |
5.1 |
288 |
41 |
Ireland |
3.6 |
117 |
125 |
Luxembourg |
0.4 |
23 |
7 |
Norway |
4.2 |
218 |
25 |
Switzerland |
6.7 |
140 |
60 |
Poland |
38.4 |
1,105 |
384 |
Croatia |
4.7 |
59 |
24 |
Slovenia |
1.9 |
66 |
22 |
Lithuania |
3.8 |
77 |
80 |
Latvia |
2.7 |
45 |
16 |
Estonia |
1.6 |
43 |
14 |
Russia1 |
3 |
50 |
20 |
Total |
437 |
14,347 |
4,180 |
1 Rough estimate of the population and emissions from the part of Russia that
has direct discharges and riverine inputs to the Baltic Sea. Air emissions and
populations are assumed to be comparable to that found in Latvia based on
the data of aquatic N inputs to the Baltic Sea.
8.6.2 Direct discharges and riverine inputs of total nitrogen and phosphorus compounds in selected countries in Europe
The direct discharges and riverine inputs of total nitrogen and phosphorus into the different sea areas surrounding Europe are depicted in the tables below. All data are finally aggregated in Table 8-13
illustrating the total direct discharges and riverine inputs to the sea of total nitrogen and phosphorus in the selected countries.
Table 8-11
Direct discharges and riverine inputs of total nitrogen and total
phosphorus into the OSPAR area (kt/year)1 in 1994 estimated from
EEA (1998a) and EEA (in press).
Country |
Total Nitrogen
(kt/year)
|
Total Phosphorus
(kt/year)
|
Germany |
355.0 |
12.5 |
United Kingdom |
376 |
36 |
France |
67 |
- |
Netherlands |
490.0 |
27.5 |
Belgium |
47 |
2.0 |
Portugal |
15.7 |
14.2 |
Sweden |
6.9 |
0.3 |
Denmark |
74.1 |
2.2 |
Ireland |
179.1 |
10.5 |
EU-15 |
1610.8 |
105.2 |
Norway |
97.2 |
4.1 |
Total |
1708.0 |
109.3 |
1 High estimates used; some countries have given low and high estimates.
Table 8-12
Direct discharges and riverine inputs of total nitrogen and total
phosphorus into the Baltic Sea area (kt/year)1 in 1995 Estimated
from EEA (1998a) and EEA (in press). This data are estimates from
1995 and not from 1994; however, the error caused by this factor is
expected to be of minor importance.
Country |
Total Nitrogen
(kt/year)
|
Total Phosphorus
(kt/year)
|
Germany |
21.4 |
0.6 |
Sweden |
130.9 |
4.7 |
Denmark |
66.5 |
2.3 |
Finland |
66.1 |
3.6 |
EU-15 |
284.9 |
11.2 |
Estonia |
46.5 |
1.3 |
Latvia |
91.1 |
2.2 |
Lithuania |
36.8 |
1.4 |
Poland |
214.7 |
14.2 |
Russia |
84.6 |
7.1 |
Total |
758.6 |
37.4 |
1 High estimates used; some countries have given low and high estimates.
The direct discharges and riverine inputs of total nitrogen and total phosphorus into the Mediterranean Sea from Europe are estimated to be about 823 kt N and 60 P kt/year (Kristensen, 1999). Discharges
of nitrogen and phosphorus are of the order of 270 and 24 kt/year, respectively, in the Adriatic region including discharges from Italy, Croatia and Slovenia (UNEP 1996).
The annual total discharges in the Black Sea, mid 1990s are estimated to about 406 kt nitrogen and about 55 kt phosphorus (EEA 1998a). Of this amount, about 236 kt nitrogen and 43 kt phosphorus
come from international rivers, thus, the total annual discharges for nitrogen and phosphorus from all the countries surrounding the Black Sea region are less than half of the total discharges from international
rivers (EEA 1998a). The annual discharges of the Danube alone are estimated to be 230 kt total nitrogen and 40 kt total phosphorus (EEA, in press).
Table 8-13
Direct discharges and riverine inputs to the seas around Europe of
total nitrogen and phosphorus in (kt/year)1 in 1994, Estimated from
EEA (1998a) and EEA ( in press).
Country |
Total Nitrogen
(kt/year)
|
Total Phosphorus
(kt/year)
|
Germany |
376.4 |
13.1 |
United Kingdom |
376 |
36 |
France |
185 |
8 |
Italy |
346 |
29 |
Spain |
185 |
11 |
Netherlands |
490.0 |
27.5 |
Greece |
117 |
7 |
Belgium |
47 |
2.0 |
Portugal |
15.7 |
14.2 |
Sweden |
137.8 |
5 |
Austria |
|
|
Denmark |
140.6 |
4.5 |
Finland |
66.1 |
3.6 |
Ireland |
179.1 |
10.5 |
Luxembourg |
|
|
Norway |
97.2 |
4.1 |
Estonia |
46.5 |
1.3 |
Latvia |
91.1 |
2.2 |
Lithuania |
36.8 |
1.4 |
Poland |
214.7 |
14.2 |
Russia2 |
84.6 |
7.1 |
Croatia and Slovenia |
76.0 |
5.0 |
Total |
3308.6 |
206.7 |
1 To the Baltic Sea area.
2 High estimates used; some countries have given low and high estimates.
8.6.3 Emissions of nutrients to air and water in selected European countries
It is estimated that 85-90% of the waterborne emissions of nitrogen and phosphorus is man-made. In this calculation, we assume that 88% (as the figure of Denmark) are man-made; however, it should be
stressed that the actual amount differs significantly from country to country. This gives total man-made waterborne nitrogen and phosphorus loading in 1994 of:
- 0.88 x 3308.6 kt N-eq./year = 2911.6 kt N-eq./year
- 0.88 x 206.7 kt P-eq./year = 181.9 kt P-eq./year.
Airborne emissions of nitrogen and phosphate from "nature" are small and in the calculations we assume that 100% are man-made. Furthermore, airborne emissions of phosphate are quite small and are
therefore not included.
Table 8-14
Emissions of nutrients in the form of nitrogen and phosphorus compounds to air
and water. The unit is kt/year. Emissions to air from Ritter (1997). Waterborne emissions from EEA (1998a).
Substance |
Formula |
1994 |
EF(N) |
EF(P) |
Impact potential
1994 |
|
Kt/year |
g N-eq./g |
g P-eq./g |
kt N-eq./
year |
kt P-eq./
year |
Airborne emissions |
Nitrogen oxides |
NOx1 |
14297 |
0.30 |
0 |
4289.1 |
0 |
Ammonia |
NH3 |
4160 |
0.82 |
0 |
3411.2 |
0 |
Waterborne emissions |
Total P |
PO4 3- -P |
181.9 |
0 |
1 |
0.0 |
181.9 |
Total N |
N |
2911.6 |
1 |
0 |
2911.6 |
0.0 |
Total |
|
|
|
|
10611.9 |
181.9 |
1 NOx as NO2
8.6.4 Normalisation references for selected European countries in 1994
Based on the total emission (1994) of 10611.9 kt N-equivalents, and 181.9 kt P-equivalents and a population (1994) of 437 million, the normalisation references for nutrient enrichment in the selected
European countries are:
Person equivalents:
24 kg N-equivalents/capita/year
0.4 kg P-equivalents/capita/year
or aggregated as
119 kg NO3- - equivalents/capita/year
8.7 Worldwide normalisation reference for nutrient enrichment
The worldwide for normalisation reference for nutrient enrichment is calculated by a simple extrapolation from the EU-15 data to a world basis by use of the GDP (Gross Domestic Product). A general
extrapolation method is described in chapter 3, Development of normalisation references for different geographic areas. The general methodology is based on the following assumptions:
- linear relationship between normalisation factor and ln(GDP/capita)
- the normalisation factor is zero when the average income expressed as GDP/capita is zero.
Because of the lack of worldwide data the world-proxy presented in this report does not consider the differences between countries regarding degree of industrialisation, wastewater treatment, agricultural
area, agricultural practice like livestock production etc.
The worldwide normalisation reference for nutrient enrichment can be calculated as:
0.8 x NormrefNutrient enrichment, EU-15
i.e:
19 kg N-equivalents/capita/year
0.3 kg P-equivalents/capita/year
or aggregated as
95 NO3- - equivalents/capita/year.
8.8 Evaluation of uncertainties
The uncertainties are scored in three categories: "low", i.e. an uncertainty expected to be considerably lower than one order of magnitude, "medium", i.e. an uncertainty expected to be up to one order of
magnitude, and "high", i.e. an uncertainty expected to be higher than one order of magnitude. The uncertainties are scored as "low" for the normalisation reference for Denmark, "medium" for EU-15, and
"high" for the world-proxy. The uncertainties are due to the applied methodology and limitations of data. It should be stressed that these uncertainties are subjective and based on the authors experiences
obtained during the project. Within the frame of this project it has not been possible to estimate the size of the uncertainties more precisely.
8.9 Recommendation for future updating
Most of the data sources used in the estimates are linked to international or national statistics, which are regularly updated. It should therefore be relatively simple to update the calculations for Denmark and
the EU-15 countries.
Extrapolation from EU-15 to the world has been discussed in other LCA projects, and the conclusion is that all extrapolation methods will be based on general assumptions and generally they will therefore
never be valid (see chapter 3, Development of normalisation references for different geographic areas). It is recommended to validate the estimated world-proxy for the normalisation reference for nutrient
enrichment by comparison with an alternative method like the one described below. It was, however, not possible within the frame of the present project to perform this validation.
An alternative method is to calculate the nutrient enrichment potential for a few reference groups of countries or geographical areas that differ in the important parameters in relation to nutrient enrichment.
When a normalisation reference for nutrient enrichment is calculated for a given country or region the selected factors of the specific country or region should be compared with the most comparable
reference country or geographical area. After the comparison the nutrient enrichment potential (NO3- - equivalents/capita/year) can be estimated. Some of the important parameters, which should be
considered when selecting the country reference groups or geographical areas, are listed in Appendix A.
8.10 References
Christensen, N., Paaby, H., and Holten-Andersen, J. 1993, Environment and society - the state of the environment in Denmark. Professional report no. 93, National Environmental Research Institute.
In Danish [9]
EEA (European Environment Agency) (in press) Excessive anthropogenic nutrients in European ecosystems.
EEA 1998a, Europe's Environment: The Second Assessment. Eurostat, European Commission, European Environment Agency. Office for Official Publications of the European Commission, Luxembourg.
EEA 1998b,. Europe's Environment: statistical compendium for the Second Assessment. Eurostat, European Commission, European Environment Agency. Office for Official Publications of the European
Commission, Luxembourg.
Hauschild, M. & Wenzel, H. 1998c, Nutrient enrichment as a criterion in the environmental assessment of products. In Hauschild M, Wenzel H (eds.). Environmental assessment of products. Volume 2:
Scientific background. London: Chapman & Hall.
Kirkegaard, J. 1995, Personal communication, Danish Environmental Protection Agency.
Kristensen, P. 1999, Personal communications, DMU, Denmark.
Kristensen P. 1998, Nutrient discharges from point sources (industry and consumers) in the European Union. Contribution from the EEA ETC/IW as input to RIVM's DGXI study on priorities.
DRAFT.
Larsen, S.E., Erfurt, J., Græsbøll, C., Kronvang, B., Mortensen, E., Nielsen, C.E., Ovesen, N.B., Paludan, C., Rebsdorf, Aa., Svendsen, L.M. & Nygaard, P. 1995, Ferske vandområder - vandløb og
kilder. Vandmiljøplanens overvågningsprogram 1994. Danmarks Miljøundersøgelser. Faglig rapport fra DMU, nr. 140.
Miljøstyrelsen 1994, Punktkilder. Vandmiljø.planens overvågningsprogram 1993. Fagdatacenterrapport. Orientering fra Miljøstyrelsen No. 8. In Danish9.
Miljøstyrelsen 1995, Vandmiljø-95. Redegørelse fra Miljøstyrelsen Nr. 3. In Danish9.
Miljøstyrelsen 1997, Vandmiljø-97. Redegørelse fra Miljøstyrelsen Nr.4. In Danish9.
Ritter, M. 1997, CORINAIR 94 - Summary Report - European Emission Inventory for Air Pollutants. Copenhagen: European Environment Agency.
Skov, H., Ellermann, T., Hertel, O., Manscher, H., & Fohn, M. 1996, Atmosfærisk deposition af kvælstof. Vandmiljøplanens Overvågningsprogram 1995. Hovedrapport. Danmarks
Miljøundersøgelser. Faglig rapport fra DMU nr. 173.
Tygesen Niels 1998, Personal communication. European Environment Agency.
Appendix A: Important parameters in relation to nutrient enrichment
- Emissions to air:
- The main sources of emission to air in relation to nutrient enrichment are NOx and NH3. The main emissions of NOx to air are from road transport, combustion in energy and transformation
industries, and other mobile sources and machinery. These three main source sectors constituted more than 80% of the total emissions of NOx in the EU-15 countries in 1994 and should
therefore some how be included in the evaluation.
- For NH3 the main source sectors are agriculture and forestry, land use and wood stock change which constituted about 95% of the total NH3 emission in the EU-15 countries in 1994.
- Information on the activity in these sectors is not available in statistics covering the whole world; however, it should be available for different selected groups of countries or geographical areas
that differ in industrial and agricultural activities.
- Airborne emissions of phosphate are quite small and are therefore not included.
- Waterborne emissions:
- The main sources of emission of nitrogen to the aquatic environment are mainly due to leaching from agricultural land. An important parameter to estimate the emission of nitrogen is therefore to
evaluate the agricultural land and compare it with the total area. Furthermore, the use of nitrogen fertiliser and the number of livestock should be included in the evaluation.
- Most of the phosphorus loading of surface water is attributable to discharges from point sources, especially municipal sewage and industrial effluent cf. waste water below. However, leaching
from agricultural land also plays a significant part and an estimation of the agricultural land and the use of phosphorus containing fertiliser should be made. Data might be available for different
selected groups of countries or geographical areas that differ in industrial and agricultural activities.
- Wastewater:
- Industrial production and household consumption result in wastewater containing nutrients. The nutrient content of wastewater from households is primarily determined by excreta from humans and
phosphorus from detergents. Generally the amount of wastewater produced is proportional to the population and only to a little extent dependent on the economic development (Kristensen, 1998). The
nutrient content of the wastewater from the industries is highly dependent on the type of industry. Only a small part of the industrial sector is responsible for the majority of wastewater containing phosphorus.
To name just a few important industries the fertiliser industry and other related chemical industries manufacturing products containing phosphorus (e.g. pesticides and detergents), as well as the pulp and
paper industry and fish processing industry should be mentioned. The extent to which the nutrients in wastewater are discharged into surface waters depends on the wastewater treatment facilities available.
The majority of the households in the EU Member States are connected to sewers and municipal wastewater treatment plants (Kristensen 1998). However, in other parts of the world only a small part of the
households may be connected to a wastewater treatment plant.
For waste water from households it might be an idea to estimate the emission of the nutrient content to the aquatic environment by looking at the population density, the amount of phosphorus sold in
detergents, and percent of people connected to a waste water treatment plant. For an evaluation of the emissions from industries it is important determine the industries that are present and the wastewater
treatment facilities that are available.
9 Human toxicity
Frans M. Christensen, Danish Toxicology Centre, Leif Hoffmann and Anders Schmidt, dk-TEKNIK ENERGY & ENVIRONMENT
9.1 Summary
The overall aim of this chapter is to update the present EDIP human toxicity normalisation references for air, water and soil according to Danish conditions and to establish similar European (EU-15)
references. A global normalisation reference for human toxicity has been estimated based on the principles described in chapter 3, Development of normalisation references for different geographic areas.
The EDIP-methodology (Hauschild et al. 1998) has been used to calculate the reference values. The normalisation references are shown in Table 9-1.
Table 9-1
Normalisation references for toxicological impact as a result of exposure via air, water and soil for Denmark, EU-15 and worldwide.
|
Denmark |
EU-15 |
Worldwide |
Air; m3 air/capita/year |
5.53*1010 |
6.09*1010 |
4.87*1010 |
Water; m3 water/capita/year |
1.79*105 |
5.22*104 |
4.18*104 |
Soil; m3 soil/capita/year |
1.57*102 |
1.27*102 |
1.02*102 |
The normalisation references for human toxicity by exposure through airborme emissions have changed significantly, compared to the values in EDIP 97. The main reason for this is that it has been possible to
include a speciation of the nmVOC emitted from road transport in the calculation. Some of the species emitted have a large toxicity potential as reflected by its effect factor and this – combined with the large
amounts being emitted – have caused an increase in the normalisation references of about a factor 25, compared to the situation where no speciation of nmVOC is included. In practice, this means that the
updated normalisation references have increased with a factor 6 instead of decreasing with a factor 4. The database and the calculations is described in more detail in section 9.3.1.2 and in Appendix B2.
The importance of road transport is also evident when looking at the relative contribution of different substances to the normalisation reference. At the EU-15 level, nmVOC from road transport accounts for
about 95% of the total impacts. On the national level a similar contribution is observed in all EU-countries with Luxembourg as an exception with "only" about 86% of the normalisation reference being
related to nmVOC from road transport.
In this context, the contribution from other substances becomes less important, although they still should be targeted for reductions. Lead and particulate matter (PM10) accounts for 3.1% and 0.3%,
respectively, at the EU-15 level. The two substances are important for all individual Member States, but in some countries also PAH and NOx are equally important. In Denmark, lead, NOx and PM10 are
the most significant contributors besides nmVOC from road transport.
The normalisation reference for human toxicity via air can, however, only be determined with a large degree of uncertainty and some of the main sources for this uncertainty are discussed in more detail in the
following sections. As a general rule, the normalisation reference is believed to provide the right order of magnitude, but more knowledge about the toxicity of emissions of nmVOC and particulate matter
(both PM10 and PM2.5) may cause significant changes of the normalisation reference in a future update.
The substances contributing with more than one percent to the normalisation reference for human toxicity by water for EU-15 are mercury (air and water emissions; 90%), dioxins (air emission; 4%), lead
(water and air emissions) and zinc. For Denmark mercury is the only substance contributing with more than one percent. The substances contributing with more than one percent to the normalisation
reference for soil for EU-15 are arsenic (air; 60%), mercury (air; 24%), tetrachloroethylene, arsenic (sludge), mercury (sludge), chromium (air), tetrachloromethane, lead and cadmium (both air).
9.2 Description of the impact category
The normalisation references for human toxicity via the environment [10] should reflect the total human toxic load in the reference area caused by human activity, i.e. the potential risk connected to exposure
from the environment (via air, soil, provisions and drinking water) as a result of emissions to the environment from industrial production, traffic, power plants etc. [11]
Ideally, all emissions of substances potentially affecting human health should be quantified and assessed. However, the multitude of known substances (>100.000) and an even larger number of emission
sources logically makes that approach unfeasible. The inventory used for calculating the normalisation references is therefore based on available emission registrations for substances, which are
believed to contribute significantly to the overall load. However, some significant emissions may still be missing. Further, the contribution from the multitude of minor emissions is not included. The contribution
to the overall toxic load from these substances is unknown. A more detailed discussion of included substances and available data sources is given in section 9.3.
There are also uncertainties connected to the methodology for estimating the toxic load of the substances and thereby the calculated normalisation references. They are discussed in section 9.5 and 9.6.
See Hauschild et al. (1998) for a further description of the impact category.
9.3 Substances and data sources
Data and data sources used for calculating the normalisation references for air, water and soil are outlined in this section. Data included are discussed for each emission route. Then, the extrapolation method
used to estimate lacking data for some countries is briefly described, and finally, some general omissions are outlined.
9.3.1 Substances included
This section present and comments different exposure routes. Groups of substances of special interest (VOC and particulate matter) is also presented.
9.3.1.1 Air
Major contributions to toxicity via air are assumed to be emissions from transport and energy production, waste incineration plants, industrial releases of POPs (Persistent Organic Pollutants) and other HPV
(High Production Volume) chemicals. Corinair 94 (Ritter 1997) is a key reference including many of the above emissions and it is assessed to be a good quality data source. However, heavy metal and POP
emissions from a number of countries are lacking. The lacking data have been extracted from other references as indicated in Appendix B.1 (e.g. POP and heavy metal emissions from 1995 and dioxin
emissions from an European survey) or estimated by extrapolation, see paragraph 9.3.2. The Corinair data has, where relevant, been supplemented with more exact data, se also Appendix A and C for
detailed references.
The quantification of emission of chemicals other than heavy metals, POPs and other traditional air pollutant (like NOx and SO2) from the (chemical) industry at the European level is poor. Data from Dutch
emission registrations (van der Auweraert et al. 1996) from major chemical enterprises have been investigated. However, apart from nmVOCs (non-methane Volatile Organic Compounds) discussed
below and vinylchloride, equivalence factors (EQFs) for these substances are lacking in the present EDIP "toxicity database" (Hauschild et al. 1998) and it has not been within the scope of this project to
establish these factors.
Potentially - when the necessary EQFs become available - the Dutch emission figures can be used as a basis for extrapolation to other European countries and to an overall European level. Initially, a
pragmatic approach is to evaluate whether the individual substances contribute significantly to the Dutch normalisation reference. Emission quantities for substances contributing less than 1% should not be
extrapolated to other countries. This approach has been used for vinyl chloride in this project, see section 9.5. The uncertainty connected to the Dutch emission registrations is difficult to quantify. The Dutch
registrations cover major emission sources.
9.3.1.2 VOC
VOCs from industrial point sources usually consist of one or a limited number of specific substances/solvents, whereas VOCs from combustion processes are a very complex mixture, usually containing a
major fraction of alkanes, medium amounts of methane, olefins, ethene and monocyclic aromatics, minor amounts of aldehydes (some of it being formaldehyde), and trace amounts of PAHs (between
others benzo(a)pyrene and naphthalene) (van der Ven 1995).
According to Corinair94 (Ritter 1997), the major European outdoor emission sources of nmVOCs are: approx. 30% from road traffic, 30% from solvent and other product use, about 20% from
agriculture, forestry, land use and wood stock exchange and approx. 10% from different energy production and manufacturing combustion activities [12]. It is difficult to assign a specific toxicity for
VOCs as a group, as it covers a wide range of substances. In the original EDIP method, nmVOC emissions were evaluated based on one equivalence factor for unspecified toxic action. This is clearly a
simplification and has underestimated the toxicity of VOCs with specific actions, as evidenced by the significantly higher normalisation reference established in the update in the current project by applying
specific factors for a large number of substances emitted from road transport. The establishing of specific effect factors for nmVOC from road transport and the importance of the emissions is described in
detail in Appendix B.2.
9.3.1.3 Particles
Particle emissions were not included in the previous EDIP methodology, but the increasing evidence that particles contribute significant to human health impact has called for the inclusion of particles in the
normalisation reference. In terms of measuring particle emission/concentration, quite a few parameters can be applied. Traditionally, suspended particles have been measured as TSP (total suspended
particulates) or as Black Smoke (BS) [13]. A more recent indicator for suspended particles is PM10 (particles with diameter below 10 m). It measures particles, which are believed to cause the major
health concerns as these may penetrate deeply into the airways. However, measurement data of this parameter are still very incomplete (EEA, 1997). Particle emissions are not included in Corinair, but a
Dutch report identifying some preliminary values has been identified (Berdowski et al. 1997b). This data source is not complete, many data have been estimated and the data are thus subject to some
uncertainty, see the reference for further details.
As can be seen from the above, more references may be relevant for a given emission depending on the country. General data sources for the different impact categories are presented in Appendix A.
Specific references for the individual emission estimates are given in the calculation sheets in Appendix C.
9.3.1.4 Water
Included in this normalisation reference are:
- Deposition of heavy metals and POPs from air. According to the EDIP methodology, substances emitted to air contribute to water and soil toxicity if the atmospheric half life is > 1 day. It is assumed
that 80% is deposited on soil and thereby contributes to soil toxicity, while 20% is assumed deposited on water.
- Deposition of other chemicals emitted to air - data from previous section
- Emissions from sewage treatment plants; detergents, metals, others
See chapter 10, Ecotoxicity, for a discussion of data sources, data uncertainties and relevant contacts.
9.3.1.5 Soil
Included in this normalisation reference are:
- Deposition of heavy metals and POPs
- Deposition of other chemicals emitted to air
- Sludge from sewage treatment plants
See chapter 10, Ecotoxicity, for a discussion of data sources, data uncertainties and relevant contacts.
9.3.2 Extrapolation method applied
For a number of countries, data are missing. Therefore, it has been necessary in relation to extrapolation to estimate these data to calculate national normalisation references as well as an EU-15 reference.
Special aspects in relation to extrapolation methodology applied for air emissions are described in Appendix B.1, whereas soil and water emissions have been dealt with in chapter 10, Ecotoxicity.
9.3.3 General omissions
No specific action has been taken in order to quantify emissions of substances, which are assumed to cause endocrine disruption, although these effects have lately been a major issue in the public and
scientific debate. This attitude is due to the substantial present uncertainty of the actual extent of these effects also including the contributing substances and their potencies. Thus, at the moment, it does not
seem possible to select substances to be included nor to assign these an equivalence factor (reflecting the potency).
The major human problem for pesticides is believed to be exposure via contaminated drinking water (this exposure route is not yet operationalised in the EDIP methodology). However, toxic effects via
other exposure routes cannot be excluded. Pesticides have not been included in the present project due to the lack of exposure data and effect factors for the multitude of pesticides applied. A proposed
methodology for future inclusion is outlined in section 9.6.
Emissions from the off-shore industry have not been quantified as they are is believed to cause minor effects compared with other emissions.
Application of anti-fouling agents has been a major debate issue lately, but mainly in relation to potential effects on wildlife. Thus, in relation to human toxicity, the same consideration as for off-shore
emissions is assumed.
Photochemical ozone is included in the effect category on Photochemical ozone formation. As "double-counting" should be avoided, it is therefore - although definitely toxic - not included here. See also the
discussion on impact categories in chapter 2, Selection of impact categories.
9.4 Methodology
9.4.1 Toxicity in LCA
In LCA, different `types' of toxicity (irritation, cancer, neurotoxicity etc.) are described in a single figure. This is scientifically questionable. However, as with many aspects of LCA, the assessment should be
made as good as possible based on the existing tools. In many LCA's, assessment of toxicity has been left out to make decisions causing an increased toxic load. EDIP (Hauschild et al. 1998) is one of the
tools developed for assessment of toxicity in LCA. A few others have been developed internationally, and as to quality, there seem to be no or little difference.
The impact potentials for human toxicity can be calculated as:
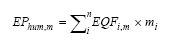
where EPhum,m is the effect potential (human toxicology) caused be exposure via the medium m (water, soil, air)
EQFi,m is the equivalency factor for the substance i caused by exposure via the medium m (water, soil, air)
mi is the emission of substance i
9.4.2 EDIP
EDIP is used in present project. It is important that the results are used with caution as it appears from the uncertainty discussions during the remaining parts of the chapter. The EDIP method could be
improved. Methodological problems are further discussed in section 9.6.
Normalisation references are calculated based on EDIP (Hauschild et al. 1998):
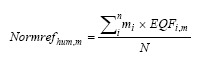
where Normrefhum,m is the normalisation reference for human toxicity caused by exposure via the medium m (water, soil, ait)
mi is emitted quantity of substance i
EQFi,m is the equivalence factor for the substance i caused by exposure via the medium m (water, soil, air)
N is the number of capita in the considered area
Quantification of emissions is carried out as outlined in section 9.3.
9.4.3 Equivalence factors (EQF's)
The current EDIP equivalence factors (EQF's) given in Hauschild et al. (1998) will be used in assessing individual substances. As particles are regarded as a significant new emission, an EQF has been
estimated, see Appendix B.2. Some EQF's cover "category" emissions as for instance nmVOC, PAH and dioxins [14]. How VOC is dealt with was discussed in paragraph 9.3.1. PAH as well as dioxins
are groups of substances of different toxicity. In order to sum up the human toxicological potential of PAH's and dioxins to a single number, toxic equivalence factors have been developed and adapted
internationally (e.g. I-TEQ). By using these equivalence factors, the total PAH emission can be expressed as benzo(a)pyrene equivalents, and the total dioxin emission can be expressed as
2,3,7,8-tetrachloro-p-dioxin equivalents (2,3,7,8-TCDD-eq. (I-TEQ)).
The dioxin equivalence factors have been used for some years and are internationally accepted whereas the PAH equivalence factors are still under development. This means that emissions of dioxins
normally are reported in I-TEQ while emissions of PAH are still reported as Σ PAH. By using the distribution of PAH's in calculation of benzo(a)pyrene-eq. and the Danish Σ PAH, a equivalency factor has
been developed to transform Σ PAH to benzo(a)pyrene (detailed information on the distribution of PAH's is available for Denmark; see Table 9-10 in Appendix B.1):
Benzo(a)pyrene-eq. = F Σ PAH = 0.12 x Σ PAH
The information on emissions of PAH in the Corinair94 database is supposed to be Σ PAH and is transformed to benzo(a)pyrene-eq. by using the above-mentioned equation.
Finally, the EQF's for dichlorobenzene and tetrachloroethylene have been used for assessing the toxicity of different chlorinated hydrocarbons for which EQF's are missing.
9.5 Normalisation references
Due to changes in, for instance, traffic load, industrial activity and remediation actions, the overall emission of toxic substances to the environment is changing in time. The normalisation references calculated
in this project are based on 1993-95 data i.e. 1993 or 1995 data has been used when 1994 data were not available. Some uncertainty in the normalisation references and the underlying data is therefore
connected to the reference year chosen.
It should be mentioned that not all substances contributing potentially to the overall toxic load have been included. The quantity of the toxic load captured is uncertain, as it is difficult to quantify the
contribution from the multitude of substances used in minor amounts.
Only emission of substances contributing more than 1% to the normalisation references presented in this section have been included in the tables. More detailed calculation sheets including data references are
found in Appendix C. The following (and appendix C) "Original EDIP97" refer to the normalisation values presented in the original Danish version of the method (Hauschild et al. 1996), `EDIP English'
to the reference values in Hauschild et al. (1998) and `EDIP revision' to the references calculated within this project.
9.5.1 Denmark
9.5.1.1 Air
The overall Danish toxicity impact potential has been calculated to: 2.91*1017 m3 air, which gives the normalisation reference
5.53*1010 m3 air/capita/year
Table 9-2 summarises the substances assessed to contribute significantly to the normalisation reference.
Table 9-2
Substances contributing by more than 1% to the normalisation reference
for air.
Substance |
Fraction (%) |
nmVOC – transport |
96.5 |
Lead (Pb) |
1.4 |
Compared to the normalisation reference calculated in EDIP English (based on 1990 emission figures), the normalisation reference has approximately increased by a factor of 6 from 9.18 109 to 5.53 1010
m3 air/capita/year.
The increase is caused by the inclusion of different species of nmVOC from road traffic. It is not surprising that the higher level of detail causes an increase in the normalisation reference, but it was rather
unexpected that the increase was of this magnitude.
Further comparison of the normalisation references from 1990 and 1994 shows that air lead emissions are rapidly decreasing due to the substitution of lead additives in gasoline. The lead contribution must
be assessed to be even smaller today, as lead has been entirely substituted in petrol. According to the collected data, also the cadmium emission has dropped dramatically. It is also mentioned that if nmVOC
from transport had not been included in the update, other "new" substances such as PAH, benzene emissions to water and particulate matter would have contributed significantly.
As explained in the methodology section 9.4, an equivalence factor (EQF) for particles has been proposed, see Appendix B.2. However, it was difficult to assign an EQF as the toxicity of particles is very
complex and highly depends on the type of particles in question. The assignment of an EQF became further complicated by the recent evidence indication that there is no threshold limit for particle exposure.
Therefore, it was decided as a first pragmatic approach to use the US one year average guidance limit as a basis for the EQF calculation, see appendix B.2. Future updates of the normalisation references
should, however, have particulate matter as one of the focus points. Drivsholm et al. (2002) thus argue that an effect factor of 5.5*108 m3/g (a factor 25,000 more than used in the present study!) may be
realistic for at least some of particles emitted. If this factor is applied, the normalisation references will once more increase significantly, perhaps by a factor 100.
If a safety factor of 10 is assigned to the particle EQF used in the present study, the contribution from particles will exceed 3%, indicating the importance of this emission. None of the particle EQF's can be
judged more correct than the other, but it can be seen that determination of EQF's is very crucial in relation to the magnitude of the normalisation reference and in relation to which substances will contribute
to the reference.
This can also be seen when comparing the figures for Original EDIP97 and EDIP English in Appendix C.1.1. Due to re-evaluation of the EQFs between the two versions (applying the same emission
figures), the relative importance of the substances has changed considerably, with N2O being the dominant in Original EDIP97 and lead in EDIP English.
9.5.1.2 Dutch experiences
Further methodological considerations can be made by comparing with a normalisation project which has been carried out in Holland (Blonk et al. 1997). In that project, the Dutch LCA toxicity
methodology, which differs substantially from the EDIP methodology, was applied. Two significant methodology differences are that the Dutch method uses a continuous modelling of the environmental fate
of the chemicals and that all toxicity contributions are condensed into one figure. EDIP applies a simple semi-quantitative fate modelling and operates with toxicity via air, water and soil, separately. A further
investigation of the Dutch figures shows that the air toxicity contribution is by far the dominating; exceeding the water toxicity contribution by about two decades. A comparison between the air toxicity for
Dutch conditions as calculated by the EDIP methodology (see Appendix C.1.8) and by the Dutch methodology (distribution fraction shown in Table 9-3) seems interesting.
Table 9-3
Substances contributing by more than 1% to the normalisation reference in
The Netherlands according to the Dutch LCA-methodology (Blonk et al.
1997).
Substance |
Fraction (%) |
NOx |
49 |
SO2 |
22 |
Rest. non-halog. arom. CHa |
5 |
Nickel (Ni) |
5 |
Lead (Pb) |
5 |
Xylene |
4 |
Benzene |
3 |
Methane |
3 |
Arsenic (As) |
1 |
Rest. non-halog. alif. HCb |
1 |
1,1,1-trichloroethane |
1 |
a Resistant non-halogenated aromatic hydrocarbons
b Resistant non-halogenated aliphatic hydrocarbons
It can be seen that the SO2 and NOx contributions are by far the dominating when applying the Dutch methodology, whereas lead contributes by only about 5%. This distribution differs significantly from any
of the normalisation references developed so far in the EDIP method, and it is striking how much the method and EQF's applied affect the toxicity assessment outcome.
The methodology uncertainties (some of which are further discussed in section 9.6), therefore seem to be of major importance and probably exceed the emission data uncertainties substantially. However,
there will always be methodology uncertainties as toxicity evaluations, especially in connection with LCA, are crude simplifications of reality.
As previously described, nmVOC emissions have so far been assessed as one fraction with an EQF based on unspecified toxic action. In this way specific toxic action of some of the VOC's have been
underestimated. Dutch emission data obtained from van der Auweraert et al. (1996) gives the possibility to examine a different approach to include nmVOC on a more detailed level. When using the Dutch
registration of emissions, formaldehyde and benzene contributes with 52% and 33%, respectively (Appendix C.1.8). The normalisation reference is, however, only about 25% of that calculated by using the
even more detailed approach applied in the present study.
The calculated Dutch normalisation reference was also intended to figure out which other chemicals (industrial releases) could be assumed to contribute significantly to the overall toxic load. However,
EQF's were missing for most of these substances. Only vinyl chloride could be assessed and it turned out to be of minor importance, see Appendix C.1.8. The significance of the other chemical releases
may or may not be significant. However, a cautious comparison with the Dutch normalisation project (Blonk et al. 1997; see also Table 9-3) indicates that these emissions are of minor importance.
9.5.1.3 Water
The overall Danish toxicity impact potential has been calculated to: 9.29*1011 m3 water, which gives the normalisation reference
1.79*105 m3 water/capita/year
Table 9-4 summarises the substances assessed to contribute significantly to the normalisation reference.
Table 9-4
Substances contributing by more than 1% to the normalisation reference for water.
Substance |
Fraction (%) |
Mercury (Hg) from air deposition |
90 |
Mercury (Hg); water emission |
9 |
The normalisation reference has increased by a factor 3 compared to EDIP English; from 5.9*104 to 1.79*105 m3 water/capita/year. This increase is mainly due to a 5-fold increase in mercury emission to
air (and the assumed deposition). The reason may be difference in the methods for emission quantification. This is also illustrated in the data behind EDIP English, where it is assumed that about 1 ton Hg is
emitted directly to the water and 0.5 ton is deposited, i.e. the major Hg toxicity was assumed to be via water.
The rationale behind the effect factor for mercury is that the distribution between air, water and soil as recipient is the same regardless of the primary recipient. The potential effects in e.g. the aquatic
environment will be the same for emissions to air, water and soil as reflected in the equivalency factors.
In EDIP English, air deposition of mercury was also the dominating contributor, but lead and Cd were also significant. However, the emission of Pb and Cd has decreased by a factor 9 and 5, respectively,
which along with the increase in mercury air emissions gives the very high mercury contribution. The dioxin contribution that was 9% in EDIP English has dropped to below 1%.
The results are striking. Firstly because mercury seems to be the only significant contributor to toxicity via water and secondly because air emissions seem to be the dominating factor for water toxicity due
to deposition of the emitted heavy metals.
As for air toxicity, the major uncertainty seems to be considerably related to the EDIP toxicity methodology, including the applied EQF's, though emission quantities are also uncertain.
9.5.1.4 Soil
The overall Danish toxicity impact potential has been calculated to: 8.19*108 m3 soil, which gives the normalisation reference
1.57*102 m3 soil/capita/year
Table 9-5 summarises the substances assessed to contribute significantly to the normalisation reference.
Table 9-5
Substances contributing by more than 1% to the normalisation reference
for soil.
Substance |
Fraction (%) |
Mercury (Hg) from air deposition |
75 |
Arsenic from air deposition |
9.1 |
Arsenic from sludge |
7.6 |
Mercury from sludge |
3.1 |
Tetrachloroethylene from air deposition |
1.7 |
The normalisation factor has decreased from 3.1*102 to 1.6*102 m3 soil/capita/year i.e. by a factor 2.
Mercury and arsenic from air deposition and sludge are the main contributors to the normalisation reference for toxicity via soil. The figures are not directly comparable with the previous survey as the
emissions are compiled in different groups. Mercury from air deposition and sludge are counted as total emissions of lead, cadmium and mercury, which constitute 29% of the previous normalisation
reference. Arsenic is counted in the groups named metals from sludge (50% of the total potential impact) and metals from electricity generation (<1% of the total potential impact).
The potential atmospheric deposition also includes a number of organic substances (POP's) of which only tetrachloroethylene contribute with 1.7% of the total potential environmental impact.
9.5.2 EU-15
9.5.2.1
Air
The overall EU-15 toxicity impact potential has been calculated to: 2.25*1019 m3 air, which gives the normalisation reference
6.09*1010m3 air/capita/year
Table 9-6 summarises the substances assessed to contribute significantly to the normalisation reference. A detailed survey of the distribution of the contributions of different substances to the toxicity potential
for different European countries and EU-15 can bee seen in appendix D.1.
Table 9-6
Substances contributing by more than 1% to the normalisation reference for air.
Substance |
Fraction (%) |
NmVOC – transport |
95.2 |
Lead (Pb) |
3.1 |
A comparison can not be made with EDIP English as EU-15 was not previously included in EDIP.
The EU-15 normalisation factor is approx. 10% higher than the Danish factor. As the main contributor is nmVOC from road transport this indicates that the traffic load in the EU in average is higher than in
Denmark. The distribution between other substances indicates a higher lead contribution in Europe than in Denmark. This may partly be due to a higher lead substitution percentage in petrol for the Danish
market. Contributions from other substances are consequently lower compared to Danish conditions.
The same assumptions and uncertainty discussions as for Danish conditions applies also to EU-15, see paragraph 9.5.1.
