| Front page | | Contents | | Previous | | Next |
AMAP Greenland and the Faroe Islands 1997-2001
5 Other contaminants
5.1 Introduction
5.2 Tributyltin (TBT)
5.3 Toxaphene
5.4 Dioxines and furanes (PCDD/Fs)
5.5 Coplanar PCBs (nPCB)
5.6 Chlorobenzene (CBz)
5.7 New chlorinated pesticides
5.8 Polybrominated diphenyl ethers (PBDE)
5.9 Polycyclic Aromatic Hydrocarbons (PAH)
5.9.1 Precipitation
5.9.2 Terrestrial Environment
5.9.3 Freshwater environment
5.9.4 Marine environment
5.10 Contaminants of future concern
5.10.1 Perfluorooctane sulfonate (PFOS)
5.10.2 Synthetic musks
5.10.3 Polychlorinated naphtalenes
5.10.4 Brominated flame retardants
5.10.5 PBDD/F
5.10.6 Triclosan
5.10.7 Aromatic amines
5.10.8 Conclusions and recommendations
5.11 References
Citation: Pécseli, M.2, F. Riget1, K. Vorkamp2, M. Glasies,2 & G. Asmund,1 In: Riget, F., J. Christensen & P. Johansen (eds). Chapter 5. other Contaminants. AMAP Greenland and the Faroe Islands. Vol. 2: The Envirorment of Geenland. Ministry of Environment, Denmark 1 Department of Arctic Environment, National Environmental Research Institute, Frederiksborgvej 399, Box 358, DK-4000 Roskilde, Denmark 2Department of Environmental Chemistry and Microbiology, National Environmental Research Institute, Frederiksborgvej 399, Box 358, DK-4000 Roskilde, Denmark
5.1 Introduction
Numerous inorganic and organic pollutants occur in the industrial products of daily use, leading to a current emission into the environment, where they will be transported by the atmosphere and the sea. Former studies in the Arctic region have shown that long-range transport of compounds produced and emitted in industrialised countries to the remote regions of the Arctic takes place. Additionally, the use of imported industrial products in the Arctic has also been indicated to lead to a certain emission of pollutants.
Up to now the main focus in this context has been on strongly hydrophobic organic pollutants such as polychlorinated biphenyls (PCBs) and chlorinated pesticides. Due to the hydrophobic character of the compounds they will primarily be linked to organic matter of similar polarity and thus be likely to concentrate in sediment and/or to accumulate in animal lipids. The compounds have been found to bioaccumulate in animals and the highest levels are found in the top predators of the Arctic. As marine food, including tissues from the top predators, contributes significantly to the diet of many people in the Arctic, humans are exposed to a high intake of organochlorines and metals, which may affect their health.
However, other groups of organic chemicals could also be of concern in the Arctic. Brominated and fluorinated compounds are examples. These compounds have many physical and chemical properties in common with their chlorinated counterparts and can therefore be expected to increase in the Arctic environment in the same manner as the cloinated substances. Some compounds might be more persistence at higher latitudes than further south. This may be a problem if the compounds are toxic of very low concentrations.
In this chapter levels of a range of compounds not previously included in the Danish AMAP programme are discussed. The groups of contaminants considered are not new strictly speaking. The group of polycyclic aromatic hydrocarbons (PAHs) has for instance been analysed in environmental samples from the Temperate Zone for the last thirty years but data from the Arctic are sparse. Other compounds have not been analysed previously in samples from Greenland.
5.2 Tributyltin (TBT)
In the late 1960’ies tributyltin (TBT) replaced cupric oxides as the most used active component in antifouling paints. Already around 1970 the compound was discovered to cause morphological changes in non-target benthic fauna. First abnormal shell thickening in pacific oyster was observed and later the phenomenon called imposex in a range of neogastropods was seen. Endocrine disruption caused by organotins has also been observed in fish, birds and mammals. Organotins also show bioaccumulative properties. High levels (mean concentration: 1400 µg/kg ww) have e.g. been measured in livers of bottlenosed dolphins (Tursiops truncatus) stranded along US Atlantic and Gulf Coasts (Kannan et al. 1997). The atmospheric TBT deposition is generally negligible due to the physical-chemical properties of these compounds (Arnold et al. 1997, Fent 1996) and thus aquatic transport processes are considered to be the main pathways.
Jacobsen and Asmund (2000) have conducted a study at Nuuk in 1997, where concentrations of butyltin compounds were investigated in blue mussels (Mytilus edulis) (five sites) and marine sediments (three sites).
Figure 5.2.1 Map of the area around Nuuk, Greenland. The mussel sampling stations are marked (*) and the sediment stations by (•). The dotted line marks the main shipping route in the area.
Possible sources in the Nuuk area would mainly be shipping, but also wastewater and effluents from the shipyard should be taken into consideration. The sampling positions are described in Figure 5.2.1 and Table 5.2.1 and 5.2.2. Blue mussels were sampled at low tide by hand, while sediments were sampled with a HAPS gravity stainless steel box corer having an internal diameter of 13.4 cm, and the upper one-cm slice was collected by use of a stainless steel kitchen knife.
The samples were extracted and analysed by the NERI standard method described in detail by Jacobsen et al. (1997) and in the AMAP, Greenland 1996-2001, Data Report. The organotin concentrations were determined by GC-PFPD (Gas Chromatography – Pulsed Flame Photometric Detector). The limit of detection for TBT was around 0.1 µg/kg ww for biota and 1 µg/kg dw for sediments. In this section all concentrations are presented as tin (Sn) in order to facilitate the comparison between TBT and degradation products.
Results and Discussion
The measured organotin concentrations in the collected bivalves are shown in Table 5.2.1, which also includes background information as distance to Nuuk, number of blue mussles collected and the mean shell length.
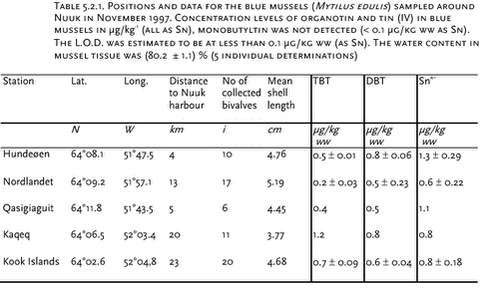 Click on the picture to see the html-version of: ‘‘Table 5.2.1‘‘
The blue mussels all contained TBT levels between 0.2 and 1.2 µg/kg ww and DBT levels between 0.5 and 0.8 µg/kg. It was noteworthy that blue mussels 20 km from Nuuk harbour had higher levels of TBT compared to blue mussels closer to Nuuk. This is suggested to relate to the shipping route in the vicinity of the sampling stations and thus TBT is very well distributed in this particular area.
In Iceland Skarphedinsdottir et al. (1996) found a body burden of 5.9 ± 5.3 µg/kg ww (as Sn) in blue mussels far from the Icelandic capital Reykjavik. This concentration represents a seasonal maximum in June / August. Near Reykjavik the concentration increased to 49 ± 37 µg/kg ww. Thus the concentrations found near Nuuk are 40 to 70 times lower than the concentrations found near Reykjavik.
A TBT study around the Faeroe Islands by Følsvik et al. (1998) showed concentrations ranging from 55 µg/kg ww near the capital Thorshavn to 8 - 12 µg/kg ww in remote places. These concentrations are comparable to findings in Iceland in autumn, but higher than found in spring when the concentrations near Reykjavik were app. 4 µg/kg ww. In comparison, the concentration of TBT in sediments in Danish waters were found to range from 1 µg/kg dw in open waters to 16 000 µg/kg in highly polluted harbour sediments (Mortensen et al.1996; Jacobsen et al.1998).
The organic content of the sediment samples was characterised by determining the loss of ignition at 550 °C. Station 1 and 2 are inside the harbour of Nuuk whereas station 3 is approximately 4 km from the harbour (Figure 5.2.1 and Table 5.2.2). The highest concentration of TBT was 171 ± 32 µg/kg dw found in the harbour of Nuuk at station 2, where also the organic content was highest (9.3 %). At station 1 within the port, the TBT level was 9.7 ± 3.4 µg/kg dw and the organic content 1.3 % (sandy sediment). At station 3 (Hundeøen) the levels were below 1 µg/kg dw.
Recently a second study of TBT concentrations in blue mussels from the westcoast of Greenland was conducted (Strand & Asmund, 2002). The focus of this study was on the difference in organotin concentrations between locations inside and outside harbours. Levels outside Nuuk in this study were comparable to those found before, while the TBT level in mussels inside the habour of Nuuk were about 20 times higher. This pattern with relatively high TBT levels inside harbours and low levels outside harbours and in open water stations was shown at all six areas investigated along the west coast. TBT levels inside Greenland harbours were comparable to levels found in coastal waters in more urbanized parts of the world. The TBT levels measured in blue mussels were compared to levels of imposex detected in neogastropods at the same stations. This revealed TBT levels inside harbours to be high enough to induce imposex in neogastropods, while the levels in open waters are generally below levels where such effects can be found.
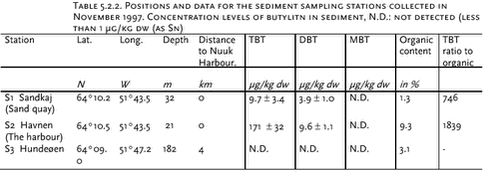 Click on the picture to see the html-version of: ‘‘Table 5.2.2‘‘
More samples are needed to elucidate the TBT contamination level in more remote areas of Greenland, especially also including samples from the east coast. A major drawback in the reviewed studies is that sediment and mussels sampling stations are not coherent. The concentrations of degradation products (such as DBT) in the samples from Greenland were low possibly due to slow degradation rates at the low temperatures in the Arctic.
Conclusions and recommendations
TBT and degradation products were detected in the marine Greenland environment. The concentrations inside harbours along the westcoast were comparable to concentrations measured elsewhere in the world, and likely to cause adverse biological effects. Concentrations measured outside the harbour area at Nuuk both in blue mussels and in sediments were much lower than found in Iceland and on the Faroe Islands.
Further screening of TBT baseline levels away from point sources is recommended, possibly in combination with effect studies, and including stations at the east coast. Considering the potential for bioaccumulation of TBT, the occurrence of TBT in the low trophic levels emphasises the importance of investigating other biological samples in the Arctic food web, especially top predators such as marine mammals.
5.3 Toxaphene
Toxaphene is a chlorinated pesticide consisting of a complex mixture of polychlorobornanes. Theoretically, there are over 30 000 possible chlorobornane isomers. Technical toxaphene, which mainly consists of C7 – C9 chlorobornanes could contain at least 6840 congeners (Muir & de Boer, 1996). Analysis of toxaphene is difficult because of the mixture’s complexity. Toxaphene is very persistent in the environment and is globally dispersed via atmospheric transport, as are several other chlorinated pesticides.
Appendix C shows the data available of toxaphene in the Greenland environment. Data are derived from the following AMAP projects: “Contaminants in Greenland human diet”, “Biological core programme”, “Temporal trend programme” and ”Population structure of West Greenland narwhals, a multidisciplinary approach”. Data of toxaphene in walrus published in Muir et al. (2000b) is also shown for comparative reasons. Several analytical laboratories have been involved. In some cases total toxaphene has been determined, while others are based on selected toxaphene congeners. Analysis of single congeners is the most precise method.
Levels
Toxaphene concentrations in biota from the terrestrial environment (ptarmigan, hare, lamb and musk ox) were low (range <0.1 – 2.6 µg/kg ww). In the marine environment the highest total toxaphene concentrations were found in narwhals (range in blubber 2033 to 8825 µg/kg ww). In monodontiids (beluga and narwhal) toxaphene is reported to be the most dominant OC (Muir et al. 1999). This was also detected in samples from Greenland. In minke whale, walrus and ringed seal blubber, the total toxaphene concentration ranged between 219 and 369 µg/kg ww, lowest in ringed seal and highest in minke whales (Appendix C). In seabird liver the total toxaphene concentrations ranged between 13.5 µg/kg ww in common and king eider and 53.0 µg/kg ww in thick-billed murre. Total toxaphene was not determined in black guillemot, only single congeners. Based on the concentrations of these congeners, the highest concentrations in seabirds were found in black guillemot (Appendix C). A few livers of marine fish sampled in southwest Greenland were analysed for some toxaphene congeners (Appendix C). The highest concentrations of CHB-50 (the dominant congener) was found in liver of Atlantic cod (62.9 µg/kg ww) and the lowest in liver of shorthorn sculpin (14.2 µg/kg ww). The concentration of CHB-50 in marine fish was in the same order of magnitude as found in seabirds. The lowest concentration of toxaphene congeners in the marine biota was found in blue mussels (Appendix C).
Compared to other Arctic regions, the toxaphene concentrations in blubber of ringed seals from Greenland were comparable to the concentrations found in the Canadian Arctic, but higher than concentrations in seals from Svalbard (Cleemann et al. 2000a). Toxaphene concentrations in walrus from Avanersuaq were at the same level as found in the Canadian Arctic (Muir et al. 1999). In walrus from Ittoqqortoormiit the toxaphene concentrations were higher (also the case for other persistent organochlorines) than in Avanersuaq, but at a similar level as walrus from Inukjuak (east Hudson Bay, Canada) (Muir et al. 1999).
