| Front page | | Contents | | Previous | | Next |
Dermal absorption of pesticides - evaluation of variability and prevention
3 Skin penetration
Skin penetration is of great importance, clinically, occupationally as well as environmentally. Many people have been and are still unintentionally exposed to toxic substances either at work or at home, such as exposure to dust, pesticides and detergents. Skin absorption (if not massive) is difficult to quantify and is therefore rarely proven to be a significant route of entry even when people experience problems after years of being exposed dermally to harmful substances.
It is therefore of great value that occupational health agencies focus on these problems and try to promote and enforce safe production methods and working conditions.
3.1 Absorption mechanism
Chemicals penetrate the stratum corneum by passive diffusion – whereas active transport plays a limited role (Scheuplein & Blank, 1971). Chemicals pass the upper skin structures into the viable epidermis and continue passively through to the dermis – the dermal-epidermal junction - where the blood vessels will transport it to the systemic circulation.
A pharmacokinetic model (Figure 2) has been made to describe the absorption through the skin. The model is linear and describes the percutaneuos absorption using three first-order rate constants.

Figure 2. Model of the absorption across the skin barrier.
k1 describes the diffusion across the stratum corneum. k2 is the transport to the viable epidermis.
k3 reflects the affinity of the penetrant for the stratum corneum versus the viable epidermis.
The figure also illustrates the potential for an accumulation of the penetrant in the stratum corneum.
The k3/k2 ratio provides the “effective partition coefficient” of the penetrant between the two layers (Guy et al., 1985). To what extent the model should also include an arrow from stratum corneum to the skin surface due to cell shedding or skin wash will be discussed in Chapter 11.
The permeability coefficient kρ depends on the solute size, lipophilicity and the diffusion path length. Even though Fick’s law describes the thickness of the skin as having influence on the penetration and Scheuplein and Blank (1971) indicate the skin thickness as being the controlling factor in skin penetration, Elias et al. (1981) were unable to determine any association between absorption and neither the thickness nor the number of cell layers in the skin. There were indications that the intercellular lipids were important factors in the regulation of epidermal permeability (Elias, 1981;Elias, 1983). Later works also show that penetration depends more on the lipid composition than on the skin thickness. Even though different sites have the same thickness or lipid content it does not mean that they by definition have the same penetration rate, all due to day-to-day structure variations (Bronaugh & Maibach, 1985).
For a substance to be transdermally absorbed some key events must take place:
- The substance interacts with the stratum corneum.
- Diffusion of the substance through the stratum corneum.
- Crossing from the lipophilic stratum corneum to the more aqueous viable epidermis.
- Continuing from the avascular epidermis to the highly perfused dermal tissue.
- Uptake through the microcirculation to the systemic circulation
(Clark NWE, 1992;Guy et al., 1987;Kao et al., 1988).
When the substance has to pass the stratum corneum it generally has two pathways in humans: a) Transcellular and b) Intercellular (Figure 3), the major route being the intercellular pathway between the corneocytes, implying that stratum corneum lipids play an important role in the skin barrier function (Cnubben et al., 2002;Elias, 1981;Michales AS et al., 1975). However, for very lipophilic and large molecules (and some electrolytes) the appendages and other diffusion shunts may also play an important role (Kao et al., 1988).
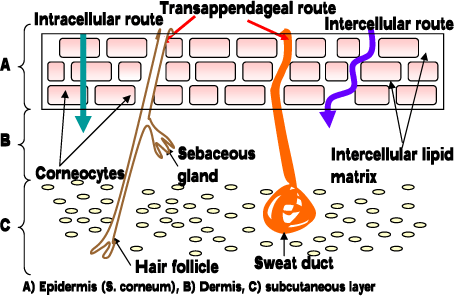
Figure 3. Pathways through the skin.
3.2 Absorption kinetics
A skin penetrating substance first has to pass the avascular and lipophilic structure (stratum corneum) and continue through a more aqueous layer (lower epidermis and dermis) to the blood vessels. The permeability coefficient of the substance increases as its lipophilicity increases (Roy & Flynn, 1989). A lipophilic compound will easily cross the stratum corneum but the penetration rate will decrease as it reaches the hydrophilic epidermis and the diffusion of the substance will slow down. The consequence is a temporary deposition within the skin. This process is called a reservoir effect and will be described and discussed later. Substances soluble in the lipophilic layer as well as in the more aqueous structures and at the same time, having a small molecular size have the best permeability through the skin barrier (Guy et al., 1987). Electrolytes on the other hand are difficult to absorb when they are applied in aqueous solutions. Ions create a field of stable hydration that increases the size of the diffusing component (Grandjean P, 1990).