9.5.2.2 Water
The overall EU-15 toxicity impact potential has been calculated to: 1.93*1013 m3 water, which gives the normalisation reference
5.22*104 m3 water/capita/year
Table 9-7 summarises which substances have been assessed to contribute significantly to the normalisation reference.
Table 9-7
Substances contributing by more than 1% to the normalisation reference for water.
Substance |
Fraction (%) |
Mercury (Hg) from air deposition |
80 |
Mercury (Hg); water emission |
10 |
Dioxin from air deposition |
4.2 |
Lead (Pb); water emission |
2.1 |
Lead (Pb) from air deposition |
1.9 |
Zinc (Zn); water emission |
1.0 |
A comparison can not be made with EDIP English as EU-15 was not previously included in EDIP.
The EU-15 normalisation factor is approximately one third of the Danish factor. The toxicity impact potential in EU-15 as well as in Denmark are dominated by air deposition and effluent of mercury, but the
air emission of mercury on EU-15 level are lower per capita than the Danish emission. As a consequence, dioxins, lead and zinc contribute with more than 1%. The reason why mercury emission to air is
higher in Denmark may be due to the application of fossil fuels with high mercury content.
9.5.2.3 Soil
The overall EU-15 toxicity impact potential has been calculated to: 4.71*1010 m3 soil, which gives the normalisation reference
1.27*102 m3 soil/capita/year
Table 9-8 summarises which substances have been assessed to contribute significantly to the normalisation reference.
Table 9-8
Substances contributing by more than 1% of the normalisation reference for soil.
Substance |
Fraction (%) |
Arsenic from air deposition |
60 |
Mercury (Hg) from air deposition |
24 |
Tetrachloroethylene from air deposition |
3.8 |
Arsenic from sludge |
3.8 |
Mercury from sludge |
1.5 |
Chromium from air deposition |
1.3 |
Tetrachloromethane from air deposition |
1.3 |
Lead from air deposition |
1.3 |
Cadmium from air deposition |
1.0 |
A comparison can not be made with EDIP English as EU-15 was not previously included in EDIP.
The EU-15 normalisation factor is approx. one half of the Danish. Mercury and arsenic dominate both normalisation factors but for EU-15 arsenic is the dominating substance and for Denmark mercury is
the dominating substance.
9.5.3 Worldwide
The worldwide normalisation references for human toxicity are calculated as proposed in chapter 3, Development of normalisation references for different geographic areas, by using a factor 0.8
normalisation reference for EU-15.
9.5.3.1 Air
The worldwide normalisation reference for exposure via air has been calculated to:
4.87*1010 m3 air/capita/year
9.5.3.2 Water
The worldwide normalisation reference for exposure via water has been calculated to:
4.18*104 m3 water/capita/year
9.5.3.3 Soil
The worldwide normalisation reference for exposure via soil has been calculated to:
1.02*102 m3 soil/capita/year
9.5.4 Comparison with the previously used normalisation reference
In Table 9-9 the estimated normalisation references are compared with the values for 1990-1992 calculated by Hauschild et al. (1998).
Table 9-9
The estimated total human toxicity normalisation references for Denmark compared to the values for the years 1992-1994 in Hauschild et al. (1998).
|
|
Denmark |
EU-15 |
World |
Air, 19901994 |
m3 air/capita/year |
9.18*109 |
- |
- |
5.53*1010 |
6.09*1010 |
4.87*1010 |
Water, 19901994 |
m3 water/capita/year |
5.9*104 |
- |
- |
1.79*105 |
5.22*104 |
4.18*104 |
Soil, 19901994 |
m3 soil/capita/year |
3.1*102 |
- |
- |
1.57*102 |
1.27*102 |
1.02*102 |
9.6 Recommendations for future updating
As can be seen from the discussions of different types of uncertainties in the previous paragraphs, the focus for future updates should be on a better understanding of the emissions from combustion sources,
i.e. both the amounts emitted from different activities and their toxicity potential. Emissions of nmVOC and particulate matter from road transport have emerged as the most important contributors to human
toxicity via air, but their assessment is still associated with a large degree of uncertainty.
One of the main tasks is to establish more precise knowledge about the amounts of different species of nmVOC being emitted. The approach used in the current project is rather straightforward, but the
results are in practice dependent on the composition of nmVOC in exhaust from different vehicles. Here, a closer examination of available scientific literature may lead to identification of better inventories
than those identified and used in Corinair.
Another main task is to establish a more precise overview of the amounts of particulate matter being emitted and distributed in the environment. Little is presently known regarding the distribution of the size
of particles being emitted from various combustion sources, but because of the assumed importance it can be anticipated that more information becomes available in the coming years.
Thirdly, better effect factors for particulate matter are needed. As described previously, it can be argued that the effect factor for fine and ultrafine particles perhaps ought to be a factor 25,000 higher than
the one used in the present study. Also here better knowledge is emerging continuously and can probably be used to establish more precise effect factors in a future update of the normalisation reference.
The three issued described above are assumed to be the major concerns of a future update. There are, however, some additional points that can be addressed in order to provide more insight into the overall
toxic load. These are mentioned briefly in the following sections.
9.6.1 Substances presently included
Emission data on the substances presently included are more or less frequently updated. However, it is believed that Corinair data and possibly data from the "Indicator project" (Eurostat, 1998) will be
improved in the future. Data on POP's and heavy metals may contain more details for more countries, and data on particle emission is assumed to be upgraded considerably in the coming years. Appendix A
lists relevant contact information in connection with a future updating.
9.6.2 Inclusion of new substances
9.6.2.1 Industrial releases
Quantification of emissions from the chemical industry may be improved. It should be investigated whether emission registrations have been made for other countries than Holland. In Germany, the database
"Sysiphus" may in the future serve as a basis for emission quantification from the German industry. It is confidential as such, but emissions at country level may be obtainable. The database does not presently
cover substantial amounts of emission data (information about Sysiphus and other data sources on use and releases from industrial chemicals can be found on: http://appli1.oecd.org).
9.6.2.2 Equivalence factors
Inclusion of the chemicals requires new EQF's to facilitate an assessment of the emissions. The stepwise methodology described in section 9.3 could be used for the selection of substances to be included in
an extrapolation to counties no having available data.
9.6.2.3 HPV chemicals
An alternative approach for HPV (High Production Volume) chemical emissions could be estimations based on assumptions on emission of given fractions of the production volumes (emission factors).
For instance, the key figures on emission ratios in the Technical Guidance Document (TGD) (EC-TGD, 1996) could be applied. These figures will give a worst case estimate, but can be highly relevant when
used as an indicator of whether or not the considered chemical contributes significantly to the overall toxic load. However, this approach demands several data on production facilities and on application of
the products, and would therefore be rather time consuming.
Further, the possibility and relevance of including the omitted data (described in section 9.3.3) should be investigated. Suggestions are presented below.
9.6.2.4 Proposal for the inclusion of pesticides
- Exposure assessment. In order to get an idea of the distribution between environmental media (air, ground water, surface water and soil) an exposure model can be used. USES 2.0
(RIVM/VROM/VWS, 1998) has been developed to include modelling of pesticide fate.
- Effect factors are calculated according to the existing EDIP methodology (Hauschild et al. 1998).
9.6.2.5 Other emissions
Other emissions assessed to be of importance at the time when the normalisation references were updated, should of course be included. As also described in the sections 9.2 and 9.5, the quantity of
missing toxic load in the normalisation references is uncertain due to the load from the diffuse use of many substances in minor quantities. The lack of emission data will tend to result in too small normalisation
references.
9.6.3 Methodological issues
As indicated in the methodology section 9.4, quite a few uncertainties are connected to the EDIP human toxicity assessment method. The equivalence factors for each substance contain both information of
the fate of the substance in the environment (exposure assessment) and of the toxicity of the substance per see (effect assessment).
9.6.3.1 Exposure method
The exposure scenarios used in the methodology causes some uncertainty. The applied semi-quantitative exposure method is rather simple. Other methods apply more sophisticated models, which should be
used with caution in LCA as they are developed for risk assessments purposes. An interesting assumption in the methods is that the contribution of air depositions is 80% on soil and 20% and water. This
is a European average and does not apply to Danish conditions. If these differences were to be included, it would mean that individual EQFs would have to be calculated for each country. That approach,
however, seems unfeasible.
An intrinsic problem with exposure assessment in LCA is caused by the marginal nature of LCA (consideration of one functional unit). Therefore, it is difficult to include local exposure levels. Consequently,
normalisation references and effect potentials are representing potential and not actual effects. This problem is dealt with in sub-project V, which should be consulted for further details.
9.6.3.2 Effect assessment
A number of uncertainties are connected to the effect assessment of the EDIP/.LCA methodology. When calculating the overall normalisation references, a multitude of emissions (and thereby toxic
modes of actions for the different substances) is added. This is also a problem, as different toxic responses are not additive and also because, the relative severity of different effects - as for instance irritation,
reversible effects and carcinogenicity - is not considered. Also the application of safety factors when calculating the EQF's may be questioned. In LCA a realistic goal for human toxicity is to be "correct on
average". LCA can not be used to obtain safe exposure levels (as is the intention in risk assessment) for all substances emitted in the product life cycle. Finally, it should be remembered that toxicity data for a
lot of substance are lacking. It might therefore be very difficult to establish a good EQF for substances, which have been poorly studied in toxicological tests.
The uncertainties involved in the exposure and effect assessment described above are not only connected to calculation of normalisation references but also to calculating effect potentials for a product or a
product system.
Due to the uncertainties in the described data, exposure and effect assessment, the normalisation references and effect potentials should be regarded as an order of magnitude rather than a numerical
correct scientific number.
Further, as discussed in section 9.5, the methodology uncertainties are probably more important in connection with assessing toxicity in LCA and thereby also in establishing normalisation references. For
instance, it seems strange that almost the entire toxicity via the water environment is caused by deposition of mercury emitted to air, and it also seems questionable that 1,3-butadiene – the most important
nmVOC species - is responsible for a main part of the toxicity via air, being highly reactive and therefore having only a short life in the environment.
Many of the problems discussed above will probably disappear when the EU-funded OMNIITOX-project is completed in 2005. The project aims at creating a common method for inclusion of toxicity and
ecotoxicity in LCA and can therefore provide a significant step forward for the EDIP method as well as other impact assessment methods. The development in the project can be followed at
www.omniitox.net.
Therefore it seems necessary to carry out an in depth analysis of the EDIP LCA toxicity method, including identification of sensitive parameters therefore seems needed. Further, the most important EQFs in
relation to calculation of the normalisation references should be reviewed thoroughly in connection with a future updating.
9.7 References
Berdowski, J.J.M., Baas, J., Bloos, J.J., Visschedijk, A.J.H. & Zanveld, P.Y.J. 1997a, "The European atmospheric emission inventory of heavy metals and persistent organic pollutants for 1990".
Forschungsbericht 104 02 672/03. TNO Institute of Environmental Sciences, Energy Research and Process Innovation and Umweltbundesamt (UBA), Germany.
Berdowski, J.J.M., Mulder, W., Veldt, C., Visschedijk, A.J.H., Zandveld, P.Y.J. 1997b, Particulate matter emissions (PM10 - PM2.5 - PM0.1) in Europe in 1990 and 1993. TNO-MEP - R 96/472.
TNO Institute of Environmental Sciences, Energy Research and Process Innovation, The Netherlands.
Blonk, H. (ed.), Lafleur, M., Spriensma, R., Stevens, S., Goedkoop, M., Agterberg, A., van Engelenburg, B. & Blok, K. 1997, Drie referentieniveaus voor normalisatie in LCA: Nederlands
grondgebied 1993/1994; Nederlandse eindconsumptie 1993/1994; West-Europees grondgebied begin jaren 1990. RIZA werkdocument 97.110x. Ministerie van Verkeer en Waterstaat,
Directoraat-General Rijkswaterstaat, RIZA Rijksinstituut voor Integraal Zoetwaterbeheer en Afvalwaterbehandlung & Ministerie van Volkshuisvesting Ruimtelijke Ordening en Milieubeheer,
Directoraat-General Miljieubeheer.
Drivsholm, T., Holm-Petersen, M., Skårup, S., Frees, N., & Olsen, S. 2002. Produkters forbrug af transport. Systemanalyse. Working report No. 44. Danish Environmental Protection Agency,
Copenhagen.
EC- TGD 1996, Technical guidance documents in support of commission directive 93/67/EEC on risk assessment for new notified substances and commission regulation (EC) No1488/94 on
risk assessment for existing substances (Parts I, II, III and IV). Office for Official Publications of the European Community, Luxembourg.
EEA 1997, Air pollution in Europe 1997. European Environmental Agency. Environmental Monograph No. 4. Copenhagen. ISBN 92-9167-059-6.
EEA 1998a, Europe's Environment: The Second Assessment. Eurostat, European Commission, European Environment Agency. Office for Official Publications of the European Commission, Luxembourg.
EEA 1998b,. Europe's Environment: statistical compendium for the Second Assessment. Eurostat, European Commission, European Environment Agency. Office for Official Publications of the
European Commission, Luxembourg.
EMEP/MSC-W 1998a, Transboundary acidifying air pollution in Europe. Part 1: Estimated dispersion of acidifying and eutrophying compounds and comparison with observations. Meteorological
Synthesising Centres West. Report 1/98.
EMEP/MSC-W 1998b, Transboundary acidifying air pollution in Europe. Part 2: Numerical Addendum. Meteorological Synthesising Centres West. Report 1/98.
EMEP/MSC-W 1997, Photochemical oxidant modelling in Europe: multi-annual modelling and source-receptor relationships. Meteorological Synthesising Centres West. Report 3/97.
EMEP/MSC-E 1998a, Long-range transport of selected persistent organic pollutants, Part I. Meteorological Synthesising Centres East. Report 2/98.
EMEP/MSC-E 1998b, Mercury in the atmosphere of Europe: concentrations, deposition patterns, transboundary fluxes. Meteorological Synthesising Centres East. Report 7/98.
EMEP/MSC-E 1998c, Modelling of long-range transport of lead and cadmium from European sources in 1996. Meteorological Synthesising Centres East. Report 5/98.
Hauschild, M. 1996, Baggrund for miljøvurdering af produkter. Miljø- og Energiministeriet, Dansk Industri. Copenhagen. (in Danish)
Hauschild, M.Z., Olsen, S.I. & Wenzel, H. 1996, Toksicitet for mennesker i miljøet som vurderingskriterium ved miljøvurdering af produkter, i Hauschild, M.Z. (ed.), Baggrund for miljøvurdering af
produkter. Miljø- og Energiministeriet, Dansk Industri. København. (in Danish)
Hauschild, M.Z., Olsen, S.I. & Wenzel, H. 1998, Human toxicity as a criterion in the environmental assessment of products. In Hauschild M, Wenzel H (eds.). Environmental assessment of products.
Volume 2: Scientific background. London: Chapman & Hall
Hauschild, M. & Wenzel, H. 1998, Environmental Assessment of Products. Volume 2 - Scientific background. First edition. Chapman & Hall, London.
Jensen, A.A. 1997, Dioxins. Sources, levels and exposures in Denmark. Working report No. 50. Danish Environmental Protection Agency, Copenhagen.
Kirschstetter, T.W., Singer, B.C, Harley, R.A., Kendall, G.R., Hesson, J.M. 1999. Impact of California reformulated gasoline on motor vehicle emissions. 2. Volatile organic compound speciation
and reactivity. Environ. Sci. Technol. 1999 (33): 329-336.
Koch, D. 1998, Air emissions - Annual topic update 1997. Topic Report no.4. European Environment Agency.
Nielsen, T., Jørgensen, H.E., Poulsen, M., Jensen, F.P., Larsen, J.C., Poulsen, M., Jensen, A.B., Schramm, J. & Tønnesen, J. 1995, Traffic PAH and other mutagens in air in Denmark.
Environmental Project no. 285. Danish Environmental Protection Agency, Copenhagen.
Nielsen, T., Jørgensen, H.E., Larsen, J.C. & Poulsen, M. 1996, City air pollution of polycyclic aromatic hydrocarbons and other mutagens: occurrence, sources and health effects. The science of
the total environment 189/190: 41-49.
Quass, U. & Fermann, M. 1997, Identification of relevant industrial sources of dioxins and furans in Europe - The European Dioxin Inventory. Final Report. B4-3040/94/884/AO/A3. Materialen
No. 43. Essen: Landesumweltamt Nordrhein-Westfalen.
Ritter, M. 1997, Corinair 94 - summary report - European Emission Inventory for Air Pollutants. European Topic Centre on Air Emissions. European Environment Agency, Copenhagen.
RIVM, VROM, VWS 1998, Uniform system for the evaluation of substances 2.0 (USES 2.0). National Institute of Public Health and the Environment (RIVM), Ministry of Housing, Spatial Planning
and the Environment (VROM), Ministry of Health, Welfare and Sport (VWS), The Netherlands. RIVM report no. 679102044
UN-ECE 1979, Convention on long-range transboundary air pollution. United Nations, Economic Commission for Europe.
US EPA 1996, Air quality criteria for particulate matter. United States, Environmental Protection Agency.
van der Auweraert, R.J.K., Berdowski, J.J.M., Jonker, W.J. & Verhoeve, P. 1996, Emissies in Nederland Bedreijfgroepen en Regio's 1994 en Rammen 1995. Publicatiereeks Emissieregistratie Nr. 33.
`s-Gravenhage: Hoofdinspectie Milieuhygiëne /IPC 680.
van der Ven, B. 1995, Default values. A discussion paper for the meeting of the SETAC workgroup "Data handling" on Sep. 4th 1995 at Gothenborg.
Appendix A: Data sources
Overview of data sources and contacts for air emissions
Overview of substances included in the normalisation and corresponding data sources.
Substances |
Reference/source |
SO2, NOx, nmVOC, CO, N2O, NH3,
heavy metals1, POP1 |
•Corinair 94 (Ritter 1997)/ Internet: www.aeat.co.uk/netcen
•Europe's Environment second assessment (EEA, 1998a,b)
•Eurostat: ESEPI (Index-project)- Draft reports (Eurostat 1998)
•EMEP-reports (EMEP/MSC-W 1997, 1998a,b; EMEP/MSC-E 1998a,b,c)
•More detailed heavy metal emission data in Koch (1998)
•Older (1990) and more detailed data can be found in Berdowski et al. (1997a) for heavy metals and POPs and for a number of substances on
ftp://inforivmnl/pub/lae/edgarv20/.
•Dioxins- Europe: The European Dioxin inventory (Quass & Fermann 1997)- DK: Dioxin. Sources, levels and exposures in Denmark (Jensen
1997)
•PAH- "Traffic PAH and other Mutagens in Air in Denmark" (Nielsen et al. 1995)- DMU, Denmark (Jytte Boll Illerup, DMU,
personalcommunication)
|
Particles, PM10 |
•TNO report (Berdowski et al. 1997b)
•Air pollution in Europe 1997 (EEA 1997)
|
Chemicals/chemical industry |
•Dutch emission registrations (van der Auweraert et al. 1996)
•Dutch normalisation report (Blonk et al. 1997)
|
1 Only available for D, UK, A, DK, L, SCH, N in CorinAir94.
Relevant contacts in relation to obtaining data on Danish and European air emissions:
- EMEP - co-operative programme for monitoring and evaluation of the long range transmission of air pollutants in Europe. Contact:
Det Norske Meteorologiske Institutt (DNMI)
Postboks 43, Blindern,
0313 Oslo
Phone 47 22 96 30 00
Fax 47 22 96 30 50
e-post: met.inst@dnmi.no
http:/www.dnmi.no
- TNO - Department for Ecological Risk Studies:
TNO Institute of Environmental Sciences, Energy Research and Process Innovation
Business Park Environmental Technology Valley
Laan van Westenenk 501
7334 DT Apeldoorn
Netherlands
Telephone: +31 (55) 549 3493
Fax:+31 (55) 541 9837
E-mail:B.A.Heide@mep.tno.nl
http://www.mep.tno.nl
- ETC Air Emissions
Umweltbundesamt UBA
Contact person: Dietmar Koch
Bismarckplatz 1
D-14193 Berlin
Germany
Phone: [49] 30 8903 2392
Fax: [49] 30 8903 2178
E-mail: dietmar.koch@uba.de
Homepage: http://www.aeat.co.uk/netcen
- Danish National Reference Centre for Air Emissions
National Environmental Research Institute
Department of Policy Analysis
Contact person: Jytte Boll Illerup
Frederiksborgvej 399
P.O. Box 358
DK-4000 Roskilde
Denmark
Phone: 45 46 30 12 00
Fax: 45 46 30 11 14
E-mail: jbi@dmu.dk
Homepage: http://www.dmu.dk
- European Environment Agency (EEA)
Kongens Nytorv 6
DK-1050 København K
Denmark
Phone: 45 33 36 71 00
Fax: 45 33 36 71 99
E-mail: eea@eea.eu.int
Homepage: http://www.eea.eu.int
- National EU focal points
- Dutch LCA methodological project
Information and contact details can be found on: http://www.leidenuniv.nl
Appendix B.1: Extrapolations and data handling
This appendix gives a survey of the air emission data (including the availability of data) used in the estimation of the total potential human toxicological impact in EU-15; see Table 9-11. Information on air
emissions is available for a number of European countries in EU-15 as well as outside EU-15. For the present purpose it is decided to use data from Norway and Switzerland (for heavy metals and
persistent organic pollutants) in order to get a better basis for extrapolation to EU-15. Below, a general extrapolation method will be presented followed by comments to the data used to estimate the total
potential human toxicological impact in EU-15.
General considerations concerning extrapolation from single countries or groups of countries to EU-15 and from groups of countries to the world is presented in chapter 3, Development of normalisation
references for different geographic areas.
As extrapolation factor GDP [15] is chosen and a general formula can be outlined:
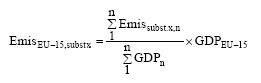
n is the countries with available information on emission of substance (subst x.).
An example on extrapolation to EU-15 is shown in the box below. Emissions of TRI (trichloroethylene) from the following countries are included: Denmark, Germany, Netherlands, Norway, and United
Kingdom.

The applied extrapolation methodology has not been verified and therefore it has to be considered as rather uncertain. However, the present extrapolation method is supposed to be as certain as any other
methodology.
Information on emission of the general air pollutants (SO2, NOx, N2O, CO and nmVOC) is available in a number of sources (e.g. CORINAIR 94 [16] (Ritter 1997)) due to different conventions on air
pollution (e.g. Con.vention on Long-range Transboundary Air Pollution (UN-ECE 1979)). ETC/AE [17] has calculated total emissions for EU-15 for the above mentioned substances. The quality of
the summary data depends on the quality and level of detail of the data delivered by the single countries. The report uses three levels of quality/details with the countries in the different groups as described
below:
- A (the emission estimates are fully detailed): Austria, Belgium, Denmark, Greece, Ireland, Luxembourg, Sweden, United Kingdom
- B (the emission estimates are detailed): Germany, Netherlands
- C (the emission data cover the main source sectors): Finland, France, Italy, Portugal, Spain
These emissions are measured regularly. This part of the estimation of the total potential human toxicological impact in EU-15 can therefore be updated regularly if preferred.
Information on emission of heavy metals (As, Cd, Cr, Cu, Hg, Ni, Pb, Se, Zn) is available in e.g. CORINAIR 94 (Ritter 1997) supplemented by 95-update (Koch 1998) due to different conventions
on air pollution (e.g. "Convention on Long-range Transboundary Air Pollution" (UN-ECE 1979)).
Data on emissions of heavy metals are only available for few countries in EU-15 (for 1994), and therefore data from Norway and Switzerland will be included in the estimates of the total potential
human toxicological impact in EU-15. Norway and Switzerland are supposed to be representative for EU-15. The 95-update also includes emission estimates from Greece, Italy, Netherlands and
Sweden. The quality of the information varies due to less developed estimation methodologies etc. The levels of quality/details is described below with the countries in the different groups:
- A (the emission estimates are fully detailed): Norway, United Kingdom
- B (the emission estimates are detailed): Austria, Germany, Switzerland
- C (the emission data cover the main source sectors): Denmark, Luxembourg
In addition to the data reported by CORINAIR, data on emissions of heavy metals in Netherlands (van der Auweraert et al. 1996) are included in the estimate.
Information on emission of persistent organic pollutants (POP's) is available in e.g. CORINAIR 94 (Ritter 1997) due to different conventions on air pollution (e.g. "Convention on Long-range
Transboundary Air Pollution" (UN-ECE 1979)). The group of persistent organic pollutants included in CORINAIR consist of polyaromatic hydrocarbons (PAH), dioxins, pentachlorophenol
(PCP), hexachlorobenzene (HCB), tetrachloromethane (TCM), trichloroethylene (TRI), tetrachloroethylene (PER), trichlorobenzene (TCB), trichloroethane (TCE) and hexachlorobenzene
(HCB).
Data on emissions of persistent organic pollutants are only available for few countries in EU-15, and therefore data from Norway and Switzerland will be included in the estimates of the total potential
human toxicological impact in EU-15. The quality of the information varies due to less developed estimation methodologies etc. The levels of quality/details is described below with the countries in
the different groups:
- A (the emission estimates are fully detailed): Norway, United Kingdom
- B (the emission estimates are detailed): Austria, Denmark, Germany, Luxembourg, Switzerland
In addition to the data reported by CORINAIR data on emissions of persistent organic pollutant in Netherlands (van der Auweraert et al. 1996) is included in the estimate.
Additional data have also been used for dioxins. EU/DG XI has financed "The European Dioxin Inventory" (Quass & Fermann 1997). Data were only taken from this source if they were not available in
CORINAIR, or if they were considered better than the data in CORINAIR and dated from the chosen reference years 1994 or 1995 (i.e. Belgium, France, Norway and Sweden). CORINAIR includes
dioxin data from Austria, Denmark, Germany, Luxembourg, Switzerland and UK.
PAH as well as dioxins are groups of substances of different toxicity. In order to sum up the human toxicological potential of PAH and dioxins to a single number, toxic equivalence factors have been
developed. By using these equivalence factors, the total PAH emission can be expressed as benzo(a)pyrene equivalents and the total dioxin emission can be expressed as 2,3,7,8-tetrachloro-p-dioxins
equivalents (2,3,7,8-TCDD-eq. (I-TEQ)). The dioxin equivalence factors have been used for a number of years and are internationally accepted whereas the PAH equivalence factors are still under
development. This means that emissions of dioxins are normally reported in I-TEQ whereas emissions of PAH are still reported as PAH.
By using the PAH and the equivalence factor for benzo(a)pyrene for all the substances, the potential human toxicological impact of PAH will be overestimated. Therefore, detailed information on distribution
of different PAH's is necessary in order to calculate a more reliable potential human toxicological impact of PAH. Detailed information on the distribution of PAH's is available for Denmark; see Table 9-10.
Table 9-10
Emissions of PAH in Denmark. Benzo(a)pyrene equivalents are calculated by
using the relative potency proposed by Nielsen et al. (1996).
|
Relative potency |
ton/year |
ton PAH-eq./year |
PAH |
|
3,70E+01 |
|
Fluoranthen |
0,05 |
2,30E+01 |
1,15E+00 |
Benzo(b)fluoranthen |
0,05 |
3,62E+00 |
1,81E-01 |
Benzo(k)fluoranthen |
0,05 |
1,36E+00 |
6,80E-02 |
Benzo(a)pyrene |
1 |
2,67E+00 |
2,67E+00 |
Benzo(g,h,i)perylene |
0,02 |
4,29E+00 |
8,58E-02 |
Indino(1,2,3-c,d)pyrene |
0,1 |
2,29E+00 |
2,29E-01 |
PAH-eq. (benzo(a)pyrene) |
|
|
4,38E+00 |
The total PAH emission ( PAH) is reported to 37 ton/year and the emission expressed as benzo(a)pyrene equivalents is estimated to 4,4 ton benzo(a)pyrene-equivalents/year. Assuming that the distribution
of the PAH's found in Denmark is representative, a correction factor can be determined to:
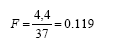
This correction factor F is used to estimate the emission benzo(a)pyrene equivalents for the countries that only have reported PAH.
Table 9-11
Air emission data available for extrapolation to EU-15.
Substance |
A |
B |
D |
DK |
E |
F |
GR |
I |
IR |
L |
NL |
P |
S |
SF |
UK |
EU-15 |
N |
SCH |
SO21 |
|
|
|
|
|
|
|
|
|
|
|
|
|
|
|
x |
|
|
NOx1 |
|
|
|
|
|
|
|
|
|
|
|
|
|
|
|
x |
|
|
N2O1 |
|
|
|
|
|
|
|
|
|
|
|
|
|
|
|
x |
|
|
CO1 |
|
|
|
|
|
|
|
|
|
|
|
|
|
|
|
x |
|
|
nmVOC1 |
|
|
|
|
|
|
|
|
|
|
|
|
|
|
|
x |
|
|
NmVOC – transport1 |
|
|
|
|
|
|
|
|
|
|
|
|
|
|
|
x |
|
|
Cd |
x |
|
x |
x |
|
|
95 |
95 |
|
|
x |
|
95 |
|
x |
|
x |
95 |
As |
x |
|
x |
x |
|
|
95 |
95 |
|
|
x |
|
95 |
|
x |
|
x |
|
Cr(VI) |
x |
|
x |
x |
|
|
95 |
95 |
|
|
x |
|
95 |
|
x |
|
x |
|
Hg |
x |
|
x |
x |
|
|
95 |
95 |
|
|
x |
|
95 |
|
x |
|
x |
95 |
Ni |
x |
|
x |
x |
|
|
95 |
95 |
|
|
x |
|
95 |
|
x |
|
x |
|
Pb |
x |
|
x |
x |
|
|
95 |
95 |
|
|
x |
|
95 |
|
x |
|
x |
95 |
Se |
x |
|
x |
x |
|
|
95 |
95 |
|
|
x |
|
95 |
|
x |
|
x |
|
Cu |
x |
|
x |
x |
|
|
95 |
95 |
|
|
x |
|
95 |
|
x |
|
x |
|
Zn |
x |
|
x |
x |
|
|
95 |
95 |
|
|
x |
|
95 |
|
x |
|
x |
95 |
Formaldehyde |
|
|
|
|
|
|
|
|
|
|
(x) |
|
|
|
|
|
|
|
Benzene |
|
|
|
|
|
|
|
|
|
|
(x) |
|
|
|
|
|
|
|
Phenol |
|
|
|
|
|
|
|
|
|
|
(x) |
|
|
|
|
|
|
|
Styrene |
|
|
|
|
|
|
|
|
|
|
(x) |
|
|
|
|
|
|
|
Toluene |
|
|
|
|
|
|
|
|
|
|
(x) |
|
|
|
|
|
|
|
Xylenes |
|
|
|
|
|
|
|
|
|
|
(x) |
|
|
|
|
|
|
|
PAH |
(x) |
|
(x) |
(x) |
|
|
|
|
|
|
|
|
|
|
(x) |
|
(x) |
|
Fluoranthen |
|
|
|
x |
|
|
|
|
|
|
x |
|
|
|
|
|
|
|
Benzo(b)fluoranthen |
|
|
|
x |
|
|
|
|
|
|
|
|
|
|
|
|
|
|
Benzo(k)fluoranthen |
|
|
|
x |
|
|
|
|
|
|
|
|
|
|
|
|
|
|
Benzo(a)pyrene |
|
|
|
x |
|
|
|
|
|
|
x |
|
|
|
|
|
|
|
Benzo(g,h,i)perylene |
|
|
|
x |
|
|
|
|
|
|
|
|
|
|
|
|
|
|
Indino(1,2,3-c,d)pyrene |
|
|
|
x |
|
|
|
|
|
|
|
|
|
|
|
|
|
|
PAH-eq. (benzo(a)pyrene) |
x |
|
x |
x |
|
|
|
|
|
|
x |
|
|
|
x |
|
x |
|
Dioxin |
x |
x |
x |
x |
|
x |
|
|
|
|
|
|
x |
|
x |
|
x |
x |
PCP |
|
|
|
|
|
|
|
|
|
|
|
|
|
|
x |
|
x |
|
Hexachlorbenzene (HCB) |
|
|
x |
|
|
|
|
|
|
|
|
|
|
|
x |
|
x |
|
Tetrachloromethane (TCM) |
|
|
|
x |
|
|
|
|
|
|
x |
|
|
|
x |
|
x |
|
Trichloroethylene (TRI) |
|
|
x |
x |
|
|
|
|
|
|
x |
|
|
|
x |
|
x |
|
Tetrachloroethylene (PER) |
|
|
x |
x |
|
|
|
|
|
|
x |
|
|
|
x |
|
x |
|
Trichlorobenzene (TCB) |
|
|
|
|
|
|
|
|
|
|
|
|
|
|
x |
|
x |
|
Trichloroethane (TCE) |
|
|
x |
x |
|
|
|
|
|
|
x |
|
|
|
x |
|
x |
|
Hexachlorohexane (HCH) |
|
|
|
x |
|
|
|
|
|
|
|
|
|
|
x |
|
x |
|
Chlorbenzenes |
|
|
|
|
|
|
|
|
|
|
(x) |
|
|
|
|
|
|
|
Vinylchloride |
|
|
|
|
|
|
|
|
|
|
x |
|
|
|
|
|
|
|
Particulate matter (PM10) |
|
|
|
|
|
|
|
|
|
|
x |
|
|
|
|
|
|
|
- Emission data are available for all the European countries.
x means that the data is used to estimate EU-15 emission.
(x) means that the data are available but are not used in the estimation of EU-15 emission in this edition; the PAH results are supposed to be PAH and therefore PAH-equivalents (benz(a)pyrene) has been
calculated.
Appendix B.2: Effect factors
Particulate matter (PM10)
Particulate matter is a chemical inhomogeneous group, and the toxicological assessment is not directly linked with the chemical composition. In most cases the heavy metals or acids emitted as particulate
matter are measured as specific substances. The toxicity of particulate matter is linked with inhalation.
Therefore, the toxicity of particulate matter is only relevant for emissions to air end direct exposure via air i.e. by inhalation.
The EQF has been determined for particulate matter (< 10µm). The EQF is based on air quality criteria: 50 µg/m3 (annual average) (US EPA 1996). The air quality criteria expressed as annual average is
assumed to be equivalent to the concentration having no adverse effects throughout a whole life i.e. equal to the human reference concentration (HRC).
Emission to air
Substance
|
CAS no. |
EF(hta)
m3/g
|
faa |
HRC
mg/m3
|
Particulate matter (PM10) |
n.a. |
2*104 |
1 |
0.050 |
It is emphasized that the suggested effect factor for particulate matter may underestimate the contribution significantly. Recent research points to fine particles (less than 2.5 µm) and ultrafine particles (less
than 0.1 µm) as being of even more concern than PM10. Drivsholm et al. (2002) arguess that the effect factor should be significantly higher (5.5 * 108 m3/g) if both physical and toxic effects from inhalation
of particles is taken into consideration.
As discussed in Drivsholm et al. (2002), modern motors may actually emit more fine particles than conventional motors. However, there is no science-based information available that allows to calculate a
precise effect factor for particles from different types of vehicles/fuels. Nor is it possible to calculate a normalisation reference because there is no information available on the amounts of different particle
sizes being emitted from human activities.
The toxicity of particulate matter should therefore be kept in focus for future updates of the normalisation reference. In the meantime, the normalisation references and the effect factors developed in this
project should be used with caution.
nmVOC from road transport
Effect factors for nmVOC from road transport, jet engines and ferries were established by using information from Corinair94 (Ritter, 1997) regarding their composition and by using the existing effect factors
for the single VOC-species where available and supplementing with the default factor used in the original EDIP97-calculations, i.e. 10.000 m3/g for all species without a specific effect factor. Table 9-12
sums up the established effect factors and the most important species, while Table 9-17 to Table 9-19 gives a detailed overview of the composition and the importance for a number of fuel/vehicles.
Fuel/Vehicle |
Effect factor ht(a)
(m3/g)
|
Most important species |
Diesel – HDV |
1.06 E+7 |
1,3-butadien (80%)
Formaldehyde (10%)
Acrolein (8%)
|
Diesel – LDV |
6.9 E+6 |
1,3-butadien (35%)
Acrolein (26%)
Formaldehyde (22%)
Acetone (12%)
|
Gasoline – EURO I |
3.35 E+6 |
1,3-butadien (68%)
Benzene (17%)
Formaldehyde (6%)
Acetone (5%)
|
Gasoline – Conventional motors |
4.65 E+6 |
1,3-butadien (76%)
Benzene (15%)
Formaldehyde (6%)
|
Gasoline – Evaporation |
1.09 E+5 |
Benzene (92%)
Isopentane (2%)
|
LPG |
1.19 E+6 |
1,3-butadien (32%)
Acrolein (25%)
Formaldehyde (16%)
|
Jet engines (Landing-Take off Cycle) |
8.33 E+6 |
1-pentene (55%)
Acrolein (23%)
Other aldehydes (14%)
|
Ferries (can be used as surrogate for nmVOC from ship transport) |
4.1 E+5 – 3.5 E+6 |
Benzene (97.9 –99.9%) |
Table 9-12. Effect factors for human toxicity (air) for nmVOC from different fuels/vehicles
A similar approach was applied in a system analysis of road transport in Denmark (Drivsholm et al, 2002). That report allows for a detailed comparison of the resulting effect factor for nmVOC from diesel
exhaust, and here there is a good accordance between the findings in the two projects. The main difference between the two calculations is that Drivsholm et al. identifies the most important species by a
qualitative approach whereas in the current project all species are included in the calculations.
The contribution to the updated normalisation references was established by combining different types of information from Corinair94 (Ritter, 1997; www.aeat.co.uk/netcen).
In Corinair, information is available regarding the amounts emitted from different sources (Table 9-13):
- Passenger cars (SNAP code 070100)
- Light duty vehicles < 3.5t (SNAP code 070200)
- Heavy duty vehicles > 3,5t and buses (SNAP code 070300)
- Mopeds and motorcycles < 50 cm3 (SNAP code 070400)
- Motorcycles > 50 cm3 (SNAP code 070500)
- Evaporation of gasoline
Four countries (Finland, Italy, Spain and Portugal) have not reported the distribution of nmVOC on the single SNAP code activities. For these four countries, it was assumed that the distribution is similar to
the average distribution for the other eleven countries. One country, Germany, does not report the amounts of nmVOC from evaporation. Here, it was assumed that the relationship between the total
amounts emitted from road transport and the amount evaporating is equal to the average proportion in the other fourteen countries.
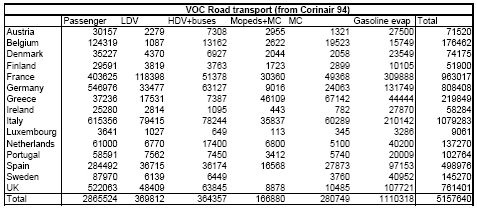
Table 9-13. nmVOC from road transport. Adapted from Corinair94 (Ritter, 1997).
The emission profiles differ significantly between diesel and gasoline fuels for the same type of vehicles, and also between countries for the same type of fuel/vehicle combination. In order to reflect these
differences, the total nmVOC emissions for each country were (re)distributed on the following types of vehicles (Table 9-14):
- Gasoline LDV
- Diesel LDV
- Gasoline PC
- Diesel PC
- Diesel HDV
- Mopeds and motorcycles
- Gasoline evaporation
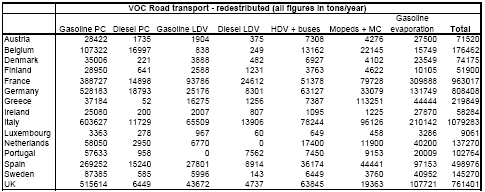
Table 9-14. nmVOC from road transport redistributed on different fuels/vehicles.
With the effect factors for different fuels/vehicles and the amounts of nmVOC being emitted from the same combinations in EU-15 countries, the overall contribution to human toxicity via air can be calculated
for each of the EU-15 countries (Table 9-15).