Sex differences
Possible differences between toxaphene concentrations in males and females were tested in cases of adult black guillemot sampled in Qeqertarsuaq 1999 and 2000 and in Ittoqqortoormiit in 2000 and of ringed seals sampled in Qeqertarsuaq and Ittoqqqortoormiit in 2000. The test was performed as a t-test on logarithmic transformed lipid normalised data for each toxaphene congener separately (CHB-26, 40, 41, 44 (only black guillemot), CHB-50 and 62). No differences between males and females were found in the case of black guillemot from Qeqertarsuaq 1999 and 2000 and for ringed seals from Qeqertarsuaq and Ittoqqortoormiit 2000, except for CHB-62, which was significantly higher in females. In black guillemot from Ittoqqortoormiit 2000 the concentrations of all congeners except CHB-41 were significantly (p<0.05) higher in females than in males.
Figure 5.3.1 shows the relationship between total toxaphene and age, represented by number of growth layers, in narwhals from west Greenland. The relationship of toxaphene with age was also typical for other OCs such as ΣPCB, HCB, ΣHCH and DDT (Riget et al. 2002). Toxaphene concentrations in females showed a decreasing trend from the yearlings to an age of about 8 to 10 years. Animals between 10 to 15 years showed an increasing concentration. In males toxaphene showed a decrease in the first few years of life followed by a gradual increase with increasing age. The lower levels of toxaphene in adult females compared to younger females and adult males are common in cetaceans and pinnipeds because of elimination of these lipophilic compounds via lactation (e.g. Addison & Smith, 1974). The increase from 10 years to 15 years was therefore unexpected. However, high levels in only 3-4 individuals created this pattern.
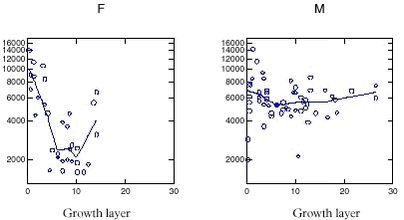 Figure 5.3.1. Relationships between total toxapene concentrations (µg/kg l.w.) and age (Growth Layer Groups) in narwhals from west Greenland. Lines represent LOWESS smoother. F=females, M=males
Area differences
Possible differences between toxaphene concentrations in west (Qeqertarsuaq) and east (Ittoqqortoormiit) were tested for black guillemot and ringed seal sampled in 2000. The test was performed as a t-test on logarithmic transformed lipid normalised data. The test was done for each toxaphene congener and sex separately (CHB-26, 40, 41, 44 (only black guillemot), CHB-50 and 62). No significant (p<0.05) differences were found in case of female black guillemot and female ringed seal between west and east. But in male black guillemot the concentration of toxaphene congeners CHB-26, 40, 41 and 50 and in male ringed seal the concentrations of all congeners except CHB-40 were significantly higher in east Greenland than in west Greenland.
The somewhat higher toxaphene concentrations in east Greenland than in west Greenland found in this study confirmed the previous findings in AMAP phase I of higher toxaphene concentrations in blubber of ringed seal and muscle of Arctic char from east Greenland than in the same species from west Greenland (Cleemann et al. 2000a, 2000b). A similar geographical pattern has been reported in walrus from east and west Greenland (Muir et al. 2000b)
In the study ”Population structure of West Greenland narwhals”, no geographical differences in total toxaphene concentrations among four locations in west Greenland were found (Riget et al. 2002).
Conclusions and recommendations
Toxaphene concentrations in the Greenland marine biota were within the range observed in other Arctic areas. Toxaphene levels in Greenland terrestrial biota were lower than in marine biota. The highest toxaphene levels were found in marine mammals especially narwhal and beluga. Only few data exist to form the basis of an assessment of temporal trends of toxaphene levels in Greenland biota. Trend studies in single key-species could reveal if the ban of toxaphene at lower latitudes leads to a decrease in toxaphene levels in the Greenland environment
5.4 Dioxines and furanes (PCDD/Fs)
Polychlorinated dibenzo-p-dioxins and furans (PCDD/Fs) are formed as by-products in industrial processes or by incinerations and are highly toxic, especially the 2,3,7,8-TCDD congener. Most PCDD/F congeners are extremely hydrophobic and resistant to biodegradation. Some congeners are known to bioaccumulate, while vertebrates readily degrade others (AMAP, 1998).
Dioxines and furans have been analysed in liver and blubber of polar bears sampled in Ittoqqortoormiit in the project “Effects of Contaminants in the Greenland Sea Polar Bear”. The National Water Research Institute, Canada conducted the chemical analyses. The following congeners were measured:
dioxins |
furans |
2,3,7,8 TCDD |
2,3,7,8 TCDF |
1,2,3,7,8 PCDD |
1,2,4,6,8 PCDF |
1,2,3,4,7,8 HxCDD |
1,2,4,7,8 PCDF |
11,2,3,6,7,8 HxCDD |
1,2,3,7,8 PCDF |
1,2,3,7,8,9 HxCDD |
2,3,4,7,8 PCDF |
1,2,3,4,6,7,9 HpCDD |
1,2,3,4,7,8 HxCDF |
1,2,3,4,6,7,8 HpCDD |
1,2,3,6,7,8 HxCDF |
1,2,3,4,6,7,8,9 OCDD |
1,2,3,7,8,9 HxCDF |
|
1,2,3,4,6,7,8 HpCDF |
1,2,3,4,7,8,9 HpCDF |
1,2,3,4,6,7,8,9 OCDF |
The total dioxins and furans levels were at the same level as found in polar bears from Arctic Canada (Norstrom & Muir, 1994). The dominant dioxin congener was HxCDD (Table 5.4.1), while in the Canadian polar bears TCDD and OCDD dominated (AMAP 1998).
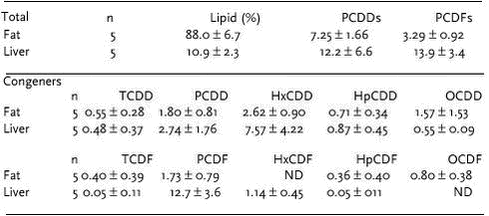 Table 5.4.1. Total dioxins (PCDDs) and furans (PCDFs) concentrations (ng/kg ww) and cogeners in five polar bears sampled in Ittoqqortoormiit 1999/2000.
The levels of dioxins and furans in polar bears from east Greenland were low, measured as WHO toxicity equivalents 2.85 ng/kg ww in fat and 4.33 ng/kg ww in liver. However, it should be noted that only few data are avilable from Greenland.
Conclusions and recommendations
Dioxins and furans were detected in polar bears. Compared to marine mammals in other Arctic regions the concentrations were relatively low. Further investigations regarding sources of these compounds and concentrations at lower levels in the foodchain is recommended, taking the high potential for long-term detrimental effects of these compounds into account.
5.5 Coplanar PCBs (nPCB)
Coplanar PCBs are PCB congeners with 3,3’,4,4’ chlorine substitutions. The lack of chlorine substituents in the 2 and 6 positions permits nPCBs to form planar configurations similar to that of polychlorinated dibenzo-p-dioxins and dibenzofurans. The coplanar PCBs are the most toxic PCB congeners and exhibit biochemical and activity much comparable to the activity of the dioxin conger TCDD.
Coplanar PCBs (PCB 77, 126 and 169) were analysed in muscle and blubber of ringed seal and liver and blubber of polar bears. The samples were from Ittoqqortoormiit, measured as part of the projects “Effects of Contaminants in the Greenland Sea Polar Bear” and “Contaminants in Greenland human diet”.The National Water Research Institute, Canada conducted the chemical analyses.
Very low concentrations of PCB 77, 126 and 169 were found in ringed seal muscle (mean toxic equivalents 0.13 ng/kg ww, n=5 (Muir & Johansen, unpubl.)) and in blubber (mean toxic equivalents 5.6 ng/kg ww, n=10 (Muir & Johansen, unpubl.)).
The concentrations of coplanar PCBs congener in polar bears (as WHO TEQ) were higher (6.33 ng/kg ww for fat and 5.67 ng/kg ww in liver) than the concentrations of dioxin congeners indicating that coplanar PCBs are more important than dioxins and furans from a toxicological point of view. Compared to ringed seals and cetaceans from Arctic Canada, the concentrations in polar bears from East Greenland were lower (AMAP, 1998).
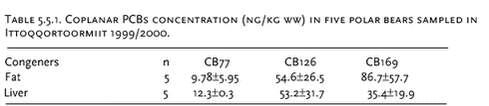 Click on the picture to see the html-version of: ‘‘Table 5.5.1‘‘
Conclusions and recommendations
Coplanar PCBs were detected in ringed seal and polar bears from east Greenland. The coplanar PCBs contributed relatively more to total toxicity, measured as WHO TEQ, than dioxins and furans (PCDD/F). Compared to marine mammals in other Arctic regions the concentrations were relatively low. Taking the high potential for long-term detrimental effects of these compounds into account further monitoring in key species is recommended.
5.6 Chlorobenzene (CBz)
Chlorobenzenes are cyclic aromatic compounds formed by the addition of 1-6 atoms of chlorine to the benzene ring. Chlorobenzenes are used as intermediates in the synthesis of pesticides and other chemicals. 1,4-DCB is used in industrial odor control and as a moth repellant. The higher chlorinated benzenes (TCBz and 1,2,3,4-TeCBzs) have been used as dielectric fluents. Hexachlorobenzene, HCB was used as a fungicide on grains, especially wheat. The release of chlorobenzenes to the environment occurs primarily during manufacture and through the dispersive nature of their uses. In general the water solubility of chlorobenzene compounds is medium to low decreasing from 293 to 0.83 mg/L with increased chlorination. Chlorobenzenes released to the aquatic environment will evaporate (boiling points in the range 132 – 277 oC), deposite in the sediment or metabolize. Chlorobenzenes are transformed mainly by photodegradation or microbial degradation, which takes place under aerobic conditions. Chlorobenzenes tend to accumulate in tissues with a high fat content due to the lipophilic nature of the compounds expresssed by log KOW in the range 2.98 – 5.03. They are generally only moderately toxic to wildlife and man, but effects on the liver, kidneys, adrenal glands, mucous membranes, brain ganglion cells and metabolising enzymes have been observed following acute exposure to non-lethal doses. Some chlorobenzenes have carcinogenic and teratogenic properties (reviewed in WHO 1991a).
Data are derived from the following AMAP projects: “Contaminants in Greenland human diet”, “Biological core programme”and “Temporal trend programme”. The National Water Research Institute, Canada conducted the chemical analyses of chlorobenzenes (1,2 DCB, 1,3 DCB, 1,4 DCB, 1,3,5 TCB, 1,2,4 TCB, 1,2,3 TCB, 1,2,3,4 TTCB, PeCB, HCB).
Appendix D shows the concentrations of higher chlorinated benzene (tetra-, penta and hexachlorobenzene) in the Greenland environment. In the terrestrial environment sCBz (sum of tetra- and penta and hexachlorobenzene) concentrations ranged from 0.17 ng/g ww in lamb muscle to 16.5 ng/g ww in musk ox muscle. The dominant chlorobenzene was HCB constituing 80-90%, except in ptarmigan liver where TeCB dominated (53%). In landlocked Arctic char muscle sCBz were low (range 0.38-0.91 ng/g ww) with HCB as the dominating chorobenzene (app. 94%).
In marine invertebrate (snow crab and shrimp), sCBz concentrations were also low (maximum 3 ng/g ww in crab hepatopancreas) and the dominating chlorobenzene was HCB, except in shrimp muscle where TeCB dominated. In marine fish, the highest sCBz concentrations were found in liver of Greenland halibut (45 ng/g ww) and in liver of shorthorn sculpin (13 ng/g). In shorthorn sculpin, higher levels were found in fish from east Greenland (Ittoqqortoormiit) than in fish from west Greenland (Qeqertarsuaq). HCB was also here the dominating chlorobenzene (81-90%). No bioaccumulation of chlorobenzene from fish to seabirds was observed, the highest sCBz concentrations were found in liver of Greenland halibut (45 ng/g ww) and in liver of shorthorn sculpin (13 ng/g). In shorthorn sculpin, higher levels were found in fish from east Greenland (Ittoqqortoormiit) than in fish from west Greenland (Qeqertarsuaq). HCB was also here the dominating chlorobenzene (81-90%). No bioaccumulation of chlorobenzene from fish to seabirds was observed, the highest levels were found in liver of thick-billed murre liver (6.5 ng/g ww). In marine mammals, the highest sCBz concentrations were found in blubber of marine mammals, especially narwhal and beluga (mean 462 and 260 ng/g ww, respectively). HCB was the dominating chlorobenzene (53% in ringed seal kidney - 96% in narwhal blubber).
In general, the CBz levels in terrestrial Greenland species appear higher than in similar species from Canada, whereas the levels in freshwater and marine Greenland species appear lower than in similar species from Canada (AMAP, 1998).