3.2.1 Fick’s first law
Fick’s first law of diffusion only applies under very specific conditions. It will, however, give a good approximation of flux rates related to dermal penetration (Grandjean P, 1990).
JSS = kP * ΔC
JSS = flux of penetrant molecule under steady-state conditions (absorption rate). k? =permeability coefficient of the penetrant through the membrane and ΔC = concentration gradient across the membrane.
The determination of the permeability coefficient (Figure 4) may also be calculated from:
kP = JSS /A * ΔC = K * D /h
A = application area (Franz cell opening). K = the skin/vehicle partition coefficient of a solution. D = apparent diffusion coefficient. h = the diffusional path length. Since all but KP are known parameters, KP may be calculated.
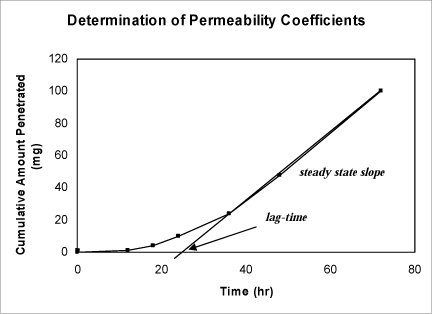
Figure 4. Graph showing how to determine steady state flux (used to calculate the permeability coefficient) and lag-time.
The steady-state flux, JSS,is the slope of the linear part of the graph of the cumulative amount penetrated as a function of time. The lag time is the time intercept of the linear portion of the graph.
3.2.2 Prediction of permeation
Over the years many calculations have been made to predict the permeability coefficient by a simple model based on the molecular weight MW of the chemical. The estimation of kp has been based on data on mainly hydrocarbons, since for hydrocarbons, the ratio of molecular weight to molecular volume is nearly constant and therefore a kp estimate based on molecular weight is as good as one based on molecular volume and it has shown that there is evidence that maximal flux and penetration decrease exponentially with molecular weight (Kasting GB et al., 1987;Potts & Guy, 1992). In 1992 Kasting developed an equation based on the theory that solute transport could follow a polar pathway with a permeability coefficient kp,polar as well as follow an intercellular lipid pathway with a permeability coefficient kp,lipid, even though the existence of a polar pathway still remains controversial. For lipophilic solutes, an aqueous layer is likely to be permeable at the stratum corneum epidermis interface with a kp,aqueous. Hence,
kp = [1/(kp,lipid +kp,polar) + 1/kp,aqueous]-1 (Kasting GB et al., 1992)
Kasting et al considered the range for kp,polar to be 10-5 to 10-6 (300/MW)½ cm/h and kp,aqueous ~ 0.15 x (300/MW)½, the second being comparable to permeability of solutes through delipidized stratum corneum.
For most solutes:
kp,lipid >> kp,polar and kp,lipid << kp,aqueous,
so that kp ~ kp,lipid. (Kasting GB et al., 1992)
Usually, solute lipophilicity favours skin permeability with the diffusivity of the solute being higher for solutes with less affinity to bind to hydrogen.
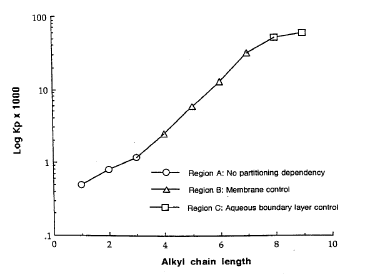
Figure 5. Consequence of the substance lipophilicity on the rate of skin penetration. (Figure from Roberts and Walters)(Roberts MS & Walters KA, 1998a): Average permeability results for aqueous alcohol solutions through the stratum corneum of human skin as a function of alcohol chain length (Scheuplein and blank 1973).
The equation from Kasting et al determinates the kp,lipidby using the molecular weight and the octanol-water partition coefficient:
log kp,lipid= log koct – (0.018/2.303)*MW – 2.87 (Kasting GB et al., 1992)
In 1992 Potts and Guy came up with a similar equation assuming a single pathway through a multiple regression of 97 solutes defined by Flynn (Flynn GL, 1990):
log kp = 0.71*log koct – 0.0061*MW – 2.72 (Potts & Guy, 1992)
For dense compounds it was found that the molecular weight was a larger relative to molecular volume than for hydrocarbons and the kp value calculated from an equation using molecular weight showed underestimation of the kp(Vecchia BE & Bunge AL, 2003).
The major limitations of models predicting the permeability coefficient are that the models are based on permeation from simple aqueous solutions where no physiological factors or formulation effects are considered.