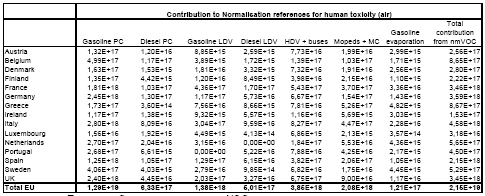
Table 9-15. Contribution from nmVOC – road transport to the normalisation references for human toxicity (air).
Table 9-16 shows the relative contribution from the different fuels/vehicles and illustrates clearly that the main contribution comes from passenger cars. As nmVOC from road transport is the main contributor
to human toxicity via air this means that when examining product systems (other than person transport), the relative importance of this impact category will be small, because person transport in general not is
included in inventories. When comparing alternatives, however, products that are demanding with respect to transportation will have a significantly larger impact when the newly established effect factors are
applied..
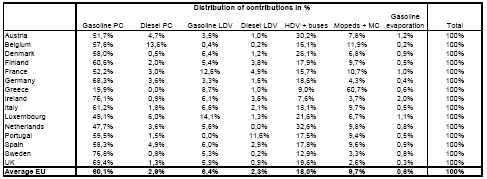
Table 9-16. Relative contribution from different fuels/vehicles
It is once more remarked that the uncertainty in the normalisation references is relatively high. The uncertainty is demonstrated by the fact that the Corinair inventory quotes two different inventories for the
composition of nmVOC from motor exhaust. They have an almost comparable level of detail, but with a little less detail in the information the effect factors for different types of fuels/vehicles decrease with
about 30%. This uncertainty is, however, within the general target of precision, i.e. to provide the right order of magnitude. This claim is supported when comparing the effect factors for different
fuels/vehicles with the effect factor for overall vehicle exhaust as measured in an American road tunnel. A detailed inventory for this can be found in Kirschstetter et al, 1998. When applying the same
calculation method as above, the effect factor for "combined exhaust" can be calculated to 2.44 E+6. This figure is smaller than that calculated for the individual types of fuels/vehicles, but is still within the
same order of magnitude.
It is also remarked that no attention was given to nmVOC from other cources of combustion, the reason being that detailed information on the composition was not available and/or that the contribution –
measured by weight – was small compared to the constribution from road traffic.
Click here to see the Table.
Table 9-17. Composition of nmVOC in exhaust from different fuels and vehicles
Click here to see the Table.
Table 9-18. Contribution from single nmVOC species to the effect factors for different fuels and vehicles
Click here to see the Table.
Table 9-19. Contribution to EF(hta) in percent
Effect factors for VOC from industrial processes
The Corinair94 Inventory allows for calculation of a number of effect factors related to the overall emissions of VOC (i.e. including methane) from specific types of plants. The level of detail is less than for
traffic related emissions and some of the key emissions are either not identified in the inventory or they are not characterized in the EDIP database. The figures in the following table should therefore only be
considered as crude estimates.
Process/plant type |
EF(hta) |
Most important emissions |
Comments |
Ethylene plants |
2.86E+5 |
Benzene: 92%
Methane: 5%
|
|
Styrene plants |
1.09E+5 |
Benzene: 98% |
|
Ethylbenzene production |
4.42E+6 |
Benzene: 99.9% |
EPA assumes that 100% of emissions are ethylbenzen, i.e. an EF(hta) of 1E+4 |
Polystyrene plants |
1.9E+3 |
Ethylbenzene: 53%
Styrene: 47%
|
|
Polypropylene plants |
1E+4 |
Propylene: 97% |
EF(hta) equals the default value for nmVOC emissions, because no specific
factors are available |
SB rubber |
3.25E+7 |
1,3-butadiene: 100% |
|
SB Latex |
6.25E+7 |
1,3-butadiene: 100% |
|
Kraft pulping |
9.76E+3 |
Methane: 24%
Ethane: 12%
butane: 10%
|
Most of the species have the default factor for nmVOC (1E+4) |
Table 9-20. Effect factors for VOC from industrial processes.
The effect factors can be integrated in the EDIP database and used with caution. Better – or more precise – estimates can be obtained by establishing effect factors for each of the species. This would most
probably mean a reduction in the effect factor for VOC from polypropylene plants, where the main emissions are propylene that is considered as almost harmless to humans.
Appendix C: Data and calculation of normalisation references
This appendix contains data used in the calculation of normalisation references concerning human toxicity. The appendix is split up in emissions contributing to respectively:
C.1 Human toxicity by inhalation
C.2 Human toxicity by intake via water
C.3 Human toxicity by intake via soil
References to the data source is given in the individual tables.
C.1.1 Denmark, normalisation reference, air
Click here to see the Table.
C.1.2 EU-15, normalisation reference, air
|
|
Effect factor |
|
EU15estm |
EU-15 |
EDIP revision |
|
|
|
EF, new |
Total |
|
1994 |
EP |
% |
|
|
|
ton/year |
ton/year |
ton/year |
|
|
Total |
|
|
|
|
|
2,25E+19 |
|
Population |
|
|
|
|
|
3,70E+08 |
|
Normalisation factor |
|
|
|
|
|
6,09E+10 |
|
|
|
|
|
|
|
|
|
Substance |
Ref. |
|
|
|
|
|
|
SO2 |
a |
1,30E+03 |
|
|
1,20E+07 |
1,57E+16 |
0,1 |
NOx |
a |
8,60E+03 |
|
|
1,25E+07 |
1,08E+17 |
0,5 |
N2O |
a |
2,00E+03 |
|
|
9,51E+05 |
1,90E+15 |
0,0 |
CO |
a |
8,30E+02 |
|
|
4,51E+07 |
3,74E+16 |
0,2 |
nmVOC |
a |
1,00E+04 |
|
|
7,42E+06 |
7,42E+16 |
0,3 |
nmVOC transport |
i |
|
3,31E+06 |
|
5,16E+06 |
2,15E+19 |
95,2 |
Cd |
|
1,10E+08 |
7,60E+01 |
1,04E+02 |
|
1,14E+16 |
0,1 |
As |
|
9,50E+06 |
1,96E+02 |
2,82E+02 |
|
2,68E+15 |
0,0 |
Cr(VI) |
|
1,00E+06 |
3,94E+02 |
5,66E+02 |
|
5,66E+14 |
0,0 |
Hg |
|
6,70E+06 |
1,02E+02 |
1,40E+02 |
|
9,35E+14 |
0,0 |
Ni |
|
6,70E+04 |
1,46E+03 |
2,09E+03 |
|
1,40E+14 |
0,0 |
Pb |
|
1,00E+08 |
5,17E+03 |
7,07E+03 |
|
7,07E+17 |
3,1 |
Se |
|
1,50E+06 |
2,22E+02 |
3,19E+02 |
|
4,79E+14 |
0,0 |
Cu |
|
5,70E+02 |
3,89E+02 |
5,60E+02 |
|
3,19E+11 |
0,0 |
Zn |
|
8,10E+04 |
5,01E+03 |
6,86E+03 |
|
5,56E+14 |
0,0 |
PAH-eq. (benzo(a)pyren) |
|
5,00E+07 |
2,33E+02 |
4,48E+02 |
|
2,24E+16 |
0,1 |
Dioxin |
|
2,90E+10 |
2,93E-03 |
3,65E-03 |
|
1,06E+14 |
0,0 |
PCP |
|
8,30E+03 |
5,55E+02 |
3,66E+03 |
|
3,04E+13 |
0,0 |
Hexachlorobenzene (HCB) |
|
8,30E+03 |
1,39E+00 |
3,25E+00 |
|
2,70E+10 |
0,0 |
Tetrachloromethane (TCM) |
|
2,90E+04 |
3,34E+03 |
1,55E+04 |
|
4,48E+14 |
0,0 |
Trichloroethylene (TRI) |
|
1,90E+04 |
3,33E+04 |
6,77E+04 |
|
1,29E+15 |
0,0 |
Tetrachloroethylene (PER) |
|
2,90E+04 |
2,57E+04 |
4,51E+04 |
|
1,31E+15 |
0,0 |
Trichlorobenzene (TCB) |
|
8,30E+03 |
6,29E+02 |
1,10E+03 |
|
9,15E+12 |
0,0 |
Trichloroethane (TCE) |
|
9,20E+02 |
5,70E+04 |
9,99E+04 |
|
9,19E+13 |
0,0 |
Hexachlorocyclohexane (HCH) |
|
8,30E+03 |
1,23E+02 |
7,19E+02 |
|
5,96E+12 |
0,0 |
Chlorobenzenes |
|
8,30E+03 |
|
|
|
|
|
Vinylchloride |
|
3,90E+05 |
1,10E+02 |
2,46E+03 |
|
9,59E+14 |
0,0 |
Particulate matter (PM10) |
d |
2,00E+04 |
|
|
2,89E+06 |
5,78E+16 |
0,3 |
Benzene (w) |
|
1,00E+07 |
|
3,69E+09 |
|
3,69E+16 |
0,2 |
Toluene (w) |
|
2,50E+03 |
|
9,24E+09 |
|
2,31E+13 |
0,0 |
Xylenes (w) |
|
6,70E+03 |
|
3,69E+09 |
|
2,47E+13 |
0,0 |
Hexachlorocyclohexane (HCH) (w) |
|
8,30E+03 |
|
1,53E+05 |
|
1,27E+09 |
0,0 |
Tetrachloroethylene (PER) (w) |
|
2,90E+04 |
|
1,83E+06 |
|
5,32E+10 |
0,0 |
Tetrachloromethane (TCM) (w) |
|
2,90E+04 |
|
7,74E+06 |
|
2,25E+11 |
0,0 |
Trichloroethane (TCE) (w) |
|
9,20E+02 |
|
1,31E+06 |
|
1,20E+09 |
0,0 |
Trichloroethylene (TRI) (w) |
|
1,90E+04 |
|
3,49E+06 |
|
6,63E+10 |
0,0 |
Trichloromethane (w) |
|
2,90E+04 |
|
5,28E+06 |
|
1,53E+11 |
0,0 |
Chlorobenzenes (w) |
|
8,30E+03 |
|
2,24E+06 |
|
1,86E+10 |
0,0 |
Hexachlorobenzene (HCB) (w) |
|
8,30E+03 |
|
3,16E+05 |
|
2,62E+09 |
0,0 |
PCP (w) |
|
8,30E+03 |
|
2,55E+05 |
|
2,12E+09 |
0,0 |
Trichlororobenzene (TCB) (w) |
|
8,30E+03 |
|
2,05E+04 |
|
1,70E+08 |
0,0 |
Chloroform (s) |
|
1,00E+05 |
|
2,97E+03 |
|
2,97E+08 |
0,0 |
Dichlorobenzene (s) |
|
8,30E+03 |
|
3,99E+04 |
|
3,32E+08 |
0,0 |
Tetrachloroethylene (PER) (s) |
|
2,90E+04 |
|
6,44E+04 |
|
1,87E+09 |
0,0 |
Trichloroethylene (TRI) (s) |
|
1,90E+04 |
|
1,06E+04 |
|
2,02E+08 |
0,0 |
Toluene (s) |
|
2,50E+03 |
|
7,58E+04 |
|
1,89E+08 |
0,0 |
a |
Ritter (1997). |
b |
van der Auweraert et al. (1996). |
c |
Blonk H et al. (1997). |
d |
Berdowski et al. (1997) |
e |
Quass & Fermann (1997) |
f |
Koch (1998) |
I |
This project |
C.1.3 Austria, normalisation reference, air
|
|
Effectfactor |
EDIP revision |
|
|
|
EF (h) |
1994 |
EP |
% |
|
|
|
ton/year |
|
|
Total |
|
|
|
2,67E+17 |
|
Population |
|
|
|
8,00E+06 |
|
Normalisation factor |
|
|
|
3,33E+10 |
|
|
|
|
|
|
|
Substance |
Ref. |
|
|
|
|
SO2 |
a |
1,30E+03 |
5,49E+04 |
7,13E+13 |
0,0 |
NOx |
a |
8,60E+03 |
1,71E+05 |
1,47E+15 |
0,6 |
N2O |
a |
2,00E+03 |
1,27E+04 |
2,53E+13 |
0,0 |
CO |
a |
8,30E+02 |
1,18E+06 |
9,80E+14 |
0,4 |
nmVOC |
a |
1,00E+04 |
2,18E+05 |
2,18E+15 |
0,8 |
nmVOC - transport |
i |
|
7,15E+04 |
2,56E+17 |
95,9 |
Cd |
a |
1,10E+08 |
2,72E+00 |
2,99E+14 |
0,1 |
As |
a |
9,50E+06 |
3,26E+00 |
3,10E+13 |
0,0 |
Cr(VI) |
a |
1,00E+06 |
6,62E+00 |
6,62E+12 |
0,0 |
Hg |
a |
6,70E+06 |
2,18E+00 |
1,46E+13 |
0,0 |
Ni |
a |
6,70E+04 |
3,55E+01 |
2,38E+12 |
0,0 |
Pb |
a |
1,00E+08 |
2,43E+01 |
2,43E+15 |
0,9 |
Se |
a |
1,50E+06 |
4,71E+00 |
7,06E+12 |
0,0 |
Cu |
a |
5,70E+02 |
9,24E+00 |
5,26E+09 |
0,0 |
Zn |
a |
8,10E+04 |
2,08E+02 |
1,69E+13 |
0,0 |
PAH |
a |
5,00E+07 |
4,58E+02 |
|
0,0 |
PAH-eq. (benzo(a)pyren) |
estm. |
5,00E+07 |
5,43E+01 |
2,71E+15 |
1,0 |
Dioxin |
a |
2,90E+10 |
2,90E-05 |
8,41E+11 |
0,0 |
PCP |
|
8,30E+03 |
|
0,00E+00 |
0,0 |
Particulate matter (PM10) |
d |
2,00E+04 |
3,70E+04 |
7,40E+14 |
0,3 |
a |
Ritter (1997). |
b |
van der Auweraert et al. (1996). |
c |
Blonk H et al. (1997). |
d |
Berdowski et al. (1997) |
e |
Quass & Fermann (1997) |
f |
Koch (1998) |
g |
Hauschild et al. (1996) |
h |
Hauschild et al. (1998) |
i |
This project |
C.1.4 Germany, normalisation reference, air
|
|
Effectfactor |
|
EDIP revision |
|
|
|
EF (h) |
1994 |
EP |
% |
|
|
|
ton/year |
|
|
Total |
|
|
|
3,73E+18 |
|
Population |
|
|
|
8,11E+07 |
|
Normalisation factor |
|
|
|
4,60E+10 |
|
|
|
|
|
|
|
Substance |
Ref. |
|
|
|
|
SO2 |
a |
1,30E+03 |
3,00E+06 |
3,90E+15 |
0,1 |
NOx |
a |
8,60E+03 |
2,27E+06 |
1,95E+16 |
0,5 |
N2O |
a |
2,00E+03 |
2,18E+05 |
4,37E+14 |
0,0 |
CO |
a |
8,30E+02 |
6,80E+06 |
5,64E+15 |
0,2 |
nmVOC |
a |
1,00E+04 |
1,34E+06 |
1,34E+16 |
0,4 |
nmVOC - transport |
i |
|
8,08E+05 |
3,59E+18 |
96,3 |
Cd |
a |
1,10E+08 |
1,07E+01 |
1,18E+15 |
0,0 |
As |
a |
9,50E+06 |
3,25E+01 |
3,08E+14 |
0,0 |
Cr(VI) |
a |
1,00E+06 |
1,15E+02 |
1,15E+14 |
0,0 |
Hg |
a |
6,70E+06 |
3,11E+01 |
2,08E+14 |
0,0 |
Ni |
a |
6,70E+04 |
1,57E+02 |
1,05E+13 |
0,0 |
Pb |
a |
1,00E+08 |
6,25E+02 |
6,25E+16 |
1,7 |
Se |
a |
1,50E+06 |
2,52E+01 |
3,78E+13 |
0,0 |
Cu |
a |
5,70E+02 |
7,91E+01 |
4,51E+10 |
0,0 |
Zn |
a |
8,10E+04 |
4,51E+02 |
3,66E+13 |
0,0 |
PAH-eq. (benzo(a)pyren) |
estm. |
5,00E+07 |
4,98E+01 |
2,49E+15 |
0,1 |
Dioxin |
a |
2,90E+10 |
3,07E-04 |
8,90E+12 |
0,0 |
PCP |
|
8,30E+03 |
|
0,00E+00 |
0,0 |
Hexachlorobenzene (HCB) |
a |
8,30E+03 |
9,00E-02 |
7,47E+08 |
0,0 |
Tetrachloromethane (TCM) |
|
2,90E+04 |
|
0,00E+00 |
0,0 |
Trichloroethylene (TRI) |
a |
1,90E+04 |
1,10E+04 |
2,09E+14 |
0,0 |
Tetrachloroethylene (PER) |
a |
2,90E+04 |
1,17E+04 |
3,39E+14 |
0,0 |
Trichlorobenzene (TCB) |
|
8,30E+03 |
|
0,00E+00 |
0,0 |
Trichloroethane (TCE) |
a |
9,20E+02 |
3,03E+04 |
2,79E+13 |
0,0 |
Particulate matter (PM10) |
d |
2,00E+04 |
1,32E+06 |
2,64E+16 |
0,7 |
a | Ritter (1997). |
b | van der Auweraert et al. (1996). |
c | Blonk H et al. (1997). |
d | Berdowski et al. (1997) |
e | Quass & Fermann (1997) |
f | Koch (1998) |
i | This project |
C.1.5 Greece, normalisation reference, air
|
|
Effect factor |
Emission |
EDIP revision |
|
|
|
EF (h) |
1994 |
EP |
% |
|
|
|
ton/year |
|
|
Total |
|
|
|
8,74E+17 |
|
Population |
|
|
|
1,04E+07 |
|
Normalisation factor |
|
|
|
8,40E+10 |
|
|
|
|
|
|
|
Substance |
Ref. |
|
|
|
|
SO2 |
a |
1,30E+03 |
5,56E+05 |
7,23E+14 |
0,1 |
NOx |
a |
8,60E+03 |
3,57E+05 |
3,07E+15 |
0,4 |
N2O |
a |
2,00E+03 |
1,47E+04 |
2,95E+13 |
0,0 |
CO |
a |
8,30E+02 |
1,29E+06 |
1,07E+15 |
0,1 |
nmVOC |
a |
1,00E+04 |
1,42E+05 |
1,42E+15 |
0,2 |
nmVOC - transport |
i |
|
2,20E+05 |
8,67E+17 |
99,2 |
Cd |
f |
1,10E+08 |
2,13E+00 |
2,34E+14 |
0,0 |
As |
f |
9,50E+06 |
4,32E+00 |
4,10E+13 |
0,0 |
Cr(VI) |
f |
1,00E+06 |
9,22E+00 |
9,22E+12 |
0,0 |
Hg |
f |
6,70E+06 |
1,24E+01 |
8,28E+13 |
0,0 |
Ni |
f |
6,70E+04 |
8,80E+01 |
5,90E+12 |
0,0 |
Pb |
f |
1,00E+08 |
7,19E+00 |
7,19E+14 |
0,1 |
Se |
f |
1,50E+06 |
8,49E-01 |
1,27E+12 |
0,0 |
Cu |
f |
5,70E+02 |
1,63E+01 |
9,26E+09 |
0,0 |
Zn |
f |
8,10E+04 |
2,08E+01 |
1,68E+12 |
0,0 |
Particulate matter (PM10) |
d |
2,00E+04 |
5,50E+01 |
1,10E+12 |
0,0 |
a | Ritter (1997). |
b | van der Auweraert et al. (1996). |
c | Blonk H et al. (1997). |
d | Berdowski et al. (1997) |
e | Quass & Fermann (1997) |
f | Koch (1998) |
g | Hauschild et al. (1996). |
h | Hauschild et al. (1998). |
i | This project |
C.1.6 Italy, normalisation reference, air
|
|
Effect factor |
Emission |
EDIP revision |
|
|
|
EF (h) |
1994 |
EP |
% |
|
|
|
ton/year |
|
|
Total |
|
|
|
4,85E+18 |
|
Population |
|
|
|
5,70E+07 |
|
Normalisation factor |
|
|
|
8,51E+10 |
|
|
|
|
|
|
|
Substance |
Ref. |
|
|
|
|
SO2 |
a |
1,30E+03 |
1,44E+06 |
1,87E+15 |
0,0 |
NOx |
a |
8,60E+03 |
2,16E+06 |
1,86E+16 |
0,4 |
N2O |
a |
2,00E+03 |
1,31E+05 |
2,62E+14 |
0,0 |
CO |
a |
8,30E+02 |
9,23E+06 |
7,66E+15 |
0,2 |
nmVOC |
a |
1,00E+04 |
1,16E+06 |
1,16E+16 |
0,2 |
nmVOC - transport |
i |
|
1,08E+06 |
4,58E+18 |
94,5 |
Cd |
f |
1,10E+08 |
2,99E+01 |
3,29E+15 |
0,1 |
As |
f |
9,50E+06 |
3,81E+01 |
3,62E+14 |
0,0 |
Cr(VI) |
f |
1,00E+06 |
1,63E+02 |
1,63E+14 |
0,0 |
Hg |
f |
6,70E+06 |
1,32E+01 |
8,86E+13 |
0,0 |
Ni |
f |
6,70E+04 |
5,40E+02 |
3,62E+13 |
0,0 |
Pb |
f |
1,00E+08 |
2,18E+03 |
2,18E+17 |
4,5 |
Se |
f |
1,50E+06 |
8,45E+01 |
1,27E+14 |
0,0 |
Cu |
f |
5,70E+02 |
1,15E+02 |
6,55E+10 |
0,0 |
Zn |
f |
8,10E+04 |
1,66E+03 |
1,35E+14 |
0,0 |
Particulate matter (PM10) |
d |
2,00E+04 |
3,00E+05 |
6,00E+15 |
0,1 |
a | Ritter (1997). |
b | van der Auweraert et al. (1996). |
c | Blonk H et al. (1997). |
d | Berdowski et al. (1997) |
e | Quass & Fermann (1997) |
f | Koch (1998) |
g | Hauschild et al. (1996). |
h | Hauschild et al. (1998). |
i | This project |
C.1.7 Luxembourg, normalisation reference, air
|
|
Effect factor |
Emission |
EDIP revision |
|
|
|
EF (h) |
1994 |
MP |
% |
|
|
|
ton/year |
|
|
Total |
|
|
|
3,68E+16 |
|
Population |
|
|
|
4,00E+05 |
|
Normalisation factor |
|
|
|
9,21E+10 |
|
|
|
|
|
|
|
Substance |
Ref. |
|
|
|
|
SO2 |
a |
1,30E+03 |
1,28E+04 |
1,67E+13 |
0,0 |
NOx |
a |
8,60E+03 |
2,26E+04 |
1,94E+14 |
0,5 |
N2O |
a |
2,00E+03 |
6,89E+02 |
1,38E+12 |
0,0 |
CO |
a |
8,30E+02 |
1,45E+05 |
1,21E+14 |
0,3 |
nmVOC |
a |
1,00E+04 |
8,59E+03 |
8,59E+13 |
0,2 |
nmVOC - transport |
i |
|
9,06E+03 |
3,18E+16 |
86,3 |
Cd |
a |
1,10E+08 |
5,31E-01 |
5,84E+13 |
0,2 |
As |
a |
9,50E+06 |
3,50E+00 |
3,32E+13 |
0,1 |
Cr(VI) |
a |
1,00E+06 |
2,97E+00 |
2,97E+12 |
0,0 |
Hg |
a |
6,70E+06 |
2,45E-01 |
1,64E+12 |
0,0 |
Ni |
a |
6,70E+04 |
2,81E+00 |
1,88E+11 |
0,0 |
Pb |
a |
1,00E+08 |
4,39E+01 |
4,39E+15 |
11,9 |
Se |
a |
1,50E+06 |
7,22E+00 |
1,08E+13 |
0,0 |
Cu |
a |
5,70E+02 |
3,49E+00 |
1,99E+09 |
0,0 |
Zn |
a |
8,10E+04 |
1,51E+02 |
1,23E+13 |
0,0 |
PAH-eq. (benzo(a)pyren) |
estm. |
5,00E+07 |
1,30E-01 |
6,52E+12 |
0,0 |
Dioxin |
a |
2,90E+10 |
2,20E-05 |
6,38E+11 |
0,0 |
Particulate matter (PM10) |
d |
2,00E+04 |
5,90E+03 |
1,18E+14 |
0,3 |
a | Ritter (1997). |
b | van der Auweraert et al. (1996). |
c | Blonk H et al. (1997). |
d | Berdowski et al. (1997) |
e | Quass & Fermann (1997) |
f | Koch (1998) |
g | Hauschild et al. (1996). |
h | Hauschild et al. (1998). |
i | This project |
C.1.8 Netherlands, normalisation reference, air
|
|
Effect factor |
Emission |
EDIP "Dutch emissions" |
EDIP revision |
|
|
|
EF (h) |
1994 |
MP |
% |
|
% |
|
|
|
ton/year |
|
|
|
|
Total |
|
|
|
1,59E+17 |
|
5,94E+17 |
|
Population |
|
|
|
1,53E+07 |
|
1,53E+07 |
|
Normalisation factor |
|
|
|
1,04E+10 |
|
3,88E+10 |
|
|
|
|
|
|
|
|
|
Substance |
Ref. |
|
|
|
|
|
|
SO2 |
a |
1,30E+03 |
1,46E+05 |
1,90E+14 |
0,1 |
1,90E+14 |
0,0 |
NOx |
a |
8,60E+03 |
5,30E+05 |
4,56E+15 |
2,9 |
4,56E+15 |
0,8 |
N2O |
a |
2,00E+03 |
4,59E+04 |
9,17E+13 |
0,1 |
9,17E+13 |
0,0 |
CO |
a |
8,30E+02 |
9,08E+05 |
7,53E+14 |
0,5 |
7,53E+14 |
0,1 |
nmVOC |
|
1,00E+04 |
3,78E+05 |
|
0,0 |
3,78E+15 |
0,6 |
nmVOC - transport |
i |
|
1,37E+05 |
|
|
5,65E+17 |
95,1 |
Cd |
b |
1,10E+08 |
1,80E+00 |
1,98E+14 |
0,1 |
1,98E+14 |
0,0 |
As |
c |
9,50E+06 |
1,54E+00 |
1,46E+13 |
0,0 |
1,46E+13 |
0,0 |
Cr(VI) |
b |
1,00E+06 |
1,08E+01 |
1,08E+13 |
0,0 |
1,08E+13 |
0,0 |
Hg |
f |
6,70E+06 |
1,04E+01 |
6,97E+13 |
0,0 |
6,97E+13 |
0,0 |
Ni |
b |
6,70E+04 |
9,47E+01 |
6,34E+12 |
0,0 |
6,34E+12 |
0,0 |
Pb |
b |
1,00E+08 |
1,60E+02 |
1,60E+16 |
10,1 |
1,60E+16 |
2,7 |
Se |
b |
1,50E+06 |
2,88E-01 |
4,32E+11 |
0,0 |
4,32E+11 |
0,0 |
Cu |
b |
5,70E+02 |
6,04E+01 |
3,44E+10 |
0,0 |
3,44E+10 |
0,0 |
Zn |
b |
8,10E+04 |
2,79E+02 |
2,26E+13 |
0,0 |
2,26E+13 |
0,0 |
Formaldehyde |
b |
1,30E+07 |
4,01E+03 |
5,21E+16 |
32,8 |
|
|
Benzene |
b |
1,00E+07 |
8,16E+03 |
8,16E+16 |
51,3 |
|
|
Phenol |
b |
1,40E+06 |
1,54E+02 |
2,16E+14 |
0,1 |
|
|
Styrene |
b |
1,00E+03 |
1,56E+03 |
1,56E+12 |
0,0 |
|
|
Toluene |
b |
2,50E+03 |
2,45E+04 |
6,13E+13 |
0,0 |
|
|
Xylenes |
b |
6,70E+03 |
1,16E+04 |
7,77E+13 |
0,0 |
|
|
PAH-eq. (benzo(a)pyren) |
|
5,00E+07 |
1,28E+01 |
6,39E+14 |
0,4 |
6,39E+14 |
0,1 |
Dioxin |
b |
2,90E+10 |
1,44E-04 |
4,18E+12 |
0,0 |
4,18E+12 |
0,0 |
PCP |
|
8,30E+03 |
|
0,00E+00 |
0,0 |
0,00E+00 |
0,0 |
Hexachlorobenzene (HCB) |
|
8,30E+03 |
|
0,00E+00 |
0,0 |
0,00E+00 |
0,0 |
Tetrachloromethane (TCM) |
b |
2,90E+04 |
1,51E+02 |
4,38E+12 |
0,0 |
4,38E+12 |
0,0 |
Trichloroethylene (TRI) |
b |
1,90E+04 |
1,04E+03 |
1,98E+13 |
0,0 |
1,98E+13 |
0,0 |
Tetrachloroethylene (PER) |
b |
2,90E+04 |
2,03E+03 |
5,89E+13 |
0,0 |
5,89E+13 |
0,0 |
Trichlorobenzene (TCB) |
|
8,30E+03 |
|
0,00E+00 |
0,0 |
0,00E+00 |
0,0 |
Trichloroethane (TCE) |
b |
9,20E+02 |
1,85E+03 |
1,70E+12 |
0,0 |
1,70E+12 |
0,0 |
Hexachlorocyclohexane (HCH) |
|
8,30E+03 |
|
0,00E+00 |
0,0 |
0,00E+00 |
0,0 |
Chlorobenzenes |
b |
8,30E+03 |
9,25E+01 |
7,68E+11 |
0,0 |
7,68E+11 |
0,0 |
Vinylchloride |
b |
3,90E+05 |
1,10E+02 |
4,29E+13 |
0,0 |
4,29E+13 |
0,0 |
Particulate matter (PM10) |
b |
2,00E+04 |
3,74E+04 |
7,48E+14 |
0,5 |
7,48E+14 |
0,1 |
Benzene (w) |
|
1,00E+07 |
1,65E+08 |
1,65E+15 |
1,0 |
1,65E+15 |
0,3 |
Toluene (w) |
|
2,50E+03 |
4,13E+08 |
1,03E+12 |
0,0 |
1,03E+12 |
0,0 |
Xylenes (w) |
|
6,70E+03 |
1,65E+08 |
1,11E+12 |
0,0 |
1,11E+12 |
0,0 |
Hexachlorocyclohexane (HCH) (w) |
|
8,30E+03 |
6,84E+03 |
5,68E+07 |
0,0 |
5,68E+07 |
0,0 |
Tetrachloroethylene (PER) (w) |
|
2,90E+04 |
8,20E+04 |
2,38E+09 |
0,0 |
2,38E+09 |
0,0 |
Tetrachloromethane (TCM) (w) |
|
2,90E+04 |
3,46E+05 |
1,00E+10 |
0,0 |
1,00E+10 |
0,0 |
Trichloroethane (TCE) (w) |
|
9,20E+02 |
5,85E+04 |
5,38E+07 |
0,0 |
5,38E+07 |
0,0 |
Trichloroethylene (TRI) (w) |
|
1,90E+04 |
1,56E+05 |
2,96E+09 |
0,0 |
2,96E+09 |
0,0 |
Trichloromethane (w) |
|
2,90E+04 |
2,36E+05 |
6,84E+09 |
0,0 |
6,84E+09 |
0,0 |
Chlorobenzenes (w) |
|
8,30E+03 |
1,00E+05 |
8,30E+08 |
0,0 |
8,30E+08 |
0,0 |
Hexachlorobenzene (HCB) (w) |
|
8,30E+03 |
1,41E+04 |
1,17E+08 |
0,0 |
1,17E+08 |
0,0 |
PCP (w) |
|
8,30E+03 |
1,14E+04 |
9,46E+07 |
0,0 |
9,46E+07 |
0,0 |
Trichlororobenzene (TCB) (w) |
|
8,30E+03 |
9,14E+02 |
7,59E+06 |
0,0 |
7,59E+06 |
0,0 |
Chloroform (s) |
|
1,00E+05 |
4,13E+01 |
4,13E+06 |
0,0 |
4,13E+06 |
0,0 |
Dichlorobenzene (s) |
|
8,30E+03 |
5,56E+02 |
4,61E+06 |
0,0 |
4,61E+06 |
0,0 |
Tetrachloroethylene (PER) (s) |
|
2,90E+04 |
8,96E+02 |
2,60E+07 |
0,0 |
2,60E+07 |
0,0 |
Trichloroethylene (TRI) (s) |
|
1,90E+04 |
1,48E+02 |
2,81E+06 |
0,0 |
2,81E+06 |
0,0 |
Toluene (s) |
|
2,50E+03 |
1,05E+03 |
2,63E+06 |
0,0 |
2,63E+06 |
0,0 |
a | Ritter (1997). |
b | van der Auweraert et al. (1996). |
c | Blonk H et al. (1997). |
d | Berdowski et al. (1997) |
e | Quass & Fermann (1997) |
f | Koch (1998) |
g | Hauschild et al. (1996). |
h | Hauschild et al. (1998). |
i | This project |
C.1.10 Sweden, normalisation reference, air
|
|
Effect factor |
Emission |
EDIP revision |
|
|
|
EF (h) |
1994 |
MP |
% |
|
|
|
ton/year |
|
|
Total |
|
|
|
5,39E+17 |
|
Population |
|
|
|
8,75E+06 |
|
Normalisation factor |
|
|
|
6,16E+10 |
|
|
|
|
|
|
|
Substance |
Ref. |
|
|
|
|
SO2 |
|
1,30E+03 |
7,42E+04 |
9,64E+13 |
0,0 |
NOx |
|
8,60E+03 |
4,44E+05 |
3,82E+15 |
0,7 |
N2O |
|
2,00E+03 |
2,36E+04 |
4,72E+13 |
0,0 |
CO |
|
8,30E+02 |
1,32E+06 |
1,09E+15 |
0,2 |
nmVOC |
|
1,00E+04 |
2,36E+05 |
2,36E+15 |
0,4 |
nmVOC - transport |
i |
|
1,45E+05 |
5,29E+17 |
98,2 |
Cd |
f |
1,10E+08 |
2,29E-01 |
2,52E+13 |
0,0 |
As |
f |
9,50E+06 |
6,46E-01 |
6,14E+12 |
0,0 |
Cr(VI) |
f |
1,00E+06 |
7,42E+00 |
7,42E+12 |
0,0 |
Hg |
f |
6,70E+06 |
5,65E-01 |
3,79E+12 |
0,0 |
Ni |
f |
6,70E+04 |
9,33E+00 |
6,25E+11 |
0,0 |
Pb |
f |
1,00E+08 |
1,56E+01 |
1,56E+15 |
0,3 |
Se |
f |
1,50E+06 |
1,60E-01 |
2,40E+11 |
0,0 |
Cu |
f |
5,70E+02 |
6,98E+00 |
3,98E+09 |
0,0 |
Zn |
f |
8,10E+04 |
3,00E+01 |
2,43E+12 |
0,0 |
Dioxin |
e |
2,90E+10 |
3,60E-05 |
1,04E+12 |
0,0 |
Particulate matter (PM10) |
d |
2,00E+04 |
4,10E+04 |
8,20E+14 |
0,2 |
a | Ritter (1997). |
b | van der Auweraert et al. (1996). |
c | Blonk H et al. (1997). |
d | Berdowski et al. (1997) |
e | Quass & Fermann (1997) |
f | Koch (1998) |
g | Hauschild et al. (1996). |
h | Hauschild et al. (1998). |
i | This project |
C.1.12 United Kingdom, normalisation reference, air
|
|
Effect factor |
Emission |
EDIP revision |
|
|
|
EF (h) |
1994 |
MP |
% |
|
|
|
ton/year |
|
|
Total |
|
|
|
3,69E+18 |
|
Population |
|
|
|
5,82E+07 |
|
Normalisation factor |
|
|
|
6,34E+10 |
|
|
|
|
|
|
|
Substance |
Ref. |
|
|
|
|
SO2 |
a |
1,30E+03 |
2,70E+06 |
3,51E+15 |
0,1 |
NOx |
a |
8,60E+03 |
2,39E+06 |
2,05E+16 |
0,6 |
N2O |
a |
2,00E+03 |
9,95E+04 |
1,99E+14 |
0,0 |
CO |
a |
8,30E+02 |
5,97E+06 |
4,95E+15 |
0,1 |
nmVOC |
a |
1,00E+04 |
1,59E+06 |
1,59E+16 |
0,4 |
nmVOC - transport |
i |
|
7,61E+05 |
3,45E+18 |
93,6 |
Cd |
a |
1,10E+08 |
2,35E+01 |
2,59E+15 |
0,1 |
As |
a |
9,50E+06 |
1,12E+02 |
1,06E+15 |
0,0 |
Cr(VI) |
a |
1,00E+06 |
6,33E+01 |
6,33E+13 |
0,0 |
Hg |
a |
6,70E+06 |
1,95E+01 |
1,31E+14 |
0,0 |
Ni |
a |
6,70E+04 |
4,67E+02 |
3,13E+13 |
0,0 |
Pb |
a |
1,00E+08 |
1,75E+03 |
1,75E+17 |
4,8 |
Se |
a |
1,50E+06 |
9,93E+01 |
1,49E+14 |
0,0 |
Cu |
a |
5,70E+02 |
7,92E+01 |
4,52E+10 |
0,0 |
Zn |
a |
8,10E+04 |
1,31E+03 |
1,06E+14 |
0,0 |
PAH-eq. (benzo(a)pyren) |
estm. |
5,00E+07 |
9,05E+01 |
4,53E+15 |
0,1 |
Dioxin |
a |
2,90E+10 |
7,93E-04 |
2,30E+13 |
0,0 |
PCP |
a |
8,30E+03 |
5,55E+02 |
4,61E+12 |
0,0 |
Hexachlorobenzene (HCB) |
a |
8,30E+03 |
1,20E+00 |
9,96E+09 |
0,0 |
Tetrachloromethane (TCM) |
a |
2,90E+04 |
3,19E+03 |
9,25E+13 |
0,0 |
Trichloroethylene (TRI) |
a |
1,90E+04 |
2,03E+04 |
3,87E+14 |
0,0 |
Tetrachloroethylene (PER) |
a |
2,90E+04 |
1,13E+04 |
3,27E+14 |
0,0 |
Trichlorobenzene (TCB) |
a |
8,30E+03 |
6,29E+02 |
5,22E+12 |
0,0 |
Trichloroethane (TCE) |
a |
9,20E+02 |
2,47E+04 |
2,28E+13 |
0,0 |
Hexachlorocyclohexane (HCH) |
a |
8,30E+03 |
1,14E+02 |
9,46E+11 |
0,0 |
Particulate matter (PM10) |
d |
2,00E+04 |
2,70E+05 |
5,40E+15 |
0,1 |
a | Ritter (1997). |
b | van der Auweraert et al. (1996). |
c | Blonk H et al. (1997). |
d | Berdowski et al. (1997) |
e | Quass & Fermann (1997) |
f | Koch (1998) |
h | Hauschild et al. (1998). |
i | This project |
C.2.1 Denmark, normalisation reference, water
|
|
EF(htw) |
Emission (1) |
EP(htw) |
Distr. |
|
|
m3/g |
g/yr |
m3/yr |
% |
Total |
|
|
|
9,29E+11 |
|
Population |
|
|
|
5,20E+06 |
|
Normalisation factor |
|
|
|
1,79E+05 |
|
Water emissions |
Substance |
|
|
|
|
Heavy metals |
Hg |
1,10E+05 |
7,26E+05 |
7,98E+10 |
8,5959 |
|
Cd |
2,80E+03 |
9,03E+05 |
2,53E+09 |
0,2722 |
|
Cu |
1,70E+01 |
4,06E+07 |
6,90E+08 |
0,0743 |
|
Zn |
2,10E+01 |
1,23E+08 |
2,58E+09 |
0,2782 |
|
Pb |
2,60E+02 |
7,43E+06 |
1,93E+09 |
0,2081 |
Aliphatic compounds |
|
|
2,47E+09 |
|
|
Aromatics |
|
|
5,38E+08 |
|
|
|
Benzene (1) |
2,30E+00 |
7,33E+07 |
1,69E+08 |
0,0182 |
|
Benz(a)pyrene |
|
1,48E+06 |
|
|
|
Ethylbenzene (1) |
|
1,72E+00 |
|
|
|
Fluoranthene |
|
7,02E+06 |
|
|
|
Phthalates |
|
5,02E+04 |
|
|
|
Isopropylbenzene |
2,10E-01 |
1,77E+05 |
3,72E+04 |
0,0000 |
|
PAH (6 comp.) (2) |
|
8,22E+06 |
|
|
|
PAH-eq. (Benzo(a)pyren) |
3,20E+02 |
9,74E+05 |
3,12E+08 |
0,0336 |
|
Toluene |
4,00E-03 |
1,84E+08 |
7,34E+05 |
0,0001 |
|
Xylenes |
1,10E-03 |
7,33E+07 |
8,06E+04 |
0,0000 |
Halogenated org. Compounds |
|
1,32E+07 |
|
|
Aliphatics |
|
|
1,27E+07 |
|
|
|
1,2-Dichloroethane |
2,00E-02 |
1,85E+05 |
3,71E+03 |
0,0000 |
|
Hexachlorbutadiene (1) |
|
0,00E+00 |
|
|
|
Hexachlorcyclohexane (1) |
|
3,04E+03 |
|
|
|
Tetrachlorethylene |
3,60E-01 |
3,64E+04 |
1,31E+04 |
0,0000 |
|
Tetrachlormethane |
3,60E-01 |
1,54E+05 |
5,53E+04 |
0,0000 |
|
1,1,1-Trichloroethane (1) |
9,90E-04 |
2,60E+04 |
2,57E+01 |
0,0000 |
|
Trichloroethene (1) |
9,10E-04 |
6,93E+04 |
6,31E+01 |
0,0000 |
|
Trichloromethane |
9,10E-04 |
1,05E+05 |
9,54E+01 |
0,0000 |
|
Vinylchloride |
4,00E-01 |
8,49E+02 |
3,39E+02 |
0,0000 |
Aromatics |
|
|
5,51E+05 |
|
|
|
Chlorobenzene (1) |
2,70E-01 |
4,44E+04 |
1,20E+04 |
0,0000 |
|
DRINS |
|
1,33E+03 |
|
|
|
Hexachlorobenzene (1) |
3,70E-01 |
6,26E+03 |
2,32E+03 |
0,0000 |
|
PCB (1) |
|
3,65E+02 |
|
|
|
Pentachlorophenol (1) |
3,70E-01 |
5,07E+03 |
1,87E+03 |
0,0000 |
|
Thichlorobenzene (1) |
3,70E-01 |
4,06E+02 |
1,50E+02 |
0,0000 |
Air emissions |
Cd |
5,60E+02 |
1,19E+06 |
6,64E+08 |
0,0715 |
|
As |
7,40E+00 |
7,42E+05 |
5,49E+06 |
0,0006 |
|
Cr(VI) |
3,60E+00 |
3,49E+06 |
1,25E+07 |
0,0014 |
|
Hg |
1,10E+05 |
7,58E+06 |
8,34E+11 |
89,8324 |
|
Ni |
3,70E-03 |
2,21E+07 |
8,16E+04 |
0,0000 |
|
Pb |
5,30E+01 |
3,95E+07 |
2,10E+09 |
0,2256 |
|
Se |
2,80E+01 |
1,32E+05 |
3,70E+06 |
0,0004 |
|
Cu |
3,40E+00 |
1,06E+07 |
3,59E+07 |
0,0039 |
|
Zn |
4,10E+00 |
1,18E+08 |
4,85E+08 |
0,0522 |
|
Dioxin |
2,20E+08 |
1,40E+01 |
3,08E+09 |
0,3317 |
C.2.2 EU-15, normalisation reference, water
|
|
EF(htw) |
Emission (1) |
EP(htw) |
Distr. |
|
Substance |
m3/g |
g/yr |
m3/yr |
% |
Total |
|
|
|
1,93E+13 |
|
Population |
|
|
|
3,70E+08 |
|
Normalisation factor |
|
|
|
5,21E+04 |
|
Water emissions |
|
|
|
|
|
Heavy metals |
Hg |
1,10E+05 |
1,69E+07 |
1,86E+12 |
9,6612 |
|
Cd |
2,80E+03 |
4,40E+07 |
1,23E+11 |
0,6396 |
|
Cu |
1,70E+01 |
1,86E+09 |
3,15E+10 |
0,1636 |
|
Zn |
2,10E+01 |
9,60E+09 |
2,02E+11 |
1,0459 |
|
Pb |
2,60E+02 |
1,54E+09 |
4,00E+11 |
2,0750 |
Aliphatic compounds |
|
|
1,24E+11 |
|
|
Aromatics |
|
|
2,71E+10 |
|
|
|
Benzene (1) |
2,30E+00 |
3,69E+09 |
8,49E+09 |
0,0441 |
|
Benz(a)pyrene |
|
7,47E+07 |
|
|
|
Ethylbenzene (1) |
|
8,66E+01 |
|
|
|
Fluoranthene |
|
3,54E+08 |
|
|
|
Phthalates |
|
2,53E+06 |
|
|
|
Isopropylbenzene |
2,10E-01 |
8,93E+06 |
1,87E+06 |
0,0000 |
|
PAH (6 comp.) (2) |
|
4,14E+08 |
|
|
|
PAH-eq. (Benzo(a)pyren) |
3,20E+02 |
4,90E+07 |
1,57E+10 |
0,0814 |
|
Toluene |
4,00E-03 |
9,24E+09 |
3,70E+07 |
0,0002 |
|
Xylenes |
1,10E-03 |
3,69E+09 |
4,06E+06 |
0,0000 |
Halogenated org. Compounds |
|
6,67E+08 |
|
|
Aliphatics |
|
|
6,38E+08 |
|
|
|
1,2-Dichloroethane |
2,00E-02 |
9,33E+06 |
1,87E+05 |
0,0000 |
|
Hexachlorbutadiene (1) |
|
0,00E+00 |
|
|
|
Hexachlorcyclohexane (1) |
|
1,53E+05 |
|
|
|
Tetrachlorethylene |
3,60E-01 |
1,83E+06 |
6,61E+05 |
0,0000 |
|
Tetrachlormethane |
3,60E-01 |
7,74E+06 |
2,79E+06 |
0,0000 |
|
1,1,1-Trichloroethane (1) |
9,90E-04 |
1,31E+06 |
1,30E+03 |
0,0000 |
|
Trichloroethene (1) |
9,10E-04 |
3,49E+06 |
3,18E+03 |
0,0000 |
|
Trichloromethane |
9,10E-04 |
5,28E+06 |
4,81E+03 |
0,0000 |
|
Vinylchloride |
4,00E-01 |
4,27E+04 |
1,71E+04 |
0,0000 |
Aromatics |
|
|
2,77E+07 |
|
|
|
Chlorobenzene (1) |
2,70E-01 |
2,24E+06 |
6,04E+05 |
0,0000 |
|
DRINS |
|
6,71E+04 |
|
|
|
Hexachlorobenzene (1) |
3,70E-01 |
3,16E+05 |
1,17E+05 |
0,0000 |
|
PCB (1) |
|
1,84E+04 |
|
|
|
Pentachlorophenol (1) |
3,70E-01 |
2,55E+05 |
9,44E+04 |
0,0000 |
|