Conclusions and recommendations
The highest chlorobenzene concentrations were found in blubber of narwhal and beluga. The far dominating chlorobezene in Greenland biota is hexachlorobenzene (HCB). Continued monitoring of the levels of this compound in top predators in Greenland are recommended in order to evaluate the time trend of concentration levels.
5.7 New chlorinated pesticides
This section deals with heptachlor and heptachlor epoxide, dieldrin, aldrin, endrin, endosulfan, metoxyclor and mirex. These compounds are not used in Greenland and are found mainly due to long-range transport from lower latitudes. The pesticides heptachlor, aldrin, dieldrin and endrin together with mirex are banned worldwide but are still found in considerable concentrations due to high persistence in the environment. Endosulfan and methoxychlor belong to a newer class of less persistent chlorinated pesticides. Information about their use, chemical proporties, environmental fate and toxicity are shown in Box 5.7.1.
Data are derived from the following AMAP projects: “Contaminants in Greenland human diet”, “Biological core programme”and “Temporal trend programme”. The National Water Research Institute, Canada conducted the chemical analyses of these compounds, except for ringed seals from Avanersuaq in 1998, which was conducted by the National Wildlife Research Center, Canada.
Aldrin and Dieldrin Aldrin is readily metabolised to dieldrin in plants and animals. As their mode of action and their acute and chronic toxicity also is similar, national and international regulatory bodies usually consider them together. Aldrin and dieldrin are organochlorine insecticides, which were used from the 1950s to the early 1970s to control soil pests and to treat seeds. Since the 1970s, a number of countries have severely restricted or banned aldrin and dieldrin from use in agriculture (WHO, 1989).
Both compounds are stable unless attacked by oxidising agents and concentrated acids. Dieldrin is more resistant to biodegradation than aldrin (WHO 1989). Aldrin and dieldrin are highly toxic for aquatic organisms, with 96-h LC50-values below 50 µg/L for crustaceans and about 1-40 µg/L for fish (WHO ,1989). Both aldrin and dieldrin are described as “possibly carcinogenic to humans” on the US-EPA’s list of chemicals evaluated for carcinogenic potential, and they are both identified as endocrine disrupters.
Both compounds are virtually insoluble in water. Their log KOW values are 7.2 and 6.2 for aldrin and dieldrin, respectively, indicating high lipophilicity (Verschueren, 1996). Volatilisation appears to be the main route of loss from soil, though atmospheric levels of dieldrin and aldrin are low (WHO, 1989). Aldrin bioaccumulates mainly as its degradation products. The bioconcentration of dieldrin in aquatic organisms is principally from the water rather than by ingestion of food (WHO, 1989).
Endrin Endrin is an organochlorine insecticide of high acute toxicity and chemically related to aldrin and dieldrin, being the stereoisomer to dieldrin. It has been produced since 1950 and was used throughout the world up to the early 1970s, when it was restricted or even banned in several countries (WHO, 1991b). In the USA, production and sale of endrin stopped in 1986 (ATSDR, 1997). Endrin was used to control a range of pests, particularly Lepidoptera on cotton (WHO, 1991b).
Endrin is practically insoluble in water and is retained in soils with a high organic matter content. Its persistence can reach up to 12 years. Volatilisation, photodegradation and microbial transformation can take place to reduce endrin residues in soils. It is mainly transported into the aquatic environment by run-off processes. Aquatic invertebrates and fish will rapidly take it up. Endrin is metabolised by animals, and the accumulation in lipids is low (WHO, 1991b).
Endrin has a high acute toxicity. It affects the liver and the central nervous system and causes neurotoxic effects. A number of metabolites have even higher acute toxicity, for instance 12-ketoendrin (about 1 mg/kg body weight) (WHO, 1991b).
Endosulfan Endosulfan is an organochlorine insecticide of the cyclodiene group. It has been used in all branches of agriculture since 1954 (Gupta & Gupta, 1979). Today, its application is prohibited in a number of countries, but is still in use in e.g. Spain and the United States (Aguilera-del Real et al. 1997, Antonius & Byers, 1997).
The technical product is comprised of 70 % alpha-endosulfan and 30 % beta-endosulfan, with alpha being more toxic than beta. The high acute toxicity are directed towards the central nervous system. In spite of its hydrophobicity (log KOW=4.7), endosulfan does not accumulate in organisms. However, it is very toxic to fish and other aquatic organisms and caused a massive fish killing in the river Rhine as the consequence of an accidental discharge (Greve & Wit, 1979; Gill et al. 1991).
Endosulfan is readily hydrolysed to endosulfan-diol or oxidised to endosulfan-sulphate in the environment. The metabolites, endosulfan-sulphate, was found to be more toxic than the parent compounds (Dubey et al. 1984).
Metoxychlor Methoxychlor is an organochlorine insecticide still in use to protect vegetables, fruits, trees, farm animals and pets against a variety of pests (US-EPA, 1988). It has a low solubility in water (0.1mg/L at 25°C) and a log KOW around 3-4.3. In water, methoxychlor is adsorbed onto suspended solids and sediments. It may be ingested and bioaccumulated by some aquatic organisms, but significant and persistent accumulation has not been shown. In soil it is mainly degraded anaerobically, with half-lives from 1 week to 2 months, while the aerobic degradation results in half-lives of more than 3 months. Animal tests indicated reproductive toxicity and a carcinogenic potential of methoxychlor.
Heptachlor Heptachlor is an organochlorine cyclodiene insecticide. During the 1960s and 1970s, it was used primarily by farmers to kill termites, ants, and soil insects in seed grains and on crops, as well as by exterminators and home owners to kill termites. Heptachlor about 10% of the pesticide formulation chlordane, discussed in preceeding chapters. The half-life of heptachlor in the environment is in the range of days to a few weeks. An important metabolite of heptachlor is heptachlor epoxide, which is an oxidation product formed from heptachlor by bacteria and many plant and animal species. Heptachlor is a moderately toxic compound in EPA toxicity class II. In 1988, the EPA canceled all uses of heptachlor in the U.S. The only commercial use still permitted is for fire ant control in power transformers. Heptachlor is still available outside the U.S.
Mirex Mirex was used as an insecticide and fire retardant, mainly in the United States and Canada, until 1978. Mirex is considered to be one of the most stable and persistent pesticides in soil, sediment, and water, with a half life in soil of up to 10 years. A log Kow of 7,4 indicates mirex will strongly adsorb to organic materials. For most part mirex is resistant to biological and chemical degradation. Photolysis of mirex may occur, especially as mirex is relatively volatile. Mirex is highly fat-soluble and has a high acute toxicity to aquatic organisms, exemplified by a LC50 of 0,1-1,0 ppb for chrustaceans. There is indication of cancerogenic and reproductive effects in laboratory animals.
| Box 5.7.1. Use, chemical proporties, environmental fate and toxcity
Results and discussion
Table 5.7.1 summarises levels of organochlorine pesticides in various terrestrial animals from different places in west Greenland. Higher concentrations were found in liver and kidney, compared to fat and muscle tissues. Levels are furthermore much lower than found in marine organisms. This phenomenon might be due to the lower trophic level of the terrestrial species analyzed.
Heptachlor epoxide, dieldrin and mirex concentrations were below detection limits for musk ox and hare. Levels of dieldrin and mirex in ptarmigan were at the same level as reported for orther Arctic regions in AMAP phase I. Levels of methoxychlor and endosulfan have not been reported in terrestrial animals from other places in the Arctic. Even though levels are generally low, the occurrence of these substances in the Greenland environment is noteworthy as these chemicals have not been used in Greenland, but are transported from lower latitudes - even endosulfan and methoxychlor which are much less persistant than the “old” OCs,
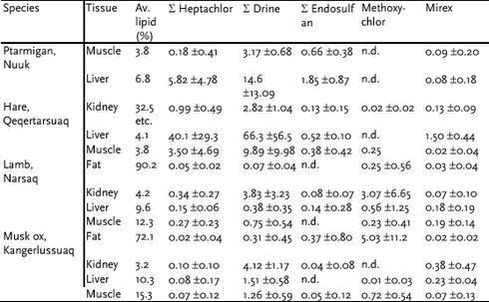 Table 5.7.1 Levels of organochlorine pesticides (ng/g lipid) in terrestrial organisms from Greenland, 1999. ∑Drine = Aldrin+Dieldrin+Endrin, Heptachlor = Heptachlor + Heptachlor epoxide, ∑Endosulfan=alfa+beta endosulfan, n=5 for all matrices
In Table 5.7.2 levels of chlorinated pesticides in whales from west Greenland are presented. Levels of mirex in beluga from the Canadian Arctic were higher than seen from Greenland, while the opposite was the case for dieldrin and heptachlorepoxide, the latter were approximately 5 times lower in this study than in belugas from the Canadian Arctic (Metcalfe et al. 1999, Muir et al. 1996). In an earlier study by Metcalfe et al. (1999), the distribution of mirex in beluga from the Canadian Arctic was somewhat different from the pattern seen in this study. Metcalfe (ibid) found higher concentrations in liver than in muscle tissue, the opposite pattern seen in Greenland.
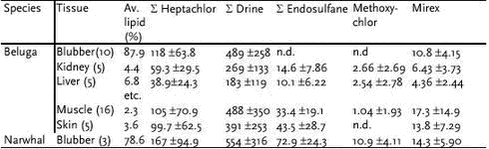 Table 5.7.2 Levels of organochlorine pesticides (ng/g lipid) in whales from Nuuk, west Greenland 1999. ∑Drine = Aldrin+Dieldrine+Endrin, ∑Heptachlor=Heptachlor+Heptachlor epoxide, ∑Endosulfan=alfa+beta endosulfan
In Table 5.7.3 pesticide levels in ringed seals from Greenland regions are summarized. As earlier reported for other OCs, levels were generally higher on the east coast compared to the west coast and levels in males are generally higher than in females (cf. Figure 5.7.1). This pattern is often reported for bioaccumulating contaminants as females excrete a part of their body load to their offspring.
Mirex was in the same range as reported for female seals in Canada 6.7 µg/kg ww and 22.9 µg/kg ww for female and male, respectively. Dieldrin was not measured in the present study and therefore the sums of drine in Table 5.7.3 includes only the sum of aldrin and endrin. For dieldrin mean values found in the Canadian Arctic were 36.5 and 34.9 in female and male seals, respectively – these values were twice as high in male seals from Avernarsuaq (Cameron et al. 1997). Levels of dieldrin and mirex were also measured in different areas of the Arctic during AMAP phase I. Dieldrin levels in blubber were in the range 13.6 to 187 µg/kg, while mirex was found in concentrations of 3.43 to 26.1 µg/kg.
Figure 5.7.1 Levels of chlorinated pesticides in seals from Qeqertarsuaq, west and ittoqqortoormiit, east Greenland. F and M denotes female and males, respectively
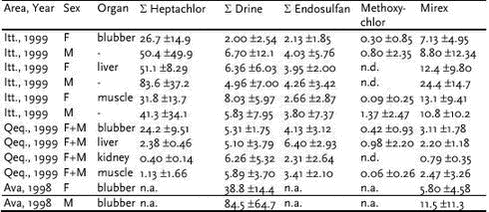 Table 5.7.3 Levels of organochlorine pesticides (ng/g lipid) ringed seals from Greenland. ∑Drine = Aldrin+Dieldrin+Endrin, ∑Heptachlor=Heptachlor+Heptachlor epoxide, ∑Endosulfan=alfa+beta endosulfan. Itt=Ittoqqortoormiit, Qeq=Qeqertarsuaq, Ava=Avannersuaq.
In Table 5.7.4 levels in birds from the Nuuk area are shown. The most interesting result is probably the higher levels of endosulfan and mirex found in kittiwake compared to the other species in the area, which probably is a reflection of migration patterns but could also reflect interspecific differences in metabolic capacities.
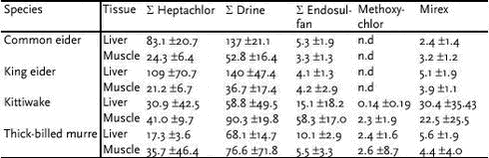 Table 5.7.4 Levels of organochlorine pesticides (ng/g lipid) in seabirds from Nuuk, western Greenland, 1999. ∑Drine = Aldrin+Dieldrin+Endrin, ∑Heptachlor=Heptachlor+Heptachlor epoxide, ∑Endosulfan=alfa+beta endosulfan, n=5 for all matrices
Only few data are available of concentrations of these chlorinated pesticides in adult birds from the Arctic as well as from lower latitudes. In AMAP phase I, levels of dieldrin, mirex and heptachlor epoxide were reported for a few liver samples of kittiwake, king eider and thickbilled murre from the Russian Arctic. Dieldrin was not found in any of these samples while levels of mirex were 0.21, <0.01-0.07, <0.01-0.67 µg/kg ww for kittiwake, king eider and thickbilled murre, respectively. For the last two species this is in the same range as measured in the Nuuk area, while kittiwake from Nuuk shows concentrations of mirex up to 20 times the level reported from Russia. Heptachlor epoxide was not detected in king eider from Russia while levels in thick billed murre were in the range 0.44-1.49 µg/kg ww which is in the same range as reported in this study. Levels in kittiwake were 3.36 µg/kg, which is similar to levels in kittiwake from Nuuk (AMAP, 1998).