3.2.3 Octanol-water partition coefficients
As mentioned above, the physicochemical constant log koct is used to describe the lipophilicity of the penetrant. koct is the octanol-water partition coefficient, defined as ratio of the equilibrium concentrations of the penetrant in a two-phase system consisting of two immiscible solvents, (octanol and water).
koct = Coctanol / Cwater
The partition coefficient is given as its logarithm to base ten, meaning that a high log koct value indicates a high lipophilicity (Clark NWE, 1992) and by that a qualitative indicator of penetration (Potts & Guy, 1992). Substances with high lipophilicity tend to remain in a reservoir in the lipophilic part of the skin. It is a matter of balance. A large amount of absorption is association with log koctvalues of 1 to 2 and decreasing considerably when exceeding 3.5 (ECETOC, 1993)
3.2.4 Lag-time
Lag-time is the time from the penetrant is applied to the skin surface (the start of the exposure) until it is possible to detect the substance on the other side of the skin. The importance of knowing the lag-time is illustrated in Figure 6 which shows the penetration of a test-substance in two different formulations (mixed with different detergents). It shows that the total amount of substance penetrated is the same, but the lag-time and flux are different.
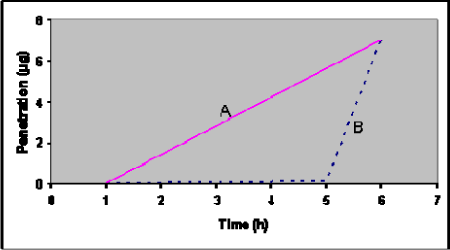
Figure 6: Theoretical penetration curves for two chemicals (A and B) with identical total penetration after 6 hours, but different lag-time and flux.
Some substances penetrate the skin slower than expected due to binding. The skin may act as a reservoir (see below) because of some substances’ physicochemical properties (Vickers, 1972). The substance will deposit in the epidermis thereby delaying the systemic effect. This phenomenon also controls the lag time. Lipid solubility is a predictor of the drug’s solubility in the skin, and with greater lipid solubility a larger deposition, longer lag time and prolonged elimination behaviour will be expected (Guy et al., 1985;Knepp et al., 1987;Plezia et al., 1989;Roy & Flynn, 1989;Roy et al., 1994;Scheuplein & Blank, 1971).
Lack of knowledge about the lag-time of different pesticides makes assessment of exposure and risk difficult. There is a risk of underestimation or completely overlooking an ongoing or recent exposure. A lag-time of 4 hours does not mean that the pesticide is still on the skin surface after 4 hours before the penetration starts, but reflects the fact that penetration takes time and depends on the penetrant. The passive absorption is a multi-step process. Initially there is absorption from the often hydrophilic donor phase on the skin surface. This is accompanied by diffusion through the skin with a temporary deposition (reservoir effect) and at the end penetration to the receptor. The passive diffusion from the often hydrophilic donor and into the skin is favoured by the lipophilicity of the substance (Nielsen et al., 2004).
3.2.5 Reservoir effect
When a substance stays and accumulates in the skin instead of passing directly through to the bloodstream it is described as a reservoir. A reservoir can be present in the stratum corneum, the viable epidermis or in the dermis (Roberts et al., 2004). The substance staying in the reservoir will often be released with a certain “delay” to the blood stream or maybe back to the skin surface, a possible option that will be discussed later in Chapter 11. The absorption of the substance into the blood stream continues from the application site at a gradually declining pace, giving the appearance of prolonged elimination (Lee FW, 2000) (Cnubben et al., 2002). The reservoir effect can be induced by occlusion with plastic film, but it will depend on the substance, the temperature of the skin and the humidity (Vickers, 1972). When studying the reservoir effect the flow-through system is useful (see below). Studies have shown that absorption from washed skin after end of application in an in vivo study on rats continued for almost all pesticides (Zendzian, 2003). Yet another study showed the importance of tracking down chemicals remaining in the skin. In 2004, Yourick et al. concluded in a flow-through study investigating the penetration of three substances that two of the substances made reservoir in the epidermis, and by that the substance should not be considered as absorbed material. Data from the last substance, however, indicated that the substance was spread throughout the epidermis and dermis and therefore could not be excluded from the absorbed dose (IPCS, 2006). In the present studies we will try to evaluate the effect of washing an exposed skin area to avoid absorption of a pesticide.
| Front page | | Contents | | Previous | | Next | | Top |
Version 1.0 May 2009, © Danish Environmental Protection Agency
|