Thichlorobenzene (1) |
3,70E-01 |
2,05E+04 |
7,57E+03 |
0,0000 |
Air emissions |
Cd |
5,60E+02 |
1,04E+08 |
5,83E+10 |
0,3023 |
|
As |
7,40E+00 |
2,82E+08 |
2,09E+09 |
0,0108 |
|
Cr(VI) |
3,60E+00 |
5,66E+08 |
2,04E+09 |
0,0106 |
|
Hg |
1,10E+05 |
1,40E+08 |
1,54E+13 |
79,6572 |
|
Ni |
3,70E-03 |
2,09E+09 |
7,75E+06 |
0,0000 |
|
Pb |
5,30E+01 |
7,07E+09 |
3,75E+11 |
1,9441 |
|
Se |
2,80E+01 |
3,19E+08 |
8,95E+09 |
0,0464 |
|
Cu |
3,40E+00 |
5,60E+08 |
1,90E+09 |
0,0099 |
|
Zn |
4,10E+00 |
6,86E+09 |
2,81E+10 |
0,1459 |
|
Dioxin |
2,20E+08 |
3,65E+03 |
8,02E+11 |
4,1617 |
C.2.3 Netherlands, normalisation reference, water
|
|
EF(htw) |
Emission (1) |
EP(htw) |
Distr. |
|
Substance |
m3/g |
g/yr |
m3/yr |
% |
Total |
|
|
|
1,31E+12 |
|
Population |
|
|
|
1,53E+07 |
|
Normalisation factor |
|
|
|
8,44E+04 |
|
Water emissions |
|
|
|
|
|
Heavy metals |
Hg |
1,10E+05 |
6,46E+05 |
7,11E+10 |
5,4310 |
|
Cd |
2,80E+03 |
1,84E+06 |
5,15E+09 |
0,3938 |
|
Cu |
1,70E+01 |
8,22E+07 |
1,40E+09 |
0,1068 |
|
Zn |
2,10E+01 |
4,95E+08 |
1,04E+10 |
0,7945 |
|
Pb |
2,60E+02 |
1,26E+08 |
3,28E+10 |
2,5038 |
Aliphatic compounds |
|
|
5,55E+09 |
|
|
Aromatics |
|
|
1,21E+09 |
|
|
|
Benzene (1) |
2,30E+00 |
1,65E+08 |
3,80E+08 |
0,0290 |
|
Benz(a)pyrene |
|
3,34E+06 |
|
|
|
Ethylbenzene (1) |
|
3,87E+00 |
|
|
|
Fluoranthene |
|
1,58E+07 |
|
|
|
Phthalates |
|
1,13E+05 |
|
|
|
Isopropylbenzene |
2,10E-01 |
3,99E+05 |
8,38E+04 |
0,0000 |
|
PAH (6 comp.) (2) |
|
1,85E+07 |
|
|
|
PAH-eq. (Benzo(a)pyren) |
3,20E+02 |
2,19E+06 |
7,01E+08 |
0,0536 |
|
Toluene |
4,00E-03 |
4,13E+08 |
1,65E+06 |
0,0001 |
|
Xylenes |
1,10E-03 |
1,65E+08 |
1,82E+05 |
0,0000 |
Halogenated org. Compounds |
|
2,98E+07 |
|
|
Aliphatics |
|
|
2,85E+07 |
|
|
|
1,2-Dichloroethane |
2,00E-02 |
4,17E+05 |
8,34E+03 |
0,0000 |
|
Hexachlorbutadiene (1) |
|
0,00E+00 |
|
|
|
Hexachlorcyclohexane (1) |
|
6,84E+03 |
|
|
|
Tetrachlorethylene |
3,60E-01 |
8,20E+04 |
2,95E+04 |
0,0000 |
|
Tetrachlormethane |
3,60E-01 |
3,46E+05 |
1,25E+05 |
0,0000 |
|
1,1,1-Trichloroethane (1) |
9,90E-04 |
5,85E+04 |
5,79E+01 |
0,0000 |
|
Trichloroethene (1) |
9,10E-04 |
1,56E+05 |
1,42E+02 |
0,0000 |
|
Trichloromethane |
9,10E-04 |
2,36E+05 |
2,15E+02 |
0,0000 |
|
Vinylchloride |
4,00E-01 |
1,91E+03 |
7,64E+02 |
0,0000 |
Aromatics |
|
|
1,24E+06 |
|
0,0000 |
|
Chlorobenzene (1) |
2,70E-01 |
1,00E+05 |
2,70E+04 |
0,0000 |
|
DRINS |
|
3,00E+03 |
|
0,0000 |
|
Hexachlorobenzene (1) |
3,70E-01 |
1,41E+04 |
5,22E+03 |
0,0000 |
|
PCB (1) |
|
8,22E+02 |
|
0,0000 |
|
Pentachlorophenol (1) |
3,70E-01 |
1,14E+04 |
4,22E+03 |
0,0000 |
|
Thichlorobenzene (1) |
3,70E-01 |
9,14E+02 |
3,38E+02 |
0,0000 |
Air emissions |
Cd |
5,60E+02 |
1,80E+06 |
1,01E+09 |
0,0770 |
|
As |
7,40E+00 |
1,54E+06 |
1,14E+07 |
0,0009 |
|
Cr(VI) |
3,60E+00 |
1,08E+07 |
3,89E+07 |
0,0030 |
|
Hg |
1,10E+05 |
1,04E+07 |
1,14E+12 |
87,4335 |
|
Ni |
3,70E-03 |
9,47E+07 |
3,50E+05 |
0,0000 |
|
Pb |
5,30E+01 |
1,60E+08 |
8,48E+09 |
0,6481 |
|
Se |
2,80E+01 |
2,88E+05 |
8,06E+06 |
0,0006 |
|
Cu |
3,40E+00 |
6,04E+07 |
2,05E+08 |
0,0157 |
|
Zn |
4,10E+00 |
2,79E+08 |
1,14E+09 |
0,0874 |
|
Dioxin |
2,20E+08 |
1,44E+02 |
3,17E+10 |
2,4212 |
C.3.1 Denmark, normalisation reference, soil
Click here to see the Table.
C.3.2 EU-15, normalisation reference, soil
Click here to see the Table.
C.3.3 Netherlands, normalisation reference, soil
Click here to see the Table.
Appendix D: Distribution of impact potentials
The appendix is split up into three separate sections, presenting the distribution of the following impact potentials:
Appendix D1: Normalisation references for human toxicity via air for EU-15 and the individual Member States
Appendix D2: Normalisation references for human toxicity via water for Denmark, the Netherlands and EU-15
Appendix D3: Normalisation references for human toxicity via soil for Denmark, the Netherlands and EU-15
D.1 Normalisation references, air; distribution of impact potentials
Click here to see the Table.
D.2 Normalisation references, water; distribution of impact potentials
|
|
EU-15 |
DK |
NL |
Total |
|
1,93E+13 |
9,29E+11 |
1,31E+12 |
Population |
|
3,70E+08 |
5,20E+06 |
1,53E+07 |
Normalisation factor |
|
5,21E+04 |
1,79E+05 |
8,55E+04 |
Water emissions |
Substance |
% |
% |
% |
Heavy metals |
Hg |
9,66 |
8,60 |
5,43 |
|
Cd |
0,64 |
0,27 |
0,39 |
|
Cu |
0,16 |
0,07 |
0,11 |
|
Zn |
1,05 |
0,28 |
0,79 |
|
Pb |
2,07 |
0,21 |
2,50 |
Aliphatic compounds |
|
|
|
|
Aromatics |
|
|
|
|
|
Benzene (1) |
0,04 |
0,02 |
0,03 |
|
Benz(a)pyrene |
|
|
|
|
Ethylbenzene (1) |
|
|
|
|
Fluoranthene |
|
|
|
|
Phthalates |
|
|
|
|
Isopropylbenzene |
|
|
|
|
PAH (6 comp.) (2) |
|
|
|
|
PAH-eq. (Benzo(a)pyren) |
0,08 |
0,03 |
0,05 |
|
Toluene |
0,00 |
0,00 |
0,00 |
|
Xylenes |
0,00 |
0,00 |
0,00 |
Halogenated org. Compounds |
|
|
|
Aliphatics |
|
|
|
|
|
1,2-Dichloroethane |
0,00 |
0,00 |
0,00 |
|
Hexachlorbutadiene (1) |
|
|
|
|
Hexachlorcyclohexane (1) |
|
|
|
|
Tetrachlorethylene |
0,00 |
0,00 |
0,00 |
|
Tetrachlormethane |
0,00 |
0,00 |
0,00 |
|
1,1,1-Trichloroethane (1) |
0,00 |
0,00 |
0,00 |
|
Trichloroethene (1) |
0,00 |
0,00 |
0,00 |
|
Trichloromethane |
0,00 |
0,00 |
0,00 |
|
Vinylchloride |
0,00 |
0,00 |
0,00 |
Aromatics |
|
|
|
|
|
Chlorobenzene (1) |
0,00 |
0,00 |
0,00 |
|
DRINS |
|
|
|
|
Hexachlorobenzene (1) |
0,00 |
0,00 |
0,00 |
|
PCB (1) |
|
|
|
|
Pentachlorophenol (1) |
0,00 |
0,00 |
0,00 |
|
Thichlorobenzene (1) |
0,00 |
0,00 |
0,00 |
Air emissions |
Cd |
0,30 |
0,07 |
0,08 |
|
As |
0,01 |
0,00 |
0,00 |
|
Cr(VI) |
0,01 |
0,00 |
0,00 |
|
Hg |
79,66 |
89,83 |
87,43 |
|
Ni |
0,00 |
0,00 |
0,00 |
|
Pb |
1,94 |
0,23 |
0,65 |
|
Se |
0,05 |
0,00 |
0,00 |
|
Cu |
0,01 |
0,00 |
0,02 |
|
Zn |
0,15 |
0,05 |
0,09 |
|
Dioxin |
4,16 |
0,33 |
2,42 |
D.3 Normalisation references, soil; distribution of impact potentials
|
|
EU-15 |
DK |
NL |
Total |
|
4,44E+10 |
8,04E+08 |
1,09E+09 |
Population |
|
3,70E+08 |
5,20E+06 |
1,53E+07 |
Normalisation factor |
|
1,20E+02 |
1,55E+02 |
7,10E+01 |
|
Substance |
% |
% |
% |
Sludge |
Acenaphthene |
|
|
|
|
Fluorene |
|
|
|
|
Phenanthrene |
|
|
|
|
Fluoranthene |
|
|
|
|
Pyrene |
|
|
|
|
Benzofluoranthene |
|
|
|
|
Benzo(a)pyrene |
|
|
|
|
Benzo(ghi)perylene |
|
|
|
|
Indeno(1,2,3-cd)pyrene |
|
|
|
|
PAH-eq. (Benzo(a)pyren) |
0,00 |
0,00 |
0,00 |
|
PCB 28 |
|
|
|
|
PCB 52 |
|
|
|
|
PCB 101 |
|
|
|
|
PCB 118 |
|
|
|
|
PCB 138 |
|
|
|
|
PCB 153 |
|
|
|
|
PCB 180 |
|
|
|
|
Sum PCB |
|
|
|
|
Butylbenzylphthalate |
|
|
|
|
Chloroform |
0,00 |
0,00 |
0,00 |
|
Di(ethylhexyl)phthalate |
|
|
|
|
Dichlorobenezene |
0,00 |
0,00 |
0,00 |
|
Di-n-butylphthalate |
|
|
|
|
Di-n-octylphthalate |
|
|
|
|
Nonylphenol + 1-2EO |
|
|
|
|
Tetrachloroethylene |
0,00 |
0,00 |
0,00 |
|
Trichlorothylene |
0,00 |
0,00 |
0,00 |
|
LAS (2) |
|
|
|
|
Toluene |
0,00 |
0,00 |
0,00 |
|
Dioxine (2,3,7,8-TCDD) |
0,00 |
0,00 |
0,00 |
|
As |
3,98 |
7,76 |
2,26 |
|
Cd |
0,08 |
0,15 |
0,04 |
|
Cr |
0,41 |
0,80 |
0,23 |
|
Cu |
0,01 |
0,02 |
0,01 |
|
Pb |
0,06 |
0,12 |
0,03 |
|
Hg |
1,60 |
3,13 |
0,91 |
|
Ni |
0,04 |
0,07 |
0,02 |
|
Zn |
0,12 |
0,23 |
0,07 |
Pesticides |
Fungicides |
|
|
|
|
Herbicides |
|
|
|
|
Insecticides |
|
|
|
Air emissions |
Cd |
1,05 |
0,66 |
0,75 |
|
As |
63,54 |
9,23 |
14,18 |
|
Cr(VI) |
1,40 |
0,48 |
1,09 |
|
Hg |
25,46 |
76,40 |
77,58 |
|
Ni |
0,57 |
0,33 |
1,05 |
|
Pb |
1,32 |
0,41 |
1,22 |
|
Se |
0,03 |
0,00 |
0,00 |
|
Cu |
0,01 |
0,01 |
0,02 |
|
Zn |
0,20 |
0,19 |
0,33 |
|
Dioxin |
0,11 |
0,02 |
0,19 |
10 Ecotoxicity
Jens Tørsløv, DHI - Water & Environment
10.1 Summary
This chapter summarises the presently available data on environmental releases of toxic organic and inorganic substances to the Danish and European environment. The estimated releases include:
Aquatic environment:
- Releases of organic pollutants with wastewater
- Releases of metals
- Emissions of oil from oil extraction and refineries
- Release of organotin compounds from antifouling paints
- Atmospheric deposition
Terrestrial environment:
- Pesticide use
- Agricultural use of sewage sludge
- Atmospheric deposition of metals and dioxins
The ecotoxicological impact is estimated from the released amounts of the individual chemicals and expressed as the volume of water and soil in 1994 (reference year) that was theoretically polluted to a level
which corresponds to the no-effect concentration of the substances, assuming an even distribution of the pollution.
The main results are presented in Table 10-1.
Table 10-1
Summary of normalisation references for ecotoxicity for Denmark, EU-15 and Worldwide in 1994.
|
|
Denmark |
EU-15 |
World |
ETWC |
m3 water/capita/year |
7.91*105 |
3.52*105 |
2.82*105 |
ETWA |
m3 water/capita/year |
7.40*104 |
2.91*104 |
2.33*104 |
ETSC |
m3 soil/capita/year |
6.56*105 |
9.64*105 |
7.71*105 |
ETWC: Ecotoxicity Potential for chronic effects on aquatic organisms.
ETWA: Ecotoxicity Potential for acute effects on aquatic organisms.
ETSC: Ecotoxicity Potential for chronic effects on soil organisms.
The dominating release of toxicants originates from the use of pesticides in agriculture and the use of antifouling paints, containing tributyltin compounds, on ships. The uncertainties of these releases are high
and probably over one order of magnitude. Consequently, the results presented above should be regarded as rough estimates with a high degree of uncertainty. In order to obtain more precise results it is
recommended to improve the estimates of the emission from these sources.
10.2 Description of the impact category
The purpose has been to prepare a normalisation reference for ecotoxicological impacts based on data collected from European national environmental agencies as well as international data centres.
The work is based on 1994 data and covers Denmark and the European Union. In addition a rough estimate of the ecotoxicity potential on a worldwide basis is presented.
The impact category ecotoxicity covers the possible effects of toxic substances released during the life cycle of a product to the environment. The sources of toxicants are quite different depending on the
type of environment as well as the methods used in the assessment of the impact. Consequently, the impact on aquatic and terrestrial systems are usually considered separately.
In principle, the normalisation reference for ecotoxicology includes all toxic substances emitted to the environment due to human activities, and it requires extensive data on all types of emissions. In general,
however, only few data on environmental releases of toxic substances are available, and the normalisation therefore relies on extrapolations from a relatively limited set of data.
The normalisation reference includes the following emission types:
Aquatic environment:
- Releases of organic pollutants with wastewater
- Releases of metals
- Emissions of oil from oil extraction and refineries
- Release of organotin compounds from antifouling paints
- Atmospheric deposition
Terrestrial environment:
- Pesticide use
- Agricultural use of sewage sludge
- Atmospheric deposition of metals and dioxins
10.3 Methodology
The presented estimates of the ecotoxicological impacts are based on estimated releases of toxicants to the aquatic and terrestrial environments on the basis of statistics on emitted volumes of wastewater,
sludges, pesticides, etc. as well as concentrations measured in different environmental media. The data sources and the methods used for estimation of the releases from different types of sources are
discussed together with an evaluation of the uncertainties involved in the sections below.
In order to obtain a quantitative and comparable value for the ecotoxicity potential (EP), the released amounts of individual substances are multiplied by a substance-specific factor, the equivalency factor
(EQF), which corresponds to the potential environmental impact of the substance according to the principles described in Hauschild et al. (1998). The EQF for the single substances are derived from the
predicted no-effect concentration (PNEC) based on the toxicity to aquatic or soil organisms, the biodegradability, the bioaccumulation potential, and the calculated environmental distribution. The EP is
calculated for the individual emission types evaluated and for the following effect types:
- Acute aquatic toxicity
- Chronic aquatic toxicity
- Chronic terrestrial toxicity
In a few cases it was necessary to calculate new EQF values as not earlier included substances were found in emission surveys, i.e. if no EQF was available or if a revision of the existing EQF was required.
In general, however, it was not possible, within the frame of the project, to revise or generate equivalency factors.
The toxicity to the microflora in wastewater treatment plants (WWTP) was included in the former Danish normalisation reference but it is excluded here for the following reasons:
- It is not possible to estimate the potential impact on WWTP because of the lack of reliable data. Estimations of the effects on wastewater treatment plants require data for toxicity to microbiological
treatment processes such as nitrification, respiration or similar relevant parameters. Such data are available only for a few organic and inorganic substances.
- Effects on wastewater treatment plants have an environmental relevance but are an indirect measure for the environmental impact from treatment plants. There is no simple relationship between an
impaired wastewater treatment and the environmental impact of the emitted wastewater.
- The impact on a wastewater treatment facility depends on the toxicity of the emitted wastewater as well as the sensitivity of the facility. A modern treatment plant with N and P removal is normally
regarded as more sensitive to toxic substances than more traditional treatment techniques. Consequently, the impact strongly depends on the wastewater technology used in different countries.
The results presented here are based on a high number of calculations and assumptions. Background data for the individual emission types and data for the different geographical regions are presented in
Appendices A-E.
10.4 Normalisation reference – Aquatic environment
The normalisation reference for the aquatic environment includes those contained in the previous normalisation reference (Hauschild et al. 1998), i.e. wastewater, emissions from oil extraction activities, and
atmospheric depositions. In addition the release of toxicants from the use of tributyltin-based antifouling paints is included. The primary data references are shown in Table 10-2.
Table 10-2
Emission types and selected references used for the estimation of emissions to the aquatic environment.
Emission type |
Data source |
Municipal wastewater |
Jepsen and Grüttner (1997)
DEPA (1995)
van der Auweraert et al. (1996)
EUROSTAT (1998)
|
Industrial wastewater |
van der Auweraert et al. (1996) |
Oil extraction
Oil emission from
refineries
|
EUROSTAT (1998)
ITOPF (1999)
|
Antifouling |
Madsen et al. (1998) |
Atmospheric deposition |
Data from chapter 9 on human toxicity |
Other data sources |
Grüttner and Neergaard Jacobsen (1994), Grüttner et al.
(1996), Lassen et al. (1996a), DEPA (1996), Lassen and Hansen (1996),
Lassen et al. (1996b), Jensen and Markussen (1993)
van der Auweraert et al. (1996)
EEA (1998)
|
Because of the methodological differences and differences in the used data sources, heavy metals, organic substances, oil emissions, antifouling, and air deposition are separately discussed in the following
sections.
10.4.1 Emission of heavy metals
10.4.1.1 Data sources
For 10 European countries the emission of heavy metals to the aquatic environment has been estimated in a recent report from the EUROSTAT (EUROSTAT, 1998). These estimates are based on data
from the European Topic Centre on Marine Environment (ENEA), the Helsinki Commission (HELCOM), the Oslo and Paris Commission (OSPAR), the Barcelona Commission, and the Danube
Commission. The emissions are based on data on riverine and direct input of heavy metals to the marine environment and include the marine and coastal areas from Gibraltar to the Gulf of Bothnia.
The OSPAR data used by EUROSTAT (1998) include high as well as low estimates of the emitted amounts of toxic heavy metals. The high estimates were based on measured concentrations, and in cases
where the substances were not detectable the detection limits were used. The low estimates were based on zero values if the substances were not detected. At emissions less than 1 tonne/year, a value of
zero is used in the present report. The data from OSPAR cover the period of 1990 – 1995, and the data from HELCOM refer to 1995.
As to the countries not included in EUROSTAT (1998), the emissions were estimated by linear extrapolation from emission data from the Netherlands (NL), reported by van der Auweraert et al. (1996) by
use of the Gross Domestic Product (GDP).
The Danish emissions of metals were estimated from measured concentrations in discharged wastewater from municipal treatment plants and estimated releases from other sources based on mass
balance studies of the individual metals (Grüttner & Neergaard Jakobsen 1994; Grüttner et al. 1996; DEPA 1995; Lassen et al. 1996a; DEPA 1996; Lassen & Hansen 1996; Lassen et al. 1996b;
Jensen & Markussen 1993).
Lead from loss of fishing equipment and cable sheets is a significant source (Danish release: 150-575 tonnes/yr., Lassen and Hansen (1996)) but is not included in the present report because metallic lead is
available to the biota only to a very limited extent.
Only emissions of mercury (Hg), cadmium (Cd), copper (Cu), zinc (Zn), and lead (Pb) are included in this report.
10.4.1.2 Results
The ecotoxicity potential (expressed as m3/yr.) for the metals are calculated according to Hauschild et al. (1998) by multiplying the total emission of the individual metals with an equivalency factor (EF)
(Table 10-3).
Table 10-3
Estimates of the ecotoxicity potential for metals emitted to the aquatic environment. The estimates are based on the emission reported by EUROSTAT (1998).
Metal emission |
Denmark |
EU-15 |
|
EU-15 |
DK |
EF |
EP |
EP |
|
|
|
m3/g |
m3/g |
m3/yr. |
m3/yr. |
m3/yr. |
m3/yr. |
|
t/year |
t/year |
etwc1 |
etwa2 |
etwc |
etwa |
etwc |
etwa |
Hg |
13 |
0.73 |
4.00*103 |
2.00*103 |
2.90*109 |
1.45*109 |
5.17*1010 |
2.59*1010 |
Cd |
40 |
0.90 |
1.20*105 |
1.20*104 |
1.08*1011 |
1.08*1010 |
4.80*1012 |
4.80*1011 |
Cu |
1855 |
41 |
1.30*104 |
1.30*103 |
5.28*1011 |
5.28*1010 |
2.41*1013 |
2.41*1012 |
Zn |
9600 |
123 |
1.00*103 |
100 |
1.23*1011 |
1.23*1010 |
9.60*1012 |
9.60*1011 |
Pb |
1538 |
7.4 |
2.00E*103 |
200 |
1.49*1010 |
1.49*109 |
3.08E*1012 |
3.08*1011 |
Sum |
|
|
|
|
7.77*1011 |
7.88*1010 |
4.16*1013 |
4.19*1012 |
etwc: ecotoxicity potential for chronic toxicity to aquatic biota
etwa: ecotoxicity potential for acute toxicity to aquatic biota
The background data for these estimates, including emission data for the individual European countries, are included in Appendix A.
10.4.1.3 Discussion
The precision of the EUROSTAT data is validated by comparing with the Danish and NL releases of heavy metals. This is done by comparing the Danish releases of metals presented above with estimated
Danish releases based on an extrapolation by use of EUROSTAT data. The extrapolation is based on linear extrapolation by use of the Gross Domestic Product (GDP). Similarly metal releases for the
Netherlands, reported by van der Auweraert et al. (1996), are compared with an estimate based on EUROSTAT-data. The data are compared in Table 10-4.
Table 10-4
Estimated emissions of selected metals to the aquatic environment.
Metal emission t/year |
EU-15 |
EU-15 |
DK |
DK |
NL |
NL |
|
Present report |
Extra- polated from NL data 19961 |
Extrapolated from EUROSTAT 19981 |
Present report3 |
EURO.STAT 1998 data |
NL data4 1996 |
Hg |
13 |
14.4 |
0.3 |
0.7 |
6 |
0.646 |
Cd |
40 |
41.1 |
0.9 |
0.9 |
13 |
1.84 |
Cu |
1855 |
1840 |
38.9 |
41 |
555 |
82.2 |
Zn |
9600 |
11100 |
213.3 |
123 |
3522 |
495 |
Pb |
1538 |
2820 |
31.9 |
7.42 |
702 |
126 |
Extrapolation based on the Gross Domestic Product (GDP). NL data by van der Auweraert et al. (1996)
The figure does not include loss of commercial fishing equipment, which is estimated to be approx. 100-275 tonnes/yr. (Lassen & Hansen 1996).
Estimates based on measured wastewater concentrations and releases from other sources.
Data by van der Auweraert et al. (1996).
The data for the EU-15, which are based on extrapolation from EUROSTAT data, correspond well with the results obtained by extrapolation from the Netherlands by use of the Gross Domestic Products
(GDP). This apparent compliance covers, however, some methodological differences and uncertainties.
The data on releases of cadmium, copper, and zinc to the Danish aquatic environment correspond within a factor of 2 with the extrapolated EUROSTAT-data. However, the figures for emission of mercury
and lead show more significant differences.
The EUROSTAT data for the NL are a factor 5 - 10 higher than the data reported by van der Auweraert et al. (1996). These data are based on measured concentrations in municipal and industrial
discharges and can be regarded as highly reliable. The differences can be explained by the fact that the EUROSTAT data are based on riverine inputs to the North Sea, i.e. measurements of river water,
including emissions from Germany and other countries upstream as well as diffuse and natural sources.
The composition of the wastewater and thus the emissions of organic micropollutants and heavy metals depend on the treatment processes applied. The well-developed treatment technologies used in some
European countries reduce the pollutants in the wastewater more efficiently than less sophisticated techniques. This will, together with differences in the composition of the industrial sectors between
countries, introduce an error when the Gross Domestic Product is used as a basis for extrapolation.
The most important uncertainties are:
- The estimates from EUROSTAT are based on riverine and direct inputs to the marine environment and thus include natural sources. The figures do not include emissions of metals, which are bound to
sediments upstream in the river basins. Furthermore, the used figures represent minimum estimates because zero values are used in case the concentrations are below the detection limits.
Comparison with Danish estimates based on measured concentrations in wastewater and estimated releases corresponds, however, well with the EUROSTAT data.
- For some countries the releases of metals are based on extrapolation from NL data. It should be stressed that differences in the industrial sources and the wastewater treatment methods applied in
European countries are an important source to uncertainty of the estimated releases.
- Only some metals are included in the estimates. By focusing on the dominating toxicants this error is, however, minimised, but the results should be regarded as a minimum estimate of the ecotoxicity
potential.
10.4.2 Emission of organic substances
10.4.2.1 Data sources
The emission of toxicants from industrial sources is difficult to estimate because of the differences in the composition of the wastewaters and the limited amount of data. The normalisation reference used by
Hauschild et al. (1998) was based on directly measured toxicities of Danish industrial wastewater emissions. These data are, however, not updated and probably not available from other countries.
In general, there are only few data on emissions of organic micropollutants to the aquatic environment in Europe. The most comprehensive data compilation is made by van der Auweraert et al. (1996),
which covers emissions from over 700 industrial sources and municipal treatment facilities in NL and includes approximately 100 individual parameters. Furthermore, a few organic micropollutants are
reported by DETR (1999). In Germany the industrial emissions are estimated for selected industrial sectors only, but no national emission reference has been found (UBA 1999).
In Denmark a few selected organic substances have been monitored in wastewater from municipal treatment plants, but information on the emission of individual organic substances from industrial
sources does not exist. Some of the most reliable Danish data are estimates of the emissions of selected substances based on mass balances on national levels and statistical reports published by the Danish
EPA on an annual basis (DEPA 1995). Moreover, the Danish EPA has published a collection of data on emission of 38 single substances including organic solvents and heavy metals (Holmegaard
Hansen, 1995). None of these data sources provides a base of data as extensive as the NL data.
Consequently, the emissions of organic substances via Danish and European wastewater sources are, in the present report, based on NL data extrapolated by use of GDP. To some extent these estimates
can be validated by comparison with available national data (see discussion).
10.4.2.2 Results
Data for the environmental release of organic pollutants in NL are presented in Table 10-5. Industrial direct outlets as well as municipal wastewater emissions are included in the figures.
Table 10-5
Emission of organic substances to the aquatic environment from industrial and municipal sources. The data from the Netherlands are based on detailed estimations and measurements from over 700 individual
industries. The data are extrapolated to Denmark and EU-15 by use of GDP. The number of decimals does not reflect the precision of the data. The uncertainties are discussed below.
Emission of organic substances |
|
NL |
DK |
EU-15 |
|
|
tonnes/yr. |
Aliphatic compounds |
|
5550.00 |
2466.01 |
124191.64 |
|
Benzene |
165.00 |
73.31 |
3692.18 |
|
Benz(a)pyrene |
3.34 |
1.48 |
74.74 |
|
Ethylbenzene |
0.00 |
0.00 |
0.00 |
|
Fluoranthene |
15.80 |
7.02 |
353.55 |
|
Phthalates |
0.11 |
0.05 |
2.53 |
|
Isopropylbenzene |
0.40 |
0.18 |
8.93 |
|
PAH1 (6 comp.) |
18.50 |
8.22 |
413.97 |
|
Toluene |
413.00 |
183.51 |
9241.65 |
|
Xylenes |
165.00 |
73.31 |
3692.18 |
Total aromates |
|
1210.00 |
537.63 |
27076.01 |
Halogenated org. compounds |
|
29.80 |
13.24 |
666.83 |
Chlorinated aliphates |
|
28.50 |
12.66 |
637.74 |
|
1,2-Dichloroethane |
0.42 |
0.19 |
9.33 |
|
Hexachlorbutadiene |
0.00 |
0.00 |
0.00 |
|
Hexachlorcyclohexane |
0.01 |
0.00 |
0.15 |
|
Tetrachlorethylene |
0.08 |
0.04 |
1.83 |
|
Tetrachlormethane |
0.35 |
0.15 |
7.74 |
|
1,1,1-Trichloroethane |
0.06 |
0.03 |
1.31 |
|
Trichloroethene |
0.16 |
0.07 |
3.49 |
|
Trichloromethane |
0.24 |
0.10 |
5.28 |
|
Vinylchloride |
0.00 |
0.00 |
0.04 |
Chlorinated aromates |
|
1.24 |
0.55 |
27.75 |
|
Chlorobenzene |
0.10 |
0.04 |
2.24 |
|
DRINS2 |
0.00 |
0.00 |
0.07 |
|
Hexachlorobenzene |
0.01 |
0.01 |
0.32 |
|
PCB3 |
0.00 |
0.00 |
0.02 |
|
Pentachlorophenol |
0.01 |
0.01 |
0.26 |
|
Trichlorobenzene |
0.00 |
0.00 |
0.02 |
1 PAH: Polycyclic Aromatic Hydrocarbons. van der Auweraert et al. (1996) report emissions of PAH to water as the sum of 6 individual and non-specified compounds.
2 DRINS: Common name for the group of commonly used cyclodien insecticides: Aldrine, dieldrine and endrine.
3 PCB: Polychlorinated Biphenyl Compounds
The estimate covers only selected prioritised industrial pollutants. Moreover, the estimated ecotoxicity potential is based only on substances where equivalency factors (EF) are published by Hauschild et
al. (1998). For a few additional organic substances, the Water Quality Standards issued by the Danish EPA were used for the estimation of the ecotoxicity potential; see Table 10-6.
Table 10-6
Estimated ecotoxicity potential (EP) for organic toxic substances emitted to the aquatic environment.
Country |
EPetwc m3/yr. |
EPetwa m3/yr. |
Netherlands |
2.89*1011 |
4.25*1010 |
Denmark |
3.75*1011 |
2.30*1010 |
EU-15 |
1.89*1013 |
1.16*1012 |
1 The estimates are based on equivalency factors (EF) according to Hauschild et al. (1998), except for aliphatic substances where the ecotoxicity has been assumed to equal that of crude oil.
The background data are included in Appendix B.1.