Mirex has later been analysed in muscle tissue from glaucous gulls (Larus hyperboreus) from Bjørnoya, Norway, and concentrations were 1.162 ± 0.931 µg/kg lw (Skagerup et al. 2002). This is lower than found in the area of Nuuk. Of all birds analysed during AMAP phase I only two cases were higher for mirex than reported here, namely levels of 253 and 40.8 µg/kg ww found in glaucous gulls and northern fulmar (Fulmaris glacialis) from the Russian Arctic (AMAP, 1998).
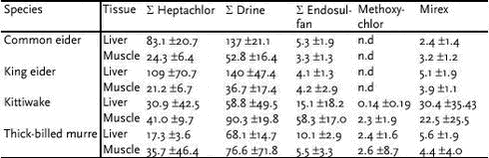 Table 5.7.4 Levels of organochlorine pesticides (ng/g lipid) in seabirds from Nuuk, western Greenland, 1999. ∑Drine = Aldrin+Dieldrin+Endrin, ∑Heptachlor=Heptachlor+Heptachlor epoxide, ∑Endosulfan=alfa+beta endosulfan, n=5 for all matrices
In Table 5.7.5 the levels of organochlorine pesticides in various fish species are summarized. In AMAP phase I, levels of dieldrin, mirex and heptachlor epoxide were measured in muscle tissue of Arctic char from the Canadian Arctic – levels of dieldrin found here were generally one order of magnitude higher than found in this study while heptachlor epoxide and mirex were in the same range. Levels of mirex and dieldrin earlier measured in the Canadian Arctic in the marine species Greenland halibut and sculpin were higher or in the same range as the data presented here.
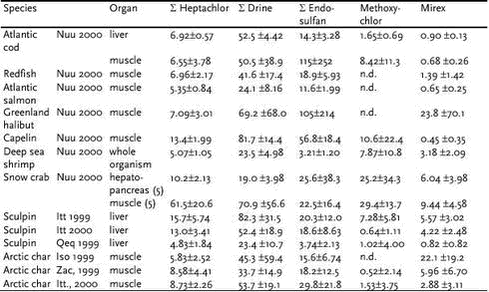 Table 5.7.5 Levels of organochlorine pesticides (ng/g lipid) in fish from Nuuk, western Greenland, 2000. ∑Drine = Aldrin+Dieldrin+Endrin, ∑Heptachlor=Heptachlor+Heptachlor epoxide, ∑Endosulfan=alfa+beta endosulfan. Nuu=Nuuk, Itt=Ittoqqortoormiit, Iso=Isortoq, Zac=Zackenbergt.
The levels in both sculpin and char show higher levels in east than in west Greenland (c.f. Figure 5.7.1), a tendency that is well described for the “old” OCs. Furthermore there is a tendency of higher levels in male fish compared to female, a picture often seen in mammals.
Figure 5.7.2 l evels of chlorinated pesticides (µg/kg lw + std) in sculpin from Qeqertarsuaq (west Greenland) and ittoqqortoormiit (Greenland).
Conclusions and recommendations
Except for methoxychlor all chlorinated pesticides analysed were found in all types of organisms included in this study, showing a tendency of bioaccumulation in the same manner as seen for the OCs traditionally analysed in the Arctic. This is in accordance with results from other parts of the Arctic. The chlorinated pesticides described here can be transported to Greenland only by long range transport. The highest levels of aldrin, dieldrin, endrin, heptaclor, endosulfan, methoxychlor and mirex were comparable to levels detected elsewhere in the Arctic. Data on levels of endosulfan and methoxychlor, two chlorinated pesticides still in use, in Arctic biota are sparse. The concentrations were lower than found in more industrialized parts of the world.
Very few data exist to make an assessment of temporal trends of chlorinated pesticides in Greenland biota, therefore it is recommended that levels are continuously monitored in key species of higher trophic level.
5.8 Polybrominated diphenyl ethers (PBDE)
Brominated flame retardants (BFRs) are a chemically diverse group of brominated organic compounds used in plastics, textiles, electronic circuity and other materials to prevent fire. Some BFRs like polybrominated diphenyl ethers (PBDEs) are additives mixed into polymers, not chemically bound to the matrix and can therefore leach from the surfaces of the products into the environment. PBDEs are insoluble in water (9x10-7 mg/L at 20oC), highly lipophilic (log Kow 6,64-6,97) and have some structural similarities to PCB and PCDD/F. Annual worldwide production is 40 000 t. Bromkal 70-5DE is one of several technical products that contains lower brominated PBDEs such as 2,2’4,4’-TeBDE (BDE-47) and 2,2’,4,4’,5-PeBDE (BDE-99). Among the PBDE congeners found in the environment BDE-47, BDE-99 and BDE-100 usually make up approximately 90 % of the total level of PBDEs in aquatic biota (Janson et al. 1993; Asplund et al. 1999) and BDE-47 constitute more than 60% of the total level (Jansson et al, 1993).
Several time trend studies exist of PBDE levels in various environmental compartments: sediments, fish, beluga and human samples indicate a rapid increase in PBDE levels during the last 20 years (Nylund et al. 1992, Luross et al. 2000, Ikonomou et al. 2002, Norén & Meironyté, 2000). In the study by Norén & Meironyté (2000) a nearly exponential increase in PBDE concentration in human milk was observed for the period 1972 to 1997, with a doubling time of 5 years.
Lucross et al. (2000) reported PBDEs in marine mammals from the Canadian Arctic for the first time, suggesting that PBDEs are ubiquitous pollutants in the Arctic environment (Aspelund et al. 1999, Lucross et al. 2000). The samples presented in this section were from the projects: “Effects of Contaminants in the Greenland Sea Polar Bear”, “Contaminants in Greenland human diet” and “Non-halogenated Organic Substances in the Greenland Environment”, representing the first investigations of PBDEs in Greenland. Chemical analyses were performed by The National Water Research Institute, Canada and by NERI, Denmark.
Results and discussion
Table 5.8.1 summarises PBDE concentrations measured in various sample types and areas of Greenland.
The spatial trend for PBDEs has earlier been reported to be much like that for PCBs and DDT with a decreasing trend in concentration levels going from south to north (Selström et al. 1993). As different sample types were collected at different latitudes of Greenland this trend could not be investigated through these data. Spatial differences were seen in PBDE levels in black guillemot. Levels are highest on the east coast compared to the west coast, the same pattern earlier seen for a range of chlorinated compounds. This difference between east and west was also seen for ringed seals. The relatively low level of PBDEs discovered in blubber of ringed seal from Qeqertarsuaq (4 ng/g ww) agrees well with earlier findings of much lower levels of PBDEs in Arctic ringed seal (58 ng/g ww) compared to e.g. the Baltic Sea (344 ng/g ww, Hagelund et al. 1997).
In the study from southern Greenland samples were collected in areas with different degree of urbanisation, Qaqortoq a town of 3200 inhabitants, Igaliko a settlement with 40 inhabitants and a background area with no human population. Total PBDE levels were positively correlated with degree of urbanisation in the sampling area indicating the importance of local sources of PBDEs (Christensen et al. 2002).
BDE-47 was the dominating congener in all marine mammals studied (de Wit, 2002). This was also consistent with the data for all samples of mussels, fish and mammals presented in Table 5.8.1.
Higher concentrations were observed in males compared to females of black guillemot. For polar bears no difference in overall PBDE level in the two sexes were seen. This is in contrast to the general trend for many other halogenated substances. The level of PBDEs in polar bears from Ittoqqortoormiit was in the same range as seen for ringed seals from the same area, in other words there were no signs of biomagnification at this trophic level. However, the proportion of BDE-47 was higher in polar bear than the other organisms studied, which agrees well with earlier findings that this congeneer shows the highest tendency of biomagnification (Jansson et al. 1993). The concentrations of PBDEs at lower trophic levels, in fish and mussels from south Greenland revealed signs of biomagnification for total PBDEs and the congener BDE-47 in particular.
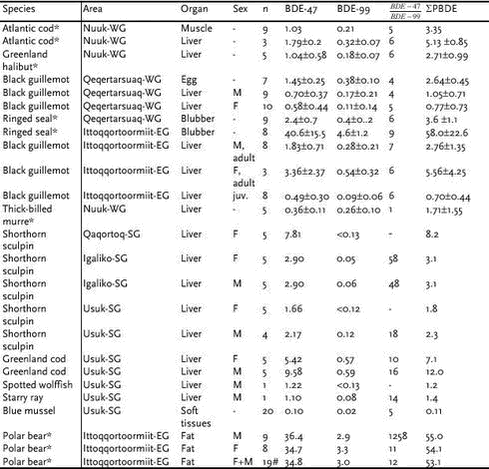 Table 5.8.1 PBDE concentrations (ng/g ww) in various samples from Greenland. * denotes samples analysed by NWOC, all other samples were analysed by NERI. F: female, M: male and juv.: juvenile. # sex was not determined for two samples.WG=west Greenland, SG=south Greenland, EG=east Greenland
Conclusions and recommendations
PBDEs are found in all matrices analysed from Greenland, showing a tendency of bioaccumulation in the same manner as seen for the OCs traditionally analysed in the Arctic. This is in accordance with results from other parts of the Arctic. PBDEs are transported to Greenland by long-range transport, but local sources of contamination are also of importance.
In the Arctic PBDE levels are low and it seems unlikely that they will affect any species here. However, the levels of PBDEs have elsewhere been seen to increase at a nearly exponential rate. Continued monitoring of PBDEs in the Greenland environment can therefore be recommended.
5.9 Polycyclic Aromatic Hydrocarbons (PAH)
In the present study, the term PAHs is used for the compounds consisting of two or more fused aromatic rings. These are planar structures, consisting of carbon and hydrogen atoms. Additionally the heterocyclic (sulfur containing) PAH analogue dibenzothiophene is included. The general physical characteristics common to the class are high melting- and boiling points, low vapour pressure and low water solubility (Sims & Overcash, 1983, Kjeldsen & Larsen, 1988), which tend to decrease with increasing molecular mass.
PAHs are emitted to the environment primarily from pyrogenic and petrogenic sources. PAH molecules with several rings are cancerogenic. The compounds also have endocrine, mutagenic, terratogenic and immunosupressive effects (e.g. Neff, 1979). PAH have proven toxicity to aquatic organisms at very low concentrations. Aqueous PAH concentrations of 0.7 µg/L can cause malformations and genetic damage in fish larvae, while sublethal effects, such as premature hatching, were observed at concentrations of 0.4 µg/L (Carls et al., 1999). Early development of flatfish was also afflicted at benzo(a)pyrene concentrations of 0.1 µg/L in the water phase (Hose et al., 1982).
For PAHs, there is little knowledge of the degree of long-range transportation into the Arctic. PAHs are more likely to have an impact in regions with direct inputs like power plants, waste incinerator, heavy traffic, oil wells and refineries. Petroleum production however now takes place in many parts of the Arctic and exploration has also taken place in Greenland. Furthermore natural oil seeps can lead to substantial elevations of PAH levels in the environment.
The survey described in this section was part of the project “Non-halogenated organic substances in the Greenland Environment” (NOSGE) carried out in Southern Greenland in 1999-2000. As little information was available on PAH levels in Greenland, the main objective of this project was a screening for PAH in the Greenland environment, including biotic and abiotic matrices. Three main sampling sites were chosen, representing different degrees of human population: Usuk (no population), Igaliko (population 40) and Qaqortoq (population 3200) (Figure 5.9.1). Thus, samples available from the three sites might also give information on the effect of human population on the PAH levels in the environment. All analyses were performed at the NERI laboratory, Denmark. The list of PAHs analysed is shown in Table 5.9.1.
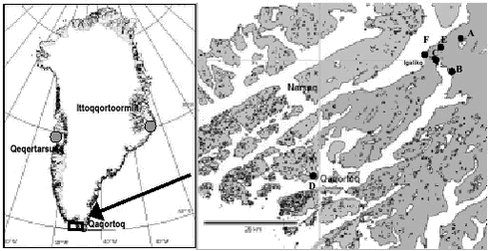 Figure 5.9.1 Sampling locations. Marine samples were collected at Usuk (B), a reference area with low degree of human activity, Igaliko (C), a settlement with approximately 40 inhabitants, and Qaqortoq (D), a village with approximately 3200 inhabitants. Freshwater samples were taken in the Lake (A) close to Igaliko. Pricipitation was collected at the locations A, B, and D.
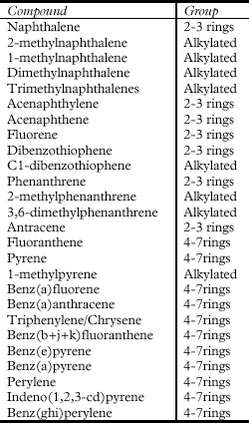 Table 5.9.1 PAHs monitored in the present study.