10.4.2.3 Discussion
The estimated ecotoxicity potential includes only approximately half of the substances presented in Table 10-5 (see Appendices B.1 and B.2). Aliphatic substances account for the major part of the
released compounds in Table 10-5. No EF is, however, available for this group of substances, but if the ecotoxicity is assumed to be equivalent to the toxicity of crude oil, the aliphatic compounds will
contribute with 66% of the chronic toxicity potential and 18% of the acute toxicity potential. The chronic and acute ecotoxicity potentials for EU would in this case be 1.89*1013 m3/yr. and 1.16*1012
m3/yr., respectively. These figures are used in the calculation of the normalisation reference as they are assumed to provide a more consistent result.
The Danish emission data presented above can be compared with reported releases of benzene, toluene, and xylenes via wastewater (Hansen 1995). The reference covers only selected industrial sources
and thus gives only a rough picture of the releases. The author reported releases of benzene <1 tonnes/yr., and releases of toluene and xylenes at 20 tonnes/yr. and >10 tonnes/yr., respectively. The low
emission reported for benzene may be explained by the fact that fuel is not included in the estimates. As for toluene the reported emission is about 9 times lower than the figures for Denmark presented in
Table 10-5.
The uncertainties of the estimated effect potentials can be validated by comparing with measured emission data. In Table 10-7 values for the release of selected organic pollutants in the UK calculated by
extrapolation from NL data are compared with measured riverine and direct inputs reported by the DETR (1999). The figures show considerable differences. The estimated emission of trichloroethane is 10
times higher than the highest values reported by the DETR. Also the estimated emissions of pentachlorophenol are a factor of 4-5 times higher than the measured riverine inputs.
The differences may partly be explained by the uncertainties introduced by extrapolation and partly by the fact that emission data based on measured riverine inputs underestimate the actual emissions, as
biodegradation, evaporation, and adsorption will reduce the concentrations of many organic substances in the water phase between the point of emission and the monitored sampling point.
In addition to the organic substances discussed above measured releases of selected metals in the UK are compared with extrapolated values in Table 10-7. The values based on riverine inputs reported by
the DETR are generally a factor of 3-10 higher than the estimated figures. It should be stressed that data on heavy metals based on riverine inputs include diffuse sources and natural background levels and
therefore tend to overestimate the emissions from man-made sources. Moreover, unlike most organic substances, metals do not degrade or evaporate from aquatic environments.
From these data it is concluded that an extrapolation from NL data to other European countries seems to give uncertain but useful estimates of the emission to the aquatic environment.
Table 10-7
Data on emission to the aquatic environment of selected organic and
inorganic substances. The values reported by DETR are based on inputs
from point sources and riverine inputs (DETR 1999). The extrapolated
values are based on data from the Netherlands (van der Auweraert et al.
1996) extrapolated by use of GDP.
Substance |
T/year. |
|
Extrapolated from NL data1 |
DETR low1 |
DETR high1 |
1,1,1-Trichloroethane |
179 |
5.90 |
17.60 |
Pentachlorophenol |
35 |
1.32 |
7.75 |
Arsenic |
15 |
82 |
163 |
Chromium |
82 |
301 |
347 |
Nickel |
103 |
307 |
348 |
1 Low values: Concentrations below detection limits are set to zero. High values: Concentrations below detection limits are considered to equal the detection limits.
The presented ecotoxicity potential for organic pollutants to the aquatic environments is associated with a number of uncertainties:
- In general, there are very few data on emission of organic toxicants to the aquatic environment. The most extensive data compilation is from the Netherlands (van der Auweraert et al., 1996), which is
used here. Of the parameters included in Table 10-5, EF are available for only about half of the substances. As discussed above the emission of aliphatic compounds may very well contribute with
over half of the actual ecotoxicity potential released to the aquatic environment in Europe. Because of lack of information on the type of substances included in this group as well as missing effect
factors, the aliphatic compounds are not included in the estimated ecotoxicity potential presented here. Consequently, the estimates should be regarded as minimum values of the release of toxicants
to the aquatic environment.
- There are considerable differences in the composition of the industrial sector between countries. This is likely to bias the estimated ecotoxicity potential. As DK has relatively few chemical industries,
the figures for DK are probably overestimated.
- There are differences in the wastewater treatment techniques applied in different European countries and thus the retention of organic pollutants in the treatment facilities. In countries like Germany, the
Netherlands, Denmark, and Sweden two- or three-step wastewater treatment techniques are used, which retain organic and inorganic pollutants more efficiently than more simple techniques in the
Mediterranean region. Consequently the estimated releases from these countries are probably underestimated.
10.4.3 Oil extraction and spills
10.4.3.1 Data sources
Oil extraction, refineries, and sea transport contribute significantly to the pollution of the marine environment. Data on emissions from refineries and production facilities in the North Sea are reported by
EUROSTAT (1998). The data include emission from land and sea based on point sources including refineries, off shore oil extraction facilities, spills from handling of oil from terminals, etc. as well as legal
spills from ships. The data do not include emissions from the point sources discussed above, i.e. wastewater emissions from industries and WWTP, as well as accidental spills from tankers, because
accidental emissions in general are not included in the normalisation reference. Spills from tankers are registered by the International Tankers Organisation (ITOPF 1999) and may contribute on a
yearly basis with quantities that are comparable in size or higher than all other sources together. Furthermore, data on emissions to the Mediterranean sea are not included.
10.4.3.2 Results
The data on releases of oil and crude oil etc. are presented in Table 10-8.
Table 10-8
Emissions of crude oil etc. from refineries, off-shore production facilities in the
North Sea, and legal discharges from ships. Accidental spills are not included.
Source |
Emission |
EF (etwc)1 |
EF (etwa)1 |
EP (etwc) |
EP (etwa) |
|
t/yeaR2 |
m3/g |
m3/g |
m3/yea. |
m3/year |
Refineries |
4200 |
100 |
1.67 |
4.20*1011 |
7.01*109 |
Off-shore |
25005 |
2.50*1012 |
4.18*1010 |
Oil terminals etc. |
2000 |
2.00*1011 |
3.34*109 |
Legal ship discharges |
5100 |
5.10*1011 |
8.52*109 |
Sum |
29205 |
|
|
3.63*1012 |
6.06*1010 |
1 The estimates are based on calculated equivalency factors (EF) as defined in Hauschild et al. (1998). See discussion below.
2 EUROSTAT (1998).
EP Ecotoxicity potential
The ecotoxicity potential from oil is calculated on the basis of a Predicted No-Effect Concentration (PNEC) for acute toxicity to aquatic organisms corresponding to 0.6 mg/l and a PNEC for chronic toxicity
of 0.01 mg/l based on data for crude oil (IUCLID, 1996). The values are thus based on more recent data than used by Hauschild et al. (1998). The corresponding equivalency factors are: etwa = 1.67
m3/g and etwc = 100 m3/g. Crude oil is here regarded as slowly degradable in the marine environment although some fractions may degrade more rapidly or evaporate from the water phase. The resulting
ecotoxicity potential is shown in Table 10-8.
10.4.3.3 Discussion
The estimated release of oil is associated with a number of uncertainties:
- The Mediterranean Sea is not included in the reported emissions.
- Accidental spills are a significant source, which according to the applied methodology is not included in the present evaluation. The reported yearly spills from the International Tankers Organisation
(ITOPF, 1999) show average spills from 1987-1997 ranging 9,000 - 435,000 tonnes on a world basis with an average of 147,000 tonnes/yr. In 1994 the spills in European seas were about 25,000
tonnes, i.e. a volume comparable with the non-accidental emissions in the North Sea.
10.4.4 Antifouling
10.4.4.1 Data sources
Antifouling paints released from ships are a significant source for toxic substances released to the marine environment. Antifouling agents for commercial ship transport are normally based on the highly
toxic tributyltin-compounds (TBT). The emission of tin to the Danish marine environment is estimated to be 0.6-4.9 tonnes TBT/yr. (DEPA 1997b). It has not been possible to identify other data sources.
10.4.4.2 Results
The release of TBT to the Danish marine waters is extrapolated to a European level by comparing the size of the Danish water area (approx. 43,000 km2) with the European water area (20% of the total
area = 809,000 km2 ). The figures should be regarded as a rough estimate of the release of organotin compounds from antifouling paints.
The Danish EPA has set a Water Quality Standard (WQS) at 0.001 mg/l of bis-tributyltiNOxide (TBTO) corresponding to 0.00097 mg TBT/l. The equivalency factor for tributyltin used here is based on
the Danish WQS (Danish Ministry of Environment, 1996). Table 10-9 shows the estimated equivalency factors based on these data.
Table 10-9
Ecotoxicity potential for organic tin-compounds (as tributyltiNOxide, TBTO)
released from antifouling paint to the Danish marine environment.
|
Emission of org. tin comp. |
EF |
EP |
|
t/year 1 |
(etwc) m3/g 2 |
(etwa) m3/g 2 |
(etwc) m3/yr. |
(etwc2) m3/g |
DK |
Low1 |
0.6 |
1.03*106 |
1.03*105 |
6.18*1011 |
6.18*1010 |
High1 |
4.9 |
5.05*1012 |
5.05*1011 |
Average |
|
2.83*1012 |
2.83*1011 |
EU-15 3 |
Low |
11.4 |
1.03*106 |
1.03*105 |
1.17*1013 |
1.17*1012 |
High |
93.1 |
9.59*1013 |
9.59*1012 |
Average |
|
5.38*1013 |
5.38*1012 |
1 Emission estimates from DEPA (1997b). Low = low end of the estimated range. High = high end of the estimated range.
2 The chronic equivalency factor for organotin is based on the water quality standards according to the Danish statutory order 921/1996 (Danish Ministry of Environment, 1996). The acute equivalency
factor is a factor of 10 lower than the chronic factor.
3 Estimates for EU-15 are based on the area of marine water: Marine area in DK = approx. 43,000 km2, marine area in EU-15 is 20% of the total area (approx. 809,000 km2).
EF Equivalency factor.
EP Ecotoxicity potential.
10.4.4.3 Discussion
The emission of organotin compounds from antifouling paints is based on a rough estimate of the marine releases in Denmark. This figure is extrapolated to a European level by use of the water area. The
uncertainty of the estimate depends on the size of the traffic through the Danish marine areas compared to that of other European waters and the size of the area included in the estimates (in this report
calculated as 20% of the total EU-15 area).
Because of the relatively large contribution of this source and the high uncertainty of the estimate, it is recommended to validate these figures further.
10.4.5 Atmospheric depositions
10.4.5.1 Data sources
The atmospheric deposition of toxicants to the aquatic environment is estimated according to the principles described in Hauschild et al. (1998). A discussion of the available data and the methods used for
the estimation of the normalisation reference is found elsewhere in this report.
10.4.5.2 Results
The estimated deposition to the aquatic environment is shown in Table 10-10. The background data are included in Appendix C.
Table 10-10
Estimated contribution of toxic substances from deposition.
Deposition |
EP Aquatic chronic m3/yr. |
DK EU-15 |
1.37*1011 1.24*1013 |
10.4.5.3 Discussion
The estimated ecotoxicity potential is primarily based on the deposition of metals and includes only the organic toxicants where effect factors are available. The estimate should therefore be regarded as a
minimum level.
10.5 Normalisation reference - Terrestrial environment
The estimated releases of toxic substances to the terrestrial environment include the same types of sources as evaluated by Hauschild et al. (1998): Pesticides, agricultural use of sewage sludge, and
atmospheric depositions. The emission types and data references included are presented in Table 10-11.
Table 10-11
Emission types and selected references used for the estimation of emissions to
the terrestrial environment.
Emission type |
Data source |
Use of pesticides |
Use of pesticides in Denmark (DEPA 1997a) EEA, second assessment (EEA 1998)
OECD Environmental Data (OECD 1997) |
Sewage sludge used for agricultural purposes |
Use of Waste Products in Agriculture (Tørsløv et al.
1997) OECD Environmental Data (OECD 1997) |
Atmospheric depositions |
Data from sub-activity on human toxicity (chapter 9) |
10.5.1 Use of pesticides
10.5.1.1 Data sources
The dominating source of toxicant emission to the terrestrial environment is the agricultural use of pesticides. Statistics on the use is available from EEA (EEA 1998), OECD (1997) and from the national
EPA's, e.g. DEPA (1997a). The toxicity pressure from pesticides depends on the toxicity of the individual active ingredient used and the number of applications recommended for different crop types. The
large number of pesticides used makes such an estimate very complex.
For this reason the approach previously used by Hauschild et al. (1998) has been applied in the present report with some modifications.
The Danish data on use of pesticides are relatively detailed and allow calculations of the number of applications used for each of the main categories of pesticides (as active ingredients): Herbicides,
fungicides, and insecticides. It is possible for each of these types to calculate an application factor expressing the average number of applications per ha for the different types of pesticides. The toxicity
pressure expressed as the number of applications multiplied by the size of the treated area can then be estimated from the use of pesticides in the different countries.
10.5.1.2 Results
The estimated toxicity potential applied with the use of pesticides is presented in Table 10-12. The background data are presented in Appendix D.
Table 10-12
Estimated ecotoxicity potential for use of pesticides.
Pesticide type |
Use1 t/year |
EP (m3/year) |
Distribution % |
DK |
|
|
|
Fungicides |
892 |
3.504*1011 |
10 |
Herbicides |
2685 |
2.848*1012 |
83 |
Insecticides |
95 |
2.147*1011 |
6 |
SUM |
|
3.413*1012 |
|
EU-15 |
|
|
|
Fungicides |
199191 |
7.825*1013 |
22 |
Herbicides |
119843 |
1.271*1014 |
36 |
Insecticides |
67148 |
1.518*1014 |
42 |
SUM |
|
3.571*1014 |
|
1 Use of pesticides (active ingredients): Danish data for 1994 from DEPA (1997a). European data for 1995 from OECD (1997).
10.5.1.3 Discussion
The estimated ecotoxicity potential is associated with a number of uncertainties:
- The differences in toxicity of the pesticides are not considered, i.e. the equivalency factor is based on a very rough estimate of a common PNEC value for all pesticides.
- Differences in application rates and praxis, crop types, climatic conditions, and national regulations are not considered.
10.5.2 Sewage sludge used for agricultural purposes
10.5.2.1 Data sources
Large quantities of sewage sludge are applied as agricultural fertiliser in most European countries. The normalisation reference estimated by Hauschild et al. (1998) was based on the content of heavy metals
and tensides only. Since then extensive studies have made more comprehensive data available on the contents of organic pollutants in sewage sludge (Tørsløv et al. 1997).
These data are used in the present report for the estimation of the environmental release of toxicants via sewage. Data for use of sludge in agriculture in the European countries are derived from a study
from 1994 by Hall and Dalimier (1994).
10.5.2.2 Results
The resulting EP from the use of sludge in agriculture is presented in Table 10-13. The background data are found in Appendices E.1 and E.2.
Table 10-13
Ecotoxicity potential from sewage sludge used in agriculture.
|
Agricultural use1 |
EP soil2 |
|
tonnes/yr. |
etwc m3/yr. |
DK |
1.29*105 |
1.89*107 |
EU-15 |
3.66*106 |
5.35*108 |
- Sewage sludge used in agriculture. Data from DEPA (1998), OECD (1997),
and Hall and Dalimier (1994).
- Ecotoxicity potential calculated from the contents of toxicants in sludge
(Tørsløv et al. 1997 and Hauschild et al. 1998). Additional equivalency
factors derived from Jensen and Folker Hansen (1995).
10.5.2.3 Discussion
The ecotoxicity from the application of sewage sludges in agriculture is based on Danish data for contents of toxicants in sludge. These figures are in agreement with reported values for Swedish sludges but
should be regarded as a low estimate of the contents of sludge from more heavily industrialised European regions. In addition the content of toxicants will depend on the design of the treatment facility, for
example the hydraulic retention time and sludge age. Moreover, only a limited number of toxic substances are included in the estimate. By focusing on the dominating toxicants this error is minimised, but
the estimate should be regarded as a realistic minimum of the ecotoxicity potential.
The overall contribution of sewage sludge is, however, small compared to other terrestrial sources.
10.5.3 Atmospheric depositions
10.5.3.1 Data sources
The atmospheric deposition of toxicants to the terrestrial environment is estimated according to Hauschild et al. (1998). A discussion of the available data and the methods used for the estimation is found in
the section on human toxicity.
10.5.3.2 Results
The estimated deposition to the terrestrial environment is shown in Table 10-14. The background data are presented in Appendix C.
Table 10-14
Estimated contribution of toxic substances to the terrestrial environment from deposition.
|
Ecotoxicity potential terrestrial chronic estc m3/yr. |
DK |
5.90*107 |
EU-15 |
3.51*1010 |
10.5.3.3 Discussion
The atmospheric deposition contributes with about half of the ecotoxicity potential emitted to the soil environment in Europe. 96% of this contribution is caused by selenium. The deposition of selenium is
twice the deposition of mercury and three times the deposition of cadmium, i.e. metals that are normally regarded as more serious pollutants. Nevertheless, the estimated toxicity potential for deposition of
selenium on soil is 50 and 180 times higher than that for mercury and cadmium, respectively.
It is recommended to re-evaluate the equivalency factor of selenium.
10.6 Normalisation references
The data and estimates presented above are summarised in Table 10-15. In addition to the calculated ecotoxicity potential for DK and EU-15 an estimate of the ecotoxicity potential on a world basis is
presented. The normalisation data for DK and EU-15 is discussed in the sections above.
Assuming correlation among acidification, GDP and ecotoxicity the worldwide normalisation reference is calculated by a simple extrapolation from the EU-15 data to a world basis by use of the Gross
Domestic Products. Data for the emission of toxic substances to the environment was found for high-income countries only. Because of the lack of data for countries with lower per capita income it was
not possible to differentiate between income groups in the extrapolation of releases of toxic substances. Thus the extrapolation presented here does not take the differences between countries with respect to
degree of industrialisation, pesticide use etc. into consideration.
Table 10-15
Ecotoxicity potentials for emissions of toxic substances to the environment in Denmark and EU-15. A worldwide normalisation reference is calculated by extrapolation from EU-15 data by use of Gross
Domestic Products.
Substances |
EP etwc (m3/yr) |
% |
EP etwa (m3/yr) |
% |
EP etsc (m3/yr) |
% |
|
|
|
|
|
|
|
DK |
|
|
|
|
|
|
Wastewater |
Organic substances |
3,75E+11 |
9 |
2,30E+10 |
6 |
|
|
Metals |
7,77E+11 |
19 |
7,88E+10 |
20 |
|
Pesticides |
Fungicides |
|
|
|
3,50E+11 |
10 |
|
Herbicides |
|
|
|
2,85E+12 |
83 |
|
Insecticides |
|
|
|
2,15E+11 |
6 |
Sludge |
Organic substances+metals |
|
|
|
1,89E+07 |
0,00 |
Deposition, water |
1,37E+11 |
3 |
|
|
|
|
Deposition, soil |
|
|
|
|
5,90E+07 |
0,00 |
Antifouling |
Organotin compounds |
2,83E+12 |
69 |
2,83E+11 |
74 |
|
DK |
|
4,12E+12 |
|
3,85E+11 |
|
3,41E+12 |
Person equivalent |
7,91E+05 |
|
7,40E+04 |
|
6,56E+05 |
|
|
|
|
|
|
|
|
EU15 |
|
|
|
|
|
|
|
|
|
|
|
|
|
Wastewater |
Organic substances |
1,89E+13 |
14 |
1,16E+12 |
11 |
|
|
Metals |
4,16E+13 |
32 |
4,19E+12 |
39 |
|
Pesticides |
Fungicides |
|
|
|
7,83E+13 |
22 |
|
Herbicides |
|
|
|
1,27E+14 |
36 |
|
Insecticides |
|
|
|
1,52E+14 |
42 |
Sludge |
Organic substances+metals |
|
|
|
5,35E+08 |
0,0001 |
Oil emission |
|
3,63E+12 |
3 |
6,06E+10 |
1 |
|
Deposition, water |
|
1,24E+13 |
10 |
|
|
|
Deposition, soil |
|
|
|
|
3,51E+10 |
0,010 |
Antifouling |
|
Organotin compounds |
5,38E+13 |
41 |
5,38E+12 |
50 |
|
|
|
|
|
|
|
EU15 |
|
1,30E+14 |
|
1,08E+13 |
|
3,57E+14 |
Person equivalent |
3,52E+05 |
|
2,91E+04 |
|
9,64E+05 |
|
|
|
|
|
|
|
|
World |
|
|
|
|
|
|
Person equivalent (extrapolated) |
2.82E+05 |
|
2.33E+04 |
|
7.71E+05 |
|
Table 10-15 shows the annual contribution of the individual emission types and types of substances to the overall ecotoxicity potential (EP) in m3 released to the environment. By using the extrapolation
method proposed in chapter 3, Development of normalisation references for different geographic areas, the worldwide normalisation reference for ecotoxicity can be estimated to 7.95*104 m3/capita/year
(etwc), 6.57*103 m3/capita/year (etwa), and 2.78*105 m3/capita/year (etsc). These normalisation references are slightly above the references estimated by extrapolation of the effect potential.
The contribution to the aquatic environment is dominated by the release of organotin compounds from antifouling paints that contributes with over 70% of the total aquatic emissions in Denmark, and about
50% of the aquatic emissions in Europe. The EP for organotin is based on an emission to the Danish aquatic environment of organotin of 2.8 tonnes per year representing a range from 0.6-4.9 tonnes per
year. If the lower end of this interval is used instead of an average value the contribution from organotin compounds is reduced to 37% of a total EP for chronic toxicity released to the Danish aquatic
environment at 1,66*1012 m3/yr. In this case metal emitted from point sources will contribute with 47%. If the high end of the interval is used organotin will contribute with 83% of the emitted EP and metals
with only 13%.
Emission of metals to the aquatic environment accounts for 40% of the emission in EU-15 and 20% of the Danish emissions. Copper contributes with 21% in EU-15 and with 14% in Denmark. Also zinc
contributes significantly (9 and 3%, respectively).
The use of pesticides contributes with almost 100% of the EP to the Danish and EU-15 terrestrial environments. This holds true even if the contribution from the pesticide use is assumed to be 10 times lower
than assumed in the estimates of the toxicity from this source. The second largest contribution to terrestrial toxicity is thus the atmospheric deposition, mainly caused by selenium. According to Hauschild
et al. (1998) selenium has a very high equivalency factor for chronic toxicity in soil, when emitted to the air (106 m3/g), compared to metals like cadmium (1.8 m3/g) and mercury (5.3 m3/g). This is caused
by a combination of a relatively low partitioning coefficient for selenium in soil and a high toxicity.
10.7 Comparison with the previously used normalisation reference
In Table 10-16 the estimated normalisation references are compared with the values for 1990-1992 calculated by Hauschild et al. (1998).
Table 10-16
The estimated total ecotoxicity normalisation reference for Denmark compared to the values for the years 1992-1994 in Hauschild et al. (1998).
|
|
Denmark |
EU-15 |
World |
ETWC, 1990
1994 |
m3 water/capita/year |
4.7*105 |
- |
- |
7.91*105 |
3.52*105 |
2.82*105 |
ETWA, 1990
1994 |
m3 water/capita/year |
4.8*104 |
- |
- |
7.40*104 |
2.91*104 |
2.33*104 |
ETSC, 1990
1994 |
m3 soil/capita/year |
3.0*104 |
- |
- |
6.56*105 |
9.64*105 |
7.71*105 |
ETWC: Ecotoxicity Potential for chronic effects on aquatic organisms.
ETWA: Ecotoxicity Potential for acute effects on aquatic organisms.
ETSC: Ecotoxicity Potential for chronic effects on soil organisms.
The EP for the aquatic environment is about 1.5 times higher than estimated in 1990-1992. Considering the uncertainties involved, the estimates are, however, comparable in size. The main differences are
the contribution from organotin compounds to the aquatic environment and the lower deposition of heavy metals in the present estimate compared to the earlier estimates.
The present estimate for the terrestrial environment is considerably higher than earlier reported. This is mainly caused by a higher estimated ecotoxicity potential for pesticides (a factor of 65 higher).
It should be stressed, however, that the observed differences are mainly caused by methodological differences rather than changes in the environmental releases.
10.8 Evaluation of uncertainties
The uncertainties discussed above are summarised in Table 10-17. The uncertainties are scored in three categories: "Low", i.e. an uncertainty expected to be considerably lower than one order of
magnitude, "medium", i.e. an uncertainty expected to be up to one order of magnitude, and "high", i.e. an uncertainty expected to be higher than one order of magnitude. The uncertainties are due to both
the applied methodology and limitations of data. It should be stressed that these uncertainties are subjective and based on the author's experiences obtained during the project. Within the frame of this project
it was not possible to estimate the size of the uncertainties more precisely.
The overall uncertainty of the normalisation reference is expected to be high as the uncertainties of the dominating sources of toxicant releases in the aquatic as well as the terrestrial environments are high, i.e.
the release of organic tin compounds from antifouling paints used on ships and from the use of pesticides in agriculture.
Table 10-17
Summary of the expected uncertainties of the presented ecotoxicity potentials of the individual sources of toxicants. It is stressed that the uncertainties are based on the author's subjective impressions
and do not represent a quantitative estimate.
Emission type |
Main uncertainties |
Contribution % (EU-15 data) |
Uncertainty |
Aquatic environment |
Heavy metals |
Release data are only available for few substances |
32-39 |
Medium |
Organic substances |
Release data and effect factors are only available for few substances |
11-14 |
Medium |
Oil extraction, etc. |
The reported emissions do not include the Mediterranean sea |
1-3 |
Medium |
Antifouling |
Very uncertain release data |
41-50 |
High |
Deposition, water |
Limitations of data and available effect factors |
10 |
Medium |
Terrestrial environment |
Pesticide use |
The applied method for estimation of the ecotoxicity potential introduces
a high uncertainty. The release figures are reliable |
100 |
High |
Sewage sludge |
The uncertainty of both data and the applied method is low |
0 |
Low |
Atmospheric deposition |
Limitations of data and available effect factors |
0 |
Medium |
10.9 Recommendations
Most of the data sources used in the estimates are linked to international or national statistics, which are regularly updated. It should therefore be relatively simple to update the calculations. The two
dominating sources of toxicant release: Pesticides and tributyltin compounds used in antifouling paints are, however, based on rather rough assumptions and non-validated data. Statistics on the use of
pesticides are available on a yearly basis, but the estimated ecotoxicity potentials are based on non-validated assumptions. Data on the release of tributyltin are not available, and the presented figures are
based on rough estimates of the release to the Danish aquatic environment. It is recommended that the ecotoxicity potentials for these emission types are further validated.
The estimated ecotoxicological impact is based on relatively limited data on environmental releases of selected organic and inorganic substances and should in general be regarded as a minimum estimate
because it was impossible to include all types of sources, and only some of the substances that are known to be emitted are included in the presented estimates.
It is recommended to validate the estimated ecotoxicity potential by comparison with chemical consumption data combined with release factors. This will probably represent a maximum estimate of the
actual release levels. It was, however, not possible within the frame of the present project to perform this validation.
10.10 References
DEPA (Danish Environmental Protection Agency) 1995, Punktkilder 1994. Vandmiljøplanens overvågningsprogram (point sources 1994, the monitoring programme of the Aquatic Environment
Plan). Information No. 10. In Danish.
DEPA (Danish Environmental Protection Agency) 1996, Massestrømsanalyse for kviksølv (mass flow analysis of mercury). Environmental Project No. 344. In Danish.
DEPA (Danish Environmental Protection Agency) 1997a, Bekæmpelses-middelstatistik 1996 (statistics for pesticides 1996). Information No. 10. In Danish.
DEPA (Danish Environmental Protection Agency) 1997b, Massestrømsanalyse for tin med særligt fokus på organotinforbindelser (mass flow analysis of tin focusing on organotin compounds). Working
report No. 7. In Danish.
DEPA (Danish Environmental Protection Agency) 1998, Affald 21 Udkast til affaldsplan 1998-2004 (draft for waste plan). Draft for a hearing of 25th September, 1998. In Danish.
DETR (1999) Department of the Environment, Transport and the Regions. Environmental statistics. http://www.detr.gov.uk.
EUROSTAT F3 Environment Unit 1998, Towards environmental indices: A first exercise. Volume 1: Background report - final. Consultora Ambiental, S.L., Spain.
EEA 1998, Europe's Environment: The Second Assessment. Eurostat, European Commission, European Environment Agency. Office for Official Publications of the European Commission, Luxembourg.
Grüttner, H. & Neergaard Jacobsen, B. 1994, Miljøfremmede stoffer i renseanlæg. Belastning og renseeffekt (xenobiotic substances in wastewater treatment plants, load and effect of treatment
process). Environmental project No. 278. In Danish. Danish Environmental Protection Agency.
Grüttner, H., Vikelsøe, J. & Pritzl, G.1996, Miljøfremmede stoffer i spildevand og slam. Massestrømsanalyser for renseanlæg (xenobiotic substances in municipal wastewater and sludge, mass flow
analyses of WWTP). Environmental project No. 325. In Danish. Danish Environmental Protection Agency.
Hall, J.E. & Dalimier, F. 1994, Waste management - sewage sludge. Part 1. Survey of sludge production, treatment, quality and disposal in the European Union. Report No. EC 3646(P). EC
reference No. B4-3040/014156/92.
Hauschild, M., Wenzel, H., Damborg, A. & Tørsløv, J. 1998, Ecotoxicity as a criterion in the environmental assessment of products in Environmental assessment of products. Volume 2 Scientific
background eds. Hauschild. M. & Wenzel. H. London: Chapman & Hall.
Hauschild, M. & Wenzel, H. 1998, Environmental assessment of products. Volume 2. Scientific background. Chapman & Hall, Thomson Science, London, UK.
Holmegaard Hansen, J. 1995, Nationale og industrielle emissioner af 38 stoffer (national and industrial emissions from 38 substances). Working report No. 64. In Danish. Danish Environmental
Protection Agency.
ITOPF 1999, Objective technical advice, expertise, assistance and information on effective response to ship-source pollution. Historical data. http://www.itopf.com. The International Tanker Owners
Pollution Federation Limited.
IUCLID 1996, International Uniform Chemical Information Database. Existing chemicals. 1 ed. European Chemicals Bureau. Environment Institute, Ispra, Italy.
Jensen, A. & Markussen, J. 1993, Forbrug af og forurening med cadmium (consumption of cadmium and pollution by cadmium). Environmental Project No. 213. In Danish. Danish Environmental
Protection Agency.
Jensen, J. & Folker-Hansen, P. 1995, Soil quality criteria for selected organic compounds. Working report No. 47. Danish Environmental Protection Agency.
Jepsen, S.-E. & Grüttner, H. 1997, Miljøfremmede stoffer i husholdningsspildevand. Måleprogram for udvalgte stoffer (xenobiotic substances in domestic wastewater, measuring programme for
selected substances). Environmental Project No. 357. In Danish. Danish Environmental Protection Agency.
Lassen, C., Drivsholm, T., Hansen, E., Rasmussen, B.& Christiansen, K. 1996a, Massestrømsanalyse for nikkel. Forbrug, bortskaffelse og udslip til omgivelserne i Danmark (mass flow analysis of
nickel, consumption, disposal, and emission to the environment in Denmark). Environmental project No. 318. In Danish. Danish Environmental Protection Agency.
Lassen, C., Drivsholm, T., Hansen, E., Rasmussen, B. & Christiansen, K. 1996b, Massestrømsanalyse for kobber. Forbrug, bortskaffelse og udslip til omgivelserne i Danmark (mass flow analysis of
copper, consumption, disposal, and emission to the environment in Denmark). Environmental project No. 323. In Danish. Danish Environmental Protection Agency.
Lassen, C. & Hansen, E. 1996, Massestrømsanalyse for bly. Forbrug, bortskaffelse og udslip til omgivelserne i Danmark (mass flow analysis of lead, consumption, disposal, and emission to the
environment in Denmark). Environmental Project No. 327. In Danish. Danish Environmental Protection Agency.
Madsen, T., Gustavson, K., Samsøe-Petersen, L., Simonsen, F., Jacobsen, J., Foverskov, S. & Mørk Larsen M. 1998, Kortlægning og vurdering af antibegroningsmidler til lystbåde i Danmark
(survey and assessment of antifoulants for pleasure craft in Denmark). Environmental project No. 384. In Danish. Danish Environmental Protection Agency.
OECD 1997, Compendium of environmental data. OECD Publications, Paris, France.
Tørsløv, J., Samsøe-Petersen, L., Rasmussen, J.O. & Kristensen, P. 1997, Use of waste products in agriculture. Contamination level, environmental risk assessment and recommendations for
quality criteria. Environmental project No. 366. Danish Environmental Protection Agency.
UBA (Umwelt Bundes Amt) 1999, Current publications. http:/www.umweltbundesamt.de.
van der Auweraert RJK, Berdowski JJM, Jonker WJ, Verhoeve P 1996, Emissies in Nederland Bedreijfgroepen en Regio's 1994 en Rammen 1995. Publicatiereeks Emissieregistratie Nr. 33.
`s-Gravenhage: Hoofdinspectie Milieuhygiëne /IPC 680.
Appendix A: Emissions to the aquatic enironment, metals
Austria
|
Emission (1) |
EF m3/g |
EP m3/yr |
EP m3/yr |
|
g/yr |
etwc |
etva |
etwc |
% |
etva |
% |
Hg |
3,86E+05 |
4,00E+03 |
2,00E+03 |
1,54E+09 |
0 |
7,72E+08 |
1 |
Cd |
1,10E+06 |
1,20E+05 |
1,20E+04 |
1,32E+11 |
11 |
1,32E+10 |
11 |
Cu |
4,91E+07 |
1,30E+04 |
1,30E+03 |
6,39E+11 |
52 |
6,39E+10 |
52 |
Zn |
2,96E+08 |
1,00E+03 |
1,00E+02 |
2,96E+11 |
24 |
2,96E+10 |
24 |
Pb |
7,53E+07 |
2,00E+03 |
2,00E+02 |
1,51E+11 |
12 |
1,51E+10 |
12 |
|
|
|
|
|
|
|
|
EP for metals (m3/yr) |
|
|
1,22E+12 |
|
1,23E+11 |
|
References
1) Based on van der Auweraert et al. (1996)
Belgium
|
Emission (1) |
EF m3/g |
EP m3/yr |
EP m3/yr |
|
g/yr |
etwc |
etva |
etwc |
% |
etva |
% |
Hg |
0,00E+00 |
4,00E+03 |
2,00E+03 |
0,00E+00 |
0 |
0,00E+00 |
0 |
Cd |
0,00E+00 |
1,20E+05 |
1,20E+04 |
0,00E+00 |
0 |
0,00E+00 |
0 |
Cu |
1,00E+06 |
1,30E+04 |
1,30E+03 |
1,30E+10 |
4 |
1,30E+09 |
4 |
Zn |
2,52E+08 |
1,00E+03 |
1,00E+02 |
2,52E+11 |
79 |
2,52E+10 |
79 |
Pb |
2,60E+07 |
2,00E+03 |
2,00E+02 |
5,20E+10 |
16 |
5,20E+09 |
16 |
|
|
|
|
|
|
|
|
EP for metals (m3/yr) |
|
|
3,17E+11 |
|
3,17E+10 |
|
References
1) Eurostat (1998)
Denmark
|
Emission |
EF m3/g |
EP m3/yr |
EP m3/yr |
|
g/yr |
etwc |
etva |
etwc |
% |
etva |
% |
Hg |
7,26E+05 |
4,00E+03 |
2,00E+03 |
2,90E+09 |
0 |
1,45E+09 |
2 |
Cd |
9,03E+05 |
1,20E+05 |
1,20E+04 |
1,08E+11 |
14 |
1,08E+10 |
14 |
Cu |
4,06E+07 |
1,30E+04 |
1,30E+03 |
5,28E+11 |
68 |
5,28E+10 |
67 |
Zn |
1,23E+08 |
1,00E+03 |
1,00E+02 |
1,23E+11 |
16 |
1,23E+10 |
16 |
Pb |
7,43E+06 |
2,00E+03 |
2,00E+02 |
1,49E+10 |
2 |
1,49E+09 |
2 |
|
|
|
|
|
|
|
|
EP for metals (m3/yr) |
|
|
7,77E+11 |
|
7,88E+10 |
|
References
Danish Environmental Protection Agency 1995
Danish Environmental Protection Agency 1996
Grüttner and Neergaard Jakobsen 1994
Grüttner et al. 1996
Jensen and Markussen 1993
Lassen and Hansen 1996
Lassen et al. 1996a
Lassen et al. 1996b
Finland
|
Emission |
EF m3/g |
EP m3/yr |
EP m3/yr |
|
g/yr |
etwc |
etva |
etwc |
% |
etva |
% |
Hg |
0,00E+00 |
4,00E+03 |
2,00E+03 |
0,00E+00 |
0 |
0,00E+00 |
0 |
Cd |
2,00E+06 |
1,20E+05 |
1,20E+04 |
2,40E+11 |
53 |
2,40E+10 |
53 |
Cu |
1,10E+07 |
1,30E+04 |
1,30E+03 |
1,43E+11 |
31 |
1,43E+10 |
31 |
Zn |
6,80E+07 |
1,00E+03 |
1,00E+02 |
6,80E+10 |
15 |
6,80E+09 |
15 |
Pb |
2,00E+06 |
2,00E+03 |
2,00E+02 |
4,00E+09 |
1 |
4,00E+08 |
1 |
|
|
|
|
|
|
|
|
EP for metals (m3/yr) |
|
|
4,55E+11 |
|
4,55E+10 |
|
References
1) Eurostat (1998)
France
|
Emission |
EF m3/g |
EP m3/yr |
EP m3/yr |
|
g/yr |
etwc |
etva |
etwc |
% |
etva |
% |
Hg |
0,00E+00 |
4,00E+03 |
2,00E+03 |
0,00E+00 |
0 |
0,00E+00 |
0 |
Cd |
1,00E+06 |
1,20E+05 |
1,20E+04 |
1,20E+11 |
14 |
1,20E+10 |
14 |
Cu |
4,70E+07 |
1,30E+04 |
1,30E+03 |
6,11E+11 |
73 |
6,11E+10 |
73 |
Zn |
2,20E+07 |
1,00E+03 |
1,00E+02 |
2,20E+10 |
3 |
2,20E+09 |
3 |
Pb |
4,00E+07 |
2,00E+03 |
2,00E+02 |
8,00E+10 |
10 |
8,00E+09 |
10 |
|
|
|
|
|
|
|
|
EP for metals (m3/yr) |
|
|
8,33E+11 |
|
8,33E+10 |
|
References
1) Eurostat (1998)
Germany
|
Emission |
EF m3/g |
EP m3/yr |
EP m3/yr |
|
g/yr |
etwc |
etva |
etwc |
% |
etva |
% |
Hg |
6,00E+06 |
4,00E+03 |
2,00E+03 |
2,40E+10 |
0 |
1,20E+10 |
2 |
Cd |
8,00E+06 |
1,20E+05 |
1,20E+04 |
9,60E+11 |
14 |
9,60E+10 |
14 |
Cu |
2,68E+08 |
1,30E+04 |
1,30E+03 |
3,48E+12 |
51 |
3,48E+11 |
50 |
Zn |
1,90E+09 |
1,00E+03 |
1,00E+02 |
1,90E+12 |
28 |
1,90E+11 |
27 |
Pb |
2,47E+08 |
2,00E+03 |
2,00E+02 |
4,94E+11 |
7 |
4,94E+10 |
7 |
|
|
|
|
|
|
|
|
EP for metals (m3/yr) |
|
|
6,86E+12 |
|
6,96E+11 |
|
References
1) Eurostat (1998)
Greece
|
Emission |
EF m3/g |
EP m3/yr |
EP m3/yr |
|
g/yr |
etwc |
etva |
etwc |
% |
etva |
% |
Hg |
1,52E+05 |
4,00E+03 |
2,00E+03 |
6,06E+08 |
0 |
3,03E+08 |
1 |
Cd |
4,32E+05 |
1,20E+05 |
1,20E+04 |
5,18E+10 |
11 |
5,18E+09 |
11 |
Cu |
1,93E+07 |
1,30E+04 |
1,30E+03 |
2,51E+11 |
52 |
2,51E+10 |
52 |
Zn |
1,16E+08 |
1,00E+03 |
1,00E+02 |
1,16E+11 |
24 |
1,16E+10 |
24 |
Pb |
2,96E+07 |
2,00E+03 |
2,00E+02 |
5,91E+10 |
12 |
5,91E+09 |
12 |
|
|
|
|
|
|
|
|
EP for metals (m3/yr) |
|
|
4,78E+11 |
|
4,81E+10 |
|
References
1) Based on van der Auweraert et al. (1996)
Ireland
|
Emission |
EF m3/g |
EP m3/yr |
EP m3/yr |
|
g/yr |
etwc |
etva |
etwc |
% |
etva |
% |
Hg |
|
4,00E+03 |
2,00E+03 |
0,00E+00 |
0 |
0,00E+00 |
0 |
Cd |
0,00E+00 |
1,20E+05 |
1,20E+04 |
0,00E+00 |
0 |
0,00E+00 |
0 |
Cu |
2,21E+08 |
1,30E+04 |
1,30E+03 |
2,87E+12 |
71 |
2,87E+11 |
71 |
Zn |
1,04E+09 |
1,00E+03 |
1,00E+02 |
1,04E+12 |
26 |
1,04E+11 |
26 |
Pb |
7,50E+07 |
2,00E+03 |
2,00E+02 |
1,50E+11 |
4 |
1,50E+10 |
4 |
|
|
|
|
|
|
|
|
EP for metals (m3/yr) |
|
|
4,07E+12 |
|
4,07E+11 |
|
References
1) Eurostat (1998)
Italy
|
Emission |
EF m3/g |
EP m3/yr |
EP m3/yr |
|
g/yr |
etwc |
etva |
etwc |
% |
etva |
% |
Hg |
2,00E+06 |
4,00E+03 |
2,00E+03 |
7,99E+09 |
0 |
4,00E+09 |
1 |
Cd |
5,69E+06 |
1,20E+05 |
1,20E+04 |
6,83E+11 |
11 |
6,83E+10 |
11 |
Cu |
2,54E+08 |
1,30E+04 |
1,30E+03 |
3,31E+12 |
52 |
3,31E+11 |
52 |
Zn |
1,53E+09 |
1,00E+03 |
1,00E+02 |
1,53E+12 |
24 |
1,53E+11 |
24 |
Pb |
3,90E+08 |
2,00E+03 |
2,00E+02 |
7,79E+11 |
12 |
7,79E+10 |
12 |
|
|
|
|
|
|
|
|
EP for metals (m3/yr) |
|
|
6,31E+12 |
|
6,34E+11 |
|
References
1) Based on van der Auweraert et al. (1996)
Luxemburg
|
Emission (1) |
EF m3/g |
EP m3/yr |
EP m3/yr |
|
g/yr |
etwc |
etva |
etwc |
% |
etva |
% |
Hg |
2,16E+04 |
4,00E+03 |
2,00E+03 |
8,66E+07 |
0 |
4,33E+07 |
1 |
Cd |
6,17E+04 |
1,20E+05 |
1,20E+04 |
7,40E+09 |
11 |
7,40E+08 |
11 |
Cu |
2,75E+06 |
1,30E+04 |
1,30E+03 |
3,58E+10 |
52 |
3,58E+09 |
52 |
Zn |
1,66E+07 |
1,00E+03 |
1,00E+02 |
1,66E+10 |
24 |
1,66E+09 |
24 |
Pb |
4,22E+06 |
2,00E+03 |
2,00E+02 |
8,44E+09 |
12 |
8,44E+08 |
12 |
|
|
|
|
|
|
|
|
EP for metals (m3/yr) |
|
|
6,83E+10 |
|
6,87E+09 |
|
References
1) Based on van der Auweraert et al. (1996)
Netherlands
|
Emission (1) |
EF m3/g |
EP m3/yr |
EP m3/yr |
|
g/yr |
etwc |
etva |
etwc |
% |
etva |
% |
Hg |
6,46E+05 |
4,00E+03 |
2,00E+03 |
2,58E+09 |
0 |
1,29E+09 |
1 |
Cd |
1,84E+06 |
1,20E+05 |
1,20E+04 |
2,21E+11 |
11 |
2,21E+10 |
11 |
Cu |
8,22E+07 |
1,30E+04 |
1,30E+03 |
1,07E+12 |
52 |
1,07E+11 |
52 |
Zn |
4,95E+08 |
1,00E+03 |
1,00E+02 |
4,95E+11 |
24 |
4,95E+10 |
24 |
Pb |
1,26E+08 |
2,00E+03 |
2,00E+02 |
2,52E+11 |
12 |
2,52E+10 |
12 |
|
|
|
|
|
|
|
|
EP for metals (m3/yr) |
|
|
2,04E+12 |
|
2,05E+11 |
|
References
1) Based on van der Auweraert et al. (1996)
Portugal
|
Emission |
EF m3/g |
EP m3/yr |
EP m3/yr |
|
g/yr |
etwc |
etva |
etwc |
% |
etva |
% |
Hg |
1,00E+06 |
4,00E+03 |
2,00E+03 |
4,00E+09 |
0 |
2,00E+09 |
1 |
Cd |
4,00E+06 |
1,20E+05 |
1,20E+04 |
4,80E+11 |
22 |
4,80E+10 |
22 |
Cu |
1,20E+08 |
1,30E+04 |
1,30E+03 |
1,56E+12 |
72 |
1,56E+11 |
71 |
Zn |
1,10E+08 |
1,00E+03 |
1,00E+02 |
1,10E+11 |
5 |
1,10E+10 |
5 |
Pb |
6,00E+06 |
2,00E+03 |
2,00E+02 |
1,20E+10 |
1 |
1,20E+09 |
1 |
|
|
|
|
|
|
|
|
EP for metals (m3/yr) |
|
|
2,17E+12 |
|
2,18E+11 |
|
References
1) Eurostat (1998)
Spain
|
Emission |
EF m3/g |
EP m3/yr |
EP m3/yr |
|
g/yr |
etwc |
etva |
etwc |
% |
etva |
% |
Hg |
|
4,00E+03 |
2,00E+03 |
0,00E+00 |
0 |
0,00E+00 |
0 |
Cd |
|
1,20E+05 |
1,20E+04 |
0,00E+00 |
0 |
0,00E+00 |
0 |
Cu |
2,20E+07 |
1,30E+04 |
1,30E+03 |
2,86E+11 |
74 |
2,86E+10 |
74 |
Zn |
9,70E+07 |
1,00E+03 |
1,00E+02 |
9,70E+10 |
25 |
9,70E+09 |
25 |
Pb |
2,00E+06 |
2,00E+03 |
2,00E+02 |
4,00E+09 |
1 |
4,00E+08 |
1 |
|
|
|
|
|
|
|
|
EP for metals (m3/yr) |
|
|
3,87E+11 |
|
3,87E+10 |
|
References
1) Eurostat (1998)
Sweden
|
Emission |
EF m3/g |
EP m3/yr |
EP m3/yr |
|
g/yr |
etwc |
etva |
etwc |
% |
etva |
% |
Hg |
0,00E+00 |
4,00E+03 |
2,00E+03 |
0,00E+00 |
0 |
0,00E+00 |
0 |
Cd |
0,00E+00 |
1,20E+05 |
1,20E+04 |
0,00E+00 |
0 |
0,00E+00 |
0 |
Cu |
3,90E+07 |
1,30E+04 |
1,30E+03 |
5,07E+11 |
71 |
5,07E+10 |
71 |
Zn |
1,83E+08 |
1,00E+03 |
1,00E+02 |
1,83E+11 |
26 |
1,83E+10 |
26 |
Pb |
1,10E+07 |
2,00E+03 |
2,00E+02 |
2,20E+10 |
3 |
2,20E+09 |
3 |
|
|
|
|
|
|
|
|
EP for metals (m3/yr) |
|
|
7,12E+11 |
|
7,12E+10 |
|
References
1) Eurostat (1998)
UK
|
Emission |
EF m3/g |
EP m3/yr |
EP m3/yr |
|
g/yr |
etwc |
etva |
etwc |
% |
etva |
% |
Hg |
2,00E+06 |
4,00E+03 |
2,00E+03 |
8,00E+09 |
0 |
4,00E+09 |
0 |
Cd |
1,50E+07 |
1,20E+05 |
1,20E+04 |
1,80E+12 |
12 |
1,80E+11 |
12 |
Cu |
6,78E+08 |
1,30E+04 |
1,30E+03 |
8,81E+12 |
59 |
8,81E+11 |
59 |
Zn |
3,35E+09 |
1,00E+03 |
1,00E+02 |
3,35E+12 |
22 |
3,35E+11 |
22 |
Pb |
4,97E+08 |
2,00E+03 |
2,00E+02 |
9,94E+11 |
7 |
9,94E+10 |
7 |
|
|
|
|
|
|
|
|
EP for metals (m3/yr) |
|
|
1,50E+13 |
|
1,50E+12 |
|
References
1) Eurostat (1998)
EU-15
|
Emission |
EF m3/g |
EP m3/yr |
EP m3/yr |
|
g/yr |
etwc |
etva |
etwc |
% |
etva |
% |
Hg |
1,29E+07 |
4,00E+03 |
2,00E+03 |
5,17E+10 |
0 |
2,59E+10 |
1 |
Cd |
4,00E+07 |
1,20E+05 |
1,20E+04 |
4,80E+12 |
12 |
4,80E+11 |
11 |
Cu |
1,86E+09 |
1,30E+04 |
1,30E+03 |
2,41E+13 |
58 |
2,41E+12 |
58 |
Zn |
9,60E+09 |
1,00E+03 |
1,00E+02 |
9,60E+12 |
23 |
9,60E+11 |
23 |
Pb |
1,54E+09 |
2,00E+03 |
2,00E+02 |
3,08E+12 |
7 |
3,08E+11 |
7 |
|
|
|
|
|
|
|
|
EP for metals (m3/yr) |
|
|
4,16E+13 |
|
4,19E+12 |
|
Appendix B.1 Emissions to the aquatic environment, organic substances - Denmark
Based on van der Auweraert et al. (1996)
Click here to see the Table.
Appendix B.2: Emissions to the aquatic environment, organic substances - EU-15
Based on van der Auweraert et al. (1996)
Click here to see the Table.
Appendix C: Emissions to the aquatic environment, atmospheric deposition
|
|
DK (80,20) |
|
EU-15 |
|
DK(80, 20) |
|
EU15 |
|
|
|
EP water |
% |
EP water |
% |
EP soil |
% |
EP soil |
% |
|
|
|
|
|
|
|
|
|
|
|
|
m3/yr |
|
m3/yr |
|
m3/yr |
|
m3/yr |
|
Substance |
Approx. |
|
|
|
|
|
|
|
|
Cd |
|
2,84E+10 |
21 |
2,50E+12 |
20 |
2,13E+06 |
4 |
1,87E+08 |
1 |
As |
|
2,82E+08 |
0 |
1,07E+11 |
1 |
2,00E+05 |
0 |
7,62E+07 |
0 |
Cr(VI) |
|
4,53E+08 |
0 |
7,35E+10 |
1 |
3,49E+04 |
0 |
5,66E+06 |
0 |
Hg |
|
3,03E+10 |
22 |
5,58E+11 |
4 |
4,02E+07 |
68 |
7,40E+08 |
2 |
Ni |
|
2,87E+09 |
2 |
2,72E+11 |
2 |
1,10E+06 |
2 |
1,05E+08 |
0 |
Pb |
|
1,58E+10 |
12 |
2,83E+12 |
23 |
3,95E+05 |
1 |
7,07E+07 |
0 |
Se |
|
5,28E+08 |
0 |
1,28E+12 |
10 |
1,40E+07 |
24 |
3,39E+10 |
96 |
Cu |
|
2,64E+10 |
19 |
1,40E+12 |
11 |
2,11E+05 |
0 |
1,12E+07 |
0 |
Zn |
|
2,37E+10 |
17 |
1,37E+12 |
11 |
5,91E+05 |
1 |
3,43E+07 |
0 |
Formaldehyde |
|
|
|
|
|
|
|
|
|
Benzene |
|
|
|
|
|
|
|
|
|
Phenol |
|
|
|
|
|
|
|
|
|
Styrene |
|
|
|
|
|
|
|
|
|
Toluene |
|
|
|
|
|
|
|
|
|
Xylenes |
|
|
|
|
|
|
|
|
|
PAH |
|
|
|
|
|
|
|
|
|
Fluoranthen |
0,05 |
|
|
|
|
|
|
|
|
Benzo(b)fluoranthen |
0,05 |
|
|
|
|
|
|
|
|
Benzo(k)fluoranthen |
0,05 |
|
|
|
|
|
|
|
|
Benzo(a)pyren |
1 |
|
|
|
|
|
|
|
|
Benzo(g,h,i)perylene |
0,02 |
|
|
|
|
|
|
|
|
Indino(1,2,3-c,d)pyren |
0,1 |
|
|
|
|
|
|
|
|
PAH-eq. (benzo(a)pyren) |
|
|
|
|
|
|
|
|
|
Dioxin |
|
7,84E+09 |
6 |
2,04E+12 |
16 |
1,68E+05 |
0 |
4,38E+07 |
0 |
PCP |
|
|
|
|
|
|
|
|
|
Hexachlorbenzene (HCB) |
|
|
|
|
|
|
|
|
|
Tetrachloromethane (TCM) |
|
|
|
|
|
|
|
|
|
Trichloroethylene (TRI) |
|
|
|
|
|
|
|
|
|
Tetrachloroethylene (PER) |
|
|
|
|
|
|
|
|
|
Trichlorobenzene (TCB) |
|
|
|
|
|
|
|
|
|
Trichloroethane (TCE) |
|
|
|
|
|
|
|
|
|
Hexachlorohexane (HCH) |
|
|
|
|
|
|
|
|
|
Chlorbenzenes |
|
|
|
|
|
|
|
|
|
Vinylchloride |
|
|
|
|
|
|
|
|
|
|
|
1,37E+11 |
|
1,24E+13 |
|
5,90E+07 |
|
3,51E+10 |
|
Appendix D: Emissions to the terrestrial environment, pesticides
Equivalency factor based on the principles used in Hauschild et al. (1998)
Click here to see the Table.
Appendix E.1: Emissions to the terrestrial environment, sewage sludge applied in agriculture - Denmark
Production in 1994 (3) |
On soil |
On soil |
|
|
|
Ton dry weight /yr |
% |
Tonnes/yr |
|
|
|
231000 |
56 |
129360 |
|
|
|
Substance |
Concentration |
Emission |
EF (1) |
EP |
EP% |
|
mg/kg dw |
g/yr |
etsc m3/g |
etsc m3/yr |
|
Acenaphthene |
0,0364 |
4709 |
|
0 |
0 |
Fluorene |
0,0707 |
9146 |
|
0 |
0 |
Phenanthrene |
0,3066 |
39662 |
|
0 |
0 |
Fluoranthene |
0,32289 |
41769 |
|
0 |
0 |
Pyrene |
0,3095 |
40037 |
|
0 |
0 |
Benzofluoranthene |
0,298 |
38549 |
|
0 |
0 |
Benzo(a)pyrene |
0,15239 |
19713 |
|
0 |
0 |
Benzo(ghi)perylene |
0,1177 |
15226 |
|
0 |
0 |
Indeno(1,2,3-cd)pyrene |
0,067 |
8667 |
|
0 |
0 |
Sum PAH (2) |
1,68118 |
217477 |
1,0 |
217477 |
1 |
PCB 28 |
0,0044 |
569 |
|
0 |
0 |
PCB 52 |
0,00901 |
1166 |
|
0 |
0 |
PCB 101 |
0,00768 |
993 |
|
0 |
0 |
PCB 118 |
0,00575 |
744 |
|
0 |
0 |
PCB 138 |
0,00708 |
916 |
|
0 |
0 |
PCB 153 |
0,00864 |
1118 |
|
0 |
0 |
PCB 180 |
0,00577 |
746 |
|
0 |
0 |
Sum PCB |
0,04833 |
6252 |
100,0 |
625197 |
3 |
Butylbenzylphthalate |
0,1751 |
22651 |
|
0 |
0 |
Chloroform |
0,00081 |
105 |
25,0 |
2620 |
0 |
Di(ethyhexyl)phthalate |
37,86 |
4897570 |
|
0 |
0 |
Dichlorobenezene |
0,01091 |
1411 |
0,5 |
692 |
0 |
Di-n-butylphthalate |
3,8832 |
502331 |
|
0 |
0 |
Di-n-octylphthalate |
0,60785 |
78631 |
|
0 |
0 |
Nonylphenol + 1-2EO |
15,176 |
1963167 |
|
0 |
0 |
tetrachloroethylene |
0,01759 |
2275 |
1,1 |
2503 |
0 |
Trichlorothylene |
0,0029 |
375 |
|
0 |
0 |
LAS (2) |
2667 |
345003120 |
0,04 |
13800125 |
73 |
Toluene |
0,02069 |
2676 |
1,0 |
2596 |
0 |
Dioxine (2,3,7,8-TCDD) |
1,00E-05 |
1 |
15000,0 |
19404 |
0 |
As |
3,71 |
479926 |
0,3 |
158375 |
1 |
Cd |
1,67 |
216031 |
2,2 |
475269 |
3 |
Cr |
35,42 |
4581931 |
0,01 |
45819 |
0 |
Cu |
287,34 |
37170302 |
0,02 |
743406 |
4 |
Pb |
72,8 |
9417408 |
0,01 |
94174 |
0 |
Hg |
2,4 |
310464 |
5,3 |
1645459 |
9 |
Ni |
29,78 |
3852341 |
0,1 |
269664 |
1 |
Zn |
887,37 |
114790183 |
0,01 |
803531 |
4 |
|
|
|
|
|
|
Sum |
|
|
|
1,89E+07 |
|
References
1. Hauschild & Wenzel (1998).
2. DEPA (1995).
3. DEPA (1998).
Appendix E.2: Emissions to the terrestrial environment, sewage sludge applied in agriculture - EU-15
Production in 1994 (3,4) |
On soil |
On soil |
|
|
|
Ton dry weight /yr |
% |
Tonnes/yr |
|
|
|
12497276 |
29 |
3661415 |
|
|
|
Substance |
Concentration |
Emission |
EF (1) |
EP |
EP% |
|
mg/kg dw |
g/yr |
etsc m3/g |
etsc m3/yr |
|
Acenaphthene |
0,0364 |
133276 |
|
0 |
0 |
Fluorene |
0,0707 |
258862 |
|
0 |
0 |
Phenanthrene |
0,3066 |
1122590 |
|
0 |
0 |
Fluoranthene |
0,32289 |
1182234 |
|
0 |
0 |
Pyrene |
0,3095 |
1133208 |
|
0 |
0 |
Benzofluoranthene |
0,298 |
1091102 |
|
0 |
0 |
Benzo(a)pyrene |
0,15239 |
557963 |
|
0 |
0 |
Benzo(ghi)perylene |
0,1177 |
430949 |
|
0 |
0 |
Indeno(1,2,3-cd)pyrene |
0,067 |
245315 |
|
0 |
0 |
Sum PAH (2) |
1,68118 |
6155498 |
1,0 |
6155498 |
1 |
PCB 28 |
0,0044 |
16110 |
|
0 |
0 |
PCB 52 |
0,00901 |
32989 |
|
0 |
0 |
PCB 101 |
0,00768 |
28120 |
|
0 |
0 |
PCB 118 |
0,00575 |
21053 |
|
0 |
0 |
PCB 138 |
0,00708 |
25923 |
|
0 |
0 |
PCB 153 |
0,00864 |
31635 |
|
0 |
0 |
PCB 180 |
0,00577 |
21126 |
|
0 |
0 |
Sum PCB |
0,04833 |
176956 |
100,0 |
17695619 |
3 |
Butylbenzylphthalate |
0,1751 |
641114 |
|
0 |
0 |
Chloroform |
0,00081 |
2966 |
25,0 |
74144 |
0 |
Di(ethyhexyl)phthalate |
37,86 |
138621176 |
|
0 |
0 |
Dichlorobenezene |
0,01091 |
39946 |
0,5 |
19574 |
0 |
Di-n-butylphthalate |
3,8832 |
14218007 |
|
0 |
0 |
Di-n-octylphthalate |
0,60785 |
2225591 |
|
0 |
0 |
Nonylphenol + 1-2EO |
15,176 |
55565636 |
|
0 |
0 |
tetrachloroethylene |
0,01759 |
64404 |
1,1 |
70845 |
0 |
Trichlorothylene |
0,0029 |
10618 |
|
0 |
0 |
LAS (2) |
2667 |
9764994125 |
0,04 |
390599765 |
73 |
Toluene |
0,02069 |
75755 |
1,0 |
73482 |
0 |
Dioxine (2,3,7,8-TCDD) |
1,00E-05 |
37 |
15000,0 |
549212 |
0 |
|
|
|
|
|
|
As |
3,71 |
13583850 |
0,3 |
4482671 |
1 |
Cd |
1,67 |
6114563 |
2,2 |
13452039 |
3 |
Cr |
35,42 |
129687324 |
0,01 |
1296873 |
0 |
Cu |
287,34 |
1052071021 |
0,02 |
21041420 |
4 |
Pb |
72,8 |
266551021 |
0,01 |
2665510 |
0 |
Hg |
2,4 |
8787396 |
5,3 |
46573200 |
9 |
Ni |
29,78 |
109036942 |
0,1 |
7632586 |
1 |
Zn |
887,37 |
3249029935 |
0,01 |
22743210 |
4 |
|
|
|
|
|
|
SUM |
|
|
|
5,35E+08 |
|
References
Hauschild et al. (1998)
DEPA (1995).
DEPA (1998).
11 Calculation of weighting factors
Niels Juul Busch, Rambøll
11.1 Summary
The aim of this chapter has been to calculate new weighting factors for a broad range of impact categories for Denmark, European and global level. The method applied for calculating the weighting factors is
based on the environmental regulation of substances, impact categories etc. Hence, it is the same methodology as the one used in the EDIP study (Hauschild & Wenzel, 1998).
However, weighting factors based on regulation that will lead to full phase out of the substances within the target period will tend to result in very high numbers or infinite. This is in particular the case for
Ozone Depletion. In these cases the weighting factors are indicated by the sign for infinity ().
Table 11-1 summarises the calculated new weighting factors for the period 1994-2004 and the similar weighting factors calculated in the EDIP study for the period 1990-2000.
Table 11-1
Summary of calculated weighted factors (WF) and comparison with EDIP factors. WFs at global scale have only been calculated for Global Warming, Ozone depletion and Photochemical Ozone, due to the
fact that regulation of the other effect categories have not been implemented at global level.
Impact category |
New weighting factors
1994 - 2004
|
EDIP weighting factors,
1990 – 2000
|
Global Warming, Denmark |
1.12 |
1.3 |
Global Warming, EU |
1.05 |
- |
Global Warming, World, Industrialised countries |
1.11 |
- |
Ozone Depletion, Denmark |
|
23 |
Ozone Depletion, EU |
2.46 |
- |
Ozone Depletion, World, Industrialised Countries |
63 |
- |
Ozone Depletion, World, Develop. Countries |
4.4 |
- |
Photochemical Ozone, Denmark |
1.26 |
1.2 |
Photochemical Ozone, EU |
1.33 |
- |
Photochemical Ozone, World |
1.00 |
- |
Acidification, Denmark |
1.34 |
1.3 |
Acidification, EU |
1.27 |
- |
Nutrient enrichment, Denmark |
1.31 |
1.2 |
Nutrient enrichment, EU |
1.22 |
- |
Ecotoxicity, Denmark, Aquatic chronic |
1.67 |
2.6 |
Ecotoxicity, Denmark, Aquatic acute |
1.73 |
2.6 |
Ecotoxicity, Denmark, Terrestrial |
1.56 |
1.9 |
Ecotoxicity, EU, Aquatic chronic |
1.18 |
- |
Ecotoxicity, EU, Aquatic acute |
1.11 |
- |
Ecotoxicity, EU, Terrestrial |
1.00 |
- |
Human toxicity, Denmark, Air |
1.11 |
1.1 |
Human toxicity, Denmark, Water |
1.02 |
2.9 |
Human toxicity, Denmark, Soil |
1.00 |
2.7 |
Human toxicity, EU, Air |
1.06 |
- |
Human toxicity, EU, Water |
1.30 |
- |
Human toxicity, EU, Soil |
1.23 |
- |
It should be emphasised that there is not much regulation on global level of the impact categories addressed in EDIP, except for global warming, ozone depletion and to a limited extent - photochemical
ozone. Calculation of a weighting factor thus requires a close examination of reduction targets in all regions of the World - a task that is outside the scope of the present study. Accordingly, global weighting
factors have only been calculated for the impact categories that are global in their scale.
Furthermore, it should be mentioned that EU-regulation of substances that contribute to, in particular the ecotoxicity and human toxicity impact categories, is very rudimentary. The reason for this is that EU
regulation can be seen as supplementary to national regulations. A precise weighting factor for EU thus requires that all national regulations and reduction targets are examined in sufficient detail. It has been
tried to obtain this information from the national ministries of environment, but only very scattered information was found. The EU weighting factors for human toxicity and ecotoxicity are therefore most
probably underestimated and should be used with some reservations.
11.2 Definition of "weighting" procedures
According to the ISO standard, ISO 14042 on Life Cycle Impact Assessment (LCIA) "weighting" is defined in the following way (ISO 2000):
"Weighting is conversion of indicator results or normalised results by using numerical factors. Weighting is an optional element with two possible procedures:
- To convert the indicator results or normalised results with selected factors
- To possible aggregate these converted indicator results or normalised result across impact categories, i.e. scoring.
The application and use of weighting methods shall be consistent with the goal and scope of the LCA study and be fully transparent. Different people, organisations and societies may have different
values; therefore it is possible that different parties will reach different weighting results based on the same indicator results. In the LCA study it may be desirable to use different weighting methods and
to conduct sensitivity analysis to assess the consequences on the life cycle impact assessment results of different value-choices and weighting methods.
All weighting methods and operations used shall be documented to provide transparency. Data and Life Cycle Impact Assessment results reached prior to weighting should be made available together
with the weighting results. This ensures that:
- Trade-offs and other information remain available to decision makers and others, and
- Users can appreciate the full extent and ramification of the results."
11.3 Scope of weighting procedure
Before choosing a weighting method and procedure to be applied for a certain LCA, it is important to take a number of different aspects into consideration:
- Does the method comply with the objectives of the LCA?
- Is the method developed to a stage, which makes it applicable to the LCA?
- Will sufficient and reliable data be available for calculating the weighting factors?
11.4 Different weighting methods
A number of different methods have been discussed at national and international level, e.g.:
- Expert based/quality based weighting systems.
- Weighting systems based on an eco-scarcity/carrying capacity approach.
- Weighting system based on political priorities within different impact categories.
These methods have been developed to different levels and have different advantages and disadvantages.
In this project - as in the Original EDIP97 - it has been decided to apply a weighting method based on "political targets and goals" established for the selected impact categories. This is due to the relatively
advanced state of the method and the availability of sufficient and reliable data and information for most impact categories.
For all impact categories, regardless of their geographical scale, the weighting factors are based on politically determined reduction targets in Denmark, the EU or at the global level. These reduction targets
are taken as an expression of the importance assigned to the impact category in those parts of the world. If important stakeholders for a study come from different parts of the world and it can be expected
that they will have environmental priorities that differ significantly from the priorities expressed through these reduction targets, it may be relevant to derive weighting factors more in line with these priorities
e.g. by basing them on political reduction targets in the important stakeholders' part of the world.
11.4.1 Weighting procedure based on political goals
The procedure for applying and using weighting in this project consist of the following steps:
- Determination of actual emission in reference year
- Determination of targeted emissions in target year
- Calculation of weighting factor.
In this project 1994 has been chosen as "reference year" and 2004 as "target year". The impact potential is either regulated directly by the political priorities through legislation, actions plans etc. or indirectly
through regulation of the emission of the substances that determines the impact category.
For each impact category x, the weighting factor (WF) is thus defined as follows:
s248
As the actual political targets for reducing certain pollutants in the different countries seldom are related to the target year selected for this project, it has been necessary to interpolate or extrapolate the data,
so that the weighting factors for all impact categories relate to year 2004.
11.4.2 Environmental impact categories
Weighting factors have been calculated for the following impact categories, corresponding to those categories for which normalisation references have been calculated in the previous chapters:
- Global warming
- Stratospheric ozone depletion
- Photochemical ozone formation
- Acidification
- Nutrient enrichment
- Ecotoxicity
- Human toxicity.
11.4.3 Geographic scope
In this project it has been decided to establish weighting factors for the following geographical areas:
- Denmark
- European Union (EU-15)
- The World
For Denmark the calculation of weighting factors will be an update of the existing weighting factors developed through the EDIP project (Hauschild & Wenzel, 1998). Calculation of weighting
factors for EU is a natural extension of the weighting factors for Denmark, which make the method valid for a larger market, e.g. Europe. It should be mentioned that weighting factors have not been
calculated for each of the individual EU member states for the following reasons:
- The environmental regulation differs from country to country.
- The environmental legislation of the 15 member states is being harmonised, and should in the long run refer to the same standards.
This means that the weighting factors developed for EU only represent a part of the environmental targets valid for the individual countries, namely that part for which common EU goals have been adopted.
It has been suggested to make this method applicable at worldwide level. However, this is only possible for few impact categories, as worldwide regulation only exist for global warming, ozone depleting
substances, and substances contributing to formation of photochemical ozone.
11.4.4 Calculation of weighting factors
In the following the weighting factors for the individual impact categories have been calculated, together with brief explanation of the data on which the weighting factors are based.
11.5 Global warming
At the global level the greenhouse gasses are regulated according to the Kyoto Protocol (UN 1997), except for those greenhouse gasses that are regulated through other international agreements.
In principle the Kyoto Protocol regulates the following greenhouse gasses:
- Carbon dioxide (CO2)
- Methane (CH4)
- Nitrous oxide (N2O)
- Hydrofluorocarbons (HFC's)
- Perfluorocarbons (PFC's)
- Sulphur hexafluoride (SF6)
Ozone Depleting Substances (ODS) are global warming gasses as well, but as they are regulated according to the Montreal Protocol (UNEP 1987), they are not regulated by The Kyoto Protocol.
However, as the ODS are contributing to the global warming the consumption of the substances – which is considered corresponding to the emission and thereby the impact on the global warming the
equivalent data of the consumption is included where appropriate. Detailed information on the regulation of ODS's according to the Montreal Protocol is dealt with in section 11.6
At the regional level the greenhouse gasses are regulated according to different agreements. Some of the gasses, VOC's and CO, are regulated according to the Convention on Long Range
Transboundary Air Pollution (UN-ECE 1979), while others are regulated according to EU resolutions.
At the national level in Denmark the greenhouse gasses were earlier regulated according to various ministerial executive regulations and the governmental action plan, Energy 21 (Energistyrelsen 1996), but
now (May 2002) as the Kyoto Protocol has been ratified by countries accounting in total for nearly 55% of the total carbon dioxide emissions for 1990, which is the criteria for implementation of the
Protocol, this will be the guiding regulation for Denmark as well.
11.5.1 Weighting factors for global warming at global level
According to the Kyoto Protocol (UN 1997) the Annex-1 parties (industrialised countries) shall reduce their anthropogenic carbon dioxide equivalent emissions with at least 5% below 1990 level in the
period 2008 to 2012. It is further stated that each of the Annex-1 parties shall have made demonstrable progress in achieving its commitments under the Protocol by 2005.
For most of the Annex-1 parties, including EU as a whole, the demand is to reduce the emission by 8%. Some individual countries have targeted a reduction by up to 28%, others have to reduce emission by
6-7%, and some countries will even be allowed to increase their emission of greenhouse gasses up to a level of 10% more than the baseline level in 1990.
According to the reporting by the parties to the Kyoto Protocol the "aggregated CO2 equivalent emissions, including CO2, CH4, N2O, HFCs, PFCs and SF6 emissions" from the Annex-1 parties in
1994 is reported to be 16.837 billion tonnes CO2-eq (UN/FCCC 2003, (On-line searchable database of GHG inventory data). With a reduction target for 2008-2012 (2010) on 5% (or 2.5% for the
present reference period) the interpolated emission for 2004 should be 16.416 billion tonnes CO2-eq.
Although USA and the Russian Federation have yet not ratified the Kyoto Protocol, their emissions of global warming gases are included in these calculations.
The total emission of global warming gases (excluding ODS) for both Article 1- and Non Article 1 countries in 1994 has been estimated to 19.548 billion tonnes CO2-eq (UN/FCCC 2003).
It should be noticed that the Kyoto Protocol until now only regulates the greenhouse gas emissions from industrialised countries and that there are different reduction targets for the individual industrialised
countries at both regional and national level.
The ozone depleting substances are divided into different chemical groups (CFC's, Halons, Methyl chloroform and HCFC's) that have different impact on the ozone layer as well as on the global warming.
The regulation of the substances differs for the different groups of substances and for the two different categories of countries ("Article 5 countries", being the developing countries and the "Non Article 5
countries" being the industrialised countries, including countries under economic transition). In Table 11-2 below the background data for calculating the CO2-eq of the ozone depleting substances have been
summarised.
Table 11-2
Calculated CO2-eq for ozone depleting substances, global level.
Article 5 parties, 1994 |
Consumption, metric tonnes 1994 |
GWP 100 |
Tonnes CO2-eq, 1994 |
Reduction target,
1996-2005
|
Emission 2004, Tonnes CO2-eq |
A I CFC-11 |
67,382 |
4600 |
309,957,200 |
50% |
154,978,600 |
A I CFC-12 |
97,726 |
10600 |
1,035,895,600 |
50% |
517,947,800 |
A I CFC-113 |
9,828 |
6000 |
58,968,000 |
50% |
29,484,000 |
A I CFC-114 |
528 |
9800 |
5,174,400 |
50% |
2,587,200 |
A I CFC-115 |
919 |
7200 |
6,616,800 |
50% |
3,308,400 |
A II HALON-1211 |
8,054 |
6900 |
55,572,600 |
50% |
27,786,300 |
A II HALON-1301 |
865 |
1300 |
1,124,500 |
50% |
562,250 |
A II HALON-2402 |
6 |
6900 |
41,400 |
50% |
20,700 |
B II Carbon Tetrachloride |
145,514 |
1800 |
|
|
|
B III Methyl Chloroform |
35,655 |
140 |
4,991,700 |
30% |
3,494,190 |
C I HCFC-21 |
13 |
? |
|
|
|
C I HCFC-22 |
77,134 |
1700 |
131,127,800 |
0% |
|
C I HCFC-123 |
460 |
120 |
55,200 |
0% |
|
C I HCFC-124 |
746 |
620 |
462,520 |
0% |
|
C I HCFC-141 |
4,281 |
700 |
2,996,700 |
0% |
|
C I HCFC-142 |
242 |
2400 |
580,800 |
0% |
|
C I HCFC-225 |
527 |
400 |
210,800 |
0% |
|
E I Methyl Bromide |
13,121 |
5 |
65,605 |
20% |
52,484 |
Total |
|
|
1,613,841,625 |
|
740,221,924 |
Non-article 5 parties, 1994 |
Consumtion, metric tonnes 1994 |
GWP 100 |
Tonnes CO2-eq, 1994 |
Reduction target, 1996-2005 |
Emission 2004, Tonnes CO2-eq |
A I: CFC-11 |
47,479 |
4600 |
218,403,400 |
100% |
0 |
A I: CFC-12 |
117,948 |
10600 |
1,250,248,800 |
100% |
0 |
A I: CFC-113 |
76,752 |
6000 |
460,512,000 |
100% |
0 |
A I: CFC-114 |
30,032 |
9800 |
294,313,600 |
100% |
0 |
A I: CFC-115 |
7,415 |
7200 |
53,388,000 |
100% |
0 |
A II: HALON-1211 |
145 |
6900 |
1,000,500 |
100% |
0 |
A II: HALON-1301 |
25 |
1300 |
32,500 |
100% |
0 |
A II: HALON-2402 |
225 |
6900 |
1,552,500 |
100% |
0 |
B II: Carbon Tetrachloride |
319,309 |
1800 |
|
|
|
B III: Methyl Chloroform |
246,884 |
140 |
34,563,760 |
100% |
0 |
C I: HCFC-21 |
322 |
? |
|
35% |
|
C I: HCFC-22 |
291,804 |
1700 |
496,066,800 |
35% |
322,443,420 |
C I: HCFC-123 |
1,824 |
120 |
218,880 |
35% |
142,272 |
C I: HCFC-124 |
2,025 |
620 |
1,255,500 |
35% |
816,075 |
C I: HCFC-141 |
67,940 |
700 |
47,558,000 |
35% |
30,912,700 |
C I: HCFC-142 |
45,208 |
2400 |
108,499,200 |
35% |
70,524,480 |
C I: HCFC-225 |
0 |
400 |
0 |
35% |
0 |
E I: Methyl Bromide |
28,306 |
5 |
141,530 |
100% |
0 |
Total |
|
|
2,967,754,970 |
|
424,838,947 |
Carbon tetrachloride is not included in the calculations as it is primarily used as feedstock for other ODS substances.
Table 11-3 below summarises the calculations of global warming gases, ODS as well as non-ODS, divided on the Article 1 and Non Article 1 countries.
Table 11-3
Summary of calculation of emissions of global warming gases
|
1994 emissions, tonnes CO2-eq |
2004 emissions |
Article 1 countries, GWG |
16,836,999,000 |
16,416,074,025 |
Article 1 countries, ODS |
2,967,754,970 |
424,838,947 |
Non Article 1 countries, GWG |
2,711,370,000 |
2,711,370,000 |
Non Article 1 countries, ODS |
1,613,841,625 |
740,221,924 |
Total Global |
22,516,123,970 |
20,292,504,896 |
Based on the combined figures for CO2, CH4, N2O, HFCs, PFCs and SF6 and the ozone depleting substances accounted for in Table11-2 the weighting factor for global warming at global level will be:

11.5.2 Weighting factors for global warming at EU level
During the negotiations of the Kyoto Protocol the EU proposed more drastic reductions of the greenhouse gasses within its own geographical area than was finally agreed upon for the Annex-1 parties in
general. Hence, in its implementation of the regulation of the greenhouse gasses the Environment Ministers Council of EU has agreed upon the following reduction targets for the individual EU countries (EU
1998):
- Luxembourg 28%
- Germany 21%
- Denmark 21%
- Austria 13%
- UK 12.5%
- Belgium 7.5%
- Italy 6.5%
- Netherlands 6%
- France 0%
- Finland 0%
- Portugal -27%
- Greece -25%
- Spain -15%
- Ireland -13%
- Sweden -4%
As it appears from the table the five last mentioned countries are allowed to increase their CO2-eq emission. For the EU as a whole the reduction target will be 8% in 2010 based on the 1990 emission level.
According to the reporting to the Kyoto Protocol the "aggregated CO2 equivalent emissions" in 1994 the emission is reported to 3.972 billion tonnes CO2-eq (UN/FCCC 2003). With a reduction target
for 2008-2012 (2010) on 8% (4% for the reference period) the interpolated emission for 2004 should be about 3.813 billion tonnes CO2-eq.
Among the ozone depleting substances only HCFC and methyl chloroform is relevant for EU. The remaining groups of ozone depleting substances are supposed to be phased out so quickly that they are not
included in the calculation. In Table 11-4 below the background data for calculating the CO2-eq of the global warming and the ozone depleting substances have been summarised.
Table 11-4
Calculated CO2-eq for ozone depleting substances as well as other green house gases (GHG) for EU.
|
Methyl chloroform |
HCFC |
GHG |
Total |
EU Consumption, 1994, tonnes |
46 000 |
73 000 |
|
|
Emission 1994, in
million CO2-eq
|
5 |
73 |
3972 |
4050 |
Reduction target, base & target year |
-100%
1996
|
-35%
1991 / 2005
|
4% |
|
Emission 2004, in million CO2-eq |
0 |
28 |
3813 |
3841 |
Based on these figures the weighting factor for GWP at EU level will be:

This is a general weighting factor for EU as whole, but as it appears from the table above different targets have been set for the individual countries.