In the calculation of sum concentrations, the parameter S higher PAH was introduced, summing all compounds from Fluorene to Benz(ghi)perylene from Table 5.9.1. This parameter was chosen to account for incomplete data sets, which sometimes lacked the smaller PAH molecules due to analytical errors. Thus, sum concentrations based on the same number of compounds could be used for comparisons between matrices.
5.9.1 Precipitation
Precipitation samples of 2.5-10 L were collected in the background area (Usuk), near Igaliko (a settlement with 40 people) and at the town Qaqortoq with about 3200 inhabitants. The data set consists of single samples collected over a period of approximately 5 months. Therefore, variation between samples is expected to be large.
During summer 2001 the PAH concentration in precipitation at Igaliko was at the same level as at Usuk (the background site). The concentration at Qaqortoq was almost nine times higher than the background concentrations, thus indicating the influence of local activities.
During winter 2001, PAH concentrations were similar to summer 2001 at Usuk, while the concentration in precipitation collected at Igaliko was 2.5 times higher than summer 2001. The somewhat higher concentrations at Igaliko could be caused by local emissions from heating during winter. Interestingly, the sum PAH concentration at Qaqortoq was somewhat lower during winter than during summer. This could indicate that the activities giving rise to the elevated PAH levels at Qaqortoq are somewhat reduced in winter. The sum of PAH in precipitation at Qaqortoq in winter is still 5.5 times higher than at Usuk. The difference in PAH levels at Qaqortoq is mainly caused by much lower concentrations of acenaphtylene (8 times lower) and fluorene (10 times lower) in winter compared to summer 2001. Interestingly, benzo(ghi)perylene is higher in winter compared to summer (25 times), while other PAHs are at the same levels in both samples from Qaqortoq. Automobile exhaust has previously been identified as a source to benzo(ghi)perylene (Masclet et al., 1986).
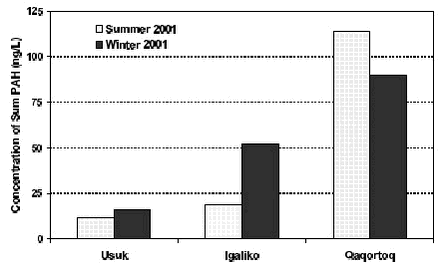 Figure 5.9.2 Concentrations of sum of PAH (ng/L) in precipitation samples from Southern Greenland.
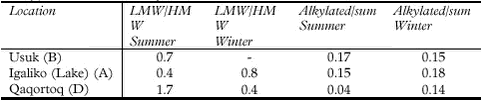 Table 5.9.2 Relative concentrations of PAHs in precipitation from southern Greenland summer and winter 2001. LMW/HMW: Ratio of sum 2-3 ringed compounds to 4-7 ringed. Alkylated/Sum: Ratio of alkylated compounds to total sum PAHs.
The PAH concentrations of 14 compounds in precipitation at Igaliko are comparable to PAH concentrations in rainwater observed during summer at background sites in France (Garban et al., 2002). Surprisingly, the PAH concentration in both summer and winter precipitation from Qaqortoq was about 2-3 times higher than average PAH concentrations at background sites in France during summer. This indicates that local pollution sources are present at Qaqortoq.
5.9.2 Terrestrial Environment
PAH concentrations found in soil and lichens (Cetraria nivalis) are presented in Table 5.9.4 and Figure 5.9.3. The suitability of the lichen species C. nivalis for biomonitoring of atmospheric deposition of elements in Greenland has previously been investigated (Riget et al., 2000). The results indicate that the lichen was well suited for this purpose.
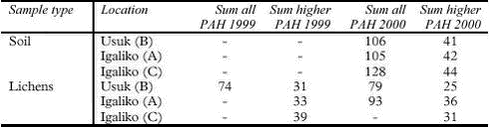 Table 5.9.3 Sum PAH in soil (µg/kg dw) and lichens (µg/kg ww) from southern Greenland. Sampling locations are shown in Figure 5.9.1.
Generally the PAH levels in both soil and lichens are within the same range.
Concentrations of PAH in soil vary only slightly among the sites, the only noteworthy difference being the somewhat higher concentration of sum of all PAH at Igaliko – farm (site C). This slightly higher concentration is mainly due to higher levels of trimetylnaphtalene, acenaphtylene and fluorene in this sample. The PAH levels in lichen samples from two consecutive years indicate that the interannual variability in PAH concentrations is low and that C. nivalis is suited for biomonitoring of atmospheric deposition of PAHs.
Figure 5.9.3 Concentrations of sum of higher PAHs in lichens (µg/kg ww) and soil (µg/kg dw) sampled in 2000.
5.9.3 Freshwater environment
The results are summarised in Table 5.9.4. Arctic chars from the two lakes have similar PAH levels. Male samples show similar ratios of alkylated to sum PAHs. PAH concentrations in Arctic char are significantly lower than levels in shorthorn sculpin.
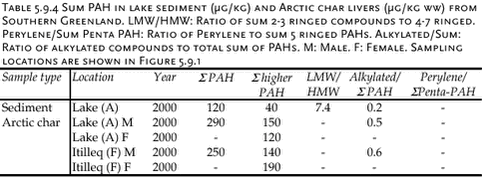 Click on the picture to see the html-version of: ‘‘Table 5.9.4‘‘
5.9.4 Marine environment
PAH concentrations have been analysed in bladder wrack (Fucus vesiculosus), blue mussels (Mytilus edilus), shorthorn sculpin (Myoxocephalus scorpius) livers and sediments. All samples originate from the three sampling locations with different numbers of population in Southern Greenland and were collected in 1999 and 2000. Usuk is regarded as a background station without any potential influence from human activity. The matrices analysed are not always consistent: Sediment samples are available from Igaliko only. Bladder wrack was only sampled in 2000, as well as shorthorn sculpins and blue mussels from Qaqortoq. Besides, data are available for several other invertebrates and macroalgae collected at either Usuk or Igaliko (Table 5.9.5).
The macroalgea summarised in Table 5.9.5 can accumulate organic pollutants from seawater. The PAH levels in bladder wrack, Arctic wrack, sea lettuce and sweet tangle are similar to the levels in blue mussels from 1999, while the sum PAH concentration in Enteromorpha are about two times lower. It has to be noted, however, that all results except for periwinkle are based on single samples. The concentrations are higher in sand hopper and particularly in lugworm, indicating either an accumulation of the compounds or a stronger exposure. Lugworms are likely to take PAH up from the sediment. Thus, the exposure is higher, in particular to higher molecular weight PAH associated with the organic matter of the sediment. This might contribute to the lower LMW/HMW ratio than observed for sand hopper and algae.
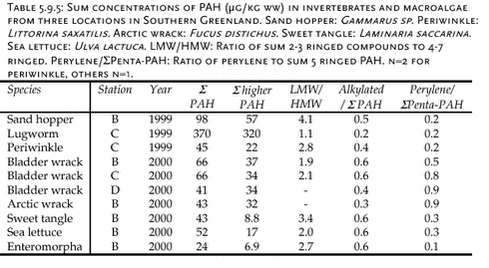 Click on the picture to see the html-version of: ‘‘Table 5.9.5‘‘
Differences between the sampling sites, which might be related to the different degrees of human activity, can be observed for blue mussels. Furthermore, an increase in sum PAH can be noticed for the samples taken at Usuk and Igaliko in 1999 and 2000 (Table 5.9.6), but not for shorthorn sculpins or sediments. Apparently, the year-to-year variation is more pronounced for blue mussels, which primarily accumulate organic compounds from seawater.
Blue mussels from 1999 include the background station Usuk and two sampling sites at Igaliko inside and outside the harbour (Table 5.9.6). The low molecular weight PAHs are omitted from the data set for most samples due to low recovery rates for these compounds in the chemical analysis of the blue mussels. The samples are divided into three groups of different sizes of mussels, which do not correlate with the sum PAH concentration. The concentrations of the samples from the inner harbour tend to be slightly higher than those from outside the harbour. The levels from Igaliko exceed the concentrations at Usuk, but due to the large variation within the Igaliko samples, this difference cannot be quantified.
Blue mussels collected in 2000 are divided into two groups, ranging from 30-50 mm and from 50-70 mm. No obvious difference in the sum PAH concentrations can be seen between the two size groups. The sum PAH concentrations in blue mussels from Igaliko exceed the concentrations from Usuk. Results from Qaqortoq, the site with the largest number of inhabitants, are about 50 % higher than concentrations at Igaliko. The LMW/HMW ratio as well as the perylene/ΣPenta-PAH ratio also reflect different PAH patterns, possible caused by different local impacts. However, the LMW/HMW ratio is not consistent: Samples from Qaqortoq, the location with the highest number of human activity had the highest percentage of low molecular weight compounds, while Igaliko has the lowest LMW/HMW ratio. This inconsistency might reflect different influences from pyrogenic and petrogenic sources. The concentrations in the mussels from 2000 are similar to levels found for blue mussels from the Baltic Sea (Baumard et al., 1999).
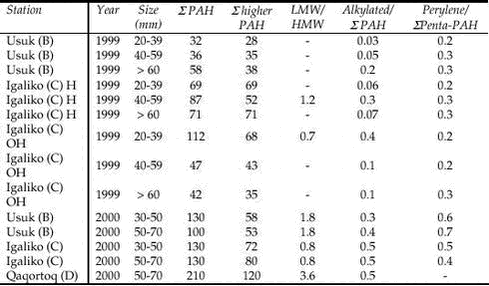 Table 5.9.6: Sum concentrations of PAH (µg/kg ww) in blue mussels from three locations in Southern Greenland. LMW/HMW: Ratio of sum 2-3 ringed compounds to 4-7 ringed. Perylene/∑Penta-PAH: Ratio of perylene to sum 5 ringed PAH. H: Harbour. OH: Outside the harbour. n=2 for Usuk (1999), others n=1.
The degree of urbanisation was found to correlate with the concentrations of brominated flame retardants in shorthorn sculpins from the three sampling sites (Christensen et al., 2002), indicating local sources of these contaminants in Greenland. Shorthorn sculpins are stationary fish and can therefore be assumed to reflect the actual concentrations in the sampling area. The increasing sum PAH concentrations in blue mussels from stations with increasing numbers of population might indicate the same phenomenon. Local sources of PAH might include combustion processes such as waste incineration and boat or vehicle engines as well as direct losses of petrol and oil to the environment.
However, the trend found in blue mussels was not confirmed for bladder wrack or shorthorn sculpins (Table 5.9.7). Being a stationary fish species, shorthorn sculpin was found to reflect local sources of brominated flame retardants from the three sampling locations. Different metabolisation processes might occur for PAH in shorthorn sculpins, but they are unlikely to even out potential differences caused by local sources. However, even though the absolute concentrations do not differ between the three sampling sites, the LMW/HMW ratio for samples from Qaqortoq tends to be higher than for the samples from the less populated stations. No difference is observed for Igaliko and Usuk. The higher percentage of low molecular weight PAH might indicate local impacts, which are different from the exposure at Igaliko and Usuk.
As other factors such as age and nutritional status might influence the concentrations in shorthorn sculpins, samples were analysed separately for small (young) and large (old) as well as for female and male fish (Table 5.9.7). No trend can be established for this differentiation. While female, large fish from Usuk have higher concentrations than the small fish, the opposite can be seen for the female fish from Igaliko. For male fish, the concentrations are highest in the small fish in three out of four cases. For persistent organic pollutants, higher concentrations in male marine animals than in female have been described in the literature (AMAP, 1998), which are explained by an excretion of the pollutants to the offspring. This observation cannot be confirmed for the PAH data collected on shorthorn sculpins from Southern Greenland, possibly due to more complex metabolisation processes.
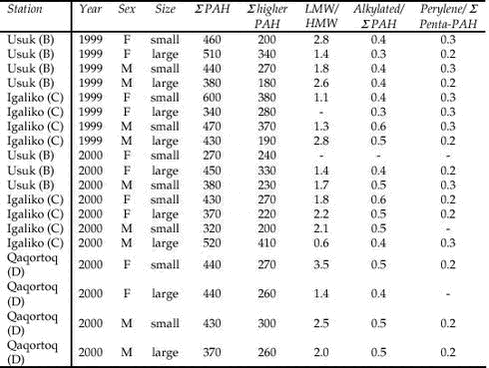 Table 5.9.7: Sum concentrations of PAH (μg/g ww) in shorthorn sculpin livers from three locations in Southern Greenland. ∑ higher PAH excludes naphthalene, methyl naphthalenes, acenaphthylene and acenaphthene. LMW/HMW: Ratio of sum 2-3 ringed compounds to 4-7 ringed. Perylene/∑Penta-PAH: Ratio of perylene to sum 5 ringed PAH. n=1, each sample is pooled of 4-5 individuals.
The PAH concentrations in the matrices analysed from Usuk, Igaliko and Qaqortoq are summarised in Figure 5.9.4, which illustrates the increasing concentrations in blue mussels with increasing number of population in the sampling area. The concentrations in biota are exceeded by the levels in sediment, which vary considerably between 1999 and 2000. However, the sediment concentrations are related to dry weight, while the biota concentrations are based on wet weight. These levels indicate the occurrence of PAH in Arctic sediments, which is likely to be a source of contaminant uptake by aquatic organisms.
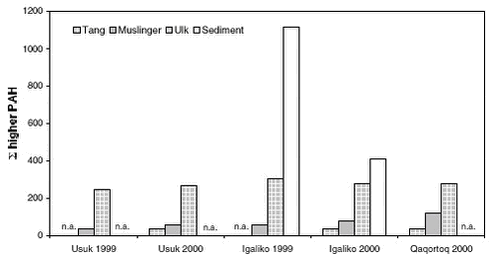 Figure 5.9.4: Sum concentrations of higher PAH in biota (ng/g ww) and sediment (ng/g dw) from three locations in southern Greenland and two sampling periods. higher PAH excludes naphthalene, methyl naphthalenes, acenaphthylene and acenaphthene. n.a.: not analysed.
For all sampling locations and both years studied, the concentrations increase from bladder wrack to blue mussels and shorthorn sculpins, which indicates bioaccumulation of PAH at low trophic levels in the marine food chain. However, it has to be noted that the concentrations are not normalised to dry weight. PAH have the potential to bioaccumulate in aquatic organisms, which lack efficient mixed-function oxidase detoxification mechanism (Law et al., 1997). Unlike the biomagnification of halogenated compounds in the Arctic marine food chain (e.g. Cleemann et al., 2000a), the main route of exposure for sediment dwelling fish is uptake from the water which includes dissolved and particular bound PAH (Law et al., 1997).
Conclusions and recommendations
This study shows the occurrence of PAH in various compartments of the Greenland environment. The precipitation and blue mussel data indicate an existence of local antropogenic sources in Greenland as the sum PAH concentration correlates with the number of population at the sampling location. More research is needed to study the extent of this phenomenon. Data available on seawater, blue mussels and shorthorn sculpins show increasing PAH concentrations with higher trophic levels, possibly reflecting bioaccumulation of PAH. However, the complex pattern of exposure, accumulation and metabolisation was not subject of this study. Hence, conclusions on the fate of PAH in the Greenland environment will require further research.
5.10 Contaminants of future concern
The contaminants described in the preceeding sections are all contaminants that have just recently been discovered in the Arctic environment or compounds that are not included in the traditional monitoring programs in the Arctic. The data presented here illustrates the fact that previously unknown or disregarded organic pollutants are likely to be emitted to the environment and transported to remote regions in a similar way as shown for PCBs and other organohalogens, or emitted from local sources in the Arctic. Recognizing this, and recognizing the vast amount of new chemicals taken into use each year, it seems obvious that some of these could be expected to become future contaminants in the Arctic. Examples of such chemicals are briefly described in this section.
5.10.1 Perfluorooctane sulfonate (PFOS)
Fluorinated organic compounds (FOCs) have been manufactured for more than 50 years, but have except for the CFC-gasses received less attention than brominated and chlorinated compounds, primarily perhaps because their measurement is more challenging. Some perfluorinated compounds are very stable in the environment. Among these fluorinated organic compounds, sulfonyl-based fluorochemicals have been produced and used for over 40 years as surface protectors in carpets, leather, paper and packaging, fabric and upholstery. It has been hypothesised that the fluorine-containing products could degrade to other fluorochemicals including perfluorooctane sulfonate (PFOS). According to UNEP the total production of PFOS-compounds is about 5000 tons a year worldwide (cited in Havelund, 2002).
Surface-water concentrations of 25-144 ng PFOS/L have been detected near to perfluorinated surfactant related manufacturing facilities (Hansen et al. 2002). PFOS is relatively soluble in water with a max. solubility of 460 mg/L (estimated on the basis of critical micelle concentration).The compound is very persistent in the aquatic environment (Sanderson et al. 2002). Giesy and Kannan (2001) recently reported PFOS levels in a number of wildlife species from all over the world. PFOS were present in most samples also from remote marine areas. However, concentrations of PFOS in these regions were several times lower than those from more industrialized areas. In the Baltic Sea levels of PFOS in the range 14-230 ng/mL were detected in blood from gray seals and ringed seals. In the same species from the Canadian and Norwegian Arctic the levels were 3-50 ng/mL. Livers of polar bears from Alaska contained 180-680 µg/kg ww. Fish-eating, predatory animals contained PFOS in concentrations higher than their diet, indicating bioaccumulation of PFOS (Giesy & Kannan, 2001). Under laboratory conditions the biomagnification factor based on the concentrations of PFOS in liver of mink was approximately 22. The level of PFOS in the general human population of the U.S. is 30-44 ng/m serum. The mean elimination half li fe in humans is about 4 years (U.S. EPA 2000 cited in Sanderson et al. 2002)
Sanderson et al. (2002) reported significant influence on zooplankton communities at a concentration of 10 mg PFOS/L and 96-hour acute toxicity tests with rainbow trout determined LC50 values of 1250-2500 mg/L (Conducted by the 3M Company, hhtp://www.chemicalindustryarchives.org/dirtysecrets/scotchgard/1.asp). Little information is available on possible long-term toxic effects of PFOS to wildlife. PFOS and related perfluorinated compounds have been shown to affect e.g. cell-cell communication and membrane transport processes. In a recent study by Seacat et al. (2002) the effect of PFOS was measured in cynomolgus monkeys. Significant adverse affects measured one year after ended treatment were observed in animals receiving 0.75 mg/kg/day, these animals showing decreased bodyweight, increased liverweights, lowered serum cholesterol, lowered triiodothyronine and lowered estradiol levels.
5.10.2 Synthetic musks
Synthetic musks are of great industrial importance. They impart desirable musk-like odours and are used on a grand scale as fragrance fixatives and materials in personal care and household products, such as cosmetics, soaps, laundry detergents, fabric softeners, household cleaning products, air fresheners etc. In 1996 the worldwide production of synthetic musk compounds amounted 8000 tons, of this production of nitro musks accounted for 25 % (mainly musk ketone and musk xylene), polycyclic musks made up 70 % (mainly HHCB and AHTN) and macrocyclic musks accounted for 5 % (Rimkus, 1999).
The synthetic musks enter the environment mainly via wastewater or household waste when dumped directly in the environment. Nitro musks have been detected in a broad range of environmental compartments: marine and fresh water as well as fish, mussels and shrimps (Rimkus & Wolf 1995, Gatermann et al. 1995) and in human addipose tissue and breast milk (Liebl & Ehrenstorfer 1993, Müller et al. 1996) Due to the high potential for bioaccumulation (log Kow = 4.31 and 4.45 for musk ketone and musk xylene respectively) musk xylene was banned in Japan in the 1980ies and was in 1998 included in the third list of chemicals for priority action of the EU and OSPAR commision. Nitro musks are highly persistent in the environment and accumulate in aquatic biota. High acute toxicity of nitro musks has been reported for several aquatic species and the compounds have been related to reproductive toxicity and endocrine effects in animals and humans (e.g. Eisenhart et al. 2001).
Polycyclic musks are now increasingly substituting the nitro musk compounds. In Europe HHCB and AHTN are being classified as high volume chemicals with an average use per capita in 1995 of about 15.5 mg/day (in Gatermann et al. 2002). In various environmental compartments the polycyclic musks exceed the concentration of the nitromusks. Biodegradation of polycyclic musks is relatively slow (half-life in soils of aprox. 180 days), and due to their lipophilic properties (log Kow = 5.7 and 5.9 for AHTN and HHCB) as conciderable bioconcentration is recorded (Balk and Ford, 1999). Polycyclic musks have been found in most environmental compartments and have also been detected in human addipose tissue and human milk (Müller et al. 1996, Rimkus & Wolf 1996). High acute toxicity have been reported for polycyclic musks (LC50 <1 mg/L) for several aquatic species (Balk & Ford, 1999).
5.10.3 Polychlorinated naphtalenes
Polychlorinated naphthalenes (PCNs) have mainly been used as dielectric fluids, flame retardants and fungicides and occur as impurities in technical PCB formulations. They are also by-products of human activities such as copper-roasting, degradation of polychlorinated alkenes and combustion processes. Total global production has been estimated to 150 000 tons (in Falandysz, 1998). PCNs have never been banned but have to a large extent been replaced by less toxic compounds.
PCNs are a mixture of 75 congeners – all planar compounds that can affect the aryl hydrocarbon (Ah) receptor and thereby elict dioxin like responses – including immunotoxicity, embryotoxicity, terratogenicity and carcinogenicity (reviewed in Hayward, 1998). TCDD equivalency factors (TEFs) for PCN congeners are reported to range from 7x10-6 to 2x10-3 (Hanberg et al., 1991). Evidence exists that these substances are widespread pollutants in the environment that co-occur with PCBs and other organohalogens (e.g. Giesy et al. 1997, Kanan et al. 2000). Results from plankton analysis in the Baltic Sea and sediment and biota in Sweden indicate long-range transportation of these pollutants (Falandysz & Rappe, 1996). PCNs are very lipophilic with log Kow from 3.9 to 8.3 (http://www.es.lancs.ac.uk/kcjgroup/6.html) and therefore tend to accumulate in sediments and biota. Signs of biomagnification have also been documented (Falandysz & Rappe, 1996, Falandysz et al. 1997) PCNs have also been measured in low concentrations in the Arctic. Levels of ΣPCN (3-7 Cl) in blubber ranged from 35.9 to 383 ng/kg lw in beluga whales and from 35.4 to 71.3 ng/kg lw in ringed seals from the Canadian Arctic. The PCN concentrations were less than 1 % of the coplanar PCBs – but as their toxic potential measured as TCDD-TEQ is 11 times higher they contributed significantly to the total toxicity (Helm et al. 1998).
5.10.4 Brominated flame retardants
Beside the polybrominated diphenylethers other compound groups of brominated flame retardants can possibly present an increasing problem in the Arctic. Little knowledge regarding concentrations and fate in the environment is available of hexabromocyclododecane (HBCD), tetrabromobisphenol A (TBBPA) and polybrominated biphenyls (PBBs). The estimated world market demand for HBCD is 15 900 t annually in 1999 (de Wit, 2002). HBCD is used as an additive in foams and expanded polystyrene. Sellström et al. (1998) detected levels of 7600 ng/g carbon in sediments and up to abot 8000 ng/g lw in pike in a river receiving wastewater from several industries. Ratios of HBCD in fish and sediments from 0.6 to 15 show that the compound is bioavailable and can potentially bioaccumulate.
TBBPA is the flame retardant produced in the highest amount globally: 60 000 t pr. year. TBBPA is incorporated in polymers as a reactive flame retardant covalently bound to the polymer. Where detected in the environment, TBBPA is mainly found in soil and sediment samples. A relatively high bioconcentration factor seems to be balanced by rapid excretion and the compound has not normally been found in environmental biological samples. The phenolic groups of TBBPA may however be methylated in the environment and the resulting Me2-TBBPA is more lipophilic. This compound has also been found in sediment, fish and shellfish (reviewed in WHO, 1995).
PBBs are a group of manufactured chemicals that may contain up to 209 individual compounds. In the United States, the production of PBB products was banned in 1977. PBBs were used mainly to prevent fires in plastic parts used in electronic products, such as radios and televisions. PBBs were also used in plastics for business machine housings and other industrial products, such as motor housings. In many ways, PBBs are similar to polychlorinated biphenyls (PCBs), they are highly persistent in the environment and are shown to bioaccumulate (reviewed in WHO, 1994). The detection of PBBs and PBDEs in sperm whales, wich feed in deep ocean waters, shows that these compounds can be considered as global pollutants (Soni et al. 1998).
5.10.5 PBDD/F
Polybrominated dibenzodioxins and dibenzofurans (PBDD/F) are formed as byproducts in chemical processes. They have been found in traces in organic chemicals such as tribromophenol and brominated flame retardants. The main source of formation is the thermal decomposition of brominated precursor compounds, for instance polybrominated diphenylethers. Thus, PBDD/F have been detected in emissions from municipal waste incineration, but to a clearly less extent than the chlorinated dioxins and furans (PCDD/F). The detection of chloro-bromo-containing dioxins or furans has been reported more frequently. These mixed halogenated species are primarily in the form of monobromo-PCDD/PCDF (Hardy, 1997). However, corresponding to the formation of PCDD/F, highest concentrations of PBDD/F and dibromoPCDD/F were found in fly ashes, which might increase with larger amounts of bromine-containing material being incinerated (Huang et al. 1992; Weber et al. 2002).
Theoretically, 75 PBDD and 135 PBDF congeners can exist. Like the chlorinated compounds, the 2,3,7,8 substituted congeners have the highest toxicity. The effects are assumed to be similar to the chlorinated compounds, but the acute toxicity has been found to be 5-10 times weaker compared to the PCDD/F (Mennear & Lee, 1994). Occupational exposure is possible in industrial branches producing and processing brominated flame retardants. Apart from studies connected to municipal waste incineration, information on environmental levels of PBDD/F is sparse, possibly due to the very sensitive analytical methods required.