11.5.3 Weighting factors for global warming for Denmark
As mentioned earlier, the EDIP97 weighting factors for all impact categories are based on political reduction targets for Denmark or Europe regardless the geographical scale of the impact. The Danish
regulation of greenhouse gasses is based on the EU regulation.
According to the Kyoto Protocol and the internal EU agreement the aggregated CO2 emission should be reduced with 21% by 2010, based on 1990 emission level.
According to the reporting to the Kyoto Protocol the "aggregated CO2 equivalent emissions" in 1994 the emission is reported to 80.152 million tonnes CO2-eq (UN/FCCC 1998). With a reduction target
for 2008-2012 (2010) on 21% the interpolated emission for 2004 should be about 71.736 million tonnes CO2-eq.
According to the Danish rules for ODS phase out all the ODS should be fully phased out before 2004. There are special rules for the HFCs, which are supposed to be phased out by 2007.
The calculation of the global warming potential of the ODS and HFCs appear from Table 2-1 below.
Table 11-5.
Calculated CO2-eq for ozone depleting substances for Denmark.
DK |
GWP 100 |
Emission 1994, 1000 tonnes |
GWP, 1994 tonnes CO2-eq |
Reduction targets, % |
2004 emission |
CFC-11 |
4600 |
0.055 |
253 |
100 |
0 |
CFC-12 |
10600 |
0.24 |
2544 |
100 |
0 |
CFC-113 |
6000 |
0.065 |
390 |
100 |
0 |
CFC-115 |
9800 |
0.025 |
245 |
100 |
0 |
HCFC-22 |
7200 |
0.75 |
5400 |
100 |
0 |
HCFC-141b |
700 |
0.51 |
357 |
100 |
0 |
HCFC-142b |
2400 |
0.145 |
348 |
100 |
0 |
HFC-134a |
1300 |
0.52 |
676 |
77 |
155.48 |
HFC-152a |
120 |
0.05 |
6 |
77 |
1.38 |
Halon 1301 |
6900 |
0.005 |
34.5 |
100 |
|
Halon 1211 |
1300 |
0 |
0 |
100 |
0 |
CH3CCl3 |
140 |
0,57 |
79.8 |
100 |
0 |
CH3Br |
5 |
0.012 |
0.06 |
100 |
0 |
Total |
|
2.947 |
10333.36 |
|
156.86 |
Based on these figures the weighting factor for global warming for Denmark will be:
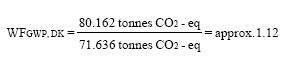
It should be emphasised that ozone depleting substances should have been included in the above calculation, just like for the other geographical areas. However, as the ozone depleting substances have been
phased out in Denmark during the target period, it would add an infinitive value to the above weighting factor, totally overruling the result of the phasing out of green house gases. Hence, it has not been
included in these calculations.
11.5.4 Comparison of existing and new weighting factors
Table 11-6 summarises the calculated weighting factors for the different groups of GWP, divided on three geographical areas. The table also includes the general GWP weighting factors for Denmark,
developed through the EDIP project.
Table 11-6
Weighting factors for Global Warming (GW).
Geographical scope |
WF (GW) |
Denmark |
1.12 |
EU (including ODS) |
1.05 |
World, Industrialised countries (including ODS) |
1.11 |
Denmark (including ODS 1990-2000) (Hauschild & Wenzel 1996a) |
1,3 |
Denmark (including ODS, 1990-2000) (Hauschild & Wenzel 1998b) |
1,2 |
As it appears the weighting factors for Global Warming are relatively low, indicating a long-term phase down of the emissions of green house gases. The relatively lower WF for Denmark in the period
1994-2004, compared to the one calculated for 1990-2000, is due to the fact that ODS are not included in the new WF which was the case in the earlier calculations (EDIP, 1990-2000).
This impact category includes relatively few substances, and the calculation of the emission of the dominating substances is well documented. Furthermore, the impact is relatively well researched. Hence,
the uncertainty of the calculated weighting factors must be considered as limited compared to some of the other weighting factors.
11.6 Stratospheric ozone depletion
The depletion of the stratospheric ozone layer is regulated through reducing the production and consumption of the ozone depleting substances (ODS).
This means that the regulation works indirectly in relation to the actual effect. For many of the applications of ODS there will be a considerable time span between the "production/consumption" of the
substances, the emission of the substances to the atmosphere as well as between the emission and the actual impact on the ozone layer. A time span on 10-20 years between consumption and impact is not
unusual.
However, it is not possible to regulate the ozone depletion directly, so this indirect regulation is the only practical option.
Almost all countries in the world have become parties of the Montreal Protocol (UNEP 1987), which is the international agreement to protect the stratospheric ozone layer. Those countries which have
not become parties to the Montreal Protocol are few and represent only a very limited consumption of ODS.
This means that the regulation prescribed by the Montreal Protocol represent the basic regulation to phase out ODS in most countries. The regulation in the Montreal Protocol is divided on two different
categories of countries:
- Article 5 countries: Any Party that is a developing country and whose annual calculated level of consumption of the controlled substances in Annex A is less than 0.3 kilograms per capita
- Non-Article 5 countries: All parties to the Montreal Protocol, except those which have got special exceptions (see below). The Non-Article 5 countries include the industrialised countries.
Apart from the overall regulation based on The Montreal Protocol a number of countries have adopted their own regulations, which are stricter than the Montreal Protocol. Especially the industrialised
countries have adopted stricter regulation, while the east and central European as well as most developing countries have adopted a regulation close to the one prescribed in the Montreal Protocol.
In the EU a general regulation has been adopted which has to be followed by the 15 members states, although these countries can adopt their own stricter regulation.
11.6.1 Weighting factors for Ozone Depletion for Denmark
Denmark has adopted its own regulation, which is stricter than the EU regulation. The Danish regulation consists of the following steps (Miljøstyrelsen 1999):
- Phase out of CFC's by 1995
- Phase out of halons by 1993
- Phase out of methyl bromide by 1998
- Phase out of HCFC's by 2002
- Phase out of carbon tetrachloride by 1992
- Phase out of 1,1,1-trichloroethane by 1996
As it appears from the above list most of the ODS should have been phased out by now, and this is also more or less the case. Some substances are still in use, and recycling is allowed under certain
conditions. The use of HCFC's is allowed until 2002, and hence by 2004, they have to be fully phased out.
This means that by 2004 all substances controlled according to the Montreal Protocol have to be phased out in Denmark. Hence, the weighting factors for the ozone depleting substances, calculated strictly
in line with the formula, will be infinite:
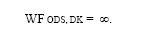
An infinite weighting factor will obviously also cause the weighted result to become infinite. This is a strong signal that the product system being investigated contributes to an environmental impact where
Danish regulation actually aims at giving no contribution and that some kind of action is strongly requested. The global weighting factor, which is generally recommended for ozone depletion, may give a more
realistic view of the importance with the current situation for phasing-out of ozone depleting substances. The difference, however, underlines the importance of using more than one weighting factor in a
sensitivity analysis.
11.6.2 Weighting factors for Ozone Depletion at EU level
The overall regulation of ODS in EU is formulated in the Council Regulation (EC) No 3093/94 of 15 December 1994 on substances that deplete the ozone layer (Hansen, 1997). The phase out schedule
for the various controlled substances can be summarised as follows:
- Phase out of CFC's by 1 January 1995
- Phase out of halons by 1 January 1994
- Reduce 1991 methyl bromide consumption with 25% by 1 January 1999
- Reduce 1991 consumption of HCFC's with 35% by 1 January 2005
- Phase out of carbon tetrachloride by 1 January 1995
- Phase out of 1,1,1-trichlorethan by 1 January 1996
On 21 December 1998 the Environment Ministers Council of EU reached political agreement on a proposal forwarded by the Commission to tighten the phase out schedule for some of the ODS's
(EU 1994):
- Phase out of methyl bromide by 2001, with exemption for critical uses
- Phase out of almost all HCFC's by 1 January 2003
If the proposed amendment of the EU regulation on ODS is finally adopted almost all ODS will be phased out before 2004, and the weighting factor will be infinite ().
However, if the previously mentioned phase out plan is applied, the WF can be calculated as follows.
The ozone depleting potential for emitted HCFCs in EU 15 in 1991 can be estimated to 2,850 tonnes ODP, based on emission data for 1989 (1,900 tonnes ODP) and 1992 (3,330 tonnes ODP). The
emission in 1994 is reported to be 5,577 tonnes ODP (UNEP, 1999). For 1995 the emission is reported to 7,631 tonnes ODP. A 35%'s reduction in the 1991 consumption would lead to a maximum
emission in 2005 of 1,853 tonnes ODP. By interpolating the emission in 2004 should not exceed 2,264 tonnes ODP.
Based on these figures the weighting factor for ODS for EU will be:
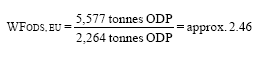
11.6.3 Weighting factors for Ozone Depletion at global level for industrialised countries
As mentioned above, the Montreal Protocol represents the regulation of ODS at global level. The regulation is divided into two categories of countries, industrialised countries (called Non-Article 5
countries in the following) and developing countries (called Article 5 countries in the following).
The regulation for Non-Article 5 countries can be summarised as follows (UNEP 1987):
- Phase out of CFC's by 1 January 1996
- Phase out of halons by 1 January 1994
- Phase out of methyl bromide by 1 January 1996
- Reduce 1989 HCFC consumption with 35% by 1 January 2004, full phase out by 2020 (except 0.5%)
- Phase out of carbon tetrachloride by 1 January 1996
- Phase out of 1,1,1-trichlorethane (methyl chloroform) by 1 January 1996
As it appears from the above list all the controlled ODS's, except HCFC's, will be phased out before 2004. This is similar to the regulation at Danish and EU level.
11.6.3.1 Weighting factor based on ODSs
The calculation of the weighting factor for ozone depletion is based on the actual consumption of all ODS's in 1994 and the allowed consumption in 2004 that according to the regulation should not exceed
65% of the 1989 consumption of HCFC.
The 1989 consumption of HCFC is reported to 11,978 ODP tonnes, and the 1994 consumption of all ODS for the whole world is estimated to 490,099 ODP tonnes of (UNEP, 2002). According to the
Montreal Protocol the HCFC consumption should in 2004 not exceed 7,786 ODP tonnes. Based on these figures the weighting factor for ozone depletion for Non-Article 5 countries will be:
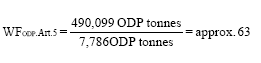
11.6.4 Weighting factors for Ozone Depletion at global level for non-industrialised countries
The phase out scheme for Article 5 countries can be summarised as follows (UNEP 1987):
- Reduce 1995-97 CFC consumption with 50% by 1 January 2005, full phase out by 2010
- Reduce 1995-97 halons consumption with 50% by 1 January 2005
- Reduce 1995-98 methyl bromide consumption with 20% by 1 January 2005, full phase out by 1 January 2015
- Freeze 2015 HCFC consumption by 1 January 2016, full phase out by 2040
- Reduce 1998-2000 carbon tetrachloride consumption with 85% by 1 January 2005, full phase out by 2010
- Reduce 1998-2000 methyl chloroform consumption with 30% by 1 January 2005, full phase out by 2015
As it appears from the above none of the ODS's will be fully phased out in the Article 5 countries by 2004. Some reduction targets have been established for most of the substances for this or the following
year.
The base line year for the first three mentioned groups of substances is set to 1996, for which data is available. There are no data available for the baseline year for the other substances. However, these
substances are not that relevant, except HCFC's, as the consumption of them is limited.
In the following the data for each of the ODS groups are presented, and in the end of the section these data are summarised and weighting factors are calculated.
11.6.4.1 CFC's
The 1996 consumption of CFC's for Article 5 countries is estimated to 149,401 ODP tonnes (UNEP, 2002). The 2005 consumption should according to the Montreal Protocol be reduced to 74,700
ODP tonnes.
11.6.4.2 Halons
Similarly, the 1996 consumption of halons is estimated to 43,410 ODP tonnes (UNEP, 2002). The 2005 consumption should according to the Montreal Protocol be reduced to 21,705 ODP tonnes.
11.6.4.3 Methyl bromide
The 1995 and 1998 consumption of methyl bromide for Article 5 countries is estimated to 8,578 ODP tonnes, respectively 10,564 ODP tonnes (UNEP, 2002). The 2005 consumption should according to
the Montreal Protocol be reduced to 7,657 ODP tonnes.
11.6.4.4 HCFC's
The reduction target for HCFC's in the Article 5 countries need to be measured in relation to the full phase out year 2040, as no earlier target can be determined. This means that a possible HCFC
consumption for 2004 has to be interpolated based on the 1994 consumption and the full phase out in 2040.
According to the UNEP statistics (UNEP, 2002) the 1994 HCFC's consumption was 4,348 ODP tonnes. It should be mentioned that this statistics is based on reporting from the parties to the Montreal
Protocol, and that for 1994 obviously there is a considerable lack of data. It is furthermore obvious that the HCFC consumption will increase in the following years. However, if the presented figures are
taken for granted the 2004 consumption will be around 3,391 ODP tonnes.
11.6.4.5 Carbon tetrachloride
The reduction target for carbon tetrachloride in the Article 5 countries is measured for 2005, for which the intermediate target is to reduce the 1999 consumption with 85%.
According to the UNEP statistics (UNEP, 2002) the 1999 carbon tetrachloride consumption was 22,099 ODP tonnes. The target consumption in 2004 will thus be 3,315 ODP tonnes
11.6.4.6 1,1,1-trichloroethane
The reduction target for 1,1,1-trichloroethane in the Article 5 countries is measured in relation to the intermediate target, i.e. a 30% reduction in 2005 in relation to the 1999 consumption.
The 1994 and 1999 consumption was 3,510 and 1,951 ODP tonnes, respectively (UNEP, 2002), and accordingly the target consumption for 2002/2005 is calculated to 1,366 ODP tonnes.
Table 11-7
Summary of targeted (2004) consumption of Ozone Depletion Substances at global level for developing countries (Article 5 countries).
Group of ODS |
Targeted consumption in 2004,
tonnes ODP
|
CFCs |
47,700 |
Halons |
21,705 |
Methyl bromide |
7,657 |
HCFCs |
3,391 |
Carbon tetrachloride |
3,315 |
Methyl chloroform |
1,366 |
Total |
112,133 |
Based on the above figures, the weighting factor for ozone depletion for Article 5 countries can be calculated as:
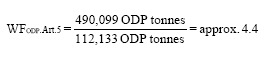
11.6.5 Comparison of existing and new weighting factors
Table 11-8 below summarises the calculated weighting factors for ozone depletion, divided into four geographical areas. The table also includes the ODP weighting factors for Denmark developed through
the EDIP project.
Table 11-8
Weighting factors for ozone depleting substances.
|
Total ODS |
Denmark |
|
EU |
2.46 |
World, Industrialised countries |
63 |
World, developing countries |
4.4 |
Denmark/World (WF for all ODS's, 1990-2000) (Hauschild &
Wenzel 1996e) |
23 |
Denmark/World (WF for all ODS's, 1990-2000) (Hauschild &
Wenzel 1998e) |
23 |
As it can be seen the weighting factors for ODS are in general high as was the case in the earlier studies.
The WF for Denmark is now estimated to be infinite, because all ODS have to be phased out within the period 1994-2004. The WF for EU 15 is moderate, because most of the ODS already are phased
out in 1994, and EU will still be allowed to emit some ODS by 2004. The WF for industrialised countries is relatively high because they in total still had a high emission of ODS in 1994, while being
supposed to phase out most of the emission before 2004. The WF for developing countries is low because they have been allowed to phase out the ODS over a longer period.
The differences in ODP weighting factors for different regions will often be reflected in the final results in an LCA if a sensitivity analysis is applied. As it is not possible in the current EDIP methodology to use
different and region-specific weighting factors in the same calculations, such a sensitivity analysis can show the weight that are put on emissions of ozone depleting substances in specific regions, allowing a
better interpretation. Identification of ozone depleting substances in any LCA should, however, always be followed by considerations on how they can be avoided.
The data on consumption of ODS is relatively well documented (based on reporting from the individual countries and cross examined with total production figures), and the uncertainty connected with the
weighting factors for this impact category must be considered as limited.
11.7 Photochemical ozone creation (POC)
The principal precursors of tropospheric ozone are:
- CH4
- nmVOC (non methane VOC)
- CO
VOC are regulated at regional level, according to the Convention on Long Range Transboundary Air Pollution (UN-ECE 1979). In some countries these substances are also regulated at national level.
CH4 is regulated as greenhouse gas at both global and regional (EU) level, but not yet at national level in Denmark (1999). CO is regulated at point sources, but it is not regulated as POCP-gas. Hence,
there are no limits for total emission of CO at neither national, nor regional and global level.
11.7.1 Weighting factors for Photochemical Ozone Creation at global level
The only POC gas that is regulated at global level is CH4. According to the Kyoto Protocol the emission of green house gases should be reduced by 5% in 2008-2012, based on the 1990 emission level.
Reduction levels for the different gases are not specified further, so in the following calculation it is assumed that CH4 has to follow the general rule.
The emission of CH4 in 1990 is estimated to 351 million tonnes. For 1994 the emission is reported to 375 million tonnes, equal to 2.625 million tonnes C2H4-equivalents. According to the regulation the
emission in 2010 should not exceed 333 million tonnes. By interpolating the emission in 2004 should not exceed 349 million tonnes, equal to 2.443 million tonnes C2H4-equivalents.
According to the technical report (Stranddorf, 2003), the World-wide emissions of gases with a photochemical ozone creating potential (POCP) equalled 76.8 million tonnes C2H4-equivalents in 1994.
Except for the reduction in emissions of CH4 outlined above, the emissions are assumed to be the same in 2004.
Based on these figures the weighting factor for POCP at global level will be:

11.7.2 Weighting factors for POCP at regional level
At the regional level the principal POCP substances are regulated according to the protocols under the Convention on Long Range Transboundary Air Pollution (UN-ECE 1979).
Emission of nmVOC is regulated according to the Geneva Protocol (UN-ECE, 1991) that was adopted in 1991. According to the Protocol the emission of VOCs should be reduced with 30% in 1999,
with a year between 1984 and 1990 as a base.
As mentioned earlier, CH4 is regulated according to the Kyoto Protocol with 5% over the period 1990-2010. CO is neither regulated at national nor regional level.
The data on which the calculation of the WF for POCP is based appears from Table 11-9 below.
Table 11-9.
Data for calculation of WF for POCP for EU-15.
Substance |
Impact potential 1994
(kt C2H4/year)
|
Reduction target, % |
Reduction period |
Targeted level in 2004 (kt C2H4/year) |
nmVOC |
7,769 |
30 |
1987-1999 |
5,438 |
CO |
1,381 |
0 |
|
1,381 |
CH4 |
158 |
5 |
1990-2010 |
153 |
Total |
9,307 |
|
|
6,972 |
Based on these figures the weighting factor for photochemical ozone formation for EU-15 will be:

11.7.3 Weighting factors for POCP for Denmark
The Danish regulation concerning photochemical ozone formation is following the regional regulation. This means that nmVOC is following the Geneva Protocol and CH4 the Kyoto Protocol. The emission
of CO is not regulated at national level. The weighting factor for POCP at national Danish level can then be calculated as follows shown in Table 11-10.
Table 11-10
Data for calculation of WF for POCP for Denmark.
Substance |
Impact potential 1994
(kt C2H4/year)
|
Reduction target, % |
Reduction period |
Targeted level in 2004 (kt C2H4/year) |
nmVOC |
72.1 |
30 |
1987-1999 |
50.47 |
CO |
28.6 |
0 |
|
28.6 |
CH4 |
3.0 |
5 |
1990-2010 |
2.925 |
Total |
103.7 |
|
|
81.995 |
Based on these figures the weighting factor for photochemical ozone formation for Denmark will be:

11.7.4 Comparison of existing and new weighting factors
Table 11-11 summarises the weighting factors calculated for photochemical ozone formation for the three geographical areas, and compares them to the previously developed weighting factors in the EDIP
project.
Table 11-11
Weighting factors for photochemical ozone formation (POCP).
Area |
This project |
EDIP (Hauschild & Wenzel 1996c) |
EDIP (Hauschild & Wenzel 1998d) |
Global |
1.002 |
|
|
EU |
1.33 |
- |
- |
Denmark |
1.26 |
|
1.2 |
As it appears from the table, the weighting factor for POCP for Denmark is somewhat higher than it was in the EDIP project. This is due to the fact that the reduction targets for CH4 according to the Kyoto
Protocol is included in these calculation, which was not the case for the original EDIP. The regulation of the POPC at global level is only determined by the regulation of CH4 through the Kyoto Protocol,
and this regulation is not so strict as the other regulation.
The uncertainty connected with the calculation of the weighting factors for POCP may be higher than that of Global Warming and Ozone Depletion. The reason for this is that the regulation of the involved
substances refers to different international agreements and that it is difficult to estimate the emission data precisely.
11.8 Acidification
Acidification is primarily a regional environmental problem that is regulated both at local, national and regional level. The overall reduction of substances that lead to acidification is based on the Convention
on Long Range Transboundary Air Pollution (LRTAP) (UN-ECE 1979), which is ratified by most of the countries in Europe and North America.
The regulation of acidification is focusing on two groups of substances, sulphur dioxide (SO2) and nitrogen oxides (NOx) each regulated according to their own protocols. NH3 is not regulated as an air
pollutant but as a water pollutant.
11.8.1 Weighting factors for acidification at global level
There is no binding regulation at global level, but as the primary industrial nations have adopted the LRTAP Convention, a weighting factor based on this can to a certain degree be applied as global weighting
factor. However, this will require a closer investigation of how the LRTAP convention is implemented in the various regions, and that is not done in this study.
11.8.2 Weighting factors for acidification at EU level
SO2 was one of the first substances to be regulated at international level. This was done through the LRTAP Convention which later on was replaced by the so-called the Helsinki Protocol (Helsinki
Commission 1974) regulating the emission of SO2 until 1993.
The Helsinki Protocol was replaced in 1994 by the Oslo Protocol, or the Second Sulphur Protocol (UN-ECE 1994). According to this protocol the parties have agreed to reduce their sulphur emissions
in accordance with the plan shown in Table 11-12. The baseline year is 1980.
Table 11-12
SO2 emission reductions according to Oslo Protocol (UN-ECE 1994).
Parties |
Emission level, 1980,
kt SO2 |
Reduction commitment according to Oslo Protocol
Target year |
|
|
2000 |
2005 |
2010 |
Austria |
397 |
80 |
|
|
Belgium |
828 |
70 |
72 |
74 |
Denmark |
451 |
80 |
|
|
Finland |
584 |
80 |
|
|
France |
3348 |
74 |
77 |
78 |
Germany |
7494 |
83 |
87 |
|
Greece |
400 |
-49 |
-45 |
-43 |
Ireland |
222 |
30 |
|
|
Italy |
3800 |
65 |
73 |
|
Luxembourg |
24 |
58 |
|
|
Netherlands |
466 |
77 |
|
|
Portugal |
266 |
0 |
3 |
|
Spain |
3319 |
35 |
|
|
Sweden |
507 |
80 |
|
|
UK |
4898 |
50 |
70 |
80 |
The following calculation of weighting factor is based on the reduction targets for 2005. For those countries, which only have set targets for 2000, these reduction figures are used instead. Where emission
figures are missing for the reference year (1994), figures for a year earlier are used instead.
Based on the figures from the UN-ECE reports (UN-ECE 1994) the total 1994 emission of SO2 in the countries is 12,105 kilotonnes SO2. The calculated emission for 2004 should according to the
reduction targets be 8,04 kilotonnes SO2
The earlier regulation of NOx, the Sofia Protocol (UN-ECE 1988a), will not effect the weighting factor for this project, as the targets have been reached several years ago. However, the Sofia Protocol
was in 1988 supplemented by a declaration (UN-ECE 1988b) adopted by 12 European countries (Austria, Belgium, Denmark, Germany, Finland, France, Italy, Liechtenstein, Netherlands, Norway,
Sweden and Switzerland).
The reduction target of the declaration is formulated as follows "the signatories will implement a reduction of national annual nitrogen oxide emission in the order of 30% as soon as possible and at the latest
by 1998, using the level of any year between 1980 and 1986 as a basis".
For the EU countries the declaration will result in reductions shown in Table 11-13. In the calculation 1983 has been used as basis for the calculations for all the countries.
Table 11-13
Reduction of NOx according to the UN-ECE Declaration of 1988 (UN-ECE 1988b.).
Parties |
Emission 1983,
>kt NOx |
Emission 1994
kt NOx |
Commitment according to UN-ECE NOx Declaration |
Target 1998, -30% |
Target 2004,-30% |
Austria |
241 |
177 |
|
|
Belgium |
442 |
345 |
|
|
Denmark |
261 |
272 |
|
|
Finland |
261 |
282 |
|
|
France |
1645 |
1682 |
|
|
Germany |
3158 |
2210 |
|
|
Italy |
1568 |
1997 |
|
|
Netherlands |
555 |
542 |
|
|
Sweden |
401 |
372 |
|
|
Total |
8532 |
7879 |
5972 |
5972 |
SO2-eq, total |
5972 |
5515 |
4181 |
4181 |
The above data diverts a bit from those used for the calculations in the normalisation chapters. However, as the above data are those used in relation to the negotiation of the regulation, they are kept here for
the calculation of the weighting factor.
For the remaining six countries that is not regulated with respect to NOx the following total emission for the reference year and the target year are applied (derived from the calculation of the normalisation
references): 1380 kt SO2-eq.
The emission of NOx for EU-15 in 1994 is thus calculated to 6895 kt SO2-eq and for 2004 5561 kt SO2-eq.
There exist no regional regulation of the third acidifying component, ammonia (NH3), so the emission figure remain the same in the reference year as in the target year: 6640 kt SO2-eq.
The figures for acidification for EU-15 are summarised in Table 11-14 below.
Table 11-14
Total Acidification in kt SO2-eq
Substance |
1994 |
2004 |
SO2 |
12105 |
8040 |
NOx |
6895 |
5561 |
NH3 |
6640 |
6640 |
Total |
25640 |
20241 |
Based on these figures the weighting factor for acidification for EU-15 will be:
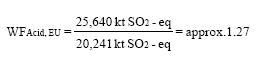
A new regulation, integrating all the primary acidifying substances, is under way but no specific targets have been established so far.
11.8.3 Weighting factors for acidification for Denmark
With regard to acidification Denmark is following the Protocols under the Convention on Long Range Transboundary Air Pollution.
According to the Oslo Protocol (UN-ECE 1994) Denmark has to reduce the SO2 emission with 80% in 2000 relative to the 1980 emission.
The 1980 and the 1994 SO2 emissions were 451,000 tonnes and 158,000 tonnes. The 2000 emission thus has to be 90,200 tonnes SO2. As there have not been established new targets, the level in 2004
is considered to be equal to the one in 2000.
According to a UN-ECE Declaration on NOx, adopted under the Sofia Protocol, the Danish emission of NOx has to be reduced with 30% in 1998 relative to the 1983 emission (or rather a year between
1980-1986).
The 1983 and the 1994 NOx emissions has been registered to 261,000 tonnes and 276,000 tonnes. The 1998 emission thus has to be 182,700 tonnes NOx. As there have not been established new targets,
the level in 2004 is considered to be equal to the one in 1998. Converted into SO2 equivalents the NOx emission in 1994 and 2004 will be 193,200, respectively 127,900 tonnes SO2-eq.
Airborne ammonia (NH3), which primarily originates in the agricultural sector, contributes to the acidification as well. It not regulated, but its contribution to acidification has to be included in the calculations.
It is estimated that the annual emission amounts to about 93 600 tonnes of nitrogen (Andersen, et al 1999). Converted into SO2-eq the emission will be 176 000 tonnes per year (equivalency factor is 1.88
(EDIP 97)).
The figures for acidification for Denmark is summarised in Table 11-15 below.
Table 11-15
Total Acidification for Denmark in kt SO2-eq
|
1994 |
2004 |
SO2 |
158 |
90,2 |
NOx |
193,2 |
127,89 |
NH3 |
175,968 |
175,968 |
Total |
527,168 |
394,058 |
Based on these figures the weighting factor for acidification will be:

11.8.4 Comparison of existing and new weighting factors
Table 11-16 summarises the weighting factors calculated for acidification for the three geographical areas, and compares them to the previously developed weighting factors in the EDIP project.
Table 11-16
Weighting factors for acidification.
Area |
This project |
EDIP (Hauschild & Wenzel 1996b) |
EDIP (Hauschild & Wenzel 1998a) |
EU |
1.27 |
- |
- |
Denmark |
1.34 |
|
1.3 |
As it appears the WF for Denmark in this study is more or less the same as the one calculated in the EDIP study, while the WF for EU is somewhat lower. It should be emphasised, however, that the target
year differs between the various countries within EU.
The uncertainty connected with the calculation of the weighting factors for acidification is judged to be at the same level as that of Global Warming and Ozone Depletion. The reason for this is that the
regulation of the involved substances is relative clear and that the emission data is relatively well documented. It is however also mentioned here that the target year for reduction for some countries is 2000
instead of 2004, adding a minor uncertainty to the weighting factor.
11.9 Nutrient enrichment
There exist both regional (EU) and national regulation of some of the substances that result in nutrient enrichment, primarily N containing substances.
The calculation of the weighting factors is based on reduction targets for the consumption and use of the two plant nutrients, N and P. EDIP 97 includes calculations of WFs for both N and P as well as an
aggregated WF for N and P. However, as there is no current regulation of P in Denmark anymore and there have never been for EU, no new WF including P has been calculated in this study.
11.9.1 Weighting factors for nutrient enrichment for Denmark
The Danish emission of N and P to the water environment has primarily been regulated through the Water Environment Action Plans. The first plan, which established reduction targets for three sectors,
covers in principle all emissions to the water environment, except precipitation from the air.
As the goals of the first Water Environment Action plan were not met, a second Action Plan (Miljøstyrelsen, 1998) was adopted in 1998 extending the period for reaching the goals. The reduction
targets are based on the data from the NPO Study, which contains the baseline data for the emission of N and P. The new target year is 2003. In Table 11-17 the reduction targets established through the
Action Plans are summarised.
Table 11-17
Reduction targets for N and P in Denmark.
Sector |
Baseline emission, 1985
1000 tonnes |
Reduction target, 2003
1000 tonnes or % |
Target met |
Nitrogen |
Agriculture |
260 |
100 tonnes |
No |
Waste water treatment plants |
25 |
15% |
Yes |
Industry |
5 |
3% |
Yes |
Phosphorous |
Agriculture |
4.4 |
n.a. |
No |
Waste water treatment plants |
7.2 |
n.a. |
Yes |
Industry |
3.4 |
n.a. |
Yes |
The only target, which is still relevant, is the reduction of the emission of N from the agriculture. The target on reduction of the emission of P from the agriculture has not been achieved, and the target has now
been given up.
So far, there has been no reduction target for the emission of NH3 but such a target is under way.
As the reduction target for N from the agriculture was not achieved during the first action plan the emission level is considered to be the same in 1994, 260,000 tonnes N. In 2004 the emission has to be
reduced to 160,000 tonnes N, as this is target already for 2003.
The reduction targets for airborne NOx is, as mentioned under Acidification, section 11.8, 30% in 1998, based on the emission level in 1983. As there have not been established new reduction targets
for airborne NOx, the 30% will also be valid for 2004. This means that the NOx emissions in the reference and targets years are 261,000 tonnes NOx (1994) and 182,700 tonnes NOx (2004).
Airborne ammonia (NH3), contributes to the nutrient enrichment as well, and has to be included in the calculations. It is assumed that the emission is more or less constant and that the annual emission
amounts to about 93,000 tonnes of nitrogen (Andersen, et al 1999).
The emission of P is assumed to be the same in both reference and the target year, and amounts to a total of 15,000 tonnes P per year. Converted into NO3-eq the amount will be 480,450 tonnes NO3-eq.
The above data and conversion of them into NO3--eq is shown in Table 11-18 below (conversion factors as in EDIP).
Table 11-18
Summary of N- and P-contribution to nutrient enrichment (tonnes)
|
Year |
N contribution |
NOx contribution |
NH3 contribution |
P contribution |
Total |
Specific substance |
1994 |
260000 |
261000 |
93000 |
15000 |
|
Specific substance |
2004 |
160000 |
182799 |
93000 |
15000 |
|
Characterisation factor |
|
4.43 |
1.35 |
3.64 |
32.03 |
|
NO3--eq |
1994 |
1151800 |
352350 |
338520 |
480450 |
2 323 120 |
NO3--eq |
2004 |
708800 |
246779 |
338520 |
480450 |
1 774 549 |
Based on the above figures the weighting factor for nutrient enrichment with N-containing substances for Denmark will be:

The weighting factor for nutrient enrichment with P-containing substances for Denmark can be estimated to:
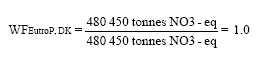
The aggregated weighting factor for nutrient enrichment for Denmark will be:
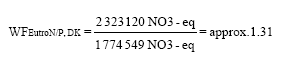
11.9.2 Weighting factors for nutrient enrichment at EU level
Within EU nutrient enrichment is regulated mainly through three directives:
- Urban Waste Water Treatment Directive (1991) (EU 1991a)
- The Nitrate Directive (1991) (EU 1991b)
- The IPPC directive (Integrated Pollution Prevention and Control Directive) (1996) (EU 1996)
According to the Urban Waste Water Treatment Directive (EU 1991a) the discharge of P via urban waste water treatment plants to "sensitive areas" shall be reduced by minimum 80% or concentration of
Total P should be kept within 2 mg/l for 10 000 - 100 000 p.e. (person equivalents) plants and within 1 mg/l for plants over 100 000 p.e. Similarly, Total N has to be reduced with minimum 70-80%, or
concentrations of 15 mg/l for 10 000 – 100 000 p.e. plants and 10 mg/l for plants over 100 000 p.e.
There are no exact reduction targets for discharge of treated wastewater to normal and less sensitive areas. The discharge of wastewater is divided on the three categories of recipients, see Table 11-19.
Table 11-19
Discharge of waste water to different areas according to EU regulation
Recipient waters |
Discharge of waste water million PE |
Sensitive areas |
158 (37%) |
Normal areas |
229 (54%) |
Less sensitive areas |
37 (9%) |
Total |
424 |
The Urban Waste Water Treatment Directive was originally adopted in 1991, and has at a later stage been revised to the above mentioned version. Unfortunately, it has not been possible to provide baseline
data, which the targets can be related to, so it has not been possible to include emission from urban areas in the calculation of weighting factors.
According to the Nitrates Directive (EU 1991b), adopted in 1991, the distribution of nitrogen containing manure on agricultural land should be reduced within certain time limits. In 1998 up to 210 kg N can
be distributed per hectare, and in 2002 the limit is 170 kg N per hectare.
The Directive does not include further reduction of the distribution of nitrogen. Hence, it is stipulated that the limit on 170 kg N per hectare for 2002 is also valid for 2004. There are no limits for
phosphorous. The IPPC Directive (EU 1996) set up procedures for regulating the emission and effluents from industries, but does not include specific values for emission of N and P to waterways.
Through extrapolation the reference amount of N in 1994 is 237 kg N per hectare. Based on these assumptions the weighting factor for N will be:

It should be emphasised that this weighting factor is based on only one basic factor in the creation of nutrient enrichment. Hence, the scope of the estimation of the weighting factor is relatively narrow in
relation to the biological mechanism of nutrient enrichment.
Due to the fact that phosphorous is not regulated at EU-level the weighting factor for P is one:
WFEutroP, EU = 1.0
If it is assumed that the emission of the nutrient enrichment substances is linear to the use of the N and P as well as the air emissions an aggregated weighting factor can be calculated as follows.
In connection with the calculation of the normalisation references the following emissions for 1994 have been estimated as it appears from Table 11-20 below.
Table 11-20.
EU-15 emissions of nutrients in the form of nitrogen and phosphorus compounds to air and water. The unit is kt/year. Emissions to air from Ritter (1997). Waterborne emissions from EEA (1998a).
Substance |
Impact potential
1994 |
Emissions in reference year |
Emissions in target year |
kt N-eq./
year |
kt P-eq./
year |
1994 |
2004 |
Airborne emissions |
Nitrogen oxides |
3763 |
0 |
3763 |
3763 |
Ammonia |
2898 |
0 |
2898 |
2898 |
Waterborne emissions |
Total P |
0.0 |
150 |
150 |
150 |
Total N |
2342 |
0.0 |
2342 |
662 |
Total |
9004 |
150 |
9155 |
7474 |
Based on these assumptions the aggregated weighting factor for nutrient enrichment with N and P will be:

11.9.3 Weighting factors for nutrient enrichment at global level
There are no initiatives to establish reduction targets for nutrient enrichment substances at global level. Hence, it has not been tried to develop weighting factors for nutrient enrichment at this level.
11.9.4 Comparison of existing and new weighting factors
Table 11-21 summarises the weighting factors calculated for nutrient enrichment for the two geographical areas, and compares them to the previously developed weighting factors in the EDIP project.
Table 11-21
Weighting factors for nutrient enrichment.
Area |
This project |
EDIP (Hauschild & Wenzel 1998c) |
EU, N |
1.39 |
- |
Denmark, N |
1.42 |
1.3 |
EU, P |
1.0 |
- |
Denmark, P |
1.0 |
1.0 |
EU, aggregated |
1.22 |
- |
Denmark, aggregated |
1.31 |
1.2 |
The uncertainty connected with the calculation of the weighting factors for nutrient enrichment is judged to be at a higher level than that of Global Warming and Ozone Depletion. The reason for this is that the
regulation of the involved substances only covers part of the impact category and that the impact mechanism and the emission data is less well documented.
11.10 Ecotoxicity
The overall impact category ecotoxicity is a relatively complex impact category, including a broad range of substances and recipients. In this project it has been decided to focus on the substances that have
been identified as the most important for three specific "sub"-impact categories, as mentioned in the section Normalisation References for the Impact Category Ecotoxicity. The groups of substances and the
"sub"-impact categories are summarised in Table 11-22.
Table 11-22
Groups of substances and "sub"-impact categories for Ecotoxicity
|
Group of substances |
Ecotox potential, water, acute |
Ecotox potential, water, chronic |
Ecotox potential, soil, chronic |
Waste water |
Organic substances |
X |
X |
|
Waste water |
Metals |
X |
X |
|
|
|
|
|
|
Pesticides |
Fungicides |
|
|
X |
Pesticides |
Herbicides |
|
|
X |
Pesticides |
Insecticides |
|
|
X |
|
|
|
|
|
Sludge |
Organic substances + metals |
|
|
X |
|
|
|
|
|
Oil emission |
|
X |
X |
|
|
|
|
|
|
Deposition, water |
|
X |
|
|
|
|
|
|
|
Deposition, soil |
|
|
|
X |
|
|
|
|
|
Antifouling |
Organotin compounds |
X |
X |
|
The substances mentioned in Table11-22 is applied for both Denmark and EU, except "Oil emission", which is assumed relevant only at EU level.
11.10.1 Weighting factors for ecotoxicity for Denmark
11.10.1.1 Organic substances and metals to water
The emission of organic substances and metals to water, which in this context means marine areas, is primarily regulated through two international conventions, the Convention on the Protection of the
Marine Environment of the Baltic Sea (The Helsinki Convention) (Helsinki Commission 1974) and the OSPAR Convention for the North Atlantic Oceans (OSPAR Commission 1992),
including the North Sea. The OSPAR Convention replaces the earlier adopted Oslo and the Paris conventions.