5.10.6 Triclosan
Triclosan is a diphenyl ether (bis-phenyl) derivative, known as either 2,4,4’-trichloro-2’-hydroxydiphenyl ether or 5-chloro-2-(2,4-dichlorophenoxy) phenol. Triclosan is manufactured in the U.S. under the trade name Irgasan DP-300, and by several other manufacturers outside of the U.S. Triclosan inhibits the enzyme ENR involved in the bacterial lipid biosynthesis and is therefore inhibitory against both gram-negative and gram-positive bacteria (Levy et al. 1999, McMurry et al. 1998). It has found increasing use in personal care products, i.e. toothpaste, deodorant soaps, deodorants, antiperspirants and body washes, detergents, dish washing liquids, cosmetics and anti-microbial creams, lotions and hand soaps. It is also used as an additive in plastics, polymers and textiles to give these materials antibacterial properties. In Sweden for example 25 % of the total amount of toothpaste sold in 1998 contained triclosan, corresponding to about 2 tons of active ingredient (from Lindström et al. 2002)
Triclosan is moderately watersolube (10 mg/L at 20 oC) and has a vapour pressure of 6,45x10 –7 mm Hg. It is relatively persistent in the sediment with half-lives of about 3 4.76. Methylated weeks (Vershueren, 1996). Triclosan is lipophilic with a log Kow of derivatives can furthermore be formed in the environment, possibly by biological methylation of triclosan (Lindström et al. 2002). Laboratory experiments showed that triclosan in the dissociated form was rapidly decomposed in lake water when exposed to sunlight (half-life less than 1 h in August at 47degrees latitude). Methyl triclosan and nondissociated triclosan, however, were relatively stable to photodegradation (Lindström et al. 2002).
Triclosan has not been shown toxic to mammals but it is toxic to water-living organisms such as fish (LOEC, rainbow trout early lifestages = 71.3 µg/L) and Daphnia magna (EC50 = 390 µg/L) and especially to algae (Scenedesmus sp. EC50 = 1.4µg/L) (Orvos et al. 2002) and, of course, to bacteria. Bioacumulation factors are reported in the range 2500 to 4100 (Orvos et al. 2002). Of further concern is the transformation of triclosan into clorinated dioxins upon incineration and influence of sunlight (Kanetoshi et al. 1988a, b).
The environmental occurrence of triclosan has been reported in wastewater and surface waters from Europe and the US in a range of 2-2300 ng/L (Paxéus 1996, Lindström et al. 2002, Kolpin et al. 2002) Triclosan and its methylated derivative has also been detected in wildlife fish (Miyazaki et al. 1984, Adolfsson-Erici et al. 2002). In the survey by Adolfsson-Erici et al. 2002 triclosan was furthermore found in samples of human milk. Due to its widespread use local sources of triclosan pollution will most likely occur in the Arctic, and due to the lipophilic properties of the compound bioaccumulation is likely to occur.
5.10.7 Aromatic amines
Monocyclic aromatic amines (MAA) are potential environmental pollutants. They contain a single aromatic ring and at least one amine function to the ring. Many of these compounds originate from azo dyes which are widely used in textiles, food and drink, pharmaceuticals, cosmetics, plastics, paper, leather, printing ink, paints, varnishes, lacquer and wood staining (Øllgaard et al. 1998). The total input of azo dyes in Denmark is 2400 t/year (Øllgaard et al. 1998). When azo dyes enter the human gastrointestinal tract through ingestion of food or drink, aromatic amines are produced by azoreductases of intestinal microorganisms and, to a lesser extent by intestinal enzymes. MAAs are also released into the environment when azo dyes are discharged into the rivers from the textile industry, the dyestuff manufacturing industry and other industries or by municipal waste. MAAs are potentially toxic, mutagenic and/or carcinogenic to humans and animals (in Chung et al. 1997).
5.10.8 Conclusions and recommendations
The compounds PFOS, synthetic musks, polychlorinated naphthalenes, brominated flame retardants (HBCD, TBBPA and PBB) other than PBDE, polybrominated dibenzodioxins and dibenzofurans, aromatic amines and the biocide triclosan are examples of high volume chemicals of high international concern found in the environment in lower latitudes. Some of these compounds have already been detected in Arctic samples. A screening of the Greenland environment for these compounds is therefore recommended. If the screening reveals abundance of the chemicals mentioned, further studies regarding baseline levels and bioaccumulation are recommended.
5.11 References
Addison, R.F. & T.G. Smith, 1974.Organochlorines residue levels in Arctic ringed seals: variation with age and sex. Oikos 25: 335-337.
Adolfsson-Erici, M., M. Pettersson, J. Parkkonen & J. Sturve, 2002. Triclosan, a commonly used bactericide found in human milk and in the aquatic environment in Sweden. Chemosphere 46: 1485-1489
Aguilera-del Real, A., A. Valverde-García, A.R. Fernandez-Alba & F. Camacho-Ferre, 1997. Behaviour of endosulfan residues in peppers, cucumbers and cherry tomatoes grown in greenhouses: Evaluation by decline curves. Pest. Sci. 51 (2): 194-200.
AMAP, 1998. AMAP Assessment Report: Arctic Pollution Issues. Arctic Monitoring and Assessment Programme (AMAP), Oslo, Norway. xii+859 pp.
Antonius, G.F. & M.E. Byers, 1997. Fate and movement of endosulfan under field conditions. Environ. Toxicol. Chem. 16 (4): 644-649.
Arnold, A., M.M. Weidenhaupt, S.R. David, S.B. Müller, S. Haderlein & R.P. Schwarzenbach, 1997. Aqueous speciation and 1-octanol-water partitioning of tributyl and triphenyltin: Effect of pH and Ion Composition, Environ. Sci. Technol. 31: 2596-2602.
Asplund, L., M. Hornung, R.E. Peterson, K. Turesson, A. Bergman, 1999. Levels of polybrominated diphenyl ethers (PBDEs) in fish from the Great Lakes and Baltic Sea. Organohalogen Compounds. 40. 351-354
ATSDR, 1997. Endrin. Agency for Toxic Substances and Disease Registry. U.S. Department of Helath and Human Services, Public Health Service.
Baird, C., 1998. Environmental Chemistry, 2nd ed. W.H. Freemann and Co.
Balk, F. & R.A. Ford, 1999. Environmental risk assessment for the polycyclic musks, AHTN and HHCB in the EU I. Fate and exposure assessment, Toxicology Letters, 111: 57-79
Balk, F. & R.A. Ford, 1999. Environmental risk assessment for the polycyclic musks, AHTN and HHCB II. Effect assessment and risk characterisation, Toxicology Letters, 111: 81-94
Baumard, P., H. Budzinski, P. Garrigues, T. Burgeot, X. Michel & J. Bellocq, 1999. Polycyclic aromatic hydrocarbon (PAH) burden of mussels (Mytilus sp.) in different marine environments in relation with sediment PAH contamination, and bioavailability. Marine Environmental Research. 47(5): 415-439
Christensen, J.H., M. Glasius, M. Pécseli, J. Platz, G. Pritzl, 2002. Polybrominated diphenyl ethers (PBDEs) in marine fish and blue mussels from southern greenland. Chemosphere 47: 631-638.
Carls, M.G., S.D. Rice & J.E. Hose, 1999. Sensitivity of fish embryos to weathered crude oil: Part I.Low level exposure during incubaion causes malformations, genetic damage, and mortality in larval pacific herring (Clupea pallasi). Environmental Toxicology and Chemistry. 18(3): 481-493
Chung, K.T., L. Kirkovsky, A. Kirkovsky & W. P. Purcell, 1997. Review of mutagenecity of monocyclic aromatic amines: quantitative structure-activity relationships. Mutation Research. 387: 1-16
Cleemann, M., F. Riget, G.B. Paulsen, J.de Boer & R. Dietz, 2000a. Organochlorines in Greenland ringed seals (Phoca hispida). Sci. Total Environm. 245: 103-116.
Cleemann, M., F. Riget, G.B. Paulsen, J.de Boer, J. Klungsøyr & R. Dietz, 2000b. Organochlorines in Greenland lake sediments and landlocked Arctic char (Salvelinus alpinus). Sci. Total Environm. 245: 173-185.
Dubey, R.K., M.U. Beg & J. Singh, 1984. Effects of endosulfan and its metabolites on rat liver mitochondrial respiration and enzyme activities in vitro. Biochem. Pharmacol. 33 (21): 3405-3410.
Eisenhardt, S., B. Runnebaum, K. Bauer & I. Gerhard, 2001. Nitromusk Compounds in Women with Gynecological and Endocrine Dysfunction, Environmental Research Section A, 87: 123-130
Falandysz J. & C. Rappe, 1996. Spatial distribution in plankton and bioaccumulation features of polychlorinated naphthalenes in a pelagic food chain in southern part of the Baltic proper. Environmental Science and Technology, 30 (11): 3362-3370
Falandysz J., L. Strandberg, P.A. Bergqvist, B. Strandberg & C. Rappe, 1997. Spatial distribution and bioaccumulation of polychlorinated naphthalenes (PCNs) in mussel and fish from the Gulf of Gdansk, Baltic Sea. Science of the total environment. 203(2): 93-104
Falandysz, J. 1998. Polychlorinated naphthalenes – an environmental update, Environmental Pollution, 101: 77-90
Fent, K., 1996. Ecotoxicology of Organotin Compounds. Critical Reviews in Toxicology. 26(1) 1-117.
Følsvik, N., E.M. Brevik, J.A. Berge & M. Dam, 1998. Organotin and imposex in the Littoral Zone in the Faroe Isands, Fróðskaparrit. 46: 67-80.
Garban, B., H. Blanchoud, A. Moteley-Massei, M. Chevreuil & D. Ollivon, 2002. Atmospheric bulk deposition of PAHs onto france: Trends from Urban to remote sites. Atmospheric environments 36: 5395-5403.
Gatermann, R., S. Biselli, H. Hühnerfuss, G.G. Rimkus, M. Hecker & L. Karbe, 2002. Synthetic Musks in the Environment. Part 1: Species-Dependent Bioaccumulation of Polycyclic and Nitro Musk Fragrances in Freshwater fish and Mussels. Arch. Environ. Contam. Toxicol. 42: 437-446
Gatermann, R., H. Hühnerfuss, G. Rimkus, M. Wolf. & S. Franke, 1995. The distribution of nitrobenzene and other nitroaromatic compounds in the North Sea. Marine Pollution Bulletin. 30: 221-227
Giesy J.P & K. Kannan, 2001. Global Distribution of Perfluorooctane Sulfonate in Wildlife, Environmental Science and Technology, 35: 1339-1342
Giesy, J.P., D.J. Jude, D.E. Tillitt, R.W. Gale, J.C. Meadows, J.L. Zajieck, P.H. Peterman, D.A.Verbrugge, J.T. Sanderson, T.R. Schwartz & M.L. Tuchman, 1997. Polychlorinated dibenzo-p-dioxins, dibenzofurans, biphenyls and 2,3,7,8-tetrachlorodibenzo-p-dioxin equivalents in fishes from Saginaw Bay, Michigan. Environ Toxicol Chem, 16: 713-724
Gill, T.S., J. Pande & H. Tewari, 1991. Effects of endosulfan on the blood and organ chemistry of freshwater fish, Barbus conchonius Hamilton. Ecotox. Environ. Safety 21: 80-91.
Greve, P.A. & S.L. Wit, 1971. Endosulfan in the Rhine river. J. Wat. Poll. Control Fed. 43 (12): 2338-2348.
Gupta, P.D. & R.C. Gupta, 1979. Pharmocology, toxicology and degradation of endosulfan – a review. Toxicology 13: 115-130.
Haglund, P.S., D.R. Zook, H.R.Buser & J. Hu, 1997. Identification and Quantification of Polybrominated Diphenyl Ethers and Methoxy-Polybrominated Diphenyl Ethers in Baltic Biota. Environmental Science & Technology. 31. 3281-3287
Hallers-Tjabbes, C.C ten, J.F. Kemp & J.P. Boon, 1994. Imposex in Whelks (Buccinum undatum) from the Open North Sea: Relation to Shipping Traffic Intensities. Marine Pollution Bulletin. 28: 311-313.
Hanberg, A., M. Stahlberg, A. Georgellis, C. de Wit, & U.G. Ahlborg, 1991. Swedish dioxin survey: evaluation of the H-4-II E bioassay for screening environmental samples for dioxin-like enzyme induction. Pharmacol Toxicol. 69: 442-449
Hansen, K.J., H.O. Johnson, J.S. Eldridge, J.L. Butenhoff & L.A. Dich, 2002. Quantitative Characterization of Trace Levels of PFOS and PFOA in the Tennessee River, Environmental Science and Technology, 36: 1681-1685
Hardy, M.L., 1997. Disposal considerations. Brominated flame retardants and products containing brominated flame retardants. SMA BFRIP, Brominated Flame Retardants Workshop for EPA Regulatory Personnel, Washington D.C.
Havelund, S., 2002. Kortlægning af perfluoroktansulfonat og lignende stoffer i forbrugerprodukter – fase 2, Miljøprojekt nr. 691, 63 p (In Danish)
Hayward D., 1998. Identification of bioaccumulating polychlorinated naphthalenes and their toxicological significance, Environmental Research, 76(1): 1-18
Helm P.A., T. F. Bidleman, G.A. Stern & K. Koczanski, 2002. Polychlorinated naphthalenes and coplanar polychlorinated biphenyls in beluga whale (Delphinapterus leucas) and ringed seal (Phoca hispida) from the eastern Canadian Arctic. Environmental Pollution, 119 (1): 69-78
Hose, J. E., J.B.Hannah, D. DiJulio, M.L. Landolt, B.S. Miller, W. Iwaoka & S.P. Felton, 1982. Effects of Benzo(a)pyrene on Early Development of Flatfish. Archives of Environmental Contamination and Toxicology. 11: 167-171
Huang, L., H. Tong & J. Donnelly, 1992. Characterization of dibromopolychlorodibenzo-para-dioxins and dibromopolychlorodibenzofurans in municipal waste incinerator fly-ash using gas chromatography mass spectrometry. Analytical Chemistry 64 (9): 1034-1040.
Ikonomu, M.G., S. Rayne & R.F. Addison, 2002. Exponential Increases of the Brominated Diphenyl Ethers, in the Canadian Arctic from 1981 to 2000. Environ. Sci. Technol 36: 1886-1892
Jacobsen, J.A., F. Stuer-Lauridsen & B. Pedersen, 1998. Organotin in the aquatic environment, distribution, behaviour and processes. Report from National Environmental Research Institute (in prep)
Jacobsen, J.A., F. Stuer-Lauridsen & G. Pritzl, 1997. Organotin Speciation in Environmental Samples by Capillary Gas Chromatography and Pulsed Flame Photometric Detection (PFPD). Appl. Organometal. Chem. 11: 737-741
Janssom, B., R. Andersson, L. Asplund, K. Litzen, K. Nylund, U. Sellström, U:B: Uvemo, C. Wahlberg & U. Wideqvist, 1993. Chlorinated and brominated persistent organic compounds in biological samples from the environment. Environmental Toxicology and Chemistry. 12(7): 1163-1174
Kannan, K., K. Senthilkumar, B.G. Loganathan, S. Takahaso, D.K. Odell & S. Tanabe, 1997. Elevated accumulation of TBT and its breakdown products in bottlenose dolphins found stranded along the US Atlantic and Gulf coasts. Environmental Science and Technology. 31: 296-301
Kannan, K., N. Yamashita, T. Imagawa, W. Decoen, J.S. Khim, R.M. Day, C.L. Summer & J.P. Giesy, 2000. Polychlorinated naphthalenes and polychlorinated biphenyls in fishes from Michegan waters including the Great Lakes, Environmental Science & Technology, 34(4): 566-572
Kanetoshi, A., H.Ogawa, E. Katsura, H. Kaneshima & T. Miura, 1988a. Formation of polychlorinated dibenzo-p-dioxin upon combustion of commercial textile products containing 2,4,4’-trichloro-2’-hydroxydiphenyl ether Irgasan DP 300, J. Chromatogr., 442: 289-299
Kanetoshi, A., H.Ogawa, E. Katsura, H. Kaneshima & T. Miura, 1988b. Formation of polychlorinated dibenzo-p-dioxin from 2,4,4’-trichloro-2’-hydroxydiphenyl ether Irgasan DP 300 and its chlorinated derivatives by exposure to sunlight, J. Chromatogr., 454: 145-155
Kjeldsen, P. & T. Larsen, 1988. Sorption af Organiske Stoffer i Jord og Grundvand. Miljøstyrelsen. Lossepladsprojektet. 1-85. (In Danish)
Kolpin, D.W., E.T. Furlong, M.T. Meyer, E.M. Thurman,S.D. Zaugg, L.B. Barber & H.T. Buxton, 2002. Pharmaceuticals, Hormones, and Other Organic Wastewater Contaminants in U.S. Streams, 1999-2000: A National Reconnaissance, Environmental Science and Technology, 36: 1202-1211
Law, R. J., V. J. Dawes, R. J. Woodhead & P. Matthiessen, 1997. Polycyclic aromatic hydrocarbons (PAH) in seawater around England and Wales; Marine Pollution Bulletin; 34(5): 306-322
Levy, S.B., A. Roujeinikova, S. Sedlnikova, P.J. Baker, A.R. Stuitje, A.R. Slabas, D.W. Rice & J.B.Rafferty, 1999. Molecular basis of Triclosan activity, Nature 398: 383-384
Liebl, B. & S. Ehrenstorfer, 1993. Nitro musks in human milk. Chemosphere. 27: 2253-2260
Lindström, A., I.J. Buerge, T. Poiger, P.A. Bergqvist, M.D. Müller & H.R. Buser, 2002. Occurence and Environmental Behavior of the Bactericide Triclosan and Its Methyl Derivative in Surface Waters and in Wastewater, Environmental Science and Technology, 36: 2322-2329
Lucross, J., M. Alaee, D.B. Sergeant, D.M. Whittle & K.R. Solomon, 2000. Spatial and temporal distribution of polybrominated diphenyl ethers in Lake Trout from the Great Lakes. Organohalogen Compounds. 47: 73-76
McMurry, L.M., M. Oethinger & S.B. Levy, 1998. Triclosan targets lipid synthesis, Nature, 394: 531-532
Masclet, P., G. Mouvier & K. Nikolaou, 1986: Relative Decay Index and sources of PAH. Atmospheric environment 20: 439-446.
Mennear, J.H. & C.C. Lee, 1994. Polybrominated dibenzo-p-dioxins and dibenzofurans: Literature review and health assessment. Environ. Health Perspect. 102: 265-274.
Miyazaki, T., T. Yamagishi & M. Matsumoto, 1984. Residues of 4-chloro-1-(2,4-dichlorophenoxy)-2-methoxybenzene(triclosan methyl) in aquatic biota. Bull. Environ. Contam. Toxicol., 32: 416-421
Mortensen, G., B. Pedersen & G. Pritzl, 1995. Determination of Organotin Compounds in Marine Sediments Using Graphite Furnace Atomic Absorption Spectrometry. Appl. Organometal. Chem., 9: 65-73.
Muir, D. & J. deBoer, 1996. Toxaphene in the marine environment: Analysis, distribution and possible biological impacts. ICES Marine Chemistry Working Group, 1996.
Muir, D., B. Braune, B. DeMarch, R. Norstrom, R. Wagemann, L. Lockhart, B. Hargrave, D. Bright, R. Addison, J. Payne & K. Reimer, 1999. Spatial and temporal trends and effects of contaminants in the Canadian Arctic ecosystem: e review. Sci. Total Environ. 230: 83-144
Muir, D.C.G., E.W. Born, K.Koczansky & G.A. Stern, 2000b. Temporal and spatial trends of persistent organochlorines in Greenland walrus (Odobenus rosmarus rosmarus). Sci. Total Environ 245 1-3: 73-86
Müller, S., P. Schmid & C. Schlatter, 1996. Occurrence of nitro and non-nitro benzoid musk compounds in human adipose tissue, Chemosphere, 33(1): 17-28
Naes, K., E. Oug & J. Knutzen, 1998. Source and species-dependent accumulation of polycyclic aromatic hydrocarbons (PAHs) in littoral indicator organisms from Norwegian smelter-affected marine waters; Marine Environmental Research; 45(2): 193-207
Neff, J. M., 1979. Polycyclic Aromatic Hydrocarbons in the Aquatic Environment. Sources, Fates and Biological Effects. 1th edition, Essex, England, Applied Science Publishers LTD. 1-262.
Norén , K. & D. Meironyté, 2000. Certain organochlorine and organobromine contaminants in Swedish human milk in perspective of past 20-30 years. Chemosphere. 40: 1111-1123
Norstrom, R.J. & D.C.G. Muir, 1994. Chlorinated hydrocarbon contaminants in arctic marine mammals. Sci. Total Environ. 154: 107-128
Nylund, K., L. Asplund, B. Jansson, P. Jonsson, K. Litzén & U. Selström, 1992. Analyis of some polyhalogenated organic pollutants in sediment and sewage sludge. Chemosphere. 24: 1721-1730
Orvos, D.R., D.J. Versteeg, J. Inauen, M. Capdevielle, A. Rothenstein & V. Cunningham, 2002. Aquatic toxicity of triclosan, Environmental Toxicology and Chemistry, 21(7): 1338-1349
Paxéus, N., 1996. Organic Pollutants in the Effluents of Large Wastewater Treatment Plants in Sweden, Water Research, 30(5): 1115-1122
Riget, F., G. Asmund & P. Astrup, 2000. The use of lichen (Cetraria rivalis) and Moss (Rracomitrium lanuginosum) as monitors of atmospheric deposition in Greenland. SCI total environ. 245: 137-148.
Riget, F., R. Dietz, P. Møller, M. Glasius, P. Pallsbøl & K. Hobson, 2002. Population structure of West Greenland narwhals. A multidiciplinary approach. NERI Technical Report No. 400, 53 pp.
Rimkus, G.G. & M. Wolf, 1995. Nitromusk fragrances in in biota from freshwater and marine environment. Chemosphere. 30: 641-651
Rimkus, G.G. & M. Wolf, 1996. Polycyclic musk fragrances in human adipose tissue and human milk, Chemosphere, 33(10): 2030-2043
Rimkus, G.G., 1999. Polycyclic musk fragrances in the aquatic environment, Toxicology Letters, 111: 37-56
Sanderson, H., T.M. Boudreau, S.A. Mabury, W.J. Cheong & K.R. Solomon, 2002. Ecological impact and environmental fate of perfluorooctane sulfonate on the zooplankton community in indoor microcosms, Environmental Toxicology and Chemistry, 21(7): 1490-1496
Seacat, A.M., P.J. Thomford, K.J. Hansen, G.W. Olsen, M.T. Case & J.L. Butenhoff, 2002. Subchronic toxicity studies on perfluorooctanesulfonate potassium salt in cynomolgus monkeys, Toxicological Sciences, 68(1): 249-264
Sellstöm, U., A. Kierkegaard, C. de Witt, B. Jansson, 1998. Polybrominated diphenyl ethers and hexabromocyclododecane in sediment and fish from a Swedish River. Environmental Toxicology and Chemistry, 17(6): 1065-1072
Sims, R. C. & M.R. Overcash, 1983. Fate of polynuclear aromatic compounds (PNAs) in soil-plant systems. Residue Reviews. 88: 1-68
Skarphédinsdóttir, H., K. Ólafsdóttir, J. Svavarsson & T. Jóhannesson, 1996. Seasonal Fluctuations of Tributyltin (TBT) and Dibutyltin (DBT) in the Dogwhelk, Nucella lapillus (L.), and the Blue Mussel, Mytilus edulis L., in Icelandic Waters. Marine Pollution Bulletin, 32: 358-361.
Skydsbjerg, H. & D. Olsen, 1998. “Grønland 1998, Kalaallit Nunaat , Statistisk Årbog Ukiumoortumik kisitsisitigut paasissutissat.” Grønlands Statistik. ISSN 0106-22Bx ISBN:87-90393-15-5
Soni, B.G., A.R. Philp, R.G. Foster & B.E. Knox, 1998. Do flame retardants threaten ocean life?, Nature, 394, 28-29
Strand, J. & G. Asmund, 2002. Tributyltin accumulation and effects in marine molluscs from West Greenland, Environmental Pollution, submitted
US-EPA 1988. Methoxychlor. US Environmental Protection Agency, Washington, DC. Pesticide Fact Sheet No. 187, PB 89-1386531).
Verschueren, K., 1996. Handbook of Environmental Data on Organic Chemicals, 3rd ed. John Wiley & Sons, Inc.
Weber, R., B. Kuch, T. Ohno, T. Sakurai, 2002. De novo synthesis of mixed brominated-chlorinated PXDD/PXDF. Dioxins 2002, Vol. 45: 181-184.
WHO, 1989. Aldrin and Dieldrin. Environmental Health Criteria 91. International Programme on Chemical Safety.
WHO, 1991a. Chlorobenzenes other than Hexachlorobenzene. Environmental Health Criteria 128. International Programme on Chemical Safety.
WHO, 1991b. Endrin. Health and Safety Guide 60. Companion volume to Environmental Health Criteria 130. International Programme on Chemical Safety.
WHO, 1994. Polybrominated Biphenyls. Environmental Health Criteria 152. International Programme on Chemical Safety.
WHO, 1995. Tetrabromobisphenol A and Derivatives. Environmental Health Criteria 172. International Programme on Chemical Safety.
Øllgaard, H., L. Frost, J. Galster & O.C. Hansen, 1998. Survey of azo-colorants in Denmark: Consumption, use, health and environmental aspects, Danish Environmental Protection Agency. No 509. 209 pp.
1) Chapter 5. Other contaminates. 2) AMAP Greenland and the Faroe Islands. Vol. 2: The Environment of Greenland.
| Front page | | Contents | | Previous | | Next | | Top |
|