According to the OSPAR Convention the emission of hazardous substances to the oceans should be reduced with 50% in relation to the emissions in 1985. The list of substances includes those, which are
mentioned under the previous sections on Normalisation References for Ecotoxicity, e.g. metals, organics, pesticides and organotin compounds. The target year was earlier 1995, but has now been
prolonged to 2000.
Initiatives have been taken to formulate a full stop for emission of selected substances before 2020. However, this initiative is still under consideration, so no specific target can be established by now.
Therefore, the target for 2004 in this context is considered as being 50% of the 1985 emissions, which corresponds to a reduction of about 20% from 1994 to 2004.
11.10.1.2 Pesticides to soil
The outlet of pesticides to the soil is primarily regulated through the Action Plan for Reduction of the Consumption of Pesticides, 1986 (Miljøstyrelsen 1986). According to this action plan the consumption
should be reduced with 25% in relation to the average annual consumption in the years 1981-85. Later on it has been decided to reduce the consumption with 25% more by 1997.
A further reduction, or even a full phase out of the use of pesticides, is under consideration in the Committee for Evaluation of Phasing Out the Use of Pesticides. The recommendation of this Committee
has not been discussed by the political institutions, so it is too early to take it for granted that there will be a full or almost full phase out of pesticides. However, it seems evident that a decision on further
reduction will take place within the coming years.
Therefore, the calculation of the reduction targets for pesticides will be based on the assumption that the current trend in the phasing out will continue anyway until 2004. This means that the consumption of
pesticides should be reduced with 75% in 2004 in relation to the consumption in 1983, which correspond to an average annual reduction of about 3.57% in the period, or 36% in the period from 1994 to
2004.
11.10.1.3 Organic substances and metals in sludge
The outlet of organic substances and metals to the soil, via sludge from wastewater treatment plants, is regulated according to the executive regulation for the Application of Waste Products for Agricultural
Purposes, issued 1996 (Miljø- og Energiministeriet 1996).
According to this executive regulation the content of one heavy metal and some organic substances (detergents) has to be reduced within a given period, see Table 11-23 below.
Table 11-23
Reduction targets for metals and organic substances in sludge.
Regulated substance |
Reference date I: 1/10/1996 |
Reference date II: 16/7/1997 |
Target date I: 1/7/2000 |
Cadmium in mg per kg dry matt. |
0.8 |
|
0.4 |
Cadmium in mg per kg Tot-P |
200 |
|
100 |
LAS in mg per kg dry matter |
|
2600 |
1300 |
PAH in mg per kg dry matter |
|
6 |
3 |
NPE in mg per kg dry matter |
|
50 |
10 |
DEHP in mg per kg dry matter |
|
100 |
50 |
As it appears from Table 11-23 the threshold limit value for cadmium has to be reduced with 50% within four years. As there are no new initiatives under way these values will for the time being also be
relevant for 2004.
For the organic substances the content has to be reduced also with 50% within the period from 1997 to 2000, except for NPE (nonylphenol ethoxylates), which will be reduced with 80% over the same
period.
The threshold limit values for other regulated heavy metals (mercury, lead and nickel) are not reduced.
Emission of oil and products based on mineral oil is regulated also through the Helsinki and the OSPAR conventions. According to these conventions the emissions has to be reduced with 50%.
11.10.1.4 Air to water and soil
Deposition from air to water and soil involves, according to the section on Human Toxicity in chapter on Normalisation references , the following substances:
- Lead (Pb)
- Mercury (Hg)
- Arsenic (As)
- Cadmium (Cd)
- Chromium (Cr)
- Polycyclic aromatic hydrocarbons (PAH)
- Particulate matter
- Dioxins
The dominating source of airborne lead has been organic lead compounds in petrol. However, as leaded petrol has been phased out a number of years ago, it is not relevant for this project.
The source of airborne mercury originates primarily from power generation, waste incineration, sludge and land filling. The emission of mercury is indirectly regulated through the use of mercury for various
purposes. According to an executive regulation on marketing and export of mercury and mercury containing products (Miljø- og Energiministeriet 1998), the use of mercury thermometers for certain
applications is prohibited from 30 June 2004 and other thermometers from 31 December 1999. For a long range of other applications the use of mercury will still be allowed.
It is estimated that the regulated applications accounts for about 20% of the total use, whereas the regulation corresponds to a reduction of the present use with about 20% by 2004.
Arsenic, cadmium and chromium do not become airborne to the same extent as mercury, so the regulation of these substances can not be considered as relevant for the deposition of the substances.
Hence, it is not possible to establish reduction targets for these substances.
The PAH originates from a long range of different sources, both stationary and mobile, but typically combustion processes. PAH is regulated as threshold limit values from some of the sources, but there are
no overall national reduction targets.
This is the same for particulate matter.
Dioxin primarily originates from combustion, and especially solid waste incineration. Denmark had earlier its own reduction target for solid waste incineration, but it has now been replaced by a general EU
reduction target on 90% of the emission by 2005, based on the 1985 emission level (EU 1992). This target corresponds to a reduction on 45% from 1994 to 2004.
Based on the figures for ecotoxicity potentials presented in the sections on Ecotoxicity, the weighted reduction target for the substances will be 10% from 1994 to 2004. It should be emphasised that only the
reduction target for mercury contributes to this weighted reduction target.
11.10.1.5 Anti-fouling to water
The outlet of anti-fouling substances to the water environment is not yet regulated as such (as of March 1999), but the Minister of Environment and Energy has proposed a executive regulation prohibiting the
use of pesticide containing anti-fouling paint for leisure boats by 2003.
According to a survey of the consumption of anti-fouling substances (tributyltin, TBT, trade name Diuren) in Denmark, conducted by the Danish EPA, the annual consumption of anti-fouling substances
(active substance) is 850-1700 tonnes (1997). There is no exact figure for the consumption of these substances for large ships, which move much abroad and therefore release their anti-fouling treatment
abroad. However, if it is assumed that half of the anti-fouling paint is used for leisure boats this means a reduction target on 50% by 2003 and also by 2004, in case there will not be established reduction
targets for other ships. Based on the above assumptions the reduction from 1994 to 2004 will be 50%.
11.10.1.6 Weighting factors
Based on the above assumptions the following weighting factors can be calculated for ecotoxicity for Denmark, see Table 11-24 below.
Table 11-24
Calculation of weighting factor for chronic aquatic ecotoxicity, Denmark.
Activity / Substances |
Scaled reduction target, 1994-2004 |
Ecotox Potential, etwc (m3/yr) |
Ecotox Potential, etwc (m3/yr) |
Weighting factor |
% |
1994 |
2004 |
|
Waste water / Organic substances |
20 |
3,75E+11 |
3,00E+11 |
|
Waste water / Metals |
20 |
7,77E+11 |
6,22E+11 |
|
Pesticides |
36 |
|
|
|
Sludge / Metals |
50 |
|
|
|
Sludge / Organic substances |
50 |
|
|
|
Oil to water |
50 |
|
|
|
Deposition to water |
10 |
1,37E+11 |
1,23E+11 |
|
Deposition to soil |
10 |
|
|
|
Antifouling / Metals |
50 |
2,83E+12 |
1,42E+12 |
|
Total |
|
4,12E+12 |
2,46E+12 |
1.67 |
Table 11-25
Calculation of weighting factor for acute aquatic ecotoxicity, Denmark.
Activity / Substances |
Scaled reduction target, 1994-2004 |
Ecotox Potential, etwa (m3/yr) |
Ecotox Potential, etwa (m3/yr) |
Weighting factor |
% |
1994 |
2004 |
|
Waste water / Organic substances |
20 |
2,30E+10 |
1,84E+10 |
|
Waste water / Metals |
20 |
7,88E+10 |
6,30E+10 |
|
Pesticides |
36 |
|
|
|
Sludge / Metals |
50 |
|
|
|
Sludge / Organic substances |
50 |
|
|
|
Oil to water |
50 |
|
|
|
Deposition to water |
10 |
|
|
|
Deposition to soil |
10 |
|
|
|
Antifouling / Metals |
50 |
2,83E+11 |
1,42E+11 |
|
|
|
|
|
|
Total |
|
3,85E+11 |
2,23E+11 |
1.73 |
Table 11-26
Calculation of weighting factor for chronic terrestrial ecotoxicity, Denmark.
Activity / Substances |
Scaled reduction target, 1994-2004 |
Ecotox Potential, etsc (m3/yr) |
Ecotox Potential, etsc (m3/yr) |
Weighting factor |
|
% |
1994 |
2004 |
|
Waste water / Organic substances |
20 |
|
|
|
Waste water / Metals |
20 |
|
|
|
Pesticides, fungicides |
36 |
3,50E+11 |
2,24E+11 |
|
Pesticides, Herbicides |
36 |
2,85E+12 |
1,82E+12 |
|
Pesticides, Insecticides |
36 |
2,15E+11 |
1,38E+11 |
|
Sludge / Metals |
50 |
1,89E+07 |
9,45E+06 |
|
Sludge / Organic substances |
50 |
|
|
|
Oil to water |
50 |
|
|
|
Deposition to water |
10 |
|
|
|
Deposition to soil |
10 |
5,90E+07 |
5,31E+07 |
|
Antifouling / Metals |
50 |
|
|
|
|
|
|
|
|
Total |
|
3,42E+12 |
2,19E+12 |
1.56 |
11.10.2 Weighting factors for ecotoxicity at EU level
This section has been organised in the same way as the above section for Denmark.
11.10.2.1 Organic substances and metals to water
For EU as a whole the emission of organic substances and metals to water, which in this context means marine areas, are primarily regulated through the Helsinki Convention and The OSPAR Convention,
as most of the EU coastline is linked to the North Atlantic and Baltic oceans. No regulation covering the relevant substances for the Mediterranean Sea has been identified. Hence, the above regulation has
been applied for this sea as well.
According to these conventions the discharge of hazardous substances to the oceans should be reduced with 50% in relation to the emissions in 1985. The list of substances includes those, which are
mentioned under sections on Normalisation References for Ecotoxicity, e.g. metals, organics, pesticides and organotin compounds. The target year was earlier 1995, but has now been prolonged to 2000.
Therefore, the target for 2004 in this context is considered as being 50% of the 1985 emissions which corresponds to a reduction of about 20% from 1994 to 2004.
11.10.2.2 Pesticides to soil
There are no regulations of the amounts of pesticides at EU level.
11.10.2.3 Organic substances and metals in sludge
The outlet of organic substances and metals to the soil, via sludge from wastewater treatment plants, is regulated through the Council Resolution 86/278/EEC. However, this regulation does not provide a
basis for establishing an overall reduction target for these substances at EU level.
11.10.2.4 Oil to water
Emission of oil and products based on mineral oil is regulated also through the Helsinki and the OSPAR conventions. According to these conventions the emissions has to be reduced with 50%.
11.10.2.5 Air to water and soil
There is no regulation at EU level that lead to reduction targets for heavy metals and organic substances above, except dioxins.
According to the EU regulation the reduction target is to reduce the emission with 90% of the 1985 emission by 2005 (EU 1992). This target corresponds to a reduction on 45% from 1994 to 2004.
11.10.2.6 Anti-fouling to water
There are no regulations of anti-fouling substances at EU level.
11.10.2.7 Weighting factors
Based on the above assumptions the following weighting factors for EU can be calculated for ecotoxicity, see Table 11-27-Table 11-29 below.
Table 11-27
Calculation of weighting factor for chronic aquatic ecotoxicity, EU.
Activity / Substances |
Scaled reduction target, 1994-2004 |
Ecotox Potential, etwc (m3/yr) |
Ecotox Potential, etwc (m3/yr) |
Weighting factor |
% |
1994 |
2004 |
|
Waste water / Organic substances |
20 |
1,89E+13 |
1,51E+13 |
|
Waste water / Metals |
20 |
4,16E+13 |
3,33E+13 |
|
Pesticides |
0 |
|
|
|
Sludge / Metals |
0 |
|
|
|
Sludge / Organic substances |
0 |
|
|
|
Oil to water |
50 |
3,63E+12 |
1,82E+12 |
|
Deposition to water |
45 |
1,24E+13 |
6,82E+12 |
|
Deposition to soil |
45 |
|
|
|
Anti-fouling / Metals |
0 |
5,38E+13 |
5,38E+13 |
|
|
|
|
|
|
Total |
|
1,30E+14 |
1,11E+14 |
1.18 |
Table 11-28
Calculation of weighting factor for acute aquatic ecotoxicity, EU.
Activity / Substances |
Scaled reduction target, 1994-2004 |
Ecotox Potential, etwa (m3/yr) |
Ecotox Potential, etwa (m3/yr) |
Weighting factor |
% |
1994 |
2004 |
|
Waste water / Organic substances |
20 |
1,16E+12 |
9,28E+11 |
|
Waste water / Metals |
20 |
4,19E+12 |
3,35E+12 |
|
Pesticides |
0 |
|
|
|
Sludge / Metals |
0 |
|
|
|
Sludge / Organic substances |
0 |
|
|
|
Oil to water |
50 |
6,06E+10 |
3,03E+10 |
|
Deposition to water |
45 |
|
|
|
Deposition to soil |
45 |
|
|
|
Anti-fouling / Metals |
0 |
5,38E+12 |
5,38E+12 |
|
|
|
|
|
|
Total |
|
1,08E+13 |
9,69E+12 |
1.11 |
Table 11-29
Calculation of weighting factor for terrestrial ecotoxicity, EU.
Activity / Substances |
Scaled reduction target, 1994-2004 |
Ecotox Potential, etsc (m3/yr) |
Ecotox Potential, etsc (m3/yr) |
Weighting factor |
% |
1994 |
2004 |
|
Waste water / Organic substances |
20 |
|
|
|
Waste water / Metals |
20 |
|
|
|
Pesticides, fungicides |
0 |
7,83E+13 |
7,83E+13 |
|
Pesticides, Herbicides |
0 |
1,27E+14 |
1,27E+14 |
|
Pesticides, Insecticides |
0 |
1,52E+14 |
1,52E+14 |
|
Sludge / Metals |
0 |
5,35E+08 |
5,35E+08 |
|
Sludge / Organic substances |
0 |
|
|
|
Oil to water |
50 |
|
|
|
Deposition to water |
45 |
|
|
|
Deposition to soil |
45 |
3,51E+10 |
1,93E+10 |
|
Anti-fouling / Metals |
0 |
|
|
|
|
|
|
|
|
Total |
|
3,57E+14 |
3,57E+14 |
1.00 |
11.10.3 Weighting factors for ecotoxicity at global level
There is no basis for establishing weighting factors for ecotoxicity at global level, as there are no overall global reduction targets for the substances involved in the calculation of normalisation references for
ecotoxicity.
11.10.4 Comparison of existing and new weighting factors
Table 11-30 summarises the weighting factors calculated for ecotoxicity for the two geographical areas, and compares them to the previously developed weighting factors in the EDIP project.
Table 11-30
Weighting factors for ecotoxicity.
Category and area |
This project |
EDIP (Hauschild et al. 1998b) |
Chronic aquatic ecotoxicity, Denmark |
1.67 |
2.6 |
Acute aquatic ecotocxicity, Denmark |
1.73 |
2.6 |
Terrestrial ecotoxicity, Denmark |
1.56 |
1.9 |
Chronic aquatic ecotoxicity, EU |
1.18 |
n.a. |
Acute aquatic ecotocxicity, EU |
1.11 |
n.a. |
Terristial ecotoxicity, EU |
1.0 |
n.a. |
The uncertainty connected with the calculation of the weighting factors for ecotoxicity is much higher than that of Global Warming and Ozone Depletion. The reason for this is:
- The impact mechanisms are very complicated
- The regulation only covers few of the involved substances
- A lot of the basic data are lacking and inaccurate
11.11 Human toxicity
11.11.1
Weighting factors for human toxicity for Denmark
According to the section on normalisation references for human toxicity in the previous chapter, the main substances contributing to the normalisation reference are those indicated in the table 1.27 below. All
the other substances will be neglected in the further calculation of weighting factor for this impact category, because their contributions are so limited.
The political reduction targets for the substances contributing to the normalisation references have been mentioned in the earlier sections. For lead, cadmium, PAH, particles and benzene the reduction targets
appear from the section on Ecotoxicity above. The political targets for SO2 and NOx appear from the section on Acidification and the targets for nmVOC and CO appear from the section on Photochemical
Ozone.
The calculated Targeted Impact Potentials for the various substances in Table 11-31 below is based on the Impact Potentials for 1994, transferred from the Normalisation chapter.
Table 11-31
Human toxicity for air, Denmark.
Substance/Activity |
Scaled reduction target in %, |
Impact potential, 1994 |
Targeted impact potential, 2004 |
1994-2004 |
|
|
NmVOC – transport |
30 |
2.80E+17 |
1.96E+17 |
Lead (Pb) |
0 |
3.95E+15 |
3.95E+15 |
NOx |
33 |
2.37E+15 |
1.59E+15 |
Particles |
0 |
1.02E+15 |
1.02E+15 |
NMVOC |
30 |
8.03E+14 |
5.62E+14 |
Benzene |
0 |
7.33E+14 |
7.33E+14 |
CO |
0 |
5.94E+14 |
5.94E+14 |
PAH |
0 |
2.19E+14 |
2.19E+14 |
SO2 |
40 |
2.05E+14 |
1.23E+14 |
Cadmium |
0 |
1.30E+14 |
1.30E+14 |
Total |
|
2.88E+17 |
2.03E+17 |
Based on these assumptions and data the weighting factor for human toxicity for air in Denmark will be:
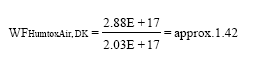
According to the section on normalisation references for human toxicity, the only substance contributing with more than 1% to the normalisation reference concerning human toxicity for water in Denmark is
mercury, divided into 90% from air deposition and 9% from wastewater emission. As there are no political reduction targets for mercury deposition from air, the only relevant reduction in this respect is the
one concerning emission to the waterways. This issue is regulated by the OSPAR convention, which prescribes a 50% reduction. This leads to the result as shown in Table 11-32.
Table 11-32
Human toxicity for water, Denmark.
Substance/ Activity |
Scaled reduction target in %, 1994-2004 |
Impact potential 1994 |
Impact potential 2004 |
Mercury (Hg) water emission |
20 |
7,98E+10 |
6,38E+10 |
Mercury (Hg) air emissions |
0 |
8,34E+11 |
8,34E+11 |
Total |
|
9,14E+11 |
8,98E+11 |
Based on these assumptions and data the weighting factor for human toxicity for Denmark will be:

According to the section on normalisation references for human toxicity, the only substances contributing with more than 1% to the normalisation reference concerning soil in Denmark is mercury and arsenic
deposited from air, arsenic, mercury and tetrachloroethylene from sludge. Threshold limit values have been established for various substances in sludge when used for agricultural purposes and for heavy
metals in air, but there are no national targets for reducing the total load of the substances to soil. Hence, the weighting factors for human toxicity for soil will be one:
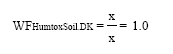
11.11.2 Weighting factors for human toxicity at EU level
This section is organised in the same way as under the section on Human toxicity for Denmark. Concerning the legal basis for the reduction targets reference is made to the previous sections.
For human toxicity for air at EU level the only regulated substances are those regulated as regional air pollutants, e.g. NOx, SO2 and nmVOC. The reduction targets are thus defined according to the
OSPAR convention. The data on which the calculation of the weighting factor is based on appears from Table 11-33 below
Table 11-33
Human toxicity for air, EU.
Substance |
Scaled reduction target in %,1994-2004 |
Impact potential, 1994 |
Impact potential, 2004 |
NmVOC – transport |
30 |
2.15E+19 |
1.51E+19 |
Lead (Pb) |
0 |
7.07E+17 |
7.07E+17 |
NOx |
33 |
1.08E+17 |
7.24E+16 |
NMVOC |
30 |
7.42E+16 |
5.19E+16 |
Particles |
0 |
5.78E+16 |
5.78E+16 |
CO |
0 |
3.74E+16 |
3.74E+16 |
Benzene |
0 |
3.69E+16 |
3.69E+16 |
PAH |
0 |
2.24E+16 |
2.24E+16 |
SO2 |
40 |
1.57E+16 |
9.42E+15 |
Cadmium |
0 |
1.14E+16 |
1.14E+16 |
Total |
|
2.25E+19 |
1.60E+19 |
Based on these assumptions and data the weighting factor for human toxicity for EU will be:
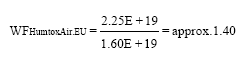
According to the chapter on normalisation reference for human toxicity the substances that contribute with more than 1% for water are: mercury from air deposition, mercury from water emission, dioxin from
air deposition, lead from water emission, lead from air deposition and zinc from water emission.
However, the only substances for which there have been established reduction targets are: metals from air deposition (20%) and metal from water emission (45%).
Table 11-34
Human toxicity for water at EU level.
Substance/ Activity |
Scaled reduction target in %,1994-2004 |
Impact potential1994 |
Impact potential 2004 |
Mercury, water emissions |
45 |
1,86E+12 |
1,02E+12 |
Zink, water emissions |
45 |
2,02E+11 |
1,11E+11 |
Lead, water emissions |
45 |
4,00E+11 |
2,20E+11 |
Mercury, air emissions |
20 |
1,54E+13 |
1,23E+13 |
Lead, air emissions |
20 |
3,75E+11 |
3,00E+11 |
Dioxin, air emissions |
20 |
8,02E+11 |
6,42E+11 |
Total |
|
1,90E+13 |
1,46E+13 |
Based on these assumptions and data the weighting factor for human toxicity for water at EU level will be:
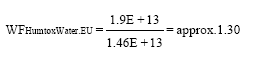
According to the chapter on normalisation reference for human toxicity the substances contributing with more than 1% for soil are: arsenic from air deposition, mercury from air deposition, arsenic from
sludge, mercury from sludge, chromium from air deposition, lead from air deposition and cadmium from air deposition.
However, the only substances for which reduction targets have been established are: metals from air deposition (20%) and metal from water emission (45%).
Table 11-35
Human toxicity for soil at EU level.
Substance/Activity |
Scaled reduction target in %,1994-2004 |
Impact potential1994 |
Impact potential 2004 |
Arsenic from air |
20 |
2,82E+10 |
2,26E+10 |
Mercury, air |
20 |
1,13E+10 |
9,04E+09 |
Chromium, air |
20 |
6,22E+08 |
4,98E+08 |
Lead from air |
20 |
5,87E+08 |
4,70E+08 |
Cadmium, air |
20 |
4,68E+08 |
3,74E+08 |
Arsenic, sludge |
0 |
1,77E+09 |
1,77E+09 |
Mercury, sludge |
0 |
7,12E+08 |
7,12E+08 |
Total |
|
4,37E+10 |
3,54E+10 |
Based on these assumptions and data the weighting factor for human toxicity for EU will be:
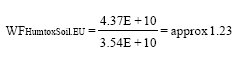
11.11.3 Weighting factors for human toxicity at the Global level
There is no basis for establishing weighting factors for human toxicity at global level, as there are no overall global reduction targets for the substances involved in the calculation of normalisation references for
human toxicity.
11.11.4 Comparison of existing and new weighting factors
Table 11-36 summarises the weighting factors calculated for human toxicity for the two geographical areas, and compares them to the previously developed weighting factors in the EDIP project.
Table 11-36
Weighting factors for human toxicity.
Category and area |
This project |
EDIP (Hauschlid et al. 1998a) |
Human toxicity, Air, Denmark |
1.42 |
1.1 |
Human toxicity, Water, Denmark |
1.02 |
2.9 |
Human toxicity, soil Denmark |
1.00 |
2.7 |
Human tocxicity, Air, EU |
1.40 |
n.a. |
Human tocxicity, Water, EU |
1.30 |
n.a. |
Human toxicity, Soil, EU |
1.23 |
n.a. |
The uncertainty connected with the calculation of the weighting factors for human toxicity is much higher than that of Global Warming and Ozone Depletion. The reason for this is:
- The impact mechanisms are very complicated
- The regulation only covers few of the involved substances
- A lot of the basic data are lacking or inaccurate
11.12 Comparison of existing and new weighting factors
The existing and new weighting factors are summarised in Table 11-37.
Table 11-37
Summary of calculated weighted factors (WF) and comparison with EDIP factors. WFs at global scale have only been calculated for Global Warming, Ozone depletion and Photochemical Ozone, due to the
fact that regulation of the other effect categories have not been implemented at global level..
Impact category |
New weighting factors 1994 - 2004 |
EDIP weighting factors, 1990 – 2000 |
Global Warming, Denmark |
1.12 |
1.3 |
Global Warming, EU |
1.05 |
- |
Global Warming, World, Industrialised countries |
1.11 |
- |
Ozone Depletion, Denmark |
|
23 |
Ozone Depletion, EU |
2.46 |
- |
Ozone Depletion, World, Industrialised Countries |
63 |
- |
Ozone Depletion, World, Develop. Countries |
4.4 |
- |
Photochemical Ozone, Denmark |
1.26 |
1.2 |
Photochemical Ozone, EU |
1.33 |
- |
Photochemical Ozone, World |
1.00 |
- |
Acidification, Denmark |
1.34 |
1.3 |
Acidification, EU |
1.27 |
- |
Nutrient enrichment, Denmark |
1.31 |
1.2 |
Nutrient enrichment, EU |
1.22 |
- |
Ecotoxicity, Denmark, Aquatic chronic |
1.67 |
2.6 |
Ecotoxicity, Denmark, Aquatic acute |
1.73 |
2.6 |
Ecotoxicity, Denmark, Terrestrial |
1.56 |
1.9 |
Ecotoxicity, EU, Aquatic chronic |
1.18 |
- |
Ecotoxicity, EU, Aquatic acute |
1.11 |
- |
Ecotoxicity, EU, Terrestrial |
1.00 |
- |
Human toxicity, Denmark, Air |
1.42 |
1.1 |
Human toxicity, Denmark, Water |
1.02 |
2.9 |
Human toxicity, Denmark, Soil |
1.00 |
2.7 |
Human toxicity, EU, Air |
1.40 |
- |
Human toxicity, EU, Water |
1.30 |
- |
Human toxicity, EU, Soil |
1.23 |
- |
11.13 Methodological concerns
11.13.1 Difference between impact category and emission targets
One of the major methodological concerns in connection with establishment of weighting factors is the difference between the scope of the impact category and the regulation and the political targets on
which the calculation of the weighting factors are based. Especially for the two impact categories, ecotoxicity and human toxicity there seem to be large differences in the scope of the regulation and the
impact mechanisms. In the following some of the aspects connected to this issue is discussed.
11.13.2 Impact category versus substances
In the case of the impact category, Global Warming, Denmark contributes to the global warming with CO2, CH4, N2O, CFCs etc., but the regulation is only directed towards the emission of CO2. This
means that in principle it is only possible to establish a weighting factor for CO2, but it has to cover the whole impact category.
Weighting factors for CH4 and CFCs can be established through the regulation of these substances according to other impact categories, i.e. photo oxidant formation and the ozone layer protection.
However, there is no regulation of N2O on which the calculation of weighting factors can be based. This may be a problem, if the LCA of a product has a major component of e.g. N2O.
11.13.3 Quick phase out
For some substances, e.g. CFCs, a relatively quick phase out has been decided, and obviously it also seems to take place. In such cases the weighting factors will be very high or even infinite. This is the
case if the weighting factor is established through dividing the actual emission in 1994 with the supposed consumption of the substances in 2004, where it is supposed to be eliminated.
This will give an infinite weighting factor for one impact category, which will overrule all other impact categories, unless the weighting factor is modified.
11.13.4 Application of weighting factors for different countries
When a product is imported to another country it may have impacts in both countries. The question is which weighting factors should be applied to the product to give a fair assessment in relation to other
product that may be imported from a third country with another weighting factor for the same impact category.
The choice will in general depend on the purpose of the weighting. The general recommendation to use EU normalisation references and weighting factors reflects the political prioritisation in Europe, which is
relevant if the decision makers or important stakeholders are located in Europe. If the stakeholders are located outside of Europe, e.g. in Asia or the United States, this approach may be less relevant, and
the World-wide normalisation references and weighting factors could be used. If should however be observed that these figures are crude estimates, and that the optimal solution would be to derive
country-specific weighting factors.
11.14 Recommendation for future updating
For some of the above mentioned impact categories the political targets and regulations are relatively clear and fits very well to the impact mechanisms. In these cases there are usually well-established
reporting systems providing reliable data, and it is relatively simple to update weighting factors.
However, in cases where the regulation and the political target setting do not fit the impact category, it requires considerable resources to provide the necessary data. Also in cases where many different
substances are involved, it requires considerable resources to collect the necessary data and to adopt them to the established methodology.
It should be considered to simplify the methodology and the calculation procedure in cases where there are larger differences between the impact category and regulation on which the weighting factors are
based.
11.15 References:
Energistyrelsen 1996, Energy 21. Danish Ministry of Environment and Energy, April 1996. Available at: http://www.ens.dk.
Energistyrelsen 1999, Energy statistics. Available at: http://www.ens.dk.
EU 1991a, Council Directive 91/271/EEC of 21 May 1991 concerning urban waste-water treatment. Available at: http://europe.eu.int.
EU 1991b, Council Directive 91/676/EEC of 12 December 1991 concerning the protection of waters against pollution caused by nitrates from agricultural sources. Available at:
http://europe.eu.int.
EU 1992, Revised Seveso Directive, EU Directive on Accident Hazards in Large Industrial plants. Council Directive 96/82/EC. Available at: http://europe.eu.int.
EU 1994, Council Regulation (EC) No 3093/94 of 15 December 1994 on substances that deplete the ozone layer. http://europe.eu.int.
EU 1996, IPPC directive (Integrated Pollution Prevention and Control directive), Council Directive 96/61/EC of 24 September 1996. Available at: http://europe.eu.int.
EU 1998, EU Environment Minister Resolution, 17 June 1998. Available at: http://europe.eu.int.
European Commission 1996, Progress Report on Implementation of the European Community Programme of Policy and Action in relation to the Environment and sustainable development. COM(95) 624,
final. January 10, 1996. Available at: http://europe.eu.int.
Hansen, J.H. 1997, Ozonlagsnedbrydende stoffer og visse drivhusgasser - 1995. Forbrug og emissioner. Miljøprojekt nr. 342. København: Miljøstyrelsen.
Hauschild, M.Z., Olsen, S.I. & Wenzel, H. 1996, Toksicitet for mennesker i miljøet som vurderingskriterium ved miljøvurdering af produkter. I Hauschild, M.Z. (ed.), Baggrund for miljøvurdering af
produkter. Miljø- og Energiministeriet, Dansk Industri. København. (in Danish).
Hauschild, M.Z. & Wenzel, H. 1996a, Drivhuseffekt som vurderingskriterium ved miljø.vurdering af produkter. I Hauschild, M.Z. (ed.) Baggrund for miljø.vurdering af produkter. Miljø- og
Energiministeriet, Dansk Industri. København. (in Danish).
Hauschild, M.Z. & Wenzel, H. 1996b, Forsuring som vurderingskriterium ved miljø.vurdering af produkter. I Hauschild, M.Z. (ed.) Baggrund for miljø.vurdering af produkter. Miljø- og
Energiministeriet, Dansk Industri. København. (in Danish).
Hauschild, M.Z. & Wenzel, H. 1996c, Fotokemisk ozondannelse som vurderingskriterium ved miljø.vurdering af produkter. I Hauschild, M.Z. (ed.) Baggrund for miljø.vurdering af produkter.
Miljø- og Energiministeriet, Dansk Industri. København. (in Danish).
Hauschild, M.Z. & Wenzel, H. 1996d, Næringssaltbelastning som vurderingskriterium ved miljøvurdering af produkter. I Hauschild, M.Z. (ed.) Baggrund for miljø.vurdering af produkter. Miljø-
og Energiministeriet, Dansk Industri. København. (in Danish).
Hauschild, M.Z. & Wenzel, H. 1996e, Stratosfærisk ozonnedbrydning som vurderingskriterium ved miljøvurdering af produkter. I Hauschild, M.Z. (ed.) Baggrund for miljø.vurdering af
produkter. Miljø- og Energiministeriet, Dansk Industri. København. (in Danish).
Hauschild, M.Z., Wenzel, H., Damborg, A. & Tørsløv, J. 1996, Økotoksicitet som vurderingskriterium ved miljøvurdering af produkter. I Hauschild, M.Z. (ed.) Baggrund for miljø.vurdering af
produkter. Miljø- og Energiministeriet, Dansk Industri. København. (in Danish).
Hauschild, M. & Wenzel, H. 1998, Acidification as a criterion in the environmental assessment of products in Environmental assessment of products. Volume 2 Scientific background eds. Hauschild,
M. & Wenzel, H. London: Chapman & Hall.
Hauschild, M.Z., Olsen, S.I. & Wenzel, H. 1998, Human toxicity as a criterion in the environmental assessment of products. In Hauschild M, Wenzel H (eds.). Environmental assessment of products.
Volume 2: Scientific background. London: Chapman & Hall
Hauschild, M. & Wenzel, H. 1998a, Acidification as a criterion in the environmental assessment of products. In Hauschild M, Wenzel H (eds.). Environmental assessment of products. Volume 2:
Scientific background. London: Chapman & Hall.
Hauschild, M. & Wenzel, H. 1998b, Global warming as a criterion in the environmental assessment of products. In Hauschild M, Wenzel H (eds.). Environmental assessment of products. Volume 2:
Scientific background. London: Chapman & Hall.
Hauschild, M. & Wenzel, H. 1998c, Nutrient enrichment as a criterion in the environmental assessment of products. In Hauschild M, Wenzel H (eds.). Environmental assessment of products. Volume 2:
Scientific background. London: Chapman & Hall.
Hauschild, M. & Wenzel, H. 1998d, Photochemical ozone formation as a criterion in the environmental assessment of products. In Hauschild M, Wenzel H (eds.). Environmental assessment of products.
Volume 2: Scientific background. London: Chapman & Hall
Hauschild, M. & Wenzel, H. 1998e, Stratospheric ozone depletion as a criterion in the environmental assessment of products. In Hauschild M, Wenzel H (eds.). Environmental assessment of products.
Volume 2: Scientific background. London: Chapman & Hall.
Hauschild, M., Wenzel, H., Damborg, A. & Tørsløv, J. 1998, Ecotoxicity as a criterion in the environmental assessment of products. In Hauschild M, Wenzel H (eds.). Environmental assessment of
products. Volume 2: Scientific background. London: Chapman & Hall.
Helsinki Commission 1974, Convention on the Protection of the Marine environment of the Baltic Sea Areas (HELCOM). Available at http://www.helcom.fi.
ISO 2000a, Environmental management – Life cycle assessment – Life cycle impact assessment. ISO 14042.
Miljø- og Energiministeriet (Ministry of Environment and Energy) 1996, Executive regulation for the Application of Waste Products for Agricultural Purposes (Executive regulation on Waste Water Sludge),
no. 823, 16 September 1996.
Miljø- og Energiministeriet (Ministry of Environment and Energy) 1998, Executive regulation on prohibition of marketing and export of mercury and mercury containing products, executive regulation no.
692, 22 September 1998.
Miljøstyrelsen (Danish EPA) 1986, Action plan for the reduction of the consumption of Pesticides, Danish EPA, 1986 (Pesticidhandlingsplanen, 1986).
Miljøstyrelsen 1998, Vandmiljøplan II (Water Environmental Action Plan II). Available at: http://www.mst.dk.
Miljøstyrelsen 1999, Homepage of Danish EPA, www/mst.dk/Ozonelayer/National regulation.
OSPAR 1992, Convention for the Protection of the Marine Environment of North-East Atlantic (1992 OSPAR Convention). Available at http://www.ospar.org.
UN 1997, Kyoto Protocol to the United Nations Framework Convention on Climate Change. December 1997.
UN-ECE 1979, Convention on Long-range Transboundary Air Pollution. United Nations, Economic Commission for Europe. Available: http//:www.unece.org.
UN-ECE 1988a, The 1988 Sofia Protocol concerning the Control of Emis.sions of Nitrogen Oxides or their Transboundary Fluxes. United Nations, Economic Commission for Europe. Available:
http://www.unece.org.
UN-ECE 1988b, Declaration on NOx. United Nations, Economic Commission for Europe. Available: http://www.unece.org.
UN-ECE 1991, Protocol to the 1979 Convention on Long-range Transboundary Air Pollution concerning the control of emissions of volatile organic compounds or their transboundary fluxes.
United Nations, Economic Commission for Europe. Available: http//:www.unece.org.
UN-ECE 1994, The 1994 Oslo Protocol on Further Reduction of Sulphur Emissions. United Nations, Economic Commission for Europe. Available: http://www.unece.org.
UNEP 1987, The 1987 Montreal Protocol on Substances that Deplete the Ozone Layer as adjusted and amended by the second Meeting of the Parties (London, 27-29 June 1990) and by the fourth
Meeting of the Parties (Copenhagen, 23-25 November 1992) and further adjusted by the seventh Meeting of the Parties (Vienna, 5-7 December 1995) and further adjusted and amended by the ninth
Meeting of the Parties (Montreal, 15-17 September 1997). Available at: http://www.unep.org.
UNEP 1999, UNEP/IE statistics. Available at: http://www.unepie.int.com.
UNEP 2002, Production and Consumption of Ozone Depleting Substances under the Montreal Protocol, Ozone Secretariat, UNEP, April 2002.
UN/FCCC 2003 (On-line searchable database of GHG inventory data) Available at: http:// http://ghg.unfccc.int/
Footnotes
[1] Gross domestic product (GDP) measures the total output of goods and services for final use occurring within the domestic territory of a given country, regardless of the allocation to domestic and foreign
claims.
[2] Gross national product (GNP) measures the total domestic and foreign income claimed by the residents of the economy.
[3] The World Bank statistics use 1987 as baseline in some of their surveys.
[4] The total GDP is calculated to 25.284 1012 $ (UN, 1996) and a midyear world population at 5,609,678,819 in 1994 (USBC, 1996). The average GDP/capita equals to 4,515 US$/capita.
[5] The Montreal Protocol is ratified/accessed/accepted/approved by 168 countries at February 8, 1999 (UNEP 1999).
[6] The London Amendment is ratified/accessed/accepted/approved by 127 countries at February 8, 1999 (UNEP 1999).
[7] The Copenhagen Amendment is ratified/accessed/accepted/approved by 86 countries at February 8, 1999 (UNEP 1999).
[8] The UN-ECE database is available at http://www.unece.org.
[9] Most of the reports from Miljøstyrelsen (the Danish Environmental Protection Agency) contain English summaries.
[10] In this context `environment' does not cover indoor consumer exposure nor work environment.
[11] It should be noticed that only actual emissions are included. Releases from environmental pools created in the past (for instance mercury from sediments and leaks from landfills) are not included.
Whether or not these releases should be included is controversial and should be discussed further in connection with a future updating of the methodology.
[12] Corinair94 operates with 10 sector sources: 1. Combustion in energy and transformation industries, 2. Non-industrial combustion plants, 3. Combustion in manufacturing industry, 4. Production
processes, 5. Extraction and distribution of fossil fuels/geothermal energy, 6. Solvent and other product use, Road transport, 8. Other mobile sources and machinery, 9. Waste treatment and disposal, 10.
Agriculture and forestry, land use and wood stock change.
[13] BS represents the black soot particles from combustion, and is dominated by coal smoke and diesel soot. BS is a relevant indicator to assess health effects, but the measurement technique provides
fairly inaccurate results (EEA 1997).
[14] Also EQF's for heavy metals are "category" EQF's as the metal may be present in the environment as pure element or as a part of various compounds with different toxicity. The EDIP EQF's for heavy
metals are calculated based on reasonable worst case assumptions.
[15] Gross domestic product (GDP) measures the total output of goods and services for final use occurring within the domestic territory of a given country, regardless of the allocation to domestic and
foreign claims.
[16] CORINAIR means "CORe INventory AIR" and is a part of the work programme of the European Environment Agency (EEA).
[17] ETC/AE means the European Topic Centre on Air Emissions.
| Top | | Front page |
Version 1.0 April 2005, © Danish Environmental Protection Agency